Abstract
Free full text

Orchestration of Neutrophil Movement by Intestinal Epithelial Cells in Response to Salmonella typhimurium Can Be Uncoupled from Bacterial Internalization
Abstract
Intestinal epithelial cells respond to Salmonella typhimurium by internalizing this pathogen and secreting, in a polarized manner, an array of chemokines which direct polymorphonuclear leukocyte (PMN) movement. Notably, interleukin-8 (IL-8) is secreted basolaterally and directs PMN through the lamina propria, whereas pathogen-elicited epithelial chemoattractant (PEEC) is secreted apically and directs PMN migration across the epithelial monolayer to the intestinal lumen. While most studies of S. typhimurium pathogenicity have focused on the mechanism by which this bacterium invades its host, the enteritis characteristically associated with salmonellosis appears to be more directly attributable to the PMN movement that occurs in response to this pathogen. Therefore, we sought to better understand the relationship between S. typhimurium invasion and epithelial promotion of PMN movement. First, we investigated whether S. typhimurium becoming intracellular was necessary or sufficient to induce epithelial promotion of PMN movement. Blocking S. typhimurium invasion by preventing, with cytochalasin D, the epithelial cytoskeletal rearrangements which mediate internalization did not reduce the epithelial promotion of PMN movement. Conversely, bacterial attainment of an intracellular position was not sufficient to induce model epithelia to direct PMN transmigration, since neither basolateral invasion by S. typhimurium nor apical internalization of an invasion-deficient mutant (achieved by inducing membrane ruffling with epidermal growth factor) induced this epithelial cell response. These results indicate that specific interactions between the apical surface of epithelial cells and S. typhimurium, rather than simply bacterial invasion, mediate the epithelial direction of PMN transmigration. To further investigate the means by which S. typhimurium induces epithelia to direct PMN movement, we investigated whether the same signaling pathways regulate secretion of IL-8 and PEEC. IL-8 secretion, but not PEEC secretion, was activated by phorbol myristate acetate and blocked by an inhibitor (mg-132) of the proteosome which mediates NF-κβ activation. Further, secretion of IL-8, but not PEEC, was activated by an entry-deficient (HilΔ) S. typhimurium mutant or by basolateral invasion of a wild-type strain. Together, these results indicate that distinct signaling pathways mediate S. typhimurium invasion, induction of IL-8 secretion, and induction of PEEC secretion in model intestinal epithelia.
Salmonella typhimurium entry into the intestinal epithelia results from a multistep process that culminates in host cell membrane ruffling and subsequent bacterial uptake (8, 9, 11, 46). The events that trigger S. typhimurium internalization appear to require an array of bacterial secreted proteins which are essential to the induction of host cell signal transduction pathways that lead to membrane ruffling (1, 3, 20–22, 24, 33, 40). For example, approximately 25 genes necessary for S. typhimurium invasion have been identified and are contained within the Salmonella pathogenicity island 1, located at or near centrisome 63 of the S. typhimurium chromosome (for a review, see reference 11). These genes encode proteins which are either structural components or secreted products of a type III protein-secretion apparatus. This secretion apparatus of S. typhimurium, as well as some of the secreted proteins themselves, are necessary for bacterial mediated endocytosis and are homologous to the type III secretion system found in Shigella spp.
In response to S. typhimurium, the intestinal epithelium promotes an intense inflammatory response consisting largely of the migration of polymorphonuclear leukocytes (neutrophils or PMN) toward and ultimately across the epithelial monolayer into the intestinal lumen (4, 23, 32, 46, 49). Transmigration of PMN in response to luminal pathogens necessarily involves movement through several anatomic compartments, each with their own microenvironment: (i) the well-characterized emigration of PMN from the microvasculature (37, 42, 45), (ii) the subsequent migration of PMN across the lamina propria (28), and (iii) the transepithelial migration (31). While the mechanisms driving these later steps have only recently begun to be characterized, it is evident that bacterial epithelial cell interactions can result in epithelial cell production of important regulators of inflammation (6, 7, 19, 28–31). For example, among the events stimulated by such pathogen and host associations is the epithelial cell release of potent PMN chemoattractants, the purpose of which is to guide PMN into the intestinal lumen and thus to the site of bacterial-epithelial contact. Notably, S. typhimurium-intestinal epithelial cell interactions induce the epithelial synthesis and basolateral release of the potent PMN chemoattractant interleukin-8 (IL-8) (28, 30). Such basolateral secretion of IL-8 imprints the subepithelial matrix with retained haptotactic gradients sufficient to resist the washout effects of the massive fluid movement which characterizes this compartment (28). Thus, the primary role for such basolateral secretion of IL-8 is the recruitment of PMN through the matrix (lamina propria) to the subepithelial space. Ultimately, however, PMN must traverse the intestinal epithelium and, in doing so, impale the epithelial tight junctions. In a model of S. typhimurium-induced inflammation, this final and rate-limiting step of PMN movement across the intestinal epithelium has recently been found to be directed by the apical epithelial release of a novel soluble factor, designated PEEC (pathogen-elicited epithelial chemoattractant) (31). These findings have led to our current view that the concerted polarized secretion of PEEC and IL-8 is largely responsible for directing PMN movement into the intestinal lumen in response to S. typhimurium.
Since variations in the abilities of Salmonella serotypes to induce model epithelia to direct PMN transmigration correlates with their abilities to cause enteritis in humans (29), S. typhimurium activation of epithelial-cell chemokine secretion is likely a key event in disease caused by this organism. Yet the means by which S. typhimurium elicits this chemokine secretion have not yet been characterized. Because S. typhimurium invasion of eukaryotic cells is a relatively well understood process, we reasoned that exploring the relationship between S. typhimurium invasion and epithelial promotion of PMN movement was a logical way to begin to elucidate the means by which nontyphoidal salmonellae induce epithelia to promote PMN movement, thus inducing enteritis. It has been shown that noninvasive mutants of S. typhimurium (phoPc, hilA, and invA), which harbor critical invasion deficiencies in some aspect of the type III secretion system, do not induce model epithelia to direct PMN transmigration (29). While this suggests that invasion might be required, it could also indicate that an aspect of the type III secretion apparatus rather than invasion per se is required. Here, we report two novel findings pertaining to the disease pathophysiology of S. typhimurium-induced enteritis. First, we show that S. typhimurium internalization can be uncoupled from the signaling mechanisms which mediate epithelial promotion of PMN movement, since S. typhimurium internalization was neither necessary nor sufficient to elicit this response. Second, we demonstrate that distinct epithelial signaling pathways mediate the secretion of IL-8 and PEEC.
