Abstract
Free full text

Despite vaccination, China needs non-pharmaceutical interventions to prevent widespread outbreaks of COVID-19 in 2021
Abstract
COVID-19 vaccination is being conducted in over 200 countries and regions to control SARS-CoV-2 transmission and return to a pre-pandemic lifestyle. However, understanding when non-pharmaceutical interventions (NPIs) can be lifted as immunity builds up remains a key question for policy makers. To address this, we built a data-driven model of SARS-CoV-2 transmission for China. We estimated that, to prevent the escalation of local outbreaks to widespread epidemics, stringent NPIs need to remain in place at least one year after the start of vaccination. Should NPIs alone be capable of keeping the reproduction number (Rt) around 1.3, the synergetic effect of NPIs and vaccination could reduce the COVID-19 burden by up to 99% and bring Rt below the epidemic threshold in about 9 months. Maintaining strict NPIs throughout 2021 is of paramount importance to reduce COVID-19 burden while vaccines are distributed to the population, especially in large populations with little natural immunity.
Main
The novel coronavirus disease 2019 (COVID-19) pandemic is far from over, with cases still surging in many countries across the globe, particularly with India suffering from a catastrophic second wave1. In 2020, epidemic suppression and/or mitigation have relied on non-pharmaceutical interventions (NPIs), including social distancing, school closure, mask use and case isolation. Although effective and widely adopted to limit severe acute respiratory syndrome coronavirus 2 (SARS-CoV-2) transmission and reduce COVID-19 burden, these interventions entail enormous economic costs and negatively affect quality of life2. Additionally, in many countries, relaxation of NPIs has led to a resurgence of the epidemic as herd immunity has not been reached thus far3.
Effective vaccines against COVID-19 remain the only foreseeable means of both suppressing the infection and returning to pre-pandemic social and economic activity patterns. Globally, several vaccines have been licensed, and vaccination programmes have been initiated in more than 200 countries/regions, including China4. However, the projected global production and delivery capacities are likely to be inadequate to provide COVID-19 vaccines to all individuals who are still susceptible to SARS-CoV-2 infection3. The effectiveness of COVID-19 vaccination campaigns will depend on several factors, including pre-existing immunity, vaccine supply, willingness to receive the vaccine and strategies for vaccine allocation and deployment5.
To avoid widespread transmission of SARS-CoV-2, since the end of the first COVID-19 wave in the spring of 2020, China has implemented strict NPIs and has successfully controlled local outbreaks, preventing a second widespread wave of COVID-19. Since December 2020, China has given conditional approval or emergency use approval for seven COVID-19 vaccines. As of 1 June 2021, 681.9 million doses (roughly corresponding to 24.3% of the population) have been administered6. However, such a coverage is still extremely low, and thus China remains highly vulnerable to importations of SARS-CoV-2 and onward transmission, as proved by several local outbreaks that occurred in the first four months of 2021, the largest of which occurring in Heilongjiang Province led to 636 reported cases and spilled over to a neighbouring province (over 300 cases were reported in Jilin)7. At present, estimating whether and when NPIs can be lifted, and the extent to which we need to rely on NPIs while vaccines roll out, represents a top priority for policy making.
This question has not been well addressed in China, one of the few countries in the world where nearly the entire population is still susceptible to SARS-CoV-2 infection and home to almost 1.4 billion individuals (roughly 18% of the world population). To fill this gap, we built on top of the wide body of work adopting mathematical models of the infection transmission process to evaluate vaccination programmes8–12. In particular, we developed an age-structured stochastic model to simulate SARS-CoV-2 transmission triggered by cases imported in Mainland China, based on a susceptible–infectious–removed (SIR) scheme (Supplementary Fig. 1). We consider a situation with (i) no ongoing widespread SARS-CoV-2 transmission, (ii) nearly no immunity in the population and (iii) high risk of importing SARS-CoV-2-infected individuals, possibly leading to an upsurge of COVID-19 cases. Since COVID-19 vaccines are expected to continue rolling out throughout 2021–2022, we consider also alternative scenarios where SARS-CoV-2 infections leading to an outbreak are imported when 10%, 20% (close to the coverage as of the end of May 2021) and 30% of the Chinese population has already been vaccinated (according to the simulated vaccination programme).
In the model, we account for heterogeneous mixing patterns by age13 and progressive vaccine deployment among different population segments based on a priority scheme (essential workers, older adults, individuals with underlying conditions, etc.)14. Further, we overlay a disease burden model on the transmission model to estimate the number of symptomatic cases, hospitalizations, intensive care unit (ICU) admissions and deaths under different vaccination scenarios and based on empirical data15–20. The resulting integrated model is informed by data on COVID-19 natural history, age-mixing patterns specific to China quantified during the pre-pandemic period and the size of the different vaccination targets in the Chinese population (for example, individuals with pre-existing conditions). A qualitative model description is reported in Methods section, a summary of model parameters and data sources is reported in Supplementary Table 1 and all other details are reported in Supplementary Files 1–5.
The combined effects of NPIs and vaccination programmes are evaluated in terms of their ability to reduce the disease burden caused by outbreaks arising from possible importation of cases.
We considered a baseline vaccination scenario where: (1) vaccination starts 15 days after an outbreak triggered by 40 breakthrough imported SARS-CoV-2 infections; (2) vaccine efficacy (VE) against SARS-CoV-2 infections for a two-dose schedule (with a 21-day interval) is set at 80%21; (3) vaccination coverage is capped at 70% across all ages14; (4) 6 million doses are administered daily (4 per 1,000 individuals, informed by the ongoing COVID-19 vaccination programme6, and estimates of vaccine supply till 2021 in China22–26); (5) the first priority target consists of older adults and individuals with underlying conditions (descriptions in detail shown in Supplementary Table 2); (6) there is no prior population immunity from natural infection, which aligns with the situation in most of China, where there has been little circulation of SARS-CoV-2 as of May 2021 (ref. 3); (7) we assume an initial reproductive number Rt=
2.5 at the start of the outbreak27–32, in the absence of NPIs and vaccination; (8) children under 15 years of age were considered to have a lower susceptibility to SARS-CoV-2 infection as compared with adults (that is, individuals aged 15–64 years), while individuals aged 65+ years had the highest susceptibility to infection (Supplementary Table 1)33,34; (9) we let the model run for 2 years. To evaluate the impact of the baseline assumptions on our results, we conduct comprehensive sensitivity analyses.
Results
Main analysis
In the absence of NPIs, the vaccination programme is too slow to lower and delay the epidemic (Fig. (Fig.1a)1a) and does not effectively reduce COVID-19 burden. Rt falls below the epidemic threshold (<1) 69 days after the epidemic start (Fig. (Fig.1b),1b), but this is primarily attributable to immunity gained through natural infection rather than vaccination. Indeed, in this time frame, 52.2% of population gets infected, while only 6.7% of population has been vaccinated (Fig. (Fig.1c).1c). The cumulative number of symptomatic cases and deaths over a 2-year period only decrease by 3.3% (95% CI 3.1–4.7%) and 6.7% (95% CI 4.5–8.9%), respectively, as compared with a reference scenario where there is no vaccination and no NPIs, which would lead to 306.7 million (95% CI 282.7–320.6 million) symptomatic cases, 99.3 million (95% CI 92.6–104.5 million) hospitalizations, 7.2 million (95% CI 6.0–7.8 million) ICU admissions and 9.4 million (95% CI 7.7–10.3 million) deaths (Fig. (Fig.22).

