Abstract
Free full text

Resolution of Secondary Chlamydia trachomatis Genital Tract Infection in Immune Mice with Depletion of Both CD4+ and CD8+ T cells
Abstract
The essential role of T cells in the resolution of primary murine Chlamydia trachomatis genital tract infection is inarguable; however, much less is known about the mechanisms that confer resistance to reinfection. We previously established that CD4+ T cells and B cells contribute importantly to resistance to reinfection. In our current studies, we demonstrate that immune mice concurrently depleted of both CD4+ T cells and CD8+ T cells resisted reinfection as well as immunocompetent wild-type mice. The in vivo depletion of CD4+ and CD8+ T cells resulted in diminished chlamydia-specific delayed-type hypersensitivity responses, but antichlamydial antibody responses were unaffected. Our data indicate that immunity to chlamydial genital tract reinfection does not rely solely upon immune CD4+ or CD8+ T cells and further substantiate a predominant role for additional effector immune responses, such as B cells, in resistance to chlamydial genital tract reinfection.
Chlamydia trachomatis, an obligate intracellular bacterial pathogen, is the most common sexually transmitted bacterial pathogen. More than 4 million new cases of chlamydial sexually transmitted infections are diagnosed each year in the United States alone (6), and women tend to suffer some of the more serious consequences of genital tract infection. Lower genital tract infection, such as cervicitis, can ascend into the upper genital tract to cause Fallopian tube damage and subsequently tubal factor infertility and ectopic pregnancy.
Among the more important and fundamental questions regarding immunity to chlamydial genital tract infection are whether natural immunity develops following human chlamydial infection and, if so, what are the immune mechanisms that contribute to the resolution of intracellular infection and protection from reinfection. The answers to those questions have been elusive, but data from several different disciplines support the notion that protective immunity develops. For example, epidemiological studies and data from human challenge experiments and vaccine trials suggest that a level of protective immunity develops in a proportion of chlamydia-infected individuals (3, 11, 16, 25), and in nearly every animal model of chlamydial infection (monkey, guinea pig, and mouse), infection resolves without antimicrobial chemotherapy (1, 31, 42). Indeed, when chlamydia-infected animals are treated with effective antimicrobial chemotherapy early during the course of genital tract infection, an inferior level of protective immunity develops (40). Collectively, those studies provide evidence that protective immunity is elicited following chlamydial infection and may contribute to a level of immune protection against reinfection.
A variety of cellular and humoral immune responses are elicited following chlamydial genital tract infection. Data from experimental infections suggest that those immune responses confer some degree of protection, although the precise roles of humoral and cellular immunity in protection against and resolution of chlamydial infection remain obscure. During the past several years, it has become evident that Th1-type responses are of utmost importance in the resolution of primary murine chlamydial infection (4, 8, 12, 15, 17, 26), but the mechanism(s) by which those responses kill or inhibit chlamydiae has not been specifically delineated. Conversely, the role of specific antibody in immune protection has evolved from being the focus of immune protection and vaccine development (7, 24, 27, 37, 41, 46, 47) to being unimportant, or at least having a very subordinate role in protective immunity (17, 18, 38). Similarly, the role of CD8+ T cells in protective immunity to chlamydial infection is controversial. While in vitro studies suggest that CD8+ T cells are cytotoxic for chlamydia-infected cells (19, 32–35), in vivo studies show that CD8+ T cells do not confer immune protection (23, 36) or play only a minor protective role in antichlamydial immunity (13, 35).