MATERIALS AND METHODS
Cell culture.
T84 intestinal epithelial cells (passages 45 to 65) were grown and maintained as confluent monolayers on collagen-coated permeable supports (5) with recently detailed modifications (26). T84 cells were grown as monolayers in a 1:1 mixture of Dulbecco-Vogt modified Eagle medium and Ham’s F-12 medium supplemented with 15 mM HEPES buffer (pH 7.5); 14 mM NaHCO3; 40 mg of penicillin, 8 mg of ampicillin, and 90 mg of streptomycin per ml; and 5% newborn calf serum. Monolayers of T84 cells were grown on 0.33-cm2 ring-supported polycarbonate filters (Costar Corp., Cambridge, Mass.) and utilized 6 to 14 days after being plated, as described previously (26). For clarity, we will refer here to this polycarbonate filter with the attached monolayer of T84 cells and matrix as “cell culture inserts.” A steady-state resistance is reached in 4 to 6 days, with variability largely related to the cell passage number. Cell culture inserts received one weekly feeding after the initial plating. Cell culture inserts of inverted monolayers, used to study transmigration of PMN in the physiological basolateral-to-apical direction, were constructed as previously described (26, 35, 39).
Bacterial strains and growth conditions.
S. typhimurium χ3306 (wild-type strain) and PhoPc (invasion-defective strain) were cultured as previously described (29). The Hil mutant carries a spontaneous deletion secondary to homologous recombination of Tn5B50 and Tn5 in hilΔ. This results in an approximately 8-kb deletion, including prgH. The media used were as follows. Luria broth (LB) was made as described by Revel (44). L agar is L broth containing 12 g of Bacto Agar (Difco) per liter. MacConkey agar (Difco) was prepared according to package instructions.
The bacterial growth conditions were as follows. Nonagitated microaerophilic bacterial cultures were prepared by inoculating 10 ml of LB with 0.01 ml of a stationary-phase culture, followed by overnight incubation (approximately 18 h) at 37°C. Bacteria from such cultures were in the late logarithmic phase of growth and correlated with 5 × 108 to 7 × 108 CFU/ml routinely. CFU were determined by diluting and plating onto MacConkey agar medium or L agar as previously detailed (29, 30).
S. typhimurium invasion into T84 intestinal epithelial monolayers.
Infection of T84 monolayers was performed by the method described previously (30). Briefly, cell culture inserts of T84 cells were prepared as described above. After confluency was established (usually 5 days after plating), the cell culture inserts were lifted from the wells, drained of media by inverting them, and gently washed by immersion in a beaker containing Hanks balanced salt solution (HBSS+; Ca2+ and Mg2+, with 10 mM HEPES [pH 7.4]; Sigma Chemical Co., St. Louis, Mo.). The cell culture inserts were placed in a new 24-well tissue culture plate with 1.0 ml of HBSS+ in the lower (basolateral) well and 0.05 ml HBSS+ added to the upper (apical) well. After 30 to 45 min of equilibration, 10 μl of washed (with HBSS+) bacteria was added apically (unless indicated otherwise) to each cell culture insert. This represents an inoculation ratio of approximately 20 bacteria/epithelial cell. S. typhimurium attachment to and entry into T84 intestinal epithelial cells was assessed after 1 h. Cell-associated salmonellae represent populations of bacteria attached to and/or internalized into the T84 monolayers and were released by incubation with 0.1 ml of 1% Triton X-100 (Sigma). Internalized bacteria were those obtained from lysis of the epithelial cells with 1% Triton X-100 90 min after the addition of gentamicin (500 μg/ml). Preliminary gentamicin dose-response studies defined the conditions required to achieve bactericidal effects on the strain used (data not shown). For both cell-associated and internalized bacteria, 0.9 ml of LB was then added, and each sample was vigorously mixed and quantified by plating for CFU on MacConkey agar medium. To determine the number of attached salmonellae cell-internalized salmonellae were subtracted from cell-associated salmonellae (since cell-associated salmonellae contain both attached and internalized bacteria).
Cytochalasin treatment.
HBSS+-washed cell culture inserts were incubated in the presence of 5.0 μg of cytochalasin D per ml (stock concentration at 5 mg/ml in dimethyl sulfoxide [1%]; Sigma) for 45 min at 37°C. Subsequently, S. typhimurium was added to the apical surface of the cell culture inserts in the continued presence of cytochalasin D. After an incubation of 1 h at 37°C, the cell culture inserts were washed free of both nonadherent bacteria and cytochalasin D and then processed for either invasion assays (as described above) or PMN transmigration assays (as described below).
EGF treatment.
To prepare for EGF treatment, the cell culture inserts were starved of serum for approximately 16 to 18 h prior to infection with S. typhimurium. After serum starvation, the cell culture inserts were washed in HBSS+ and equilibrated for 20 min at 37°C, and S. typhimurium was added to the apical surface as described above. Epidermal growth factor (EGF) (16 nM; Sigma) was added during the last 30 min of incubation as previously described by (12). Cell culture inserts were assessed for both S. typhimurium internalization (see above) and S. typhimurium-induced PMN transepithelial migration (see below).
IL-8 ELISA assay.
IL-8 was measured by enzyme-linked immunosorbent assay (ELISA) as previously described (30) except for the following minor modifications: 96-well plates (Linbro/Titretek; ICN Biochemicals, Aurora, Ohio) were coated overnight with goat anti-human IL-8 (R&D Systems, Minneapolis, Minn.). The detecting antibody used was rabbit anti-human IL-8 (Endogen, Woburn, Mass.).
Gentamicin treatment.
The S. typhimurium was allowed to colonize T84 monolayer as described above. Then, 45 min after the bacteria were applied, nonadherent bacteria were washed off (via three rinses with HBSS+), and monolayers were placed in 500 μg of gentimicin per ml (or, as a control, in HBSS+) for 1 h at 37°C. The cell culture inserts were then rinsed three times with HBSS+ and placed in fresh HBSS+. The basolateral supernatants were collected 3 h later.