a, Number of symptomatic cases over time as estimated in the no-NPIs scenario (initial Rt=
2.5) in the absence or presence of vaccination. b, Net reproduction number Rt over time, as estimated using a Bayesian framework (Supplementary File 5) from the time series of symptomatic cases in the no-NPIs scenario in the presence of vaccination. The horizontal line indicates the epidemic threshold Rt
=
1, and the vertical line indicates where Rt crosses this threshold. Note that, for the first few generations of cases, Rt shows an increasing pattern linked to the highly stochastic nature of epidemics in their initial phase when epidemics with initially larger Rt are more likely to survive69. For the same reason, the adopted methodology tends to overestimate Rt in the epidemic tail69; as such, Rt is shown in the core part of the epidemic only. c, Absolute numbers and proportion of the Chinese population infected and vaccinated over time in the no-NPIs scenario in the presence of vaccination. The population of China in 2020 is 1,439,324,000 (ref. 57). d–f, As in a–c but for the mild NPIs scenario (initial Rt
=
1.5). g–i, As in a–c but for the moderate NPIs scenario (initial Rt
=
1.3). j–l, As in a–c but for the strong NPIs scenario (initial Rt
=
1.1). Line denotes median, and shadow denotes quantiles 0.025 and 0.975.

a, Cumulative number of symptomatic cases as estimated under the different scenarios in the absence or presence of vaccination over the simulated 2-year period. No vaccination + no NPIs with Rt=
2.5 at the beginning of the outbreak is called the ‘reference scenario’, described using dark orange bars. Light-yellow bars indicate scenarios including vaccination and/or different levels of NPIs. b, Reduction in the cumulative number of symptomatic cases with respect to the reference scenario. Orange bars and values written in black indicate the contribution of NPIs; blue bars and values written in black indicate the overall contribution of vaccination and NPIs, while the values written in white indicate net contribution of vaccination. c,d, As in a,b but for hospitalized cases. e,f, As in a,b but for cases admitted to ICU. g,h, As in a,b but for deaths. Number denotes median, and error bars denote quantiles 0.025 and 0.975.
Provided that NPIs are in place and can keep Rt at 1.3 in the absence of vaccination (‘moderate NPIs scenario’), the vaccination programme could reduce COVID-19 burden by about 99% compared with the ‘reference scenario’, with 5.5 million (95% CI 2.5–13.4 million) symptomatic cases, 1.8 million (95% CI 0.8–4.4 million) hospitalizations, 73,500 (95% CI 7,300–152,100) ICU admissions and 76,700 (95% CI 8,200–165,700) deaths (Fig. (Fig.2).2). In this context, vaccination decreases COVID-19 burden by about 40% (Fig. (Fig.2)2) compared with a situation with moderate NPI alone, and Rt falls below the epidemic threshold about 9 months after the epidemic start (Fig. (Fig.1h).1h). At the time that Rt falls below 1, we estimate that 50.8% of the total population would have been vaccinated, while 0.8% would have been naturally infected (Fig. (Fig.1i).1i). This highlights that a relevant proportion of the population would still be susceptible to SARS-CoV-2 at that time. Although in the long term vaccination can ultimately lead to the suppression of transmission, it is necessary to maintain NPIs for one year after the onset of vaccination. Indeed, if NPIs are relaxed from moderate (Rt=
1.3) to mild (Rt
=
1.5) 9 months after vaccination start, the cumulative number of symptomatic cases could double (Extended Data Figs. Figs.11 and and2),2), and the cumulative death toll could increase from 76,700 to 173,000 (Extended Data Fig. Fig.3).3). In contrast, a small increase in cumulative deaths from 76,700 to 81,700 is expected if this relaxation occurs one year after vaccination start (Extended Data Fig. Fig.3),3), while earlier or more drastic relaxations of NPIs lead to substantial increases in deaths (Extended Data Fig. Fig.33).
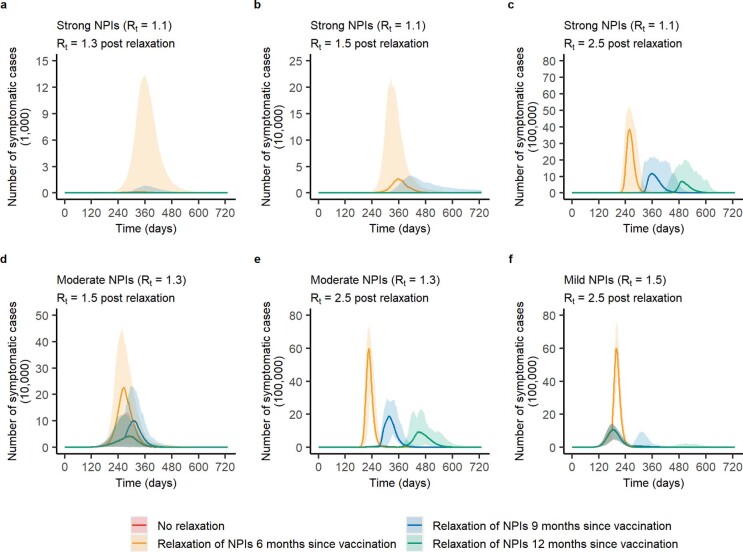
a-c, For scenario with strong NPIs (Rt=
1.1), we increment the value of Rt to 1.3, 1.5, 2.5 after 6, 9, and 12 months since vaccination, respectively; d-e, for Rt
=
1.3, we increment the value of Rt to 1.5 and 2.5 after 6, 9, 12 months, respectively; f, for Rt
=
1.5, we increment the value of Rt to 2.5 after 6, 9, 12 months, respectively. Line denotes median, and shadow denotes quantiles 0.025 and 0.975. Note that the red line is barely visible as it is almost entirely overlapping with the green one.

a-c, For scenario with strong NPIs (Rt=
1.1), we increment the value of Rt to 1.3, 1.5, 2.5 after 6, 9, and 12 months since vaccination, respectively; d-e, for Rt
=
1.3, we increment the value of Rt to 1.5 and 2.5 after 6, 9, 12 months, respectively; f, for Rt
=
1.5, we increment the value of Rt to 2.5 after 6, 9, 12 months, respectively. Number denotes median, and error bars denote quantiles 0.025 and 0.975.
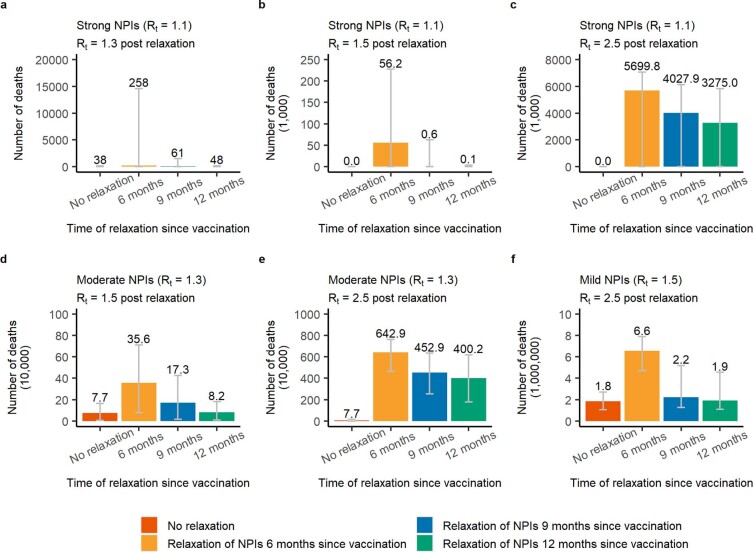
a-c, For scenario with strong NPIs (Rt=
1.1), we increment the value of Rt to 1.3, 1.5, 2.5 after 6, 9, and 12 months since vaccination, respectively; d-e, for Rt
=
1.3, we increment the value of Rt to 1.5 and 2.5 after 6, 9, 12 months, respectively; f, for Rt
=
1.5, we increment the value of Rt to 2.5 after 6, 9, 12 months, respectively. Number denotes median, and error bars denote quantiles 0.025 and 0.975.
