Abstract
Free full text

A tetracycline derivative, minocycline, reduces inflammation and protects against focal cerebral ischemia with a wide therapeutic window
Abstract
The only treatment of patients with acute ischemic stroke is thrombolytic therapy, which benefits only a fraction of stroke patients. Both human and experimental studies indicate that ischemic stroke involves secondary inflammation that significantly contributes to the outcome after ischemic insult. Minocycline is a semisynthetic second-generation tetracycline that exerts antiinflammatory effects that are completely separate from its antimicrobial action. Because tetracycline treatment is clinically well tolerated, we investigated whether minocycline protects against focal brain ischemia with a wide therapeutic window. Using a rat model of transient middle cerebral artery occlusion, we show that daily treatment with minocycline reduces cortical infarction volume by 76 ± 22% when the treatment is started 12 h before ischemia and by 63 ± 35% when started even 4 h after the onset of ischemia. The treatment inhibits morphological activation of microglia in the area adjacent to the infarction, inhibits induction of IL-1β-converting enzyme, and reduces cyclooxygenase-2 expression and prostaglandin E2 production. Minocycline had no effect on astrogliosis or spreading depression, a wave of ionic transients thought to contribute to enlargement of cortical infarction. Treatment with minocycline may act directly on brain cells, because cultured primary neurons were also salvaged from glutamate toxicity. Minocycline may represent a prototype of an antiinflammatory compound that provides protection against ischemic stroke and has a clinically relevant therapeutic window.
Ischemic stroke is the third leading cause of death in western industrialized countries and a major cause of long-lasting disability (1, 2). The only preventive treatment of stroke is antiplatelet therapy for patients with transient ischemic attack or stroke, which produces a modest but clinically worthwhile benefit (3). In acute stroke, only a small fraction of patients benefit from intravenous administration of recombinant tissue plasminogen activator, which is the only drug with proven effectiveness in reducing the size of infarct in humans (4, 5). Even though a large number of different compounds have been proven to reduce the size of brain infarct in animal studies, replication of the experiments with the neuroprotectives in humans have regularly failed. The reasons for the unsuccessful clinical trials have been either the toxic side effects, which have overridden the neuroprotective potential of the compounds determined in animals, or a limited time window for human therapy. Therefore, the search is on for compounds with no or tolerable side effects combined with a protective potential when administered several hours after ischemic insult.
Recent studies have indicated that brain ischemia, especially a clinically common focal stroke caused by occlusion of the middle cerebral artery (MCA), involves secondary inflammation that significantly contributes to the outcome after ischemic insult (6–10). Because the inflammatory response is a delayed process, the molecules participating in this secondary response are potential targets for human therapy with a sufficiently wide therapeutic window. These molecules include cyclooxygenase-2 (COX-2), an inducible prostaglandin-producing enzyme (9, 11), and IL-1β, a proinflammatory cytokine released mainly by microglia after ischemia (12).
Minocycline is a semisynthetic second-generation tetracycline that exerts antiinflammatory effects that are completely separate and distinct from its antimicrobial action (13–15). The drug is clinically well tolerated and is currently considered for treatment of rheumatoid arthritis, a severe inflammatory human disease (16). We have recently shown that very high doses of doxycycline and minocycline reduce the loss of hippocampal pyramidal neurons when administered before or within 30 min of the insult in a gerbil model of global ischemia (mimicking cardiac arrest; ref. 10). Herein, we show that minocycline at relatively low doses is very effective neuroprotective drug against focal ischemia even when the administration is started 4 hours after the insult, indicating a clinically relevant therapeutic time window for this tetracycline derivative. In addition, we report that the beneficial effect is associated with reduction of COX-2 expression and prostaglandin production and with decreased induction of IL-1β-converting enzyme (ICE) in microglia, which remain in resting stage when the animal is treated with minocycline. Further, minocycline reduces glutamate neurotoxicity in cell cultures, indicating that the neuroprotection does not depend on its potential effects on blood vessels and circulatory cells.