MG-132 treatment.
HBSS+-washed cell culture inserts were incubated in the presence of MG-132 (50 μM; Calbiochem) for 1 h at 37°C. Subsequently, S. typhimurium was added to the apical surface, as described above, in the continued presence of mg-132, and incubated for 1 h at 37°C. After this incubation, S. typhimurium-infected cell culture inserts were processed for either invasion assays (see above) or PMN transepithelial migration assays (see below).
Electrical measurements.
To assess the transepithelial potentials and resistance, a commercial voltage clamp (Bioengineering Department, University of Iowa) was employed and interfaced with an equilibrated pair of calomel electrodes submerged in saturated KCl along with a pair of Ag-AgCl electrodes submerged in HBSS+. Agar bridges were used to interface the electrode with the solutions on either side of the cell culture inserts (one calomel and one Ag-AgCl electrode in each well), and measurements of the short-circuit current and resistance were made as detailed elsewhere (25).
PMN transepithelial migration assay.
The physiologically directed (basolateral-to-apical) PMN transepithelial migration assay has been previously detailed (39). Human PMN were isolated from normal volunteers as described elsewhere (17, 39). Briefly, PMN were routinely isolated from anti-coagulated sodium citrate (13.2 g) and dextrose (11.2 g) in 500 ml of water (pH 6.5) and whole blood (150 to 500 ml) collected by venipuncture from normal donors of both sexes. The buffy coat is obtained via spinning at 400 × g at room temperature. The plasma and mononuclear cells were removed by aspiration, and the majority of erythrocytes were removed by using a 2% gelatin sedimentation technique as previously described (38). Residual erythrocytes were then removed by gentle lysis in cold NH4Cl lysis buffer. This technique allows for rapid isolation (90 min) of functionally active PMN (>98% as detected by trypan blue exclusion) at greater than 90% purity. The PMN were subsequently suspended in modified HBSS (without Ca2+ and Mg2+ and with 10 mM HEPES, pH 7.4; Sigma) at a concentration of 5 × 107/ml.
Before the addition of PMN in this assay system, inverted cell culture inserts (38) were extensively rinsed in HBSS+ to remove residual serum components. S. typhimurium were prepared by two washes in HBSS+ and then resuspended at a final concentration of approximately 5 × 109/ml. Inverted cell culture inserts were removed from each well and placed in a moist chamber such that the epithelial apical membrane was oriented upward. The bacterial suspension (25-μl bacterial aliquots [ca. 1.25 × 108]) was gently distributed onto the apical surface and incubated for 60 min at 37°C. Nonadherent bacteria were removed by three washes in HBSS+ buffer. The inverted cell culture inserts were then transferred back into the 24-well tissue culture tray containing 1.0 ml of HBSS buffer in the lower (apical membrane now oriented upward and colonized with S. typhimurium) reservoir and 160 μl in the upper (basolateral interface) reservoir (30). For simplicity, the reservoir will be referred to according to which epithelial membrane domain they interface with (i.e., apical or basolateral). To the basolateral bath, 40 μl (106) of isolated PMN were added to each cell culture insert and incubated for 120 min at 37°C. Positive control transmigration assays were performed by the addition of chemoattractant (10 nM N-formylmethionylleucyl phenylalanine [fMLP]) to the opposing apical reservoir. All of the experiments were performed in a 37°C room to ensure that epithelial monolayers, solutions, and plasticware were maintained at a uniform 37°C temperature.
Transmigration was quantified by assaying for the PMN azurophilic granule marker myeloperoxidase as described previously (38, 39). After each transmigration assay, nonadherent PMN were extensively washed from the surface of the cell culture inserts, and the PMN cell equivalents, estimated from a standard curve, were assessed as the number of PMN associated with the cell culture inserts and the number that had completely traversed the cell culture insert (i.e., into the basolateral reservoir).
Assay for relative PEEC concentration by measuring its ability to induce cytoplasmic [Ca++] changes in PMN.
PMN were loaded with the calcium indicator Indo-1 and cytoplasmic [Ca++] and measured as previously described (2, 15) with a Hitachi F-4500 spectrofluorimeter. PMN (2 × 106) were suspended in 960 μl of HBSS and stimulated with 40 μl of 50-fold-concentrated PEEC (the final PEEC concentration was two times that found in apical supernatants).
Data presentation.
Since variations exist in both transepithelial resistance between groups of monolayers (baseline resistance range, 650 to 1,500 ohm · cm2) and in PMN obtained from different donors, individual experiments were performed with large numbers of monolayers and PMN from single blood donors on individual days. PMN isolation was restricted to 10 different donors (repetitive donations) over the course of these studies. The S. typhimurium invasion and myeloperoxidase assay data were compared by using the Student’s t test. PMN transmigration results are represented as PMN cell equivalents (CE) derived from a daily standard PMN dilution curve. PMN which completely traversed the monolayer are represented as the number of PMN CE per milliliter (total volume = 1 ml). Values are expressed as the mean ± the standard deviation (SD) of an individual experiment done in triplicate and repeated at least three times.
RESULTS
S. typhimurium internalization alone is insufficient to induce model intestinal epithelia to orchestrate PMN transmigration.
We recently reported that some Salmonella serotypes which are as efficient as S. typhimurium at gaining entry into model intestinal epithelia are unable to induce epithelia to direct PMN transmigration (29). This suggested that perhaps S. typhimurium attainment of an intracellular position was insufficient to activate epithelial cells to secrete the chemokines which direct PMN transepithelial migration. To address this issue more thoroughly, the following approaches were used. First, we investigated whether the ability of S. typhimurium to enter model epithelia when applied at either the apical or the basolateral membrane domain correlated with its ability to induce model epithelia to direct PMN transmigration. As shown in Fig. Fig.1,1, although S. typhimurium displayed comparable ability to attach to and enter model epithelia when applied on either membrane domain, only S. typhimurium which was allowed to interact with the apical membrane domain was able to induce model epithelia to direct PMN transmigration. It should be noted that regardless of which domain a pathogen is applied on, if epithelial cells are activated to secrete chemokines which direct PMN movement, the polarity of this chemokine secretion is expected to always be such that PMN movement will be basolateral to apical, e.g., a basolateral application of shigellae still induces model epithelia to direct PMN movement from the basolateral to the apical aspect (31a).