Recently, we began to investigate adaptive immunity to Chlamydia trachomatis genital tract reinfection (23). In that study, we evaluated the course of secondary chlamydial genital tract infection in immune mice in which lymphocyte subpopulations were subsequently depleted. We found that the in vivo depletion of CD4+ T cells but not CD8+ T cells in immune B-cell-deficient mice resulted in a secondary chlamydial genital tract infection that failed to resolve. Conversely, immune wild-type C57BL/6 mice depleted of either CD4+ T cells or CD8+ T cells prior to secondary infectious challenge rapidly resolved the infection. Collectively, those data demonstrate that both CD4+ T cells and B cells confer a level of protective immunity to chlamydial reinfection. The ability of either CD4- or CD8-depleted wild-type C57BL/6 mice to resolve secondary infection was attributed to immune B cells. However, the possibility that the depletion of one T-cell subset was compensated for by a heightened response of another T-cell subset could not be eliminated. In the current study, we further substantiate that immunity to secondary chlamydial genital tract infection does not rely solely upon CD4+ or CD8+ T cells and provide further indirect evidence that B cells may play an important and substantive role in immunity to reinfection.
MATERIALS AND METHODS
Mice.
Female C57BL/6 (B6) mice were purchased from the National Cancer Institute (Bethesda, Md.), maintained in the Animal Resources Center at Montana State University, and used at 8 to 10 weeks of age.
Chlamydiae.
The mouse pneumonitis (MoPn) biovar of C. trachomatis (Chlamydia muridarum) was grown in HeLa 229 cells and purified by discontinuous density gradient centrifugation (5). Infectivity was determined on HeLa cell monolayers as previously described (21).
In vivo depletion.
Hybridoma clones GK1.5 (anti-CD4) and 2.43 (anti-CD8) were purchased from the American Type Culture Collection and grown in serum-free medium, and antibodies were concentrated and purified by ammonium sulfate precipitation. Purified rat immunoglobulin G2a (IgG2a) was used as an isotype-specific immunoglobulin control for in vivo depletion experiments.
The in vivo depletion of CD4+ and CD8+ T cells was accomplished by injecting groups of mice intraperitoneally (i.p.) with 0.5 mg of anti-CD4 and anti-CD8 (anti-CD4/CD8) monoclonal antibodies for 3 consecutive days, followed by injections every third day thereafter, with the final injection being administered at 23 days (23). Thus, mice were injected with anti-CD4/CD8 on days 50, 51, 52, 55, 58, 61, 64, 67, 70, and 73 post-primary infection. Groups of control mice were injected i.p. with either 0.5 mg of purified rat IgG2a or 0.5 ml of phosphate-buffered saline (PBS; 10 mM phosphate, 0.13 M NaCl [pH 7.4]) following the same injection schedule. Depletion of specific lymphocyte subsets was monitored as described below.
Experimental design.
To evaluate the effect of the in vivo depletion of T-cell subpopulations on the ability of immune mice to resist reinfection, a group of 24 mice were injected subcutaneously with 2.5 mg of Depo-Provera (medroxyprogesterone acetate) 5 days prior to intravaginal inoculation of 5 × 104 inclusion-forming units (IFU) of C. trachomatis, an inoculum equivalent to 100 50% infectious doses (ID50). The course of primary infection was followed by enumerating the number of IFU recovered from cervicovaginal swabs using indirect immunofluorescence (21). Fifty days following primary infection, a time at which mice had resolved infection and had acquired a level of resistance to reinfection (21, 38), three groups of immune mice consisting of eight mice each were treated with either anti-CD4/CD8, rat IgG2a, or PBS as described above. Five days prior to secondary infectious challenge, mice were treated with Depo-Provera as described above. At 56 days following primary infection, mice were rechallenged vaginally with 100 ID50 of C. trachomatis MoPn, and the course of secondary infection was monitored by enumerating infectious chlamydiae from cervicovaginal swabs (21). Also, at the time of secondary infectious challenge, a group of five naive mice were infected and served as nonimmune control animals for the secondary challenge. For each group of eight mice, five mice were used to monitor the course of infection (vaginal cultures); three mice were sacrificed 7 days following secondary challenge, and spleens and livers were removed, homogenized, and cultured for chlamydiae. At 17 days post-secondary challenge, the remaining five mice in each group were tested for delayed-type hypersensitivity (DTH) responses to chlamydial antigen (described below). At 20 days post-secondary challenge, mice were bled and then euthanized, and genital tracts were removed for immunohistochemical staining.
