Abstract
Free full text

Viral Density and Virus-to-Bacterium Ratio in Deep-Sea Sediments of the Eastern Mediterranean
Abstract
Viruses are now recognized as a key component in pelagic systems, but their role in marine sediment has yet to be assessed. In this study bacterial and viral densities were determined at nine deep-sea stations selected from three main sites (i.e., the Sporades Basin, the Cretan Sea, and the Ierapetra Trench at depths of 1,232, 1,840, and 4,235 m, respectively) of the Eastern Mediterranean. The three areas were characterized by different phytopigment and biopolymeric carbon concentrations and by changes in the protein and carbohydrate pools. A gradient of increasing trophic conditions was observed from the Sporades Basin (North Aegean) to the Ierapetra Trench (South Aegean). Viral densities (ranging from 1 × 109 to 2 × 109 viruses ml of sediment−1) were significantly correlated to bacterial densities (n = 9, r2 = 0.647) and reached values up to 3 orders of magnitude higher than those generally reported for the water column. However, the virus-to-bacterium density ratio in deep-sea sediments was about 1 order of magnitude lower (range of 2 to 5, with a modal value of 2.6) than in pelagic environments. Virus density decreased vertically with depth in sediment cores at all stations and was below detection limits at the 10-cm depth of the abyssal sediments of the Ierapetra Trench. Virus density in the sediment apparently reflected a gradient of particle fluxes and trophic conditions, displaying the highest values in the Sporades Basin. The low virus-to-bacterium ratios and their inverse relationship with station depth suggest that the role played by viruses in controlling deep-sea benthic bacterial assemblages and biogeochemical cycles is less relevant than in pelagic systems.
Viruses are now considered to be an important component of aquatic microbial communities. The reevaluation of the virus role in marine ecosystems is mostly due to discovery of very abundant viral densities in all pelagic systems, densities that generally exceed bacterial densities by 1 to 2 orders of magnitude (30). Due to their high density (109 to 1010 liter−1) and their high capability of infecting bacteria and phytoplankton, viruses may have profound effects on microbial loop dynamics (14, 29).
An increasing amount of information indicates that viruses might be responsible for ca. 10 to 30% (up to 72%) of bacterial mortality in aquatic systems (18). These processes have cascade effects also on the biogeochemical cycling of organic matter in the marine environment (24). The ability of viruses to destroy bacteria (through infection followed by host cell lysis) is recognized as one of the most relevant mechanisms of DOM release (including dissolved DNA [14]), thus affecting nutrient recycling and the pathways of organic carbon utilization by bacteria.
Previous studies on virus-bacterium interactions in pelagic systems have demonstrated that viral density and capability of infecting bacteria (the so-called “viral-loop” control) increase with increasing trophic conditions, with the highest percentage of infected cells being in highly eutrophic systems (31). However, all studies dealing with virus distributions have been surprisingly restricted to the plankton domain and, excluding one study from Canadian lake sediments (20) and one from interstitial waters (12, 30), no information is available on virus concentration and distribution in the entire sediment matrix.
Epidemiological models predict that viral infection increases with increasing host cell density (32). In this regard, marine sediments could represent the optimal environment for virus development and for testing the hypothesis of a stronger viral loop control with increasing eutrophication. In fact, marine sediments are characterized by (i) high organic matter concentrations, ca. 5 orders of magnitude higher in the sediments than in the water column (ca. 10 to 30 mg of C ml of wet sediment−1 versus 100 to 300 μg of C liter−1 in the water column), (ii) high bacterial densities, ca. 3 orders of magnitude higher than in the water column (generally from 108 to 109 ml of sediment−1 versus 108 to 109 liter−1), and (iii) as a result benthic bacteria display the lowest intercell distance and the probability of virus-bacterium contact is 3 orders of magnitude higher.
