Abstract
Free full text
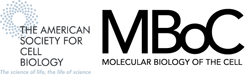
Syntaxin 7 and VAMP-7 are Soluble N-Ethylmaleimide–sensitive Factor Attachment Protein Receptors Required for Late Endosome–Lysosome and Homotypic Lysosome Fusion in Alveolar Macrophages
Abstract
Endocytosis in alveolar macrophages can be reversibly inhibited, permitting the isolation of endocytic vesicles at defined stages of maturation. Using an in vitro fusion assay, we determined that each isolated endosome population was capable of homotypic fusion. All vesicle populations were also capable of heterotypic fusion in a temporally specific manner; early endosomes, isolated 4 min after internalization, could fuse with endosomes isolated 8 min after internalization but not with 12-min endosomes or lysosomes. Lysosomes fuse with 12-min endosomes but not with earlier endosomes. Using homogenous populations of endosomes, we have identified Syntaxin 7 as a soluble N-ethylmaleimide-sensitive factor attachment protein receptor (SNARE) required for late endosome–lysosome and homotypic lysosome fusion in vitro. A bacterially expressed human Syntaxin 7 lacking the transmembrane domain inhibited homotypic late endosome and lysosome fusion as well as heterotypic late endosome–lysosome fusion. Affinity-purified antibodies directed against Syntaxin 7 also inhibited lysosome fusion in vitro but had no affect on homotypic early endosome fusion. Previous work suggested that human VAMP-7 (vesicle-associated membrane protein-7) was a SNARE required for late endosome–lysosome fusion. A bacterially expressed human VAMP-7 lacking the transmembrane domain inhibited both late endosome–lysosome fusion and homotypic lysosome fusion in vitro. These studies indicate that: 1) fusion along the endocytic pathway is a highly regulated process, and 2) two SNARE molecules, Syntaxin 7 and human VAMP-7, are involved in fusion of vesicles in the late endocytic pathway in alveolar macrophages.
INTRODUCTION
The endocytic apparatus consists of an amorphous mixture of tubules and vesicles. These structures continuously undergo rounds of fusion and fission with both newly internalized and preexisting vesicles. The steady-state size and number of endocytic vesicles are maintained, indicating tight regulation of the rates of vesicle fusion and fission. Although fusion and fission are dynamic processes, membranes retain their biochemical identity, indicating a selectivity of fusion events. The specificity of vesicle fusion is determined primarily by the ability of organelles/vesicles to recognize each other through molecules referred to as soluble N-ethylmaleimide–sensitive factor (NSF) attachment protein receptors (SNAREs). SNAREs on one class of vesicles are hypothesized to recognize and form complexes with their cognate receptors, SNAREs on other vesicles (Sollner et al., 1993, 1994; Ferro-Novick and Jahn, 1994; Rothman and Warren, 1994). SNAREs responsible for specific organelle fusion events have been identified for both the endocytic and secretory pathways. Two major approaches have been used to identify SNAREs: genetic analysis of yeast mutants defective in vesicular trafficking (Fischer von Mollard and Stevens, 1998; Abeliovich et al., 1998; Fischer von Mollard and Stevens, 1999; Gerst, 1999; Pelham, 1999) and biochemical identification of SNAREs in mammalian systems through immunoidentification or the use of inhibitors (Coorssen et al., 1998; Hackam et al., 1998; Huang et al., 1998; Carr et al., 1999; Ungermann et al., 1999).
The endocytic apparatus in cells is asynchronous, making biochemical purification of specific endocytic vesicles difficult. We previously developed a procedure that synchronizes endocytosis in alveolar macrophages and permits the isolation of endocytic vesicles at different stages of maturation/development (Ward et al., 1995). These vesicles are capable of fusion in vitro, and this fusion shows specificity: early endosomes do not fuse with lysosomes (Ward et al., 1997). Using this system, we demonstrate specific homotypic and heterotypic fusion along the endocytic pathway. The addition of recombinant SNAREs or vacuolar protein sorting (VPS) proteins as competitive inhibitors of fusion has identified Syntaxin 7 and vesicle-associated membrane protein-7 (VAMP-7) as SNAREs required for homotypic and heterotypic fusion events in the late portion of the endocytic pathway.