Immunohistochemistry.
Immunohistochemistry was used to monitor the in vivo depletion of lymphocyte subsets. Blood and genital tract tissues were collected at the indicated times and processed for immunohistological staining as previously described (22, 23). CD4+ T cells and CD8+ T cells were visualized using the Vectastain ABC peroxidase complex (Vector Laboratories, Burlingame, Calif.) and anti-CD4 (clone RM4-5) or anti-CD8 (clone 53-6.7), respectively (Pharmingen, San Diego, Calif.). Rat IgG2a (clone R35–95) (Pharmingen) was used as a negative isotype control antibody.
DTH.
DTH responses were assessed by injecting the hind footpads of groups of mice with 25 μl of either buffer or heat-inactivated (80°C, 30 min) MoPn elementary bodies as described previously (21). Footpad swelling represents the difference in footpad thickness prior to inoculation and at 24 h postinoculation.
Antichlamydial serological response.
Serum antibody responses to MoPn elementary bodies were measured by enzyme-linked immunosorbent assay (ELISA) as described previously (21).
Statistical analysis.
Student's t test was used to analyze IFU counts and DTH responses of control and experimental groups.
RESULTS AND DISCUSSION
Immune B6 mice resolve secondary chlamydial genital tract infection despite the depletion of CD4+ or CD8+ T cells prior to reinfection (23). Conversely, B-cell-deficient gene knockout mice are unable to eradicate secondary infection in the absence of immune CD4+ T cells (23). Those findings imply that both CD4+ T cells and B cells contribute to immune protection against chlamydial reinfection. The important role of B cells in CD4- or CD8-depleted wild-type B6 mice is inferred by the results from B-cell-deficient mice. An alternative plausible explanation for those data is that depletion of one immune T-cell subset (i.e., CD4+ T cells) is compensated for by another immune T-cell subset (i.e., CD8+ T cells). Thus, to test that hypothesis, immune B6 mice were simultaneously depleted of both CD4+ and CD8+ subpopulations of T cells prior to secondary infection. Primary chlamydial genital tract infection of B6 mice resolves in approximately 4 weeks (21, 23). Following the resolution of primary infection, and at a time when mice exhibit a significant level of protective immunity (21, 23), CD4+ and CD8+ T cells were depleted, and mice were rechallenged with infectious chlamydiae. Concurrent depletion of both CD4+ and CD8+ T cells had only a very limited effect on the course of secondary infection (Fig. (Fig.1).1). A marginally increased recovery of IFUs was noted in the anti-CD4/CD8-treated group compared to PBS-treated mice on day 3 post-secondary infection (P < 0.05), but those data were not significantly different from that of the rat IgG-treated group at that time. All animals, regardless of in vivo treatment, resolved infection by 7 to 14 days postchallenge and shed far fewer infectious chlamydiae (>3 log10 decrease) compared to primary-infection controls (naive controls, Fig. Fig.1).1). Thus, the ability of mice to resolve secondary chlamydial genital tract infection was not compromised by the depletion of immune CD4+ and CD8+ subpopulations of T cells.
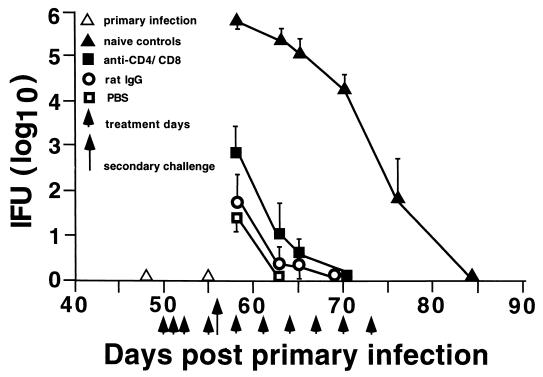
Effect of anti-CD4/CD8 depletion on the resolution of secondary chlamydial genital tract infection. Following the resolution of primary C. trachomatis genital tract infection (day 50), the in vivo treatment regimen was initiated. Treated mice were reinfected with chlamydiae on day 56 post-primary infection, and infection was monitored by swabbing the vaginal vault and enumerating IFU. Data are presented as log10 IFU ± standard error of the mean for five mice per group. The data for the naive control group of mice depict the course of primary infection.