Among marine environments, deep-sea sediments, covering about 50% of the world's surface, are responsible for processes controlling organic carbon cycling on a global scale, and knowledge of the mechanisms regulating benthic bacteria is essential for a better understanding of organic matter diagenesis (10). Deep-sea benthic bacteria are assumed to be controlled “bottom-up” (by the flux of organic carbon from the photic layer [11] and by organic matter availability [5]) and/or “top-down” (e.g., by nanoflagellate grazing [7]) and possibly by viral infection, although this latter hypothesis is yet to be tested. In this study we examined deep-sea sediment viral and bacterial densities and the biochemical composition of the sedimentary organic matter (measured as lipids, proteins, carbohydrates, and phytopigments), comparing highly oligotrophic bathyal sediments with deep-sea trenches characterized by benthic hot spots of microbial activity (3). The specific aims of this investigation were (i) to gather information about the relative significance of bacteria and viruses in deep-sea sediments and (ii) to identify their possible interactions and the role of different environmental conditions in controlling the virus-to-bacterium density ratio.
MATERIALS AND METHODS
Study area and sampling.
Sediment samples were collected in the Aegean Sea (Eastern Mediterranean) between 28 December 1997 and 18 January 1998. The sampling strategy included three areas characterized by different ecological conditions: the Sporades Basin (average depth of sampled area, 1,232 m), the Ierapetra Basin (average depth of sampled area, 4,235 m), and the Cretan Sea (average depth of sampled area, 1,840 m) (Table (Table1).1). The Sporades Basin is located in the northwestern Aegean and is characterized by nutrient-rich waters coming from the Black Sea. The Cretan Sea is considered to be one of the most oligotrophic areas of the Mediterranean, due to the extremely low primary productivity (7, 13). Finally, the Ierapetra Basin is located southeast of Crete and is considered a trap for particulate matter and a benthic microbial “hot-spot” area (3). The sampling schedule included three stations in the Sporades Basin, four stations in the Ierapetra Basin, and two stations in the Cretan Sea (Table (Table1).1). Undisturbed sediment cores were collected using a multicorer. Immediately after sampling, sediment corers were vertically sectioned into six layers: 0 to 0.3, 0.3 to 1, 1 to 2, 2 to 4, 4 to 6, and 6 to 10 cm in depth. For bacterial and viral analysis, replicate subsamples (n = 3) of ca. 0.5 ml were added to 3 ml of prefiltered (0.02-μm [pore size]) seawater containing 2% formalin and stored at 4°C.
TABLE 1
Stations location, depth, water content, and porosity in surface sediments (0 to 0.3 mm) at the different locations of the AegeanSea
Area | Station | Latitude (north) | Longitude (east) | Depth (m) | Water content (%) | Porosity |
---|---|---|---|---|---|---|
Sporades Basin | 1 | 39°16.53′ | 23°42.46′ | 1,250 | 38.7 | 0.62 |
2 | 39°15.50 | 23°42.48′ | 1,221 | 29.4 | 0.52 | |
3 | 39°14.97′ | 23°42.55′ | 1,224 | 32.5 | 0.56 | |
Cretan Sea | 8 | 35°49.01′ | 25°15.97′ | 1,840 | 34.2 | 0.57 |
9 | 35°50.52′ | 25°15.99′ | 1,840 | 34.6 | 0.58 | |
Ierapetra Trench | 4 | 34°26.01′ | 26°03.99′ | 4,157 | 30.8 | 0.54 |
5 | 34°25.00′ | 26°04.00′ | 4,260 | 32.6 | 0.56 | |
6 | 34°24.54′ | 26°04.52′ | 4,261 | 37.2 | 0.61 | |
7 | 34°24.85′ | 26°05.79′ | 4,263 | 30.3 | 0.53 |
For the analysis of the biochemical composition of sedimentary organic matter, two replicate cores were collected at each station, vertically sectioned as described above, placed in sterile petri dishes, and frozen at −20°C until analyzed. Sediments were dried at 60°C until achieving a constant weight. Sediment water content (wc) was calculated as the difference between the wet and dry weights and was expressed as a percentage. Sediment porosity was determined according to the following equation: (wc/1.02)/{[(1 − wc)/2.64] + wc/1.02}, where the wc is equal to (wet sediment weight − dry sediment weight)/wet sediment weight (9).
Phytopigment analysis.