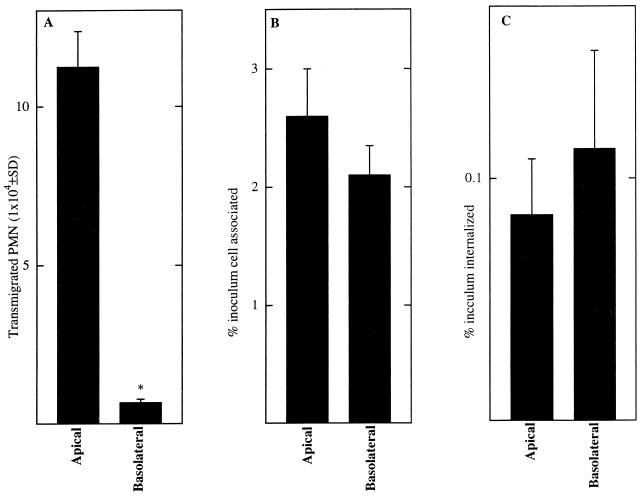
S. typhimurium can associate with and invade epithelia via either the apical or basolateral membrane domain but can only induce epithelia to direct PMN transmigration when interactions occur with the apical membrane. Monolayers of T84 epithelial cells were exposed on either their apical or their basolateral surface to S. typhimurium as described in Materials and Methods. Elicitation of PMN transmigration (A), bacterial adherence (B), and bacterial internalization (C) were measured. Data are the means (±SD) of a representative experiment performed in triplicate. An asterisk indicates a significant difference (P < 0.02) from the apical value in that panel.
To assess whether S. typhimurium internalization was sufficient to activate epithelia to direct PMN transmigration, we sought to determine whether having an invasion-defective mutant attain an intracellular position would be sufficient to activate epithelia to direct PMN transmigration. This was achieved by treating model epithelia with EGF (9, 12). Ruffles elicited by invasive S. typhimurium have also been found to directly mediate the invasion of noninvasive bacteria in a macropinocytotic fashion termed passive entry (9, 12). Although the mechanism of ruffle induction by S. typhimurium is not understood, such structures are identical to the ruffles induced by hormonal or oncogenic stimuli in their kinetics of induction, morphologies, and rearrangements of filamentous actin. It has been shown that invasion-defective mutants are unable to induce these ruffles and that inducing them with a growth factor rescues the ability of these mutants to enter eukaryotic cells (9, 12). As shown in Fig. Fig.2,2, the addition of EGF to polarized epithelial cell monolayers increased the invasion proficiency of an invasion-defective type III secretion mutant strain of S. typhimurium (PhoPc) to 50% of that elicited by wild-type S. typhimurium. Yet, such internalization was insufficient to activate the epithelia to direct PMN transmigration. To see if this EGF treatment had simply failed to reach a requisite threshold level of intracellular bacteria (considering that under conditions of EGF treatment entry by PhoPc was still only 50% of the wild-type internalization levels), the level of wild-type inoculum was decreased so that the number of internalized bacteria matched that of PhoPc plus EGF. As expected, reducing the wild-type S. typhimurium inoculum in this manner modestly reduced the epithelial promotion of PMN movement. However, under these conditions, where equivalent numbers of internalized mutant and wild-type S. typhimurium were achieved, only wild-type S. typhimurium was able to induce epithelia to direct a significant amount of PMN transmigration (Fig. (Fig.2).2). It was also observed that the mere induction of membrane ruffles by EGF was insufficient to lead to PMN transmigration (data not shown).
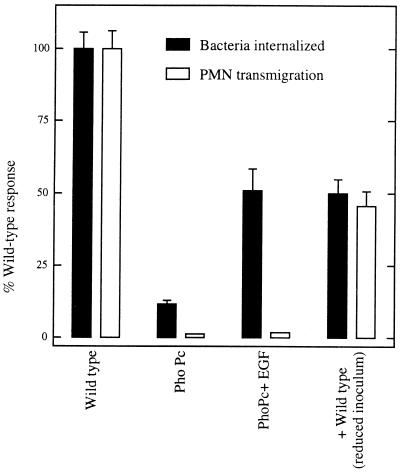
S. typhimurium internalization alone is insufficient to induce epithelia to direct PMN transmigration. Monolayers of T84 epithelial cells were apically colonized with wild-type or PhoPc mutant (which fails to invade unless induced by EGF [see the text]) S. typhimurium as described in Materials and Methods. Bacterial internalization and elicitation of PMN transmigration were measured. Data are the normalized means (±SD) of a representative experiment performed in triplicate.
S. typhimurium strains carrying mutations in their type III secretion system which fail to induce their uptake by epithelia also fail to induce epithelia to direct PMN transmigration (29). Therefore, to reconcile the possibility that such results may involve a component of the type III secretion apparatus, we assayed an additional S. typhimurium mutant, hilΔ, which harbors a mutation in the hil locus of the type III secretion apparatus. For these studies experiments were designed to decrease the wild-type S. typhimurium inoculum so that the number of internalized bacteria would be equivalent to that of the hilΔ (type III secretion defective) and PhoPc (invasion-defective pleotrophic mutant) in the absence of EGF. As shown in Fig. Fig.3,3, even with inocula in which the wild-type, hilΔ, and invasion-defective PhoPc strains achieved internalization in similar numbers, only wild-type S. typhimurium was able to activate epithelia to direct a significant amount of PMN transmigration. Together, these results indicate that S. typhimurium interaction with the apical surface of epithelial cells (likely requiring a functional type III apparatus), rather than S. typhimurium simply gaining entry into its host, results in activation of the epithelial signaling pathways that mediate the epithelial secretion of chemokines which direct PMN movement.