To ensure that the depletion of T-cell subsets was maintained for the duration of the infection, peripheral blood was monitored for CD4+ T cells and CD8+ T cells throughout the period of antibody treatment. Mice treated with anti-CD4/CD8 were depleted of peripheral blood CD4+ T cells and CD8+ T cells throughout the duration of antibody treatment (Table (Table1).1). The ratio of CD4+ T cells and CD8+ T cells in peripheral blood was unaffected by PBS or rat IgG treatment. Because the resolution of secondary infection in anti-CD4/CD8-treated mice was nearly identical to that of untreated mice, it was necessary to confirm that T-cell subpopulations were also depleted in the local genital tract tissues. Immunohistochemical staining for CD4+ T cells and CD8+ T cells confirmed that the antibody treatment regimen effectively depleted genital tract T-cell subpopulations (Fig. (Fig.2).2). Thus, the resolution of secondary chlamydial genital tract infection in mice depleted of CD4+ and CD8+ T cells could not be attributed to the ineffective depletion of T-cell subsets.
TABLE 1
In vivo depletion of T-cell subpopulationsa
Treatment | Mean % positive staining cells on indicated day after secondary infectious challenge
| |||||||||||||
---|---|---|---|---|---|---|---|---|---|---|---|---|---|---|
Pretreatment
| 1
| 5
| 9
| 13
| 16
| 20
| ||||||||
CD4 | CD8 | CD4 | CD8 | CD4 | CD8 | CD4 | CD8 | CD4 | CD8 | CD4 | CD8 | CD4 | CD8 | |
PBS | 21 | 13 | 20 | 15 | 25 | 13 | 25 | 18 | 24 | 16 | 20 | 14 | 22 | 17 |
Rat IgG | 22 | 11 | 26 | 17 | 26 | 17 | 25 | 18 | 24 | 17 | 19 | 17 | 21 | 19 |
Anti-CD4/CD8 | 21 | 12 | 0 | 0 | 0 | 0 | 1 | 2 | 0 | 0 | 1 | 1 | 0 | 0 |
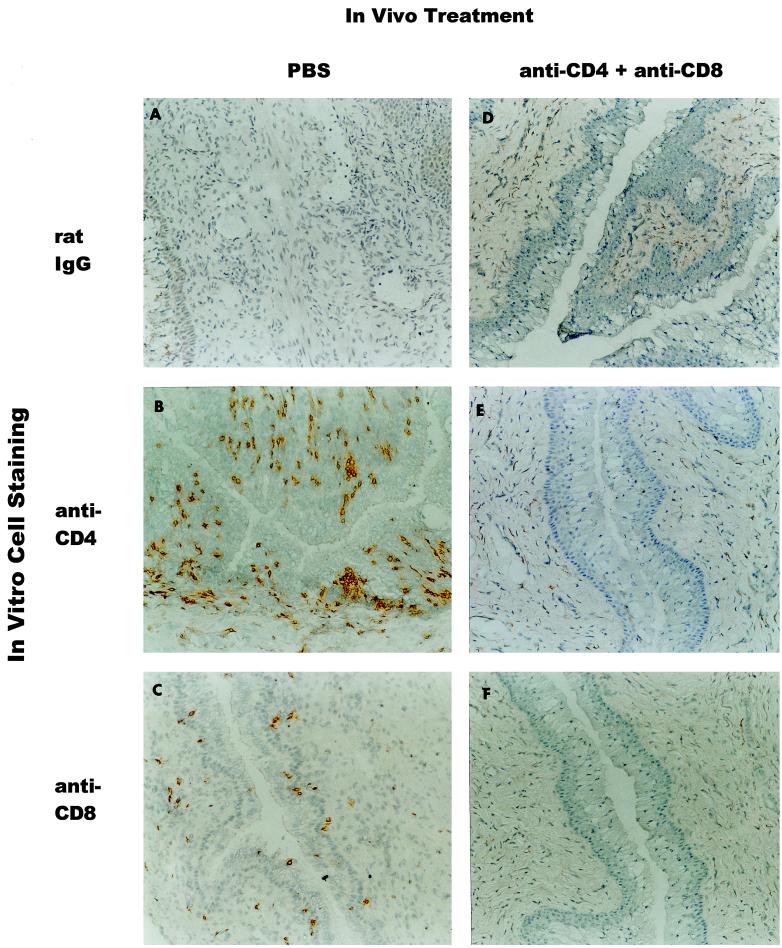
Depletion of CD4+ T cells and CD8+ T cells in genital tract tissues of anti-CD4/CD8-treated mice following secondary infectious challenge. Twenty days following secondary infectious challenge, five mice from each treatment group were sacrificed and genital tracts were processed for immunohistochemical staining. Data are representative of vaginal tissues from four or five mice. Uterine tissues were similarly depleted. In vivo treatment was done with PBS (A to C) or anti-CD4/CD8 (D to F). In vitro immunohistochemical staining was done with control rat IgG (A and D), anti-CD4 (B and E), and anti-CD8 (C and F). Original magnification, ×300.