Chloroplastic pigments (chlorophyll a and pheopigments) were determined according to the method of Lorenzen and Jeffrey (19). Pigments were extracted from ca. 1 g of sediment with 90% acetone overnight. After centrifugation (800 × g), the supernatant was used to determine the chlorophyll a concentration and then acidified with 0.1 N HCl to estimate pheopigments using a Perkin-Elmer fluorometer (model LS50B).
Biochemical composition of sediment organic matter.
Total sedimentary carbohydrates were analyzed according to the method of Gerchacov and Hatcher (15). Glucose solutions were used as a standard. Proteins were determined according to the method of Hartree (17) as modified by Rice (26) to compensate for phenol interference. Albumin solutions were used as a standard. For each analysis ca. 0.5 g of sediment was used. Lipids were extracted from dried sediment samples by direct elution with chloroform and methanol. Analyses were carried out using the methods of Bligh and Dyer (2) and Marsh and Weinstein (21). All analyses were carried out on ca. 0.5 g of sediment previously sonicated for 3 h in Milli-Q water to increase the extraction efficiency. Sediments treated in the muffle furnace (450°C, 2 h) were used as blanks for all analyses, and these analyses were performed in three to six replicates per sediment layer. Biopolymeric carbon (BPC) concentrations were calculated by converting protein, carbohydrate, and lipid concentrations into carbon equivalents assuming the conversion factors (4) of 0.49, 0.40, and 0.75 μg of C μg−1, respectively.
Viral and bacterial abundance.
Bacterial and viral counts were made from the same sample using 0.02-μm-pore-size filters. Each sample was treated with pyrophosphate (0.01 M) for 15 min (6, 20), which was efficient for both bacteria and viruses, and sonicated three times (Branson Sonifier 2200; 60 W for 1 min in an ice bath) to optimize extraction. The concentration of pyrophosphate was selected after testing different concentrations down to 0.001 M. Subsamples were diluted 100 to 500 times. Aliquots of the subsamples were stained with SYBR-1 (22) and filtered on Anodisc Al2O3 filters (0.02-μm pore size). The filters were analyzed by epifluorescence microscopy using a Zeiss Axioplan microscope equipped with a 50-W lamp. From 10 to 50 fields were viewed, and a minimum of 400 cells were counted for both viruses and bacteria. Detection limits for viral density were calculated on the basis of no cells encountered in more than 50 fields (equivalent to a density lower than 4 × 103 viruses g of sediment−1). Viruses were also analyzed by transmission electron microscopy (TEM) to gather information on their morphology and characteristics. Using the method of Maranger and Bird (20), ca. 3 ml was centrifuged at 3,000 × g for 30 min. A subaliquot of 1 ml was diluted 1- to 100-fold and centrifuged at 100,000 × g directly for 30 min onto a 400-mesh Formvar-coated Cu and stained with uranyl acetate. Counts were done using electron microscopy at magnifications of ×50,000 to ×80,000. Methodological aspects of these experiments will be discussed elsewhere (R. Danovaro, A. Dell'Anno, A. Trucco, M. Serresi, and S. Vanucci, manuscript in preparation).
RESULTS
Environmental parameters.
No clear differences were observed between sampling areas in terms of water content and porosity (Table (Table1).1). The water content of the superficial layer (0 to 0.3 cm) for the three investigated areas ranged from 32.7% (in the Ierapetra Basin) to 34.4% (in the Cretan Sea). Differences in average depth (4,235 versus 1,840 m, for the Ierapetra Trench and the Cretan Sea) did not influence sediment compactness. Porosity varied with water content, ranging from 0.56 in the Ierapetra Basin to 0.58 in the Cretan Sea.
Phytopigments and biochemical composition of sedimentary organic matter.