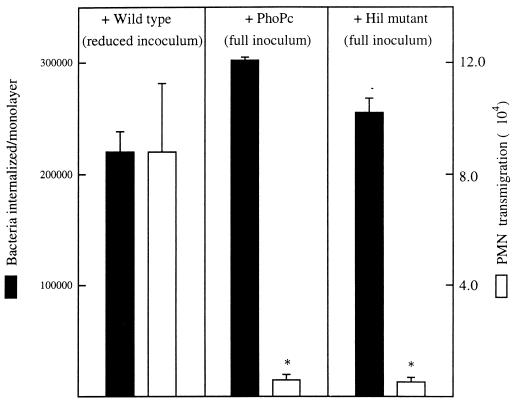
Matching invasion of wild-type S. typhimurium to invasion by mutants by decreasing the inoculum of the former does not eliminate its ability to induce epithelia to direct PMN transmigration. Monolayers of T84 epithelial cells were apically colonized with a reduced inoculum (described in Materials and Methods) of wild-type S. typhimurium or a full inoculum of PhoPc or HilΔ mutant S. typhimurium. Bacterial internalization and elicitation of PMN transmigration were measured. Data are the means (±SD) of a representative experiment performed in triplicate. An asterisk indicates a significant difference (P < 0.02) between that mutant and the wild-type values.
S. typhimurium need not be internalized in order to induce epithelia to orchestrate PMN transmigration.
We next investigated whether S. typhimurium internalization was necessary for this pathogen to induce epithelia to direct PMN transmigration. It is clearly established that an intact host cytoskeleton is required for S. typhimurium internalization, since drugs such as cytochalasin B and D, which disrupt microfilament integrity, also prevent bacterial entry (8, 10). We first verified that this was also the case for our polarized monolayers of T84 intestinal epithelial cells. Host microfilament disruption produced via the presence of cytochalasin D markedly inhibited S. typhimurium invasion (Fig. (Fig.4)4) to levels equivalent to that achieved by S. typhimurium invasion-defective strains such as PhoPc. Thus, as is the case for nonpolarized eukaryotic cells, host cytoskeletal rearrangements are required for S. typhimurium invasion into polarized monolayers of intestinal epithelial cells. This cytochalasin treatment did not alter the ability of S. typhimurium to adhere to the apical surface of these epithelial monolayers. Having shown that cytochalasin D treatment blocked S. typhimurium entry but not its adherence, we next assessed whether S. typhimurium could still induce cytochalasin D-treated epithelia to orchestrate PMN transmigration. As shown in Fig. Fig.4,4, this cytochalasin D treatment did not reduce S. typhimurium-induced epithelial promotion of PMN movement. Because this drug also perturbs epithelial-cell tight junctions (a potential limiting barrier to PMN movement), we next determined whether cytochalasin D affected exogenously driven (i.e., fMLP-induced) PMN transepithelial migration. Cytochalasin D did not have a significant effect on fMLP-induced PMN transepithelial migration (29.1 [±35] × 104 versus 30.35 [±4.2] × 104 CE in the absence versus the presence of cytochalasin D), nor did it affect the low basal level of transmigration supported by noncolonized monolayers (0.98 [±0.13] × 104 versus 0.67 [±0.38] × 104 CE in the absence versus the presence of cytochalasin D). Further, the invasion-deficient S. typhimurium strain (PhoPc) still remained unable to induce PMN transepithelial migration in the presence of cytochalasin treatment (2.7 [±0.59] × 104 versus 3.4 [±0.56] × 104 CE in the absence versus the presence of cytochalasin D). These results indicate that the PMN transepithelial migration induced by apical colonization with S. typhimurium is not dependent upon this bacterium attaining an intracellular position.
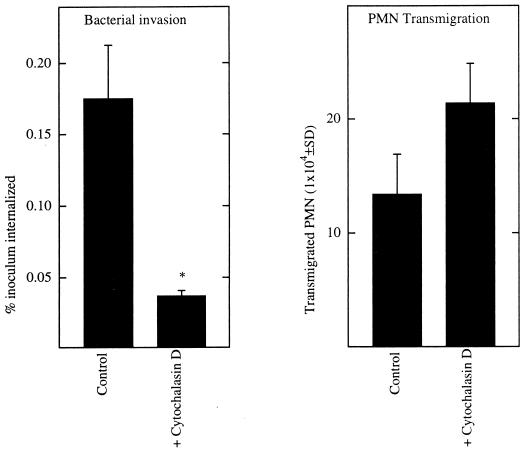
Cytochalasin D blocks internalization of S. typhimurium but does not reduce its induction of epithelial cell direction of PMN transmigration. Monolayers of T84 epithelial cells were apically colonized with S. typhimurium in the presence of cytochalasin D or vehicle (dimethyl sulfoxide). Bacterial internalization and elicitation of PMN transmigration were measured. Data are the means (±SD) of a representative experiment performed in triplicate. An asterisk indicates a significant difference (P < 0.02) between the control and the cytochalasin-treated model epithelia.
Epithelial secretion of IL-8 and PEEC are mediated by distinct signaling pathways.
Having demonstrated that S. typhimurium entry into model epithelia can be uncoupled from the epithelial promotion of PMN movement, we further investigated whether secretion of PEEC and IL-8 were also controlled by separate signaling pathways. Intestinal epithelial cells secrete IL-8 basolaterally and PEEC apically (30, 31). It is thought that IL-8 guides PMN through the lamina propria to a subepithelial compartment, whereas PEEC directs PMN movement across the intestinal epithelial cell monolayer (28, 31). The suggestion that secretion of IL-8 and PEEC might be controlled by distinct pathways came from our observation that S. typhi induces secretion of IL-8 (S. typhi, S. paratyphi, and S. typhimurium induced 2.1 ± 0.52, 2.4 ± 0.43, and 2.61 ± 0.61 ng of IL-8 secretion per ml, respectively) but not of PEEC (29). IL-8 secretion induced by either cytokines or bacteria is thought to be mediated by activation of the transcription factor NF-κβ (18). Thus, as expected, we observed that inhibition of proteosome-mediated NF-κβ activation via the compound mg-132 (43) led to a marked reduction in S. typhimurium-induced IL-8 secretion (Fig. (Fig.5,5, left panel). However, mg-132 did not significantly reduce S. typhimurium-induced PMN transmigration (i.e., PEEC secretion, the limiting factor in our model system) (Fig. (Fig.5,5, right panel). This suggests that, in contrast to IL-8 secretion, S. typhimurium-induced PEEC secretion is not mediated by NF-κβ activation.