To assess the functional effect of CD4 and CD8 depletion on chlamydia-specific cell-mediated and humoral immunity, animals were tested for DTH and antibody responses, respectively. Seventeen days following secondary infectious challenge, a time when anti-CD4/CD8-treated mice were depleted of T-cell subpopulations, mice were tested for chlamydia-specific DTH. The DTH response of CD4/CD8-depleted mice was significantly (P < 0.05) lower than the markedly positive DTH responses of PBS-treated and rat IgG-treated groups of mice and was not significantly different from that of naive (noninfected) mice (Fig. (Fig.3).3). Humoral antichlamydial responses were measured at 20 days post-secondary challenge. Except for a few minor variations in titer, such as a heightened IgG1 antichlamydial response in the rat IgG-treated group, overall the antibody response of anti-CD4/CD8-treated mice was remarkably similar to that of PBS-treated and rat IgG-treated groups of mice (Fig. (Fig.4).4). These data confirm that anti-CD4/CD8 treatment diminished chlamydia-specific cell-mediated immunity but had no apparent effect on the humoral immune response. Thus, mice with impaired cell-mediated immunity resolved secondary chlamydial genital tract infection with kinetics nearly indistinguishable from those of immunocompetent wild-type mice.
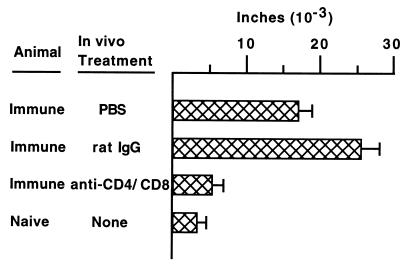
DTH responses in treated groups of mice following secondary chlamydial genital tract infection. Seventeen days following secondary infectious challenge, groups of mice were tested for Chlamydia-specific DTH responses. Data are presented as the mean ± standard error of the mean (five mice per group) of the difference in footpad thickness before inoculation and at 24 h. Immune anti-CD4/CD8-treated mice exhibited DTH responses that were not significantly different from those of naive mice and which were markedly diminished compared to those of immune PBS-treated and immune rat IgG-treated mice (P < 0.05).