A combination of more stringent NPIs (that is, capable of keeping Rt=
1.1) and vaccination (‘vax
+
strong NPIs’ scenario) could suppress the epidemic, with <2,300 symptomatic cases, and <50 deaths on average. Although the majority of the reduction of COVID-19 burden is ascribable to NPIs in this case (over 85%), the deaths averted due to vaccination are about 1.2 million (Figs. 1j–l and and22).
If we consider a set of mild NPIs (‘vax+
mild NPIs’ scenario), even a relatively low initial reproduction number under NPIs of Rt
=
1.5 could still lead to a disastrous epidemic, with nearly 2 million deaths. Despite the high death toll of the resulting epidemic, NPIs and vaccination would jointly reduce around 80% of the disease burden compared with a scenario with no NPIs and no vaccination (namely, 239 million symptomatic cases and 8.2 million deaths averted) (Figs. 1d–f and and22).
Vaccine distribution capacity
Should the daily vaccination rollout be limited to 1.3 million doses (1 per 1,000 individuals, a slower rate than during the 2009 H1N1 pandemic), vaccination would not effectively reduce COVID-19-related deaths unless there was adoption of stringent NPIs. In a scenario where vaccination capacity reaches 10 million doses administered per day (7 per 1,000 individuals), vaccination would reduce COVID-19-related deaths to <5,000 for moderate NPIs and <30 for strong NPIs. Should the daily vaccination capacity be increased to 15 million doses (10 per 1,000 individuals), vaccination could effectively reduce deaths to <100,000 (similar to the annual influenza-related death toll in China35) even in the presence of mild NPIs. However, even if the daily vaccination capacity could be increased to 30 million doses (20 per 1,000 individuals), in the absence of NPIs, we estimate that over 7.7 million deaths would still occur (Fig. (Fig.3).3). Similar patterns are estimated for the number of symptomatic cases, hospitalizations and ICU admissions (Extended Data Fig. Fig.44 and Supplementary Figs. 2 and 3).
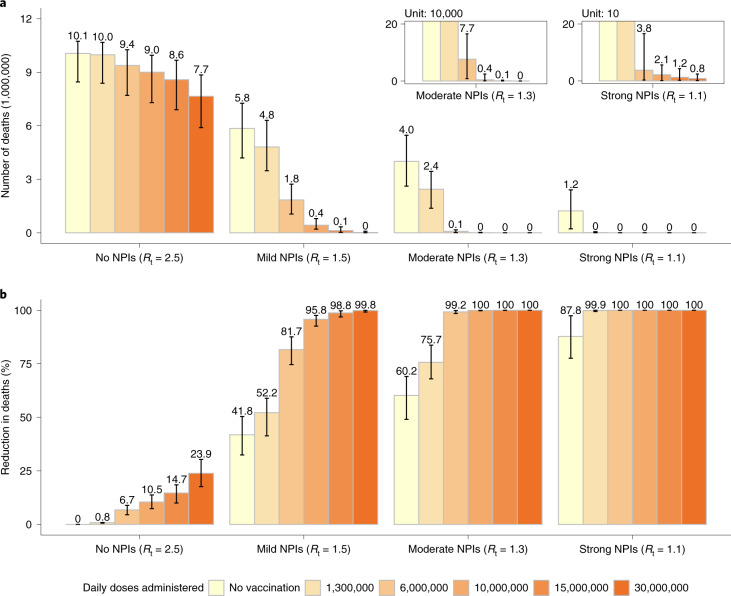
a, Cumulative number of COVID-19 deaths (millions) as estimated in the different scenarios under progressively increasing values of the daily vaccination capacity. b, Proportion of deaths averted compared with the reference scenario, that is, no vaccination+
no NPIs with Rt
=
2.5 at the beginning of the outbreak. Number denotes median, and error bars denote quantiles 0.025 and 0.975.

a, Cumulative number of COVID-19 symptomatic cases as estimated in the different scenarios under progressively increasing values of the daily vaccination capacity; b, Proportion of symptomatic cases averted compared to the reference scenario, that is, no vaccination + no NPIs with Rt=
2.5 at the beginning of the outbreak. Number denotes median, and error bars denote quantiles 0.025 and 0.975.
Increasing daily vaccination capacity could largely shorten the time needed to control SARS-CoV-2 transmission. For instance, when considering a daily capacity of 10 million and 15 million doses and moderate NPIs, Rt would drop below 1 about 8 and 6 months, respectively, after epidemic onset (compared with the 9.3 months estimated with the baseline capacity of 6 million doses). At that time, over 60% of the population would be vaccinated and ≤0.1% would be naturally infected. An upscale in the daily capacity to 10 (Extended Data Figs. Figs.55 and and6)6) or 15 million doses (Extended Data Figs. Figs.77 and and8)8) would allow a relaxation of NPIs from moderate to mild already 6–9 months after vaccination start, that is, 3–6 months earlier with respect to the baseline. On the other hand, more drastic relaxations of NPIs (for example, from moderate to no NPIs) would still lead to substantial increases in symptomatic cases and deaths (Extended Data Figs. 5–8).

a-c, For scenario with strong NPIs (Rt=
1.1), we increment the value of Rt to 1.3, 1.5, 2.5 after 6, 9, and 12 months since vaccination, respectively; d-e, for Rt
=
1.3, we increment the value of Rt to 1.5 and 2.5 after 6, 9, 12 months, respectively; f, for Rt
=
1.5, we increment the value of Rt to 2.5 after 6, 9, 12 months, respectively. Line denotes median, and shadow denotes quantiles 0.025 and 0.975. Note that the red line is barely visible as it is almost entirely overlapping with the green one.
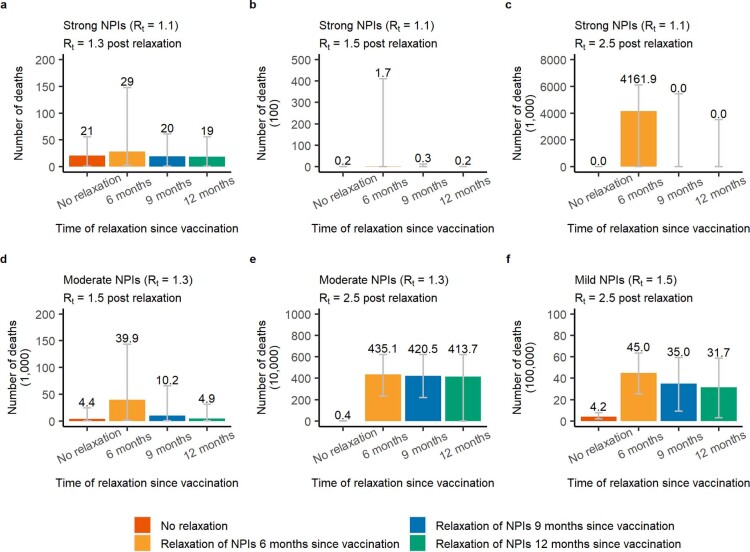
a-c, For scenario with strong NPIs (Rt=
1.1), we increment the value of Rt to 1.3, 1.5, 2.5 after 6, 9, and 12 months since vaccination, respectively; d-e, for Rt
=
1.3, we increment the value of Rt to 1.5 and 2.5 after 6, 9, 12 months, respectively; f, for Rt
=
1.5, we increment the value of Rt to 2.5 after 6, 9, 12 months, respectively. Number denotes median, and error bars denote quantiles 0.025 and 0.975.

a-c, For scenario with strong NPIs (Rt=
1.1), we increment the value of Rt to 1.3, 1.5, 2.5 after 6, 9, and 12 months since vaccination, respectively; d-e, for Rt
=
1.3, we increment the value of Rt to 1.5 and 2.5 after 6, 9, 12 months, respectively; f, for Rt
=
1.5, we increment the value of Rt to 2.5 after 6, 9, 12 months, respectively. Line denotes median, and shadow denotes quantiles 0.025 and 0.975. Note that the red line is barely visible as it is almost entirely overlapping with the green one.