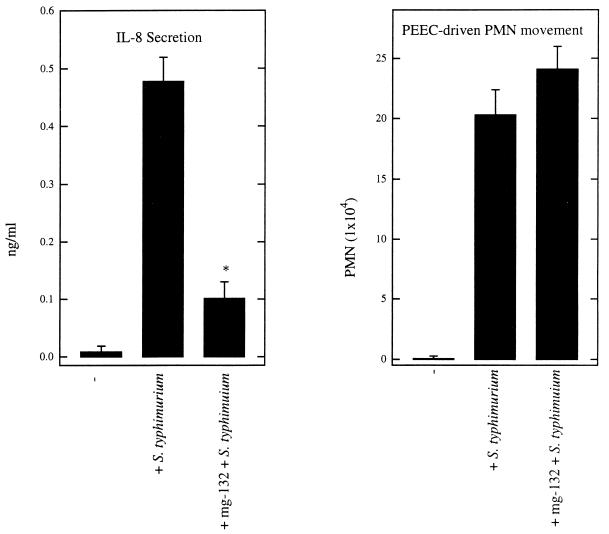
S. typhimurium-induced IL-8 secretion but not PEEC secretion is inhibited by mg-132. Monolayers of T84 epithelial cells were treated with the proteosome inhibitor mg-132, which inhibits NF-κb nuclear translocation, or vehicle (dimethyl sulfoxide) for 1 h and then apically colonized with S. typhimurium. IL-8 secretion (left) and PMN transmigration (right) were measured. Data are the means (±SD) of a representative experiment performed in triplicate. An asterisk indicates a significant difference (P < 0.02) between the control and the mg-132-treated model epithelia.
Next we measured whether phorbol myristate acetate (PMA), a known potent nonphysiologic activator of IL-8 secretion, could also activate PEEC secretion. To avoid the possible complication of PMA directly influencing PMN movement across model epithelia, we assessed relative PEEC secretion by measuring the ability of partially purified PEEC isolates to induce a cytosolic Ca2+ increase in PMN, as this is a known activity of this chemoattractant (14, 31). The specificity of this assay to measure PEEC release is based on the fact that most other molecules which can be released by epithelial cells and could perhaps induce a Ca2+ increase in PMN would be excluded by the relatively narrow molecular size range of our purification (1 to 3 kDa) (e.g., CXC chemokines and arachidonate metabolites would be excluded) and by our observation that the ability of PEEC isolates to drive PMN transmigration correlates with their ability to induce a Ca2+ increase in PMN (31). Although PMA induced far greater IL-8 secretion than S. typhimurium (Fig. (Fig.6,6, left panel), PMA did not induce model epithelia to secrete detectable amounts of PEEC (Fig. (Fig.6,6, right panel). As PMA is also known to activate epithelial secretion of a number of other immune inflammatory responsive chemokines (34, 48), our results indicate that PEEC secretion is controlled via a process distinct from those which have been characterized for IL-8 and these other chemokines.
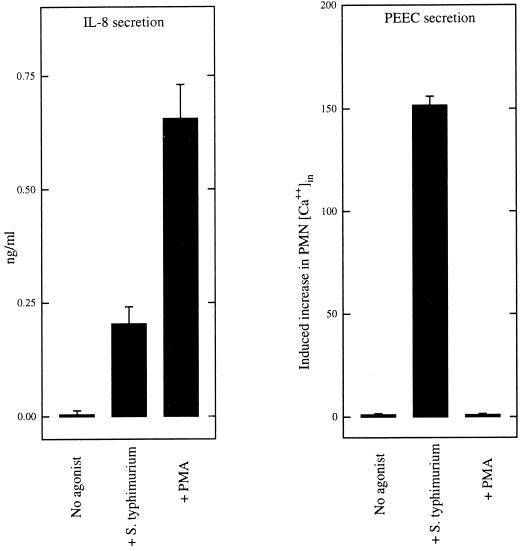
IL-8 secretion but not PEEC secretion is activated by PMA. Monolayers of T84 epithelial cells were stimulated with PMA (100 ng/ml), and supernatants were collected 4 h later. Basolateral supernatants were assayed for IL-8 by ELISA, and apical supernatants were partially purified and assayed for PEEC by measuring their ability to induce an increase in PMN cytosolic Ca++ as described in Materials and Methods. Data are the means (±SD) of a representative experiment performed in triplicate.
Lastly, we investigated whether S. typhimurium mutants (or conditions) which did not elicit PEEC secretion (i.e., PMN transmigration) could perhaps elicit IL-8. The two invasion-defective S. typhimurium strains (PhoPc and HilΔ) which we have tested were both unable to elicit PEEC secretion (29). While one of these mutants (PhoPc) was also unable to elicit IL-8 secretion, the other one (HilΔ) elicited IL-8 secretion almost as effectively as wild type (Fig. (Fig.7).7). Further, while basolateral S. typhimurium colonization does not elicit PEEC secretion, it does induce a significant amount of epithelial secretion of IL-8, albeit not quite as efficiently as when applied apically. Together, these results agree with our pharmacologic-based approach, which indicated that the epithelial secretion of IL-8 and PEEC is mediated by separate pathways. However, like PEEC secretion, maximal activation of the signals which mediate IL-8 secretion may be dependent on sustained S. typhimurium-epithelial cell interactions, since S. typhimurium-induced IL-8 secretion was attenuated when noninternalized bacteria were killed with gentamicin (66.1 ± 6.2% of control values, P < 0.05). Since PMA-induced IL-8 secretion was not affected by gentamicin (105 ± 9.1% of control values, which is not significant), the effect of this antibiotic was probably the result of its action on S. typhimurium rather than its acting nonspecifically on the model epithelia.