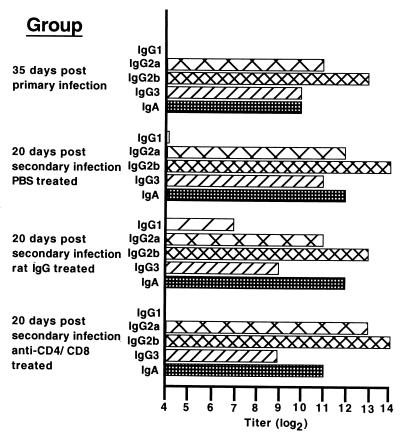
Serum immunoglobulin class and subclass-specific anti-Chlamydia response. Antibody titers are expressed as the inverse of the highest serum dilution (log2) giving an absorbance at 405 nm of at least 0.3. Data are presented as the mean titer of duplicate determinations of pooled serum from groups of five mice. The titer was identical for duplicate determinations.
Anti-CD4/CD8 treatment severely compromises chlamydial cell-mediated immune responses (Fig. (Fig.3).3). Previous studies have shown that some strains of immunocompromised mice essentially resolve chlamydial genital tract infection but are incapable of preventing the subsequent dissemination of chlamydial infection to distant organs, such as the liver and spleen (8, 26). Even though all groups of mice in our current study resolved secondary infection of the genital tract, it was important to determine whether they also precluded the dissemination of chlamydial infection. The spleens and livers of three mice from each group of animals were harvested at 7 days post-secondary infection and cultured for infectious chlamydiae. Infectious chlamydiae were never cultivated from tissue homogenates of any of the groups of treated mice (data not shown). Thus, animals immunocompromised by anti-CD4/CD8 treatment resolved secondary chlamydial genital tract infection and prevented dissemination of infection to distant sites.
Defining the immune mechanisms that protect against chlamydial infection is fundamentally important to the development of a protective vaccine. Toward that goal, two experimental approaches are generally used: (i) genital tract infection of immunocompromised mice and (ii) infection of naive mice that have been passively immunized with immune cells. For example, through the use of specific gene knockout mice, it is clear that major histocompatibility complex class II-restricted responses, αβT cells, and to some extent gamma interferon are required for the development of immune responses that lead to resolution of primary chlamydial genital tract infection (8, 21, 26). The passive transfer of immune T cells or chlamydia-pulsed dendritic cells to naive recipient mice before primary genital tract infection confers a level of immune protection which is characterized by decreased bacterial shedding and infection of shortened duration (14, 36, 39). Thus, both approaches have provided data demonstrating the prominent role of cell-mediated immunity in the resolution of chlamydial genital tract infection.
The concept that cell-mediated immune responses alone impart immunity to intracellular pathogens has recently been challenged. A number of very provocative studies demonstrate a protective role for specific antibody and/or B cells in mediating immunity to infection by intracellular bacterial pathogens and provide new insight into plausible mechanisms of host resistance to intracellular microbial infection (9, 10, 20, 43–45). The notion that specific antibody confers a level of protective immunity to chlamydial infection is not new but has been overshadowed in large part by the plethora of studies documenting the importance and dominance of cell-mediated immune responses in resolving primary chlamydial genital tract infection (2, 28). Previous studies using the guinea pig model of chlamydial genital tract infection demonstrate that humoral immune responses confer a level of protective immunity to reinfection in T-cell-depleted guinea pigs (29, 30). Although a protective role for both T cells and B cells is implied from those studies, the outcome of secondary chlamydial reinfection in T-cell-depleted animals differs between the murine and guinea pig models. The resolution of secondary reinfection in immune T-cell-depleted mice is remarkably similar to that in nondepleted mice (Fig. (Fig.1),1), whereas secondary reinfection of immune T-cell-depleted guinea pigs results in an infection of longer duration compared to untreated guinea pigs (30). Because of the many differences that exist between the murine and guinea pig models of infection and the methods of experimental analysis, it is difficult to directly compare the findings from the two infection models. Nevertheless, B cells and/or antibody appears to impart a level of protective immunity to chlamydial genital tract infection in both animal models.