a-c, For scenario with strong NPIs (Rt=
1.1), we increment the value of Rt to 1.3, 1.5, 2.5 after 6, 9, and 12 months since vaccination, respectively; d-e, for Rt
=
1.3, we increment the value of Rt to 1.5 and 2.5 after 6, 9, 12 months, respectively; f, for Rt
=
1.5, we increment the value of Rt to 2.5 after 6, 9, 12 months, respectively. Number denotes median, and error bars denote quantiles 0.025 and 0.975.
Vaccination prioritization
We consider alternative vaccination scenarios that prioritize essential workers (staff working in healthcare, law enforcement, security and community services, individuals employed in cold chain, etc.) to maintain essential services and then explore different prioritization strategies for the rest of the population. Our results suggest that the relative timing of the epidemic and of the vaccination rollout play a key role in determining the most effective strategy. In particular, if we consider vaccination to start two weeks after 40 cases are detected, there is no clear prioritization strategy that minimizes deaths, as the outcome of the vaccination campaign depends heavily on the timing at which the epidemic unfolds (Fig. (Fig.44 and Supplementary Figs. 4 and 5). Instead, if the epidemic is already underway when the vaccination campaign starts (>5,000 cases), prioritizing working-age groups minimizes the number of deaths when Rt≤
1.3. In contrast, prioritizing older adults and individuals with underlying conditions is more effective when Rt
≥
1.5 (direct benefits are higher; Fig. Fig.44 and Supplementary Figs. 4 and 5). Two results are independent of the adopted prioritization strategy: (i) if Rt
≥
1.5, then an epidemic cannot be avoided, and (ii) when Rt
=
1.1, over 99% of deaths can be averted (Supplementary Figs. 4 and 5).
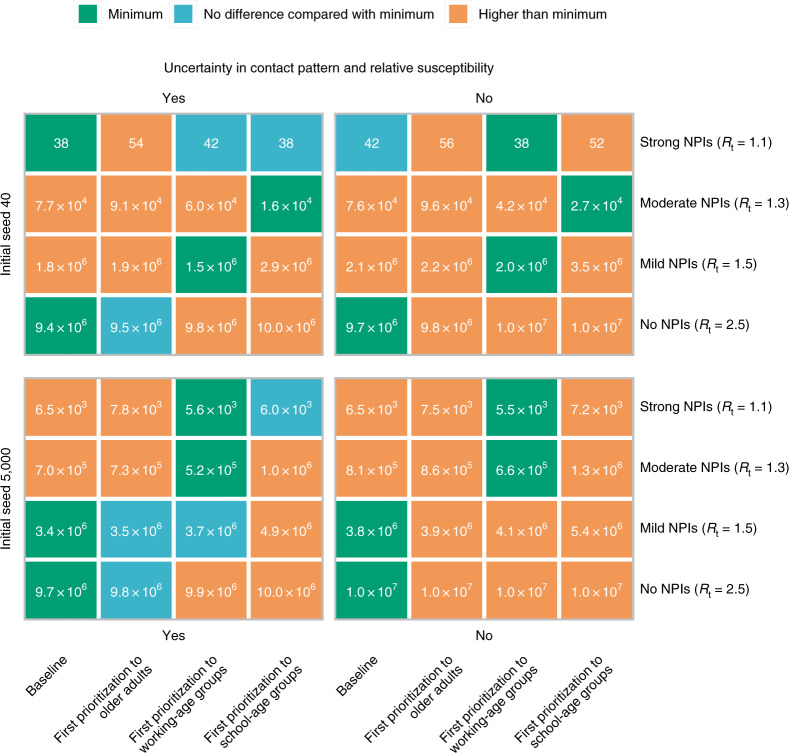
Initial cases denote breakthrough COVID-19 cases, which initiate the epidemic. We consider the impact of uncertainty (‘Yes’, which means 200 stochastic model realizations are performed to account for the ranges) in contact patterns and relative susceptibility on prioritization, and use their mean values as well (‘No’). Baseline denotes first prioritizing older adults and individuals with underlying conditions. Number in the box denotes the death toll (median). Minimum denotes the lowest deaths in each scenario on the basis of median value. We compare other strategies with the one with minimum deaths using a rank-sum test. For example, in the context of 5,000 initial cases, Rt=
1.5 and using mean values of contact patterns and relative susceptibility, the baseline is the optimal strategy to minimize deaths.
Population immunity at the onset of an outbreak
In December 2020, vaccination started in China, while measures to detect imported cases and case surveillance are in place. The number of doses distributed per day has changed over time, following an increasing trend and with a daily average of about 6 million doses over the period between late March and mid May 2021 (Supplementary File 7). As of 1 June 2021, the vaccination coverage is about 24.3%6. The effectiveness of the vaccination programme and NPIs in preventing new COVID-19 outbreaks and limiting COVID-19 burden will thus depend on the level of vaccine-induced immunity in the population should an outbreak of locally transmitted cases start to unfold.
To simulate this situation, we initialize the model by considering different fractions of vaccinated population (SA1: 10%; SA2: 20%; SA3: 30%) at the time the infection is seeded. Given a certain level of NPIs in the absence of immunity, increasing proportions of vaccinated individuals will decrease the effective reproduction number (for example, Rt=
1.1 in the absence of immunity corresponds to Rt below the epidemic threshold if 10% or more of the population has been vaccinated).
Should 30% of the population already have been vaccinated before the start of a new wave, continuing the vaccination programme while adopting mild NPIs would reduce the death toll by 98% (42,400 deaths as compared with 1.8 million if no one was vaccinated). However, in the absence of NPIs, even if 30% of population were already vaccinated before the start of a new wave, carrying on with the vaccination programme alone would not be enough to prevent a widespread epidemic leading to 6 (95% CI 4–7) million deaths (Fig. (Fig.55).

In this additional analysis, the epidemic starts when 10%, 20% or 30% of population has been vaccinated. a–d, Cumulative number of symptomatic cases (a), hospitalizations (b), cases admitted to ICU (c) and deaths (d) as estimated over the simulated 2-year period. Number denotes median, and error bars denote quantiles 0.025 and 0.975.
Vaccination coverage
No significant difference in willingness to vaccinate between age groups has been reported in China36–38. Accordingly, we use a homogeneous vaccine coverage of 70% among all age groups in the main analysis. Here, we present the results of a set of sensitivity analyses assuming vaccination coverage of 50% (SA4) and 90% (SA5) among all age groups, and considering heterogeneous coverage by age: (1) 70% for adults ≥20 years and 50% for younger individuals (SA6), (2) 90% for adults ≥20 years and 70% for younger individuals (SA7) and (3) 70% for adults ≥20 years and no vaccination for younger individuals (SA8).
By considering moderate NPIs (Rt=
1.3) and vaccination coverage of 50% in all age groups, the number of symptomatic individuals is estimated to decrease by 33% with an 8% decrease of the death toll with respect to the baseline vaccination scenario (70% coverage) (Supplementary Figs. 6 and 7). In fact, the lower coverage in older age groups would lead to an earlier vaccination in younger age groups who are characterized by the highest contact rates13. Conversely, increasing the coverage to 90% would decrease the death toll but lead to an increase of symptomatic cases (Supplementary Figs. 6 and 7). It is important to remark that these results consider that moderate NPIs remain in place over the entire duration of the epidemic, and they do not provide indications of the coverage needed to suppress any further resurgences of cases.