Materials and Methods
Animals.
Male Sprague–Dawley rats weighing 210–250 g were housed at a standard temperature (22 ± 1°C) and in a light-controlled environment (lights on from 7:00 a.m. to 9:00 p.m.) with ad libitum access to food and water. The animals were divided randomly into minocycline-treatment and control groups. The experiments were approved by the animal committee at the University of Kuopio.
Induction of Focal Cerebral Ischemia.
Focal cerebral ischemia was produced by introduction of an intraluminal nylon thread. The rats were anesthetized with 5% (vol/vol) isoflurane (70% N2O/30% O2); during the operation, isoflurane concentration was reduced to 0.5%. The rectal temperature was maintained between 37.0°C and 37.5°C with a heating pad. The right common carotid artery was exposed, and the external carotid artery was ligated. A 0.25-mm monofilament nylon thread (Kuusamo Uistin, Kuusamo, Finland) with the tip blunted with sandpaper was inserted 22–23 mm into the internal carotid artery up to the MCA. After 90 min of ischemia, the MCA blood flow was restored by removing the thread. For recording physiological variables, a polyethylene catheter was inserted into the femoral artery. Arterial blood pressure, PO2, PCO2, pH, and plasma glucose were measured during and 15 min after ischemia.
Induction of Spreading Depression (SD).
In a separate set of animals, cortical SD was produced without MCA occlusion. After placing a rat in a stereotaxis frame under halothane anesthesia, a 2-mm craniotomy was made bilaterally, 4 mm lateral to the sagittal suture and 4 mm posterior to the bregma. Without disruption of the dura, the brain was exposed to 3 M KCl for 60 min to induce SD in the right hemisphere. The left hemisphere was exposed to 0.9% (wt/vol) NaCl and served as a control. For recording of extracellular dc potentials, a third craniotomy was made before SD induction 4 mm anterior to the site of KCl exposure. An extracellular tungsten needle electrode (resistance 4.5 MΩ; exposed tip 1.2 mm) was inserted 1.2 mm into the cortex, and the signals were led through a dc amplifier to an instrumentation tape recorder. The data were assessed with the Mann–Whitney U Wilcoxon Rank Sum W test.
Determination of Infarct Volume.
After 3 days of reperfusion, the rats were anesthetized with pentobarbital (60 mg/kg) and killed. The brains were quickly removed and chilled in ice-cold saline for 10 min. Coronal sections (1 mm thick; n = 11) were cut with a tissue slicer, beginning +4.5 mm from the bregma, and the slices were immersed in a saline solution containing 2.0% (vol/vol) 2,3,5-triphenyltetrazolium chloride (Sigma) at 37°C for 20 min. After staining, each slice was scanned by using a Bio-Rad Imaging Densitometer GS-700. The unstained areas in each image were quantified with the multi-analyst 1.02 program, and the infarct volume was calculated by summing up the infarct area in the 11 slices.
Reverse Transcriptase–PCR (RT-PCR).
The Titan One Tube RT-PCR System (Roche Molecular Biochemicals) was applied for the RT-PCR in which 1 μg of DNase-treated (RQ1 RNase-free DNase; Promega) total RNA served as a template in each reaction. To detect the extent of ICE mRNA expression, the specific primers were 5′-CCA GAG CAC AAG ACC TCT GAC-3′ and 5′-TGG TGT GGA AGA GCA GAA AGC-3′ recognizing bases 661–681 (in exon 6) and 978–998 (in exon 7), respectively, in the human sequence. Reverse transcription was done at 50°C for 30 min, and initial denaturation was done at 95°C for 2 min. Altogether, 35 cycles consisting of denaturation (95°C, 1 min), annealing (58°C, 1 min), and polymerization (72°C, 2 min) were carried out. The reaction was concluded with polymerization at 72°C for 10 min. As a reference for the ICE expression, RT-PCR of glyceraldehyde-3-phosphate dehydrogenase (GAPDH) was also done from each sample. The specific GAPDH primers were 5′ACC ACA GTC CAT GCC ATC AC-3′ and 5′-TCC ACC ACC CTG TTG CTG TA-3′ (527–546 and 959–978 in GenBank accession no. J02642). RT-PCR was performed as described above for ICE, with the exception that only 25 cycles were done. The RT-PCRs were performed in PCT-100 Programmable Thermal Controller (MJ Research, Cambridge, MA), and amplification products were analyzed by running 10-μl samples from each reaction on a 2.5% agarose gel. The PCR products were analyzed by using a Bio-Rad Imaging Densitometer GS-700 and the multi-analyst 1.02 program.