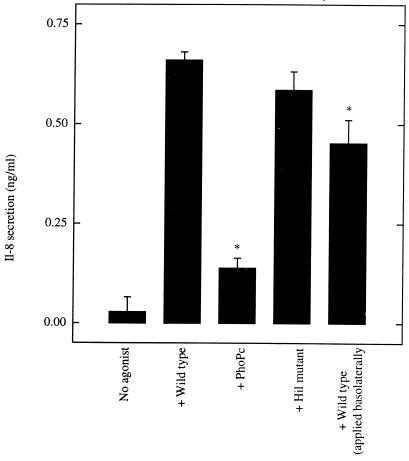
IL-8 secretion can be induced by an invasion-defective mutant which was unable to elicit PEEC secretion and PMN transmigration. Monolayers of T84 epithelial cells were colonized apically (except where otherwise indicated) with wild-type or mutant S. typhimurium (as indicated) as described in Materials and Methods. Basolateral supernatants were assayed 4 h later for IL-8 by ELISA. Data are the means (±SD) of a representative experiment performed in triplicate. An asterisk indicates a significant difference (P < 0.02) from the wild type strain applied apically.
DISCUSSION
The ability of Salmonella serotypes to elicit diffuse enteritis in humans correlates well with their ability to elicit the epithelial promotion of PMN transmigration, but it does not correlate well with their ability to invade epithelial cells (29). These observations indicate that salmonella-induced epithelial recruitment of immune cells is likely a key virulence mechanism underlying the enteritis elicited by some Salmonella serotypes, such as S. typhimurium. Yet, in contrast to the means by which bacteria gain entry into host cells, the mechanisms and microbial determinants by which S. typhimurium elicits a mucosal inflammatory response have not been well characterized. In this report, we present two principal findings fundamental to an understanding of the molecular mechanisms by which S. typhimurium initiates an inflammatory response (defined as the epithelial direction of PMN transmigration). First, our studies revealed that S. typhimurium induced signaling cascades which mediate the epithelial direction of PMN transmigration can be uncoupled from bacterial internalization. Further, distinct epithelial signaling pathways mediate S. typhimurium induction of IL-8 and PEEC secretion.
To better understand how S. typhimurium induces epithelia to direct PMN transmigration, we asked whether S. typhimurium internalization was necessary or sufficient to elicit this response. We found that neither S. typhimurium uptake via the basolateral membrane domain nor EGF-induced apical uptake of an invasion-defective (type III secretion system) mutant could elicit epithelia to secrete PEEC. Thus, as is the case for Salmonella serotypes which do not cause enteritis, S. typhimurium attainment of an intracellular position, by itself, is insufficient to induce epithelia to direct PMN transmigration. Further, blocking S. typhimurium internalization with cytochalasin showed that attainment of an intracellular position is not necessary for this bacterium’s interactions with epithelial cells to lead to PMN transmigration.
The molecular interactions between S. typhimurium and intestinal epithelial cells that are necessary and sufficient to activate epithelia to mediate this aspect of the immune inflammatory response are not yet clear, but some insights can be drawn. S. typhimurium activation of epithelial promotion of PMN transmigration requires a sustained interaction between this bacterium and the apical membrane of the epithelium. This is evidenced by our observation that only S. typhimurium which interacted with the apical (but not the basolateral) aspect of model epithelia could elicit PMN transmigration. That the interaction must be sustained is based on our observation that if apically attached noninternalized S. typhimurium are killed via the addition of gentamicin, S. typhimurium-induced PMN transmigration is reduced by about 90% (30). Further, this interaction would seem to be dependent on a functional S. typhimurium type III secretion system, since the mutants of this type which we have tested are unable to induce epithelia to direct PMN transmigration (30). Thus, we propose that, in addition to its role in mediating bacterial uptake, the inv/spa locus/type III secretion apparatus also plays a role in mediating S. typhimurium activation of epithelial signaling pathways which regulate PMN transmigration.
We envisage at least two possible means by which S. typhimurium might activate epithelia to direct PMN transmigration. It is possible either that S. typhimurium engages a surface receptor that activates the epithelial signaling pathway(s) which mediate this response or that S. typhimurium translocates an effector protein, not necessarily directly connected to invasion, into the host cell that may activate the controlling signaling pathway. If the signaling pathway activated by S. typhimurium that results in epithelial promotion of PMN transmigration involves bacterial ligation of a cell surface receptor, the bacterial surface expression of that ligand would seem to be dependent upon a functional type III secretion system. While this cannot be ruled out, a precedent for the latter explanation has been established by the recent work of Galyov and coworkers (13, 47). These investigators have elegantly identified a novel secreted effector protein of S. dublin, SopB (Salmonella outer protein), that is able to translocate into epithelial cells via a sip-dependent pathway and mediate, in part, mucosal inflammation and fluid secretion when examined in the ileal mucosae of calves (13). Notably, the S. dublin SopB mutant exhibited an approximately 50% decrease in both fluid secretion as well as in PMN influx in the calf ileal mucosa, despite showing wild-type levels of invasiveness for either cultured epithelial cells or intestinal mucosa. Moreover, cytochalasin treatment prevented S. typhimurium entry but did not prevent the translocation of SopB, indicating that the translocation of SopB can be performed by extracellular bacteria (13). These results are in agreement with our conclusion that S. typhimurium invasion and the epithelial promotion of PMN transmigration are separable events. Other work by Galyov has also determined that another secreted effector protein, SopE, is translocated into the eukaryotic target cell by a sip-dependent mechanism and promotes bacterial entry (47). Although a recent study (16) has described SopE as an effector molecule capable activating several GTPases of the Rho subfamily (namely, CDC42 and rac-1) and stimulating both cytoskeletal and nuclear responses in the host cell, its role in mediating S. typhimurium-induced PMN transepithelial migration seems less clear than SopB given that SopE mutants were found to be invasion defective as well as unable to elicit PMN transmigration (26a). Moreover, in addition to SopB (and possibly SopE), there may be other effectors acting in concert with these two proteins in the host cell. Nonetheless, SopB appears to be a good candidate to play a role in activating the signaling events that mediate the epithelial promotion of PMN transmigration. Whether or not SopE, or cdc42 and rac-1, might also perhaps be involved awaits further study.