Using an in vivo approach to define immune mechanisms that confer resistance to chlamydial genital tract reinfection, we previously confirmed that CD4+ T cells are important in antichlamydial immunity and also provided compelling evidence that B cells, or their products, are fundamentally important to immune protection in the murine model of genital tract infection (23). In our current study, we extend those findings by demonstrating that immune mice depleted of both CD4+ and CD8+ T cells, which exhibit diminished antichlamydial cell-mediated immunity, resist a secondary infectious challenge nearly as well as immune wild-type mice (Fig. (Fig.11 and and3).3). Those data provide further indirect evidence that B cells are an important element of immune protection to murine chlamydial genital tract infection. The mechanism by which B cells confer protection against genital tract reinfection has not been defined. Further investigations are needed to determine whether B cells and/or B-cell products function independently or in combination with other cell populations (e.g., T cells) to resolve chlamydial infection and if similar immune mechanisms function in resolving human chlamydial infection.
ACKNOWLEDGMENT
This work was supported by National Institutes of Health grant AI38991 (R.P.M.).
REFERENCES
Articles from Infection and Immunity are provided here courtesy of American Society for Microbiology (ASM)
Full text links
Read article at publisher's site: https://doi.org/10.1128/iai.69.4.2643-2649.2001
Read article for free, from open access legal sources, via Unpaywall:
https://europepmc.org/articles/pmc98202?pdf=render
Citations & impact
Impact metrics
Citations of article over time
Article citations
Immunity to Sexually Transmitted Bacterial Infections of the Female Genital Tract: Toward Effective Vaccines.
Vaccines (Basel), 12(8):863, 01 Aug 2024
Cited by: 0 articles | PMID: 39203989 | PMCID: PMC11359697
Review Free full text in Europe PMC
Protective anti-chlamydial vaccine regimen-induced CD4+ T cell response mediates early inhibition of pathogenic CD8+ T cell response following genital challenge.
Pathog Dis, 82:ftae008, 01 Feb 2024
Cited by: 1 article | PMID: 38684476 | PMCID: PMC11149721
Evaluation of three formulations based on Polymorphic membrane protein D in mice infected with Chlamydia trachomatis.
Front Immunol, 14:1267684, 16 Nov 2023
Cited by: 0 articles | PMID: 38045697 | PMCID: PMC10690417
Plasmid-mediated virulence in Chlamydia.
Front Cell Infect Microbiol, 13:1251135, 17 Aug 2023
Cited by: 6 articles | PMID: 37662000 | PMCID: PMC10469868
Review Free full text in Europe PMC
A Machine Learning-Based Analytic Pipeline Applied to Clinical and Serum IgG Immunoproteome Data To Predict Chlamydia trachomatis Genital Tract Ascension and Incident Infection in Women.
Microbiol Spectr, 11(4):e0468922, 15 Jun 2023
Cited by: 2 articles | PMID: 37318345 | PMCID: PMC10434056
Go to all (75) article citations
Similar Articles
To arrive at the top five similar articles we use a word-weighted algorithm to compare words from the Title and Abstract of each citation.
Immunity to murine Chlamydia trachomatis genital tract reinfection involves B cells and CD4(+) T cells but not CD8(+) T cells.
Infect Immun, 68(12):6979-6987, 01 Dec 2000
Cited by: 166 articles | PMID: 11083822 | PMCID: PMC97807
A predominant role for antibody in acquired immunity to chlamydial genital tract reinfection.
J Immunol, 175(11):7536-7542, 01 Dec 2005
Cited by: 154 articles | PMID: 16301662 | PMCID: PMC3514507
Immunological memory in B-cell-deficient mice conveys long-lasting protection against genital tract infection with Chlamydia trachomatis by rapid recruitment of T cells.
Immunology, 102(2):199-208, 01 Feb 2001
Cited by: 21 articles | PMID: 11260325
Cellular immunity and Chlamydia genital infection: induction, recruitment, and effector mechanisms.
Int Rev Immunol, 22(1):3-41, 01 Jan 2003
Cited by: 33 articles | PMID: 12710502
Review
Funding
Funders who supported this work.
NIAID NIH HHS (3)
Grant ID: R01 AI038991
Grant ID: AI38991
Grant ID: R56 AI038991