Immunohistochemistry.
Free-floating (50-μm) sections were reacted with monoclonal primary antibodies to COX-2 (Transduction Laboratories, Lexington, KY; diluted 1:100) and OX-42 (against CD11b antigen; Serotec, Oxford; diluted 1:1,000). After incubating with biotinylated anti-mouse serum and avidin–biotin complex (Vectastain Elite kit; Vector Laboratories) for 3 h each, the avidin–biotin complex was visualized with 0.05% diaminobenzidine and 0.02% H2O2. After rinsing, the slides were examined with a Leica 3000RB microscope.
Measurement of Prostaglandin E2 (PGE2) Production.
The tissue concentration of PGE2 was determined by using an enzyme immunoassay kit (Cayman Chemicals, Ann Arbor, MI). Samples (50–100 mg) from the infarcted cortex, penumbra, and the contralateral cortex to the MCA occlusion were dissected 24 h after ischemia (as shown in Fig. Fig.2b2b Upper Left). After homogenization in 0.05 M TrisHCl (pH 7.4; 4 ml/g), the tissues were extracted with four volumes of 100% (vol/vol) ethanol and centrifuged. The supernatants were diluted with acidified 0.05 M phosphate buffer (pH 4; eight volumes of ethanol added) and applied to activated ODS-silica reverse phase columns (Sep-Pak C18, Waters). The columns were rinsed with 5 ml of distilled water followed by 5 ml of hexane, and PGE2 was eluted twice with 2 ml of ethyl acetate containing 1% methanol. The ethyl acetate fraction was evaporated to dryness by vacuum centrifugation and resuspended in 1 ml of buffer. PGE2 concentration was determined in duplicate samples according to the instructions provided with the kit.
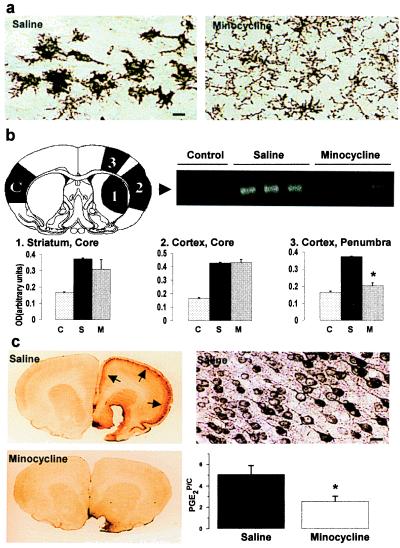
(a) Minocycline treatment inhibits ischemia-induced activation of microglia. Amoeboid-shaped CD11b-immunoreactive cells are seen around the infarction core 24 h after ischemia in saline-treated, but not in minocycline-treated, animals. (Bar = 20 μm.) (b) The ischemia-induced expression of ICE mRNA is prevented by minocycline treatment. The numbers and letter C in the brain section (B Upper Left) show the site of samples: (1) infarcted core in the striatum, (2) infarcted core in the cortex, (3) the cortical area adjacent to ischemia core (penumbra), and (C) the contralateral cortex used as control tissue. The arrowhead in the gel (B Upper Right) points to RT-PCR amplification products of ICE mRNA detected in the samples of the contralateral cortex (control) and in the penumbra (area 3) of saline- and minocycline-treated animals 12 h after ischemia. The histograms (B Lower) show quantitation of ICE bands derived from the three different regions of the ischemic hemisphere. Minocycline treatment (M) significantly reduced expression of the ICE message in the penumbra compared with expression after saline treatment (S; n = 4; , P < 0.01, one-way ANOVA followed by Bonferroni test; C refers to control tissue). (c) Minocycline-treatment prevents ischemia-induced COX-2 immunoreactivity and PGE2 production in the penumbra. COX-2 immunoreactivity (arrows in C Upper Left and at higher magnification in C Upper Right) is seen in cortical neurons 24 h after ischemia. At the same time point, the increase in PGE2 concentration (C Lower Right) is reduced by 51% (
, P < 0.01, one-way ANOVA followed by Bonferroni test). The results are from three different experiments (n = 5 + 5 in each). PGE2P/C, ratio of PGE2 concentrations in penumbra and the contralateral cortex.