As compared with the baseline vaccination scenario (70% vaccination coverage in all age groups), if the vaccine is not distributed among individuals aged less than 20 years, we estimate an increase of the death toll of 55% and of symptomatic infections of 69% (Supplementary Figs. 6 and 7). The scenario assuming 90% vaccination coverage for adults ≥20 years and 70% for younger individuals leads to a lower reduction of COVID-19 burden as compared with the baseline vaccination scenario (Supplementary Figs. 6 and 7). The higher coverage in the adult population results in a delayed start of vaccination of the young population, which is characterized by higher contact rates13. Nonetheless, it is important to remark that this result depends on the timing of the introduction of the initial seeds (see Population immunity at the onset of an outbreak section).
Vaccine efficacy
Three types of COVID-19 vaccine are currently in use in China, including inactivated, recombinant protein subunit and adenovirus-vectored vaccines. VE for these vaccines ranges from 65% to 90%, with the exception of the one tested in Brazil where P1 variant is prevalent (VE 50%)39–43. With respect to an 80% VE adopted in the baseline scenario, by considering VE of 60% (SA9), we estimate a 1.64-fold increase of the death toll and 1.02-fold increase of symptomatic cases, whereas a 37% and 29% decrease of deaths and symptomatic cases, respectively, is estimated for VE of 90% (SA10). (Supplementary Figs. 6 and 7)
SARS-CoV-2 variants
Multiple SARS-CoV-2 variants have been documented globally, three of which are of particular concern: lineage B.1.1.7 identified in the United Kingdom, B.1.351 in South Africa and P.1 in Brazil44. These variants are estimated to have higher transmissibility45,46 and possibly increased mortality47,48. To assess the effect of vaccination in this context, we consider higher values of Rt (that is, 1.7, 1.9 and 2.1, about 30–60% increased transmissibility with respect to the main analysis45) to account for enhanced transmissibility, and use a mean death hazard ratio of 1.64 to account for higher mortality47. With the assumption of VE of 80% against the new variant, COVID-19 burden substantially increases compared with the scenario based on the historical lineage. The number of symptomatic cases increases from 2,000 to 173 million, and deaths increase from <50 to 7 million even when strict NPIs are implemented (Extended Data Fig. Fig.99).
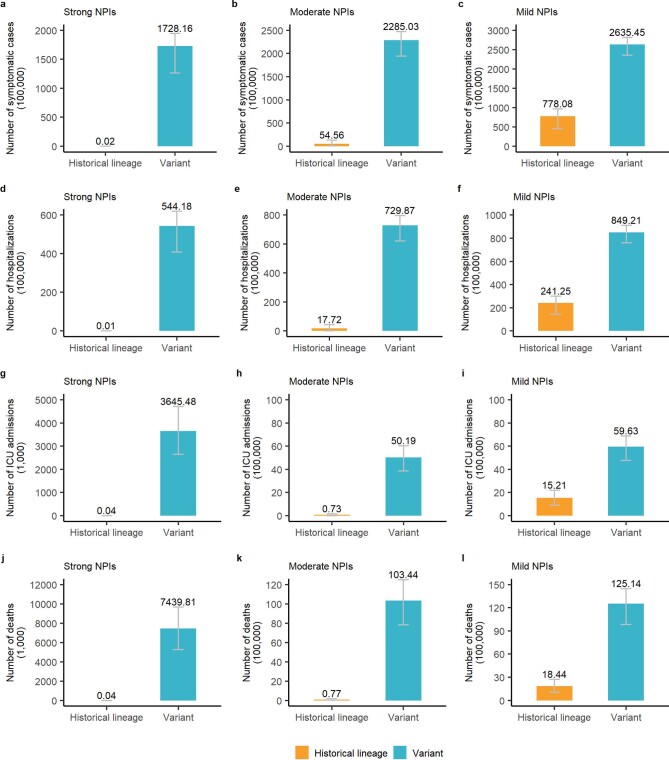
We consider higher values of Rt to account for enhanced transmissibility of SARS-CoV-2 variants. a, The number of symptomatic cases in the context of strong NPIs, Rt=
1.1 for historical lineage, while Rt
=
1.7 for variants; b, the number of symptomatic cases in the context of moderate NPIs, Rt
=
1.3 for historical lineage, while Rt
=
1.9 for variants; c, the number of symptomatic cases in the context of mild NPIs, Rt
=
1.5 for historical lineage, while Rt
=
2.1 for variants. d-f, as a-c but for the number of hospitalizations. g-i, as a-c but for the number of ICU admissions. j)-l) as a-c but for the number of deaths. Number denotes median, and error bars denote quantiles 0.025 and 0.975.
Alternative vaccination parameters and scenarios
A further set of sensitivity analyses are conducted to evaluate the impact of baseline assumptions on our results for Rt=
1.3 (moderate NPIs). Provided that vaccination can only protect against illness (SA11) but not SARS-CoV-2 infections, COVID-19-related deaths increase by 33-fold with respect to the baseline: from 76,700 to 2.66 million (Supplementary Fig. 6). In this case, maintaining stringent NPIs measures in place for a prolonged time horizon would be necessary as such vaccine would not be effective to suppress transmission (as reported previously49). Assuming a shorter duration of vaccine-induced protection of 6 months (SA12) instead of lifelong protection (that is, longer than the 2-year time horizon considered; Supplementary Fig. 6) has a similarly large effect on projections.
In our main analysis, we use the contact matrix estimated from a contact survey conducted in Shanghai before the COVID-19 pandemic13. Should a new COVID-19 wave start to unfold in China, it is unclear to what extent pre-pandemic contact patterns could be representative of such a situation. Therefore, we add a sensitivity analysis in which we assume the mixing patterns estimated in Shanghai in March 202050, when schools were still closed as a response to the COVID-19 pandemic (SA13). For Rt=
1.3 and the baseline parameters for the vaccination, the estimated number of deaths would be 16,000 as compared with 76,700 estimated using the pre-pandemic mixing patterns (79% decrease; Supplementary Fig. 6). In fact, the relative contribution of the adult population (which is the main target of the vaccination campaign) to the overall transmission as compared with children is higher than when considering pre-pandemic mixing patterns (when schools were open and school-age individuals had the highest number of contacts).
Other factors such as excluding detected symptomatic cases from vaccination (SA14 and SA15), the time interval between two doses (SA16 and SA17) and assuming an all-or-nothing vaccine (SA18) do not substantially affect estimates of deaths and symptomatic infections (Supplementary Figs. 6 and 7). A similar trend is observed for hospitalized cases and ICU admissions.
Discussion
Using a stochastic dynamic model of SARS-CoV-2 transmission and COVID-19 burden tailored to the epidemiological situation in China, we find that, in the absence of NPIs and independently of the vaccine prioritization strategy and capacity of the vaccination campaign, timely rollout of an effective vaccine (VE 80%) would not be enough to prevent a local outbreak from escalating to a major widespread epidemic. Provided that NPIs are in place and capable of bringing Rt to 1.3, a daily vaccine rollout of 4 doses per 1,000 individuals could reduce COVID-19 burden by around 99%, and bring Rt below the epidemic threshold about 9 months after the start of the vaccination campaign. A relaxation of NPIs that brings the value of Rt to 1.5 could not prevent sustained epidemic growth, which would cause 1.8 million deaths. A net reproduction number of 1.5 could only be sustained when accompanied by an improvement of the vaccine administration capacity up to 10 doses per 1,000 individuals per day. Relaxation of NPIs in the first 6–9 months of vaccine rollout could lead to substantial increases of COVID-19 burden if daily vaccination capacity could not be enhanced to 10–15 million doses.
Bubar et al. evaluated COVID-19 vaccine prioritization strategies and found that prioritizing older adults is a robust strategy to minimize deaths across countries when Rt=
1.5, while prioritization shifted to the 20–49-year-old group when Rt
=
1.15 (ref. 51). The broad scope of that multi-country analysis does not account for features of COVID-19 epidemiology and vaccination programme that are unique to China. In particular, differently from most countries where natural immunity is building up after widespread epidemics, China has been able to suppress SARS-CoV-2 transmission for most of 2020. As a result, prior immunity is very low, thus calling for specifically tailored analysis. Nonetheless, our findings confirm that, if NPIs can maintain transmission rates at low levels during the vaccination campaign, strategies that target indirect benefits perform better, while if transmission rates remain high, strategies maximizing direct benefits may save more lives51.