The final and perhaps rate-limiting step of PMN movement to the intestinal lumen is thought to be governed by the epithelial secretion of PEEC. PEEC is secreted apically and appears to direct the final step of PMN migration across the epithelial monolayer to the intestinal lumen (31). IL-8 is secreted basolaterally and directs the prerequisite journey (at least in large part) PMN must take through the lamina propria (28, 30). Using a pharmacological approach, we found that there are considerable differences in the epithelial signaling pathways which mediate the secretion of these chemoattractants. Specifically, we observed that IL-8 secretion, but not PEEC secretion, is potently activated by PMA and potently inhibited by an inhibitor (mg-132) of the NF-κβ-activating proteosome. There are also differences in the microbial determinants which elicit these responses, as we observed that one S. typhimurium mutant which does not induce PEEC secretion induces IL-8 secretion to a similar extent as the wild type. That this mutant is invasion defective indicates that, as is the case for PEEC secretion, S. typhimurium need not be internalized to elicit IL-8 secretion.
Bacteria with the ability to induce IL-8, but not PEEC, secretion is not limited to laboratory strains, since we found that this same pattern was exhibited by two different wild-type strains of S. typhi (see Results and reference 29). Thus, it seems reasonable to consider that S. typhi eliciting basolateral IL-8 secretion but not apical PEEC secretion might explain the observation that PMN migrate to the gut in response to S. typhi but do not transmigrate into the intestinal lumen (36). It has recently been observed that S. typhi invades epithelia via a mechanism distinct from that used by S. typhimurium. That is, S. typhi but not S. typhimurium entry is dependent on the expression of cystic fibrosis transmembrane conductance regulator (CFTR) (41). Therefore, it is tempting to speculate that the CFTR-mediated interactions between S. typhi and the apical membrane domain of epithelia are sufficient to activate the signaling pathways which mediate secretion of IL-8 but not PEEC. Like PEEC secretion, maximal IL-8 secretion appears to require a sustained interaction between bacteria and host, since killing noninternalized bacteria with gentamicin also reduced IL-8 secretion in response to S. typhimurium.
It is interesting to consider some of the evolutionary implications of the interactions between these various Salmonella serotypes and the intestinal epithelia. From the epithelial-cell viewpoint, the differential control over the release of these two PMN chemoattractants (IL-8 and PEEC) would seem to provide the host appropriate flexibility in initiating the immune inflammatory response. The host would not want to direct large-scale PMN migration to the intestinal lumen unless needed, as the resulting disruption in epithelial barrier function exposes the host to a myriad of other dangers and problems (see reference 27). From the bacterial point of view, it is interesting to consider the question of whether S. typhimurium persists, in part, because of its ability to elicit PMN transepithelial migration or in spite of it. Considering that in immunocompromised individuals, S. typhimurium can infect systemically, the immune inflammatory response normally induced by this bacterium would seem to be a hindrance to this organism. However, a potential benefit of inducing an inflammatory response resulting in diarrhea would be to aid in S. typhimurium dissemination. Indeed, successful spread of this pathogen, as well as other enteric pathogens, can occur via this route, as is particularly evident in developing countries.
ACKNOWLEDGMENTS
These studies were supported by National Institutes of Health grants DK-47662 (J.L.M.), DK-35932 (J.L.M.), and DK-50989 (B.M.). A.G. is supported by an individual National Research Service Award.
We thank Andrew S. Neish for helpful discussions concerning this work.
REFERENCES
Articles from Infection and Immunity are provided here courtesy of American Society for Microbiology (ASM)
Full text links
Read article at publisher's site: https://doi.org/10.1128/iai.67.2.608-617.1999
Read article for free, from open access legal sources, via Unpaywall:
https://iai.asm.org/content/iai/67/2/608.full.pdf
Free after 4 months at iai.asm.org
http://iai.asm.org/cgi/reprint/67/2/608
Free after 4 months at iai.asm.org
http://iai.asm.org/cgi/content/full/67/2/608
Free to read at iai.asm.org
http://iai.asm.org/cgi/content/abstract/67/2/608
Citations & impact
Impact metrics
Article citations
Cross-Talk Between the Intestinal Epithelium and Salmonella Typhimurium.
Front Microbiol, 13:906238, 06 Jun 2022
Cited by: 11 articles | PMID: 35733975 | PMCID: PMC9207452
Review Free full text in Europe PMC
Dual RNA-seq unveils noncoding RNA functions in host-pathogen interactions.
Nature, 529(7587):496-501, 20 Jan 2016
Cited by: 256 articles | PMID: 26789254
Understanding the complexities of Salmonella-host crosstalk as revealed by in vivo model organisms.
IUBMB Life, 67(7):482-497, 15 Jul 2015
Cited by: 6 articles | PMID: 26179888
Review
Gene Expression Profiles of Chicken Embryo Fibroblasts in Response to Salmonella Enteritidis Infection.
PLoS One, 10(6):e0127708, 05 Jun 2015
Cited by: 8 articles | PMID: 26046914 | PMCID: PMC4457728
Salmonella-host interactions - modulation of the host innate immune system.
Front Immunol, 5:481, 07 Oct 2014
Cited by: 71 articles | PMID: 25339955 | PMCID: PMC4188169
Review Free full text in Europe PMC
Go to all (64) article citations
Similar Articles
To arrive at the top five similar articles we use a word-weighted algorithm to compare words from the Title and Abstract of each citation.
Apical secretion of a pathogen-elicited epithelial chemoattractant activity in response to surface colonization of intestinal epithelia by Salmonella typhimurium.
J Immunol, 160(1):455-466, 01 Jan 1998
Cited by: 119 articles | PMID: 9552004
Regulation of Salmonella-induced neutrophil transmigration by epithelial ADP-ribosylation factor 6.
J Biol Chem, 276(51):48431-48439, 18 Oct 2001
Cited by: 27 articles | PMID: 11641400
Salmonella typhimurium attachment to human intestinal epithelial monolayers: transcellular signalling to subepithelial neutrophils.
J Cell Biol, 123(4):895-907, 01 Nov 1993
Cited by: 282 articles | PMID: 8227148 | PMCID: PMC2200157
Neutrophil influx during non-typhoidal salmonellosis: who is in the driver's seat?
FEMS Immunol Med Microbiol, 46(3):320-329, 01 Apr 2006
Cited by: 25 articles | PMID: 16553804
Review
Funding
Funders who supported this work.
NIDDK NIH HHS (5)
Grant ID: DK-50989
Grant ID: DK-35932
Grant ID: DK-47662
Grant ID: R37 DK035932
Grant ID: R01 DK047662