Primary Neuronal Cultures.
Spinal cords were excised from 14-day-old rat embryos (Wistar; University of Kuopio), and the meninges and dorsal root ganglia were removed. Tissues were minced and trypsinized (0.25% trypsin-EDTA in 0.1 M PBS; GIBCO/BRL) for 15 min at 37°C. After centrifugation for 5 min at 1,000 rpm (Labofuge GL, Heraeus, Hannover, Germany), the tissues were resuspended in DMEM (high glucose; GIBCO/BRL) containing 10% (vol/vol) FBS and 10% (vol/vol) heat-inactivated horse serum and then triturated with a fire-polished Pasteur pipette. Single-cell suspension was collected; the cells were counted with a Bürker hemocytometer and diluted to the density of 1 × 106 cells per ml. The cells were plated into cell culture plates (1 × 105 cells per well in a 96-well plate) and maintained at 37°C in a 7.5% CO2 incubator. The medium was changed the next day to a medium containing 5% of both sera. After 4 days in vitro, 5 μM cytosine β-d-arabinofuranoside (Sigma) was added for 24 h. The cultures were exposed to 500 μM glutamate with and without minocycline pretreatment (0.02 μM). The total neuronal cell death was quantified by measuring lactate dehydrogenase (cytotoxicity detection kit, Roche Molecular Biochemicals) in culture medium 20–24 h after glutamate exposure.
Results and Discussion
Treatment with minocycline (45 mg/kg i.p. twice a day for the first day; 22.5 mg/kg for the subsequent 2 days) did not affect rectal temperature, arterial blood pressure, plasma glucose, or arterial blood gases (Fig. (Fig.11a). However, the treatment started 12 h before ischemia reduced the size of the infarct in the cerebral cortex by 76% and in the striatum by 39% (Fig. (Fig.11 b and c). Starting the minocycline treatment 2 h after the onset of ischemia resulted in a reduction in the size of cortical (by 65%) and striatal (by 42%) infarct, a reduction similar to the one obtained with pretreatment. The cortical infarct size was reduced by 63% even when the treatment was started 4 h after the onset of ischemia. These results indicate that minocycline treatment provides significant protection against focal ischemia and has a wide therapeutic window. The protection did not involve hypothermia, because the rectal temperature remained unaltered even 24 h after starting the treatment (saline-treated, 36.94 ± 0.28; minocycline-treated, 37.07 ± 0.33; P > 0.05, Student’s t test). Also, judging from the fact that postischemic treatment was equally effective as pretreatment, changes in the cerebral blood flow during ischemia played no role in the protective mechanism.