As highlighted in vaccination studies in Italy52, in the race between the vaccination campaign to build population herd immunity and the progress of the epidemic, the speed of vaccine deployment is critical. Considering the average vaccine distribution capacity of the current COVID-19 vaccination campaign in China6, we use 6 million doses administered per day in the baseline analysis. Several manufacturers state that a total of 3.9 billion doses of COVID-19 vaccine could be produced in 2021, equivalent to about 10 million doses per day22–26. China committed to provide COVID-19 vaccines to >100 countries, which could reduce the number of doses to be distributed locally. Even if these candidate vaccines could be licensed and manufactured smoothly, it would take about one year to vaccinate 70% of the population.
Six months after initiating vaccination programme, roughly 24.3% of Chinese population has been vaccinated6. Limited vaccine production capacity, particularly at the initial stage, could slow the speed of vaccine rollout. Slower rates of vaccine production and administration may result in a longer period of SARS-CoV-2 transmission. It is thus crucial to keep monitoring local outbreaks and invest resources in outbreak management (as currently done in China) to keep Rt close to the epidemic threshold at least for the next 1–2 years. In the very unique context of China, a value of Rt of 1.3 would result in about 76,700 cumulative deaths, comparable to the annual influenza-related death toll in China35. The development of detailed logistical plans and tools to support an increased vaccination capacity as well as effective logistic (vaccine transport, storage and continuous cold-chain monitoring) are key factors for a successful mass vaccination campaign.
In the early phase of COVID-19 spread in Wuhan in 2019, before interventions were put in place, R0 was estimated to be in the range 2.0–3.5 (refs. 27–32). Given the knowledge of mechanisms of SARS-CoV-2 transmission, and the devastating consequences of an uncontrolled COVID-19 epidemic, the Chinese population would maintain cautious behaviour (such as cleaning hands often, coughing or sneezing in bent elbow, avoiding close contact with someone who is sick, etc.) even without the need to impose NPIs. As such, in our analysis simulating an epidemic triggered by imported cases, we decided to consider an initial reproduction number of 2.5, which is at the lower end of the estimated spectrum.
SARS-CoV-2 variants are circulating globally and quickly became dominant in countries such as the United Kingdom and Italy (lineage B.1.1.7) and South Africa (lineage B.1.351). Recently, variant B.1.617 identified in India has raised global concern. Mainland China border control screenings have already identified imported cases with SARS-CoV-2 lineage B.1.1.7 and B.1.617. Our study shows that the spread of new more transmissible and/or more lethal variants could substantially decrease the net benefit of vaccination. Strict border quarantine and isolation as well as genomic surveillance will be key while vaccines roll out in China.
Our analysis on the VE shows that, if we consider VE of 60%, both the number of symptomatic cases and deaths are estimated to double as compared with the baseline vaccination coverage of 80%. Given that the final composition of a nationwide rollout will likely include a combination of vaccines with varying efficacy, monitoring VE on the ground will remain a priority.
Here, we propose a general framework to evaluate the impact of COVID-19 vaccination programmes in the absence/presence of NPIs and to explore priority target populations to minimize multiple disease outcomes. The proposed modelling framework is adaptable to other country-specific contexts. However, this requires the collection of country-specific data about the epidemiological situation (for example, landscape immunity of the local population, prevalence of infections), vaccination parameters (for example, vaccine supply and capacity of immunization services, efficacy of different vaccines, target age groups), socio-demographic characteristics of the population (for example, size of the priority population by age group, age-mixing patterns) and the priorities of the pandemic responses (for example, limiting the death toll or preventing infections).
Our study has a number of limitations. First, we integrate the impact of NPIs through a simple reduction in the value of Rt at the beginning of the outbreak, homogeneously across age groups. However, our analysis does not suggest which combination of NPIs should be adopted to lower Rt to a certain level or how this would affect transmission rates in different age groups. Li et al. estimated that individual NPIs, including school closure, workplace closure and public events bans, were associated with reductions in Rt of 13–24% on day 28 after their introduction53. Further studies are needed to pinpoint the specific NPIs to be adopted in parallel with the vaccination campaign and their impact on the quality of life of the population.
Second, in China, vaccines have not been licensed for children, so we assume a 50% lower or equivalent VE for them compared with other adults. Although we show that variations in these rates do not substantially affect the overall effect of the vaccination campaign, further data on age-specific VE could help refine priority groups. Our sensitivity analyses on vaccine coverage reveal the importance of extending the vaccination to the young population once the use of vaccines is authorized for that age segment of the population.
Third, we assumed that immunity after natural infections lasts longer than the time horizon considered (2 years). If this is not the case, waning of immunity would inflate the rate of susceptible individuals and thus require booster vaccinations. This could become an issue with the emergence of immune-escape variants, as reported in South Africa54. Given limited information at this stage, we did not consider this scenario in our analyses, but this is an important area of future research.
Fourth, age-mixing patterns are key to assess the impact of vaccination as individuals of different ages are exposed to different transmission risks. In the main analysis, we assumed the mixing patterns to correspond to those estimated before the COVID-19 pandemic, indicating the goal of a return to pre-pandemic interactions. We have also performed a sensitivity analysis based on the mixing patterns estimated in China in March 202050, after the lockdown was lifted but schools were still closed. How the population would mix in case of a new wave of COVID-19 starts to unfold in China remains to be seen.
Moreover, our study is performed at a national scale and thus our estimates of the impact of vaccination should be interpreted cautiously at the local scale. In fact, spatial heterogeneities within China in terms of risk of case importation, socio-demographic characteristics of the population, mixing and mobility patterns, vaccination coverage and capacity may affect our results55.
Enhanced vaccination efforts in conjunction with NPIs have been successfully used during the COVID-19 outbreak in Ruili City (Yunnan Province, China) in March–April 2021. Our analysis, however, focuses on the assessment of whether and to what extent we need to rely on NPIs to prevent a COVID-19 epidemic while vaccines are rolled out. As such, our results cannot be used to guide a reactive spatially targeted strategy. To properly capture the peculiarity of that context, specific modelling tools mirroring the interventions adopted in China as a response to emerging outbreaks are needed.
Finally, it would be interesting to analyse adaptive vaccination prioritizations that change as the epidemiological situation evolves over time, but that would require the development of dynamic optimization algorithms that lie beyond the scope of this work56. Nonetheless, our study provides estimates of the effect of relaxing NPIs over the course of the epidemic.
In conclusion, vaccination alone could substantially reduce COVID-19 burden, but in the foreseeable future may not be enough to prevent local outbreaks from escalating to major widespread epidemics due to limitation in the vaccine production and supply (particularly at the initial stage of the vaccination), as well as the capacity of vaccination system. This is especially relevant in contexts where most of the population is still susceptible to SARS-CoV-2 infection, as is the case in most of China. Maintaining NPIs (such as social distancing, testing, case isolation and contact tracing, wearing masks and limitation on large gatherings) throughout 2021 is necessary to prevent resurgence of COVID-19 epidemics until a sufficiently high level of immunity is reached, which depends on the transmissibility of the variants circulating at that time.
Methods
SARS-CoV-2 transmission and vaccination models
We developed a model of SARS-CoV-2 transmission and vaccination, based on an age-structured stochastic SIR scheme, accounting for heterogeneous mixing patterns by age as estimated in Shanghai13. The Chinese population was distributed into 18 age groups (17 age groups of 5 years from 0 to 84 years and one age group for individuals aged 85 years or older)57. Each age group was further split into two subgroups: individuals with or without underlying conditions, where the former was considered to be associated with an increased risk of severe outcome of COVID-19 (ref. 14).