Chloroplastic pigment concentrations in the sediments are illustrated in Fig. Fig.1.1. The chlorophyll a concentration was, on average, 0.04 μg g−1 in all of the investigated areas. At all stations, total pheopigment concentrations were significantly higher than those for chlorophyll a. Organic matter biochemical composition is illustrated in Fig. Fig.2.2. Carbohydrates were the dominant biochemical class of organic compounds and, in the top 3 mm of the sediment, displayed concentrations more than double for the Ierapetra Trench with respect to the Cretan Sea (on average, 2,406 and 1,092 μg g−1, respectively). The second main biochemical class was represented by proteins that followed a gradient of decreasing productivity ranging from 479 to 760 μg g−1 (at the Ierapetra Trench and the Sporades Basin, respectively). Finally, lipids displayed the same trend reported for proteins with the highest values at the Sporades Basin (on average, 279 μg g−1) and the lowest values at the Ierapetra Trench (on average, 163 μg g−1). Soluble compounds generally accounted for a minor fraction of carbohydrates and lipids (5 to 20% [data not shown]) but represented the main protein fraction, accounting always for more than 80% of the total protein concentrations.
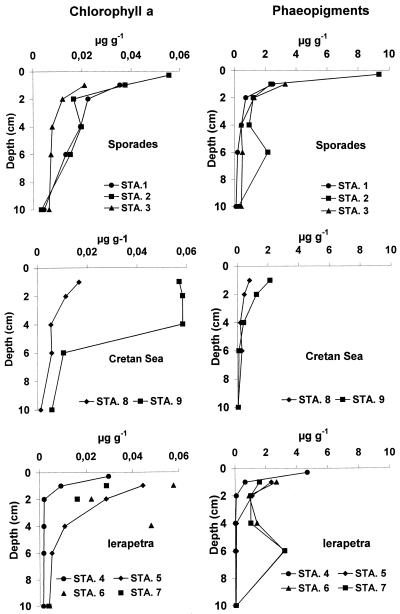
Vertical distribution of phytopigment concentrations determined fluorometrically at the three sampling areas. Shown are results for chlorophyll a and pheopigments at the Sporades, Ierapetra, and Cretan locations. Average values for each basin are indicated (n = 3 to 6). Data are expressed as micrograms per gram of sediment (dry weight).
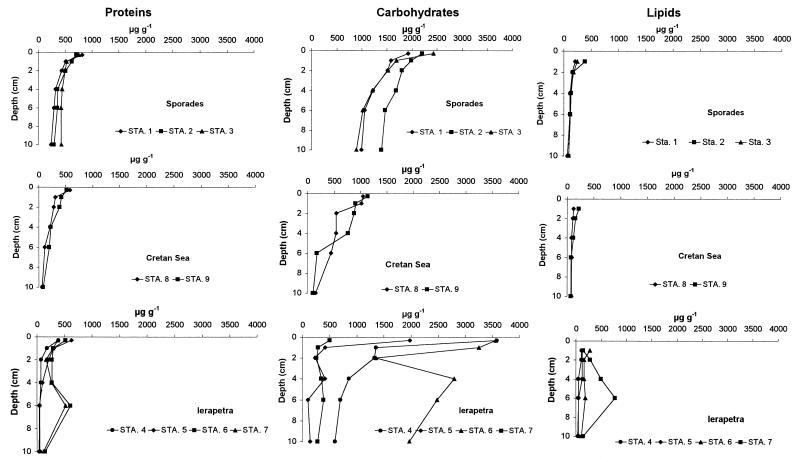
Vertical distribution of the main biochemical classes of organic compounds in the sediments of the deep Eastern Mediterranean. Shown are results for proteins, carbohydrates, and lipids. Average values for each basin are indicated (n = 3 to 6). Data are expressed as micrograms per gram of sediment (dry weight).
The top 3 mm of the sediment generally displayed concentrations ca. 10-fold higher than at the 10-cm depth, except for the richer Sporades Basin, where concentrations decreased only for ca. 50%. In the Ierapetra Trench, stations 6 and 7 displayed, according to phytopigment profiles, a subsurface peak (2- to 6-cm depth) in the concentration for all biochemical classes considered. BPC concentrations were lowest at the Cretan Sea and highest in the Sporades Basin, but the quality of the organic matter, as suggested by the total protein to carbohydrate ratio, was higher in the Cretan Sea (on average, 0.5), followed by the Sporades Basin (on average, 0.35) and the Ierapetra Trench (on average, 0.2).
Benthic bacteria and viruses.