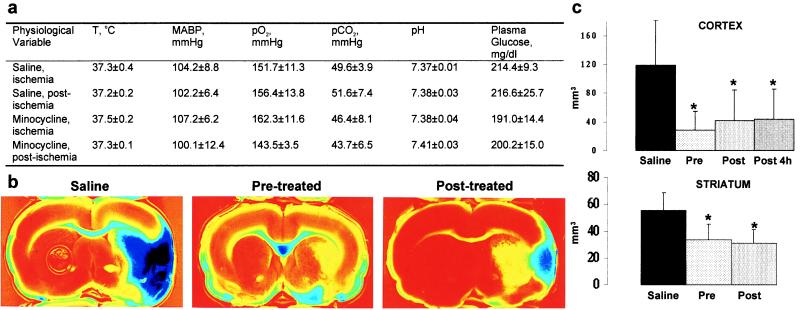
(a) Minocycline treatment started 12 h before ischemia does not alter body temperature (T), mean arterial blood pressure (MABP), arterial PO2, PCO2, pH, or plasma glucose, as determined by measurements taken during the ischemia or 15 min after the ischemia (n = 5 in each group). (b) Minocycline treatment started 12 h before (pretreated) or 2 h after (posttreated) the onset of ischemia reduces the infarction area seen in triphenyltetrazolium chloride-stained brain slices 3 days after ischemia compared with saline-treated animals. (c) The infarction area is significantly reduced in the cortex and striatum compared with saline-treated animals when the minocycline treatment is started 12 h before ischemia (Pre, n = 10), 2 h after the onset of ischemia (Post, n = 10), or 4 h after the onset of ischemia (Post 4 h, n = 12). Values are means ± SD. *, P < 0.01 (one-way ANOVA followed by the Bonferroni test).
We next studied whether minocycline affects cortical SD, which is an energy-consuming wave of transient depolarizations of astrocytes and neurons and which contributes to the evolution of ischemia to infarction in this model of focal ischemia (17–19). Compounds that inhibit cortical SD by blocking either N-methyl-d-aspartic acid-receptor (NMDA)-type glutamate receptors or gap junctions, both of which are needed for cortical SD, reduce the size of the cortical infarct (17–19). Previous studies have shown that cortical SDs evoked chemically in the intact region of the cortex are prolonged when reaching ischemic penumbra and become indistinguishable from periinfarct SDs, which rise spontaneously from ischemic tissue (19, 20). In a separate set of rats that were not subjected to MCA occlusion, the effect of minocycline was tested on KCl-induced cortical SD. On a 60-min exposure to topical 3 M KCl, the number, duration, and amplitude of dc potentials (mean ± SD) in control animals (n = 5) were 5.4 ± 2.98, 48.8 ± 16.34 s, and 9.75 ± 5.34 mV, respectively, and the corresponding values in minocycline-treated animals (n = 5) were 4.1 ± 1.7, 45.7 ± 18 s, and 8.8 ± 4.5 mV, respectively. The values do not show statistically significant differences between control and minocycline-treated animals, whereas MK-801, an NMDA receptor antagonist known to reduce partially ischemic damage by blocking cortical SD, completely prevented KCl-induced dc potentials (not shown).
As nonneuronal cells are characteristically activated in the brain in response to ischemic injury (21–23), we studied astrogliosis and microglial activation by using antibodies to glial fibrillary acidic protein (GFAP) and CD11b (OX-42) as histochemical markers. At 24 h after 90 min of ischemia, a strong induction of CD11b immunoreactivity was observed around and inside the infarction core in untreated rats. The CD11b-immunoreactive cells had an amoeboid shape in the penumbra zone. Minocycline treatment started 12 h before ischemia decreased the number of CD11b-immunoreactive cells and prevented the appearance of the amoeboid-shaped microglia adjacent to the infarction core. Instead, GFAP-immunoreactivity in the ischemic hemispheres of untreated and minocycline-treated animals was similarly increased (not shown). These results suggest that minocycline inhibits microglial activation without interfering with early astrogliosis.
Activated microglia precede the manifestation of tissue injury and may exert a cytotoxic effector function by producing proinflammatory cytokines, such as IL-1β, which is a major cytokine produced after ischemia (8, 12, 21–23). To determine whether minocycline also inhibited the expression of ICE, the enzyme that cleaves the inactive pro form to the active mature IL-1β (11, 24), a semiquantitative RT-PCR for ICE mRNA was done. A 2.5-fold increase in ICE mRNA was detected in the ischemic core and in the penumbra 12 h after the insult. Pretreatment with minocycline decreased the induced ICE mRNA levels by 83% in the penumbra, indicating that minocycline treatment inhibits expression of the enzyme needed for IL-1β activation in microglia (Fig. (Fig.22b).