In the main analysis, susceptibility to SARS-CoV-2 infection was assumed to be heterogeneous across ages. Children under 15 years of age were considered less susceptible to infection compared with adults aged 15–64 years, while the older adults more susceptible33,34. Homogeneous susceptibility across age groups was explored in sensitivity analysis SA19. Asymptomatic and symptomatic individuals were assumed to be equally infectious33,34, and infectiousness was also assumed to be the same across age groups33,34.
Vaccine is administered with a two-dose schedule. In the baseline model, we assumed that: (i) vaccination reduces susceptibility to SARS-CoV-2 infection; (ii) only susceptible individuals are eligible for vaccination, that is, we excluded all individuals that have experienced SARS-CoV-2 infection; (iii) duration of vaccine-induced protection lasts longer than the time horizon considered (2 years).
The baseline model is shown schematically in Supplementary Fig. 1 and is described by differential systems presented in Supplementary Files 1 and 2.
Model initialization
In China, the first pandemic wave of COVID-19 was controlled by intense NPIs58,59. Almost the entire population of Mainland China is still susceptible to COVID-19 (ref. 3). As such, the model is initialized with a fully susceptible population.
China has been facing mounting pressure from imported COVID-19 cases. Containment of COVID-19 has been possible only through a combination of measures such as complete or partial lockdown, citywide mass screening using reverse-transcriptase polymerase chain reaction (RT-PCR) testing, tracing of contacts and contacts of contacts of COVID-19 cases, which were promptly applied wherever COVID-19 transmission emerged in Mainland China60. Despite all the efforts, containment of COVID-19 appears to be hit and miss, and sporadic outbreaks inevitably occur. Simulations are thus initialized with 40 cases, roughly corresponding to the number of cases with symptoms onset in Beijing before the detection of a local outbreak on 11 June 2020 (ref. 61).
Vaccination scenarios
To explore the impact of vaccination, we ran a set of simulations in which neither NPIs nor vaccination are implemented as a reference scenario (no vax+
no NPIs, that is, effective reproductive number Rt
=
2.5 at the beginning of simulations17,28,58), and compared it with a scenario in which vaccination only is implemented (vax
+
no NPIs). Further, we considered different sets of simulations in which NPIs are used to bring Rt respectively down to 1.5 (mild NPIs), 1.3 (moderate NPIs) and 1.1 (strong NPIs), with (vax
+
mild/moderate/strong NPIs) or without vaccination programme (no vax
+
mild/moderate/strong NPIs). In the main analysis, vaccination is assumed to begin 15 days after the epidemic start. Alternative scenarios about the seeding of the epidemic were explored as sensitivity analyses. In particular, we considered the epidemic to start when 10% (SA1), 20% (SA2) and 30% (SA3) of the Chinese population has already been vaccinated.
The model is run considering daily time steps. Gradual delivery of vaccine doses is implemented by vaccinating a fixed number of individuals each day. Although manufacturers state that a total of 3.9 billion doses of vaccines could be available by the end of 2021 (refs. 22–26), scale-up and delivery will take months. On the basis of the 2009 H1N1 influenza pandemic vaccination programme implemented in Mainland China62, in the main analysis we assumed that 6 million doses of COVID-19 vaccines could be administered each day (4 doses per 1,000 individuals) until uptake reaches 70% for all groups14. Different values of the daily vaccine administration capacity, that is, 1.3 (SA20), 10 (SA21), 15 (SA22) and 30 (SA23) million dose per day, are explored in separate sensitivity analyses. Sensitivity analyses were also performed on the vaccination coverage, which is assumed to be either homogeneous (SA4 and SA5)14 or heterogeneous by age (SA6, SA7 and SA8).
In the main analysis, vaccination is administered to susceptible individuals only. This represents an ideal scenario where we assume that all infected individuals can be identified (for example, either via RT-PCR while infected or via serological assays later on) and that SARS-CoV-2 infection confers long-lasting immunity. Since infection ascertainment could be challenging and pose additional strain on the health system, we also consider two sensitivity analyses in which only detected symptomatic cases are excluded from vaccination (SA14 and SA15).
In the context of fast RT-PCR-based mass screening if there is an outbreak, under-ascertainment of symptomatic cases could be only related with the sensitivity of RT-PCR tests. The sensitivity is quite high (98%) if the interval between symptom onset and RT-PCR test is within 7 days, but decreases to 68% if the time interval is 8–14 days (ref. 63). The mean time interval from symptom onset to the date of collection of the sample for PCR testing was estimated to be 4.7 days in Hunan33. Accordingly, we considered as ascertainment probabilities of symptomatic cases 70% (SA14) and 90% (SA15).
Vaccination schedule and efficacy
Since December 2020, China has given conditional approval or emergency use approval for seven COVID-19 vaccines. The National Health Commission recommends that inactivated vaccines are administered on a two-dose schedule with an interval of ≥21 days, recombinant subunit vaccines administered on a three-dose schedule with an interval of ≥28 days, and recombinant adenovirus type-5-vectored vaccines administered one dose. For simplicity, in the main analysis, we modelled the administration of an inactivated vaccine developed by the Beijing Institute of Biological Products64, which entails a two-dose schedule across all age groups with an interval of 21 days. In separate sensitivity analyses, we explored an interval of 14 and 28 days (SA16 and SA17).
China approved its first local COVID-19 vaccine (developed by Sinopharm) for general public use on 31 December 2020, with an estimated VE of 79.3%21. In the main analysis, we used a VE of 80% against infection in individuals aged 20–59 years. In the developed model, vaccination confers partial protection, that is, vaccinated individuals are 80% less likely to develop infection upon an infectious contact. Sensitivity analyses using a VE of 60% (SA9) and 90% (SA10) were performed separately. The alternative values of VE were selected on the basis of published upper efficacy of vaccines of 94–95% and in such a way to cover a plausible efficacy range of forthcoming vaccines65–67.
Phase 2 clinical trials demonstrated that vaccine immunogenicity was lower among older individuals than in younger adults64. And for other inactivated vaccines such as influenza vaccine, a lower VE is observed in children compared with young adults68. Accordingly, we assumed an age-dependent VE. In particular, given a baseline efficacy VE among individuals aged 20–59 years (80% in the main analysis), we assumed a 50% lower VE in individuals <20 and ≥60 years of age (namely 40%). A scenario without age-specific variations in VE was explored as sensitivity analysis SA24.
Individuals vaccinated with the first dose could still develop infections without any immune protection, while the second dose vaccination could produce the expected VE after an average of 14 days. In the main analysis we assume both natural infection-induced and vaccine-induced immunity to SARS-CoV-2 infection does not wane within the considered time horizon (2 years). In additional sensitivity analyses, we considered an average duration of vaccine-induced protection of 6 months (SA12) and 1 year (SA25). We also consider a sensitivity analysis assuming that vaccination is effective in preventing symptomatic illness but not infection (SA11), and another one assuming an all-or-nothing vaccine, that is, the vaccine confers full protection to VE percent of vaccinated individuals (SA18).
Priority order of vaccination
The doses available to be distributed daily (6 million in the main analysis) are assigned by considering the following order of priority14: In the main analysis, healthcare workers are considered as the top priority (tier 1 of the vaccination strategy); law enforcement and security workers, personnel in nursing home and social welfare institutes, community workers and workers in energy, food and transportation sectors are included in tier 2; adults ≥60 years of age with underlying conditions, and adults ≥80 years of age without underlying conditions, who are at the highest risk of severe/fatal COVID-19, are considered in tier 3; individuals aged <60 years with pre-existing medical conditions and pregnant women are included in tier 4; individuals aged 20–59 years without underlying conditions are included in tier 5; school-age children and younger children aged ≤5 years without underlying conditions are recommended for vaccination in tier 6 (Supplementary File 3).