Data on bacterial and viral densities in the sediments of the Eastern Mediterranean are reported in Fig. Fig.3.3. Bacterial densities at the Sporades Basin were ca. twice those at the Ierapetra Trench, on average (11.0 × 108 versus 4.9 × 108 cells g of sediment−1, respectively), and almost threefold higher than in the Cretan Sea (on average, 4.0 × 108 cells g−1). Similar patterns were observed for the bacterial biomass (data not shown). The viral densities displayed very similar values in the Sporades Basin and the Cretan Sea (23.75 × 108 and 20.2 × 108 cells g of sediment−1, respectively), with densities about double that at the Ierapetra Trench (on average, 12.1 × 108 cells g−1). As a result, the virus-to-bacterium density ratio was highest in the Cretan Sea (on average, 5.3), with values approximately twice that in the Ierapetra Trench and the Sporades Basin (on average, 2.2 and 2.6, respectively). Vertical patterns indicated a clear decrease of both bacterial and viral densities with depth in the sediment, but the viral-abundance decrease was much sharper, leading to a strong decrease of the virus-to-bacterium ratio with increasing sediment depth. At one of the four Ierapetra Trench stations, the virus density under the 4-cm depth was below detection limits.
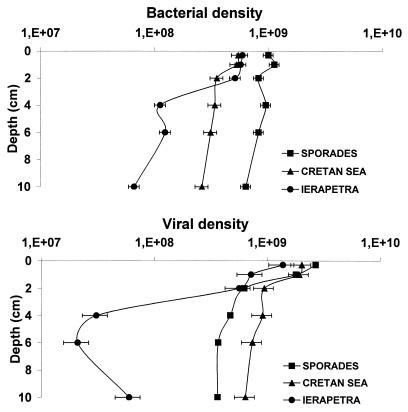
Vertical distribution of microbial parameters in the deep Eastern Mediterranean. Shown are the bacterial densities (cells per gram of sediment [dry weight]) and viral densities (viruses per gram of sediment [dry weight]). Average values for each basin are indicated. The data are expressed as viruses or bacteria (108) per gram of sediment (dry weight).
Statistical analysis.
A Spearman rank correlation analysis was carried out to test the relationships among bacteria, viruses, and environmental parameters. The bacterial density (log transformed) was significantly correlated with viral density (n = 9, r = 0.805, P < 0.01), soluble carbohydrates (r = 0.734, P < 0.01), and total (r = 0.786, P < 0.01) and soluble (r = 0.662, P < 0.05) proteins. Virus density values were significantly correlated with total protein concentrations (r = 0.679, P < 0.05). Differences among stations were tested by using one-way analysis of variance (ANOVA) at the 5% level, after testing of the homogeneity of variance.
DISCUSSION
Trophic conditions in deep-sea sediments of the Eastern Mediterranean.
It has been recently proposed that viral density and bacterial mortality due to viral infection increase with the degree of eutrophication (31). In this study we compared deep areas characterized by evident differences in trophic conditions. The three sites were different both in organic matter content (as indicated by changes in biopolymeric carbon concentrations) and in quality (as highlighted by changes in the relative significance of protein and carbohydrate pools). A clear gradient of increasing trophic conditions was observed along the axis of the North to the South Aegean (i.e., moving from the bathyal Sporades Basin to the abyssal Ierapetra Trench). This gradient reflected differences in primary productivity and particle fluxes previously reported for the North and South Aegean (9). Organic matter concentrations (expressed as protein, carbohydrate, and lipid content of the sediment) were generally low compared with equally deep sediments in temperate coastal areas and similar to those reported in productive environments (5). A specific feature of sedimentary organic matter composition in oligotrophic deep-sea environments is the dominance of carbohydrates over other biochemical components, resulting in a very low protein-to-carbohydrate ratio (5).
Phytopigment concentrations, assumed to represent a tracer of the primary organic input to the sea floor, confirmed the gradient described for vertical particle fluxes (8, 9). The pheopigment content in the top 1-cm portion of the sediment of the Sporades Basin was significantly higher than at the Ierapetra Trench or in the Cretan Sea (ANOVA, P < 0.05). Previous studies have shown that viruses might be adsorbed to sinking particles and thus transported to the sea floor (25). This suggests that deep-sea sediments receiving higher particle inputs from the water column, as in the Sporades Basin, might receive larger virus inputs.