COX-2 is highly expressed in the ischemic brain and produces proinflammatory prostaglandins such as PGE2 (9, 17). In general, expression of COX-2 is reduced by antiinflammatories and can be induced by cytokines, including IL-1β (25). Because minocycline treatment inhibited microglial activation, we studied whether the treatment also affects COX-2. In untreated rats, the PGE2 concentration was increased 5-fold in the ischemic penumbra and was preceded by the induction of COX-2 immunoreactive neurons. Pretreatment with minocycline reduced the PGE2 concentration in the penumbra by 55% and almost completely prevented the appearance of COX-2 immunoreactivity (Fig. (Fig.22c).
Tetracyclines, including minocycline, have been shown to inhibit matrix metalloproteinases (MMP) and possibly superoxide production in polymorphonuclear neutrophils (PMNs; refs. 13 and 26). Because MMP-9 (gelatinase B) is induced in neutrophils and endothelial cells in early focal ischemia and because MMP-9 together with free radicals derived from PMNs may contribute to ischemic damage by increasing the permeability of the blood–brain barrier (27, 28), minocycline could provide neuroprotection by inhibition of these peripheral mechanisms. We therefore studied whether minocycline protects neurons against glutamate in primary neuronal cultures. We chose to use cultures that consisted of neurons (70%), astrocytes (24%), and microglia (6%) and that were devoid of endothelial cells and peripheral cells, because previous studies have shown that microglia may contribute to excitotoxicity (29). When the cultures were pretreated with 0.02 μM minocycline, the neurotoxicity of 500 μM glutamate, a major mediator of neuronal death in the brain, was decreased by 85% (n = 3 sister cultures in each group; P < 0.05, Student’s t test). Therefore, minocycline provides major neuroprotection against excitotoxicity in mixed brain cell cultures by a mechanism that is independent of peripheral systems.
Previous studies have shown that minocycline and synthetic tetracycline derivatives that are devoid of antibacterial activity may be beneficial in rheumatoid arthritis, osteoporosis, and periodontal disease (13, 14). In light of the present results, minocycline or synthetic tetracycline derivatives might serve as new therapeutic strategies for the treatment of stroke. The exact mechanism that mediates the salvage of the ischemic tissue by minocycline is unclear, but inhibition of microglial activation may play a crucial role. Microglia are resident tissue macrophages of the central nervous system comprising up to 20% of the glial cell population in the rodent brain (30). They become activated in various types of brain injury including trauma, Alzheimer’s disease, multiple sclerosis, and ischemia and exert a cytotoxic function by releasing reactive oxygen species, proteolytic enzymes, arachidonic acid metabolites, and inflammatory cytokines (21, 23). One of these cytokines, IL-1β, is activated by ICE, which was found to be down-regulated by minocycline. The reduction of COX-2 expression and PGE2 production by minocycline may be indirect via microglial and ICE deprivation, because IL-1β is thought to contribute to COX-2 induction in focal brain ischemia (31). In addition, the possibility that the reduction in COX-2 expression results from the fact that minocycline-treated rats have smaller infarcts—and, consequently, the inflammatory reaction is less pronounced—cannot be excluded. This hypothesis is supported by in vitro experiments on macrophages, which suggest that the direct effect of tetracyclines on COX-2 is enhancement rather than inhibition (32).