Different priority orders are explored as sensitivity analyses. Healthcare workers and the other essential workers listed above are fixed in tier 1 and 2 of vaccination, while the remaining population is vaccinated as described in Supplementary Table 2 by considering different orders of prioritization only based on age and disregarding the presence of underlying conditions (SA26: first prioritization to old adults; SA27: first prioritization to working-age groups; SA28: first prioritization to school-age groups). We explore the impact of 5,000 initial cases on the prioritization strategy (SA29). To understand the impact in terms of number of infections by age, we compare the prioritization strategy when we account for the uncertainty in the contact matrix and in the susceptibility to infection by age, or not (in this context, median values of contact numbers and relative susceptibility are used).
COVID-19 burden model
The main output of above transmission model is the age-specific number of new infections per day in the subpopulation with or without underlying conditions. On top of that, we developed a model of COVID-19 disease burden to estimate the number of symptomatic cases, hospitalization, ICU admissions and deaths in different scenarios in the presence/absence of vaccination.
We computed the age-specific number of symptomatic infections in individuals with and without underlying conditions on a daily basis by applying an age-specific probability of respiratory symptoms of 18.1%, 22.4%, 30.5%, 35.5% and 64.6% respectively for 0–19, 20–39, 40–59, 60–79 and 80+ years of age, as estimated from contact tracing data in Lombardy20. We assume that individuals with and without underlying conditions have the same age-specific probability of developing symptoms.
The daily age-specific number of hospital admissions in the two subpopulations was computed by applying the age-specific proportion of laboratory-confirmed symptomatic cases requiring hospitalization (Supplementary File 4), delayed by an average time of 3.8 days between symptom onset and hospitalization17.
The daily age-specific number of patients admitted to ICU in the two subpopulations was computed by applying to hospitalized cases an age-specific probability of being admitted to ICU19, and distinguishing patients requiring intensive care in survivors and non-survivors. Survivors are admitted to ICU after an average time of 7 days from hospitalization. Non-survivors are admitted to ICU after an average time of 8 days after hospitalization16.
The daily age-specific number of deaths in the two subpopulations was computed by applying the age-specific fatality ratio among symptomatic cases (Supplementary File 4), delayed by an average time of 13.9 days between symptom onset and death18.
Data analysis
For each scenario, 200 stochastic model realizations were performed. The outcome of these simulations determined the distributions of the number of symptomatic infections, hospitalizations, ICU admissions and deaths. We defined 95% credible intervals as quantiles 0.025 and 0.975 of the estimated distributions. We used a Bayesian approach to estimate Rt from the time series of symptomatic cases by date of symptom onset and the distribution of the serial interval17. The methods are described in detail in Supplementary File 5.
Reporting summary
Further information on research design is available in the Nature Research Reporting Summary linked to this paper.
Supplementary information
Supplementary Files 1–7, Supplementary Figs. 1–8 and Supplementary Tables 1–4.
Acknowledgements
The study was supported by grants from the National Science Fund for Distinguished Young Scholars (no. 81525023, H.Y.), Key Emergency Project of Shanghai Science and Technology Committee (no. 20411950100, H.Y.), European Union Grant 874850 MOOD (MOOD 015, V.M., G.G., P.P. and S.M.). The funders had no role in study design, data collection and analysis, decision to publish or preparation of the manuscript.
Extended data
Author contributions
H.Y. conceived the study. H.Y., S.M. and M.A. designed and supervised the study. J.Y., J.Z., J.C., W.W., Q.W., W.Z., Z.Z, K.D. and G.Z. participated in data collection. V.M., G.G., P.P. and F.T. developed the model. J.Y., V.M. and X.D. analysed the model outputs and prepared the tables and figures. J.Y. prepared the first draft of the manuscript. H.Y., V.M., C.V. and M.A. commented on the data and its interpretation, and revised the content critically. All authors contributed to review and revision and approved the final manuscript as submitted and agree to be accountable for all aspects of the work.
Data availability
Data used in this study can be downloaded from GitHub at https://github.com/DXW-sola1015/2021_Yang_COVID-19-Vax_China_Code.
Code availability
The code used to generate these analyses is available on GitHub at https://github.com/DXW-sola1015/2021_Yang_COVID-19-Vax_China_Code.
Competing interests
H.Y. has received research funding from Sanofi Pasteur, GlaxoSmithKline, Yichang HEC Changjiang Pharmaceutical Company and Shanghai Roche Pharmaceutical Company. M.A. has received research funding from Seqirus. None of this research funding is related to COVID-19. All other authors report no competing interests.
Ethics approval
All these data were in the public domain. Ethical review for the re-use of these secondary data is not required.
Footnotes
Peer review informationNature Human Behaviour thanks Xiaoming Shi for their contribution to the peer review of this work.
Publisher’s note Springer Nature remains neutral with regard to jurisdictional claims in published maps and institutional affiliations.
Disclaimer
This article does not necessarily represent the views of the NIH or the US government.
These authors contributed equally: Juan Yang, Valentina Marziano.
These authors jointly supervised this work: Stefano Merler, Marco Ajelli, Hongjie Yu.
Extended data
is available for this paper at 10.1038/s41562-021-01155-z.
Supplementary information
The online version contains supplementary material available at 10.1038/s41562-021-01155-z.
References
Full text links
Read article at publisher's site: https://doi.org/10.1038/s41562-021-01155-z
Read article for free, from open access legal sources, via Unpaywall:
https://www.nature.com/articles/s41562-021-01155-z.pdf
Citations & impact
Impact metrics
Citations of article over time
Alternative metrics

Discover the attention surrounding your research
https://www.altmetric.com/details/108013187
Article citations
Revisiting the complex time-varying effect of non-pharmaceutical interventions on COVID-19 transmission in the United States.
Front Public Health, 12:1343950, 21 Feb 2024
Cited by: 0 articles | PMID: 38450145
Assessing the impact of interventions on the major Omicron BA.2 outbreak in spring 2022 in Shanghai.
Infect Dis Model, 9(2):519-526, 28 Feb 2024
Cited by: 0 articles | PMID: 38463154 | PMCID: PMC10924171
Predictive models for health outcomes due to SARS-CoV-2, including the effect of vaccination: a systematic review.
Syst Rev, 13(1):30, 16 Jan 2024
Cited by: 0 articles | PMID: 38229123 | PMCID: PMC10790449
Review Free full text in Europe PMC
Behavioral Changes Associated With COVID-19 Vaccination: Cross-National Online Survey.
J Med Internet Res, 25:e47563, 31 Oct 2023
Cited by: 3 articles | PMID: 37906219 | PMCID: PMC10646669
Differential practice and associated factors of COVID-19 personal preventive measures among the slum and estate communities of Uganda: A community-based cross-sectional survey.
J Glob Health, 13:06039, 15 Sep 2023
Cited by: 1 article | PMID: 37712388 | PMCID: PMC10502765
Go to all (51) article citations
Data
Data behind the article
This data has been text mined from the article, or deposited into data resources.
BioStudies: supplemental material and supporting data
Similar Articles
To arrive at the top five similar articles we use a word-weighted algorithm to compare words from the Title and Abstract of each citation.
Impact of non-pharmaceutical interventions and vaccination on COVID-19 outbreaks in Nunavut, Canada: a Canadian Immunization Research Network (CIRN) study.
BMC Public Health, 22(1):1042, 25 May 2022
Cited by: 5 articles | PMID: 35614429 | PMCID: PMC9130454
Lifting non-pharmaceutical interventions following the COVID-19 pandemic - the quiet before the storm?
Expert Rev Vaccines, 21(11):1541-1553, 05 Sep 2022
Cited by: 18 articles | PMID: 36039786
Review
Funding
Funders who supported this work.
China National Funds for Distinguished Young Scientists (1)
Grant ID: 81525023