Virus and bacterium abundance and distribution in deep-sea sediments.
It is generally recognized that bacterial distribution is largely dependent upon the amount of utilizable organic matter in the sediments, which in turn is largely controlled by the sedimentation and degradation rates in the water column (11). The data reported here are consistent with this rule, and the bacterial density is significantly correlated with the amounts of labile organic compounds (5, 7, 9, 10). However, our data from deep-sea environments contrast with most studies on bacterial distribution in marine sediments, which have reported quite-conservative bacterial densities in marine sediments, when densities are expressed with respect to fluid volume (data not shown) instead of to dry sediment mass (27). Bacterial densities were significantly higher at the Sporades Basin than in other sampling areas (ANOVA, P < 0.01) and were correlated to organic-matter content and viral density (Fig. (Fig.4).4). Similar relationships have been observed in lake sediments (20) and in pelagic systems (31).
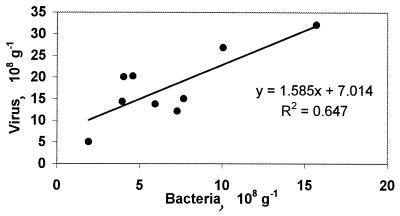
Relationship between bacterial and viral densities in deep-sea sediments of the Eastern Mediterranean.
Viral densities were generally high and rather constant (1 × 109 to 2 × 109 viruses g−1), with values close to those previously reported for sediments. Paul et al. (23) and Maranger and Bird (20) pointed out that these values are up to 3 orders of magnitude higher than those reported for the overlying water column (16). In the only study available on deep-ocean waters, the virus density (range, 0.6 × 105 to 38 × 105 viruses ml−1) decreased about 20-fold from the surface to a 5,000-m depth.
Maranger and Bird (20) asserted that if sediment viruses are as active as those in the water column, benthic viruses might play an important role in sediment biogeochemistry. However, it should be noted that despite these high virus densities, their relative significance compared to bacteria (i.e., the virus-to-bacterium ratio) is much lower than in pelagic environments. Previous water column studies reported a virus-to-bacterium ratio ranging from 10 to 100 (with virus abundance being, on average, 5 to 25 times the bacterium abundance [14]), whereas our results from deep-sea sediments reported a virus-to-bacterium ratio ranging from 2 to 5, with a modal value of 2.6. Similarly low virus-to-bacterium ratios have been reported only from lake sediments (20) and from deep-sea environments (16, 22). In a study on viruses associated with sinking particles, despite high bacterial densities in the deepest sediment trap (400-m depth), no viruses were found there (25). Similarly, no virus infection was observed in the pore water of the sediments collected from sediment pore waters from the Bering Sea (30).
The nonspecific adsorption to settling particles and subsequent sedimentation has been suggested as one of the major mechanisms of virus removal from the water column (31). In agreement with our expectations, the highest virus densities were observed in the Sporades Basin (ANOVA, P < 0.05 [in the top 1-cm portion of the sediment]), indicating that a gradient of particle fluxes and trophic conditions (including a higher protein content) is reflected by the virus density. However, at the moment no techniques exist to distinguish between viruses that are locally produced in the sediments and viruses that have reached the sediment attached to settling particles. Moreover, the ratio of virus-to-bacterium abundance decreased vertically with depth in the sediment core at all stations, suggesting that viruses are, at least partially, supplied by particle fluxes.
The lack of much higher virus/bacterium ratios in deep-sea sediments could be the result of less-hospitable physical conditions that limit the ability of viruses to find new hosts (20). The role of pressure in controlling allochthonous viral populations has not been evaluated yet, but the lower deep-sea temperature, decreasing the bacterial growth rate or increasing the percentage of quiescent bacteria, may not allow phage replication, though it has been recently demonstrated that some quiescent bacteria allow infection and phage replication (28). In addition, the presence of surface organic film or clay particles binding bacteria with ionic strength might provide some protection from viruses. Finally, oxygen availability is likely to play a minor role in virus survival in the sediment since the studied deep-sea sediments are oxidized down to a 10-cm depth (data not shown).