Recently, ischemic focal damage has been found to mature and enlarge for several days, which at least partially is a result of the development of inflammatory responses during the first 2 days after ischemia (31). The relevance of inflammation as a late contributing mechanism in brain ischemia has been proved by previous studies, which show that compounds that inhibit COX-2 or inducible nitric oxide synthase (iNOS) are protective in focal brain ischemia even when administered several hours after the insult (9, 33). Our previous studies have shown that, in global ischemia of gerbils, minocycline reduces iNOS expression (10), which may contribute to the neuroprotective effect of minocycline. Our preliminary results suggest that, in focal brain ischemia, minocycline treatment reduces iNOS expression in the penumbra region only minimally and without statistical significance (J.Y., R.K., and J.K., unpublished results). Because the iNOS-producing cells are localized inside infarcted tissue and in a narrow zone at the infarction border (33), inhibition of iNOS as a neuroprotective mechanism in minocycline-treated focal brain ischemia awaits detailed and more careful studies. Altogether, the present study establishes that compounds such as minocycline that reduce expression and/or activity of COX-2, caspases, and IL-1β represent potential therapies against stroke.
Abbreviations
COX-2 | cyclooxygenase-2 |
ICE | IL-Iβ-converting enzyme |
MCA | middle cerebral artery |
PGE2 | prostaglandin E2 |
RT-PCR | reverse transcriptase–PCR |
SD | spreading depression |
Footnotes
This paper was submitted directly (Track II) to the PNAS office.
References
Articles from Proceedings of the National Academy of Sciences of the United States of America are provided here courtesy of National Academy of Sciences
Full text links
Read article at publisher's site: https://doi.org/10.1073/pnas.96.23.13496
Read article for free, from open access legal sources, via Unpaywall:
https://europepmc.org/articles/pmc23976?pdf=render
Citations & impact
Impact metrics
Article citations
Minocycline mitigates sepsis-induced neuroinflammation and promotes recovery in male mice: Insights into neuroprotection and inflammatory modulation.
Physiol Rep, 12(19):e70032, 01 Oct 2024
Cited by: 1 article | PMID: 39370294 | PMCID: PMC11456363
Phase 2 Trial Evaluating Minocycline for Geographic Atrophy in Age-Related Macular Degeneration: A Nonrandomized Controlled Trial.
JAMA Ophthalmol, 142(4):345-355, 01 Apr 2024
Cited by: 0 articles | PMID: 38483382
Tetracyclines enhance antitumor T-cell immunity via the Zap70 signaling pathway.
J Immunother Cancer, 12(4):e008334, 15 Apr 2024
Cited by: 1 article | PMID: 38621815 | PMCID: PMC11328671
MINocyclinE to Reduce inflammation and blood-brain barrier leakage in small Vessel diseAse (MINERVA): A phase II, randomized, double-blind, placebo-controlled experimental medicine trial.
Alzheimers Dement, 20(6):3852-3863, 17 Apr 2024
Cited by: 3 articles | PMID: 38629936 | PMCID: PMC11180856
Genetic contribution to microglial activation in schizophrenia.
Mol Psychiatry, 29(9):2622-2633, 22 Mar 2024
Cited by: 3 articles | PMID: 38519640 | PMCID: PMC11420079
Go to all (694) article citations
Data
Data behind the article
This data has been text mined from the article, or deposited into data resources.
BioStudies: supplemental material and supporting data
Nucleotide Sequences
- (1 citation) ENA - J02642
Similar Articles
To arrive at the top five similar articles we use a word-weighted algorithm to compare words from the Title and Abstract of each citation.
Minocycline inhibits 5-lipoxygenase expression and accelerates functional recovery in chronic phase of focal cerebral ischemia in rats.
Life Sci, 86(5-6):170-177, 16 Dec 2009
Cited by: 26 articles | PMID: 20006627
Interaction between inducible nitric oxide synthase and cyclooxygenase-2 after cerebral ischemia.
Proc Natl Acad Sci U S A, 95(18):10966-10971, 01 Sep 1998
Cited by: 198 articles | PMID: 9724813 | PMCID: PMC28004
Minocycline provides neuroprotection against N-methyl-D-aspartate neurotoxicity by inhibiting microglia.
J Immunol, 166(12):7527-7533, 01 Jun 2001
Cited by: 367 articles | PMID: 11390507
Minocycline and neurodegenerative diseases.
Behav Brain Res, 196(2):168-179, 11 Oct 2008
Cited by: 250 articles | PMID: 18977395
Review