Recent plaque technique results indicate that the structure of the phage populations in the sediment is different from the one reported in the water column (23). Ackerman (1) reported that 96% of the phages are tailed and only <4% are filamentous or pleomorphic. Preliminary TEM analyses carried out in this study revealed a higher morphological diversity (data not shown). Future studies should also take into account benthic viral morphodiversity and activity so as to better assess the ecological role of phages and their potential in controlling benthic bacterial dynamics.
ACKNOWLEDGMENTS
We are particularly indebted to two anonymous reviewers for suggestions and comments that resulted in a substantial improvement of the manuscript, to A. Dell'Anno (University of Ancona) for suggestions for the biochemical analyses, and to the crew of the R/V Meteor and to A. Tselepides and N. Papadopoulou (IMBC, Athens, Greece) for providing sediment samples. M. Armeni (University of Ancona) kindly collaborated on the TEM analyses.
This work has been carried out as part of the Programme MATER (MAS3-CT96-0051), with EU funding.
REFERENCES
Articles from Applied and Environmental Microbiology are provided here courtesy of American Society for Microbiology (ASM)
Full text links
Read article at publisher's site: https://doi.org/10.1128/aem.66.5.1857-1861.2000
Read article for free, from open access legal sources, via Unpaywall:
https://europepmc.org/articles/pmc101423?pdf=render
Citations & impact
Impact metrics
Citations of article over time
Smart citations by scite.ai
Explore citation contexts and check if this article has been
supported or disputed.
https://scite.ai/reports/10.1128/aem.66.5.1857-1861.2000
Article citations
Phage-inclusive profiling of human gut microbiomes with Phanta.
Nat Biotechnol, 42(4):651-662, 25 May 2023
Cited by: 14 articles | PMID: 37231259
Covariance of Marine Nucleocytoplasmic Large DNA Viruses with Eukaryotic Plankton Communities in the Sub-Arctic Kongsfjorden Ecosystem: A Metagenomic Analysis of Marine Microbial Ecosystems.
Microorganisms, 11(1):169, 09 Jan 2023
Cited by: 2 articles | PMID: 36677461 | PMCID: PMC9862967
Distribution Characteristics of Soil Viruses Under Different Precipitation Gradients on the Qinghai-Tibet Plateau.
Front Microbiol, 13:848305, 07 Apr 2022
Cited by: 4 articles | PMID: 35464951 | PMCID: PMC9022101
Isolation and Characterization of the First Temperate Virus Infecting Psychrobacillus from Marine Sediments.
Viruses, 14(1):108, 08 Jan 2022
Cited by: 5 articles | PMID: 35062312 | PMCID: PMC8779076
Novel Viral Communities Potentially Assisting in Carbon, Nitrogen, and Sulfur Metabolism in the Upper Slope Sediments of Mariana Trench.
mSystems, 7(1):e0135821, 04 Jan 2022
Cited by: 15 articles | PMID: 35089086 | PMCID: PMC8725595
Go to all (56) article citations
Similar Articles
To arrive at the top five similar articles we use a word-weighted algorithm to compare words from the Title and Abstract of each citation.
Higher abundance of bacteria than of viruses in deep Mediterranean sediments.
Appl Environ Microbiol, 68(3):1468-1472, 01 Mar 2002
Cited by: 55 articles | PMID: 11872504 | PMCID: PMC123732
Viruses, prokaryotes and DNA in the sediments of a deep-hypersaline anoxic basin (DHAB) of the Mediterranean Sea.
Environ Microbiol, 7(4):586-592, 01 Apr 2005
Cited by: 36 articles | PMID: 15816935
Bacterial diversity in deep Mediterranean sediments: relationship with the active bacterial fraction and substrate availability.
Environ Microbiol, 6(7):745-753, 01 Jul 2004
Cited by: 30 articles | PMID: 15186353
A Brief Review of Bioactive Metabolites Derived from Deep-Sea Fungi.
Mar Drugs, 13(8):4594-4616, 23 Jul 2015
Cited by: 52 articles | PMID: 26213949 | PMCID: PMC4556995
Review Free full text in Europe PMC