MATERIALS AND METHODS
Cells
Rabbit alveolar macrophages were obtained by bronchial lavage (Myrvik, 1961) and maintained as described previously (Kaplan, 1980)
Genetic Constructs
Recombinant human Syntaxin 7 protein, lacking the predicted transmembrane domain (amino acids 1–237; GenBank accession number U77942), was expressed as a GST fusion protein in bacteria. To generate this construct, a cDNA clone encoding human Syntaxin 7 was amplified by PCR with the use of oligonucleotide primers (5′-CGG AAT TCC CAT GTC TTA CAC TCC AGG AGT-3′ and 5′-ACG CGT CGA CGC ACA GGG TTT TTC TGG ATT-3′ containing the restriction enzyme sites EcoRI and SalI, respectively) and subcloned into the pGEX-KG vector. Transformed bacteria were induced with isopropyl β-d-galactopyranoside, and recombinant GST/Syntaxin 7 was purified by glutathione agarose chromatography according to GST fusion protein protocols from Pierce Chemical (Rockford, IL). Other recombinant fusion proteins were generated by similar strategies. VAMP-7 cDNA (D'Esposito et al., 1996) was amplified from clone 125189 (American Type Culture Collection, Rockville, MD) and subcloned into pGEX-KG with the use of NcoI and HindIII restriction enzyme sites. Rat (r)-vps33a (U35244), r-vps33b (U35245), and human (h)-vps45 (NM-007258) cDNAs (Pevsner et al., 1996) were subcloned into pGEX-KG vector with the use of the restriction enzyme pairs EcoRI and SalI, EcoRI and NcoI, and XbaI and XhoI, respectively. h-vps28 (accession number AF182844; P. Hunt and J. Pevsner, unpublished data) was subcloned into pGST-T2 vector (Pharmacia, Arlington Heights, IL) with the use of SalI and NotI restriction enzyme sites. All constructs were sequenced on both strands.
Synchronization of Endocytosis and Isolation of Endosomes and Lysosomes
Endocytosis was synchronized with the use of the hypoosmotic K+ (hypo-K+) technique described previously (Ward et al., 1995). Briefly, alveolar macrophages were incubated in hypo-K+ buffers for 30 min at 37°C. Cells were then placed in isoosmotic Na+ buffers containing either biotinylated HRP (b-HRP) or avidin for 4 min. Cells were placed at 0°C and washed extensively with 5 mM EDTA containing buffer to remove surface-bound ligand. The cells were then placed at 37°C in hypo-K+ buffers containing 2.0 mg/ml mannan. (HRP is a mannose-containing glycoprotein and will bind to mannose-terminal receptors in a calcium-dependent manner. EDTA and mannan reduce ligand binding, preventing uptake of recycled ligand.) At specified times, cells were homogenized and endosomes or lysosomes were isolated by Percoll gradients as described previously (Ward et al., 1997).
Fusion Assay
Fusion was assayed by measuring the formation of avidin–b-HRP complexes as described previously (Ward et al., 1997). Recombinant SNAREs or VPS proteins were added to the fusion reactions with either NSF-treated vesicles or cytosol present during the fusion reaction.
Materials
b-HRP, avidin D, and antiavidin were obtained from Vector Laboratories (Burlingame, CA). Glutathione–agarose was obtained from Pierce. HRP-conjugated goat anti-rabbit antibodies were obtained from Jackson Immunoresearch (West Grove, PA). Recombinant NSF was obtained from Dr. S.W. Whiteheart (University of Kentucky, Lexington). All other reagents used were obtained from Sigma Chemical (St. Louis, MO).
RESULTS
Endocytosis and intracellular vesicle movement in alveolar macrophages can be synchronized by exposing cells to a hypoosmotic solution containing 70 mM K+ as the major cation. Incubation of cells in hypoosmotic solutions results in water influx, leading to the opening of cell surface K+ channels. The concentration gradient favors K+ influx because the intracellular K+ concentration is 60 mM and the extracellular K+ concentration is 70 mM (Novak et al., 1988). Cation and water influx results in an increase in cell volume, which inhibits endocytosis without affecting the movement or behavior of previously formed endocytic vesicles. Recycling to the cell surface and movement to lysosomes continue at normal rates (Ward et al., 1995). A consequence of the inhibition of endocytosis and continued vesicle movement is the depletion of the early endosomal apparatus. Endocytosis and reformation of the endocytic apparatus occur when cells are placed back into isoosmotic Na+ medium. Reexposure of cells to hypo-K+ incubation again blocks endocytosis, resulting in a “square wave” of endocytic activity. Isolation of endosomes at specific times after the release of the hypo-K+ block yields a cohort of endosomes with similar biochemical properties.
Fusion between different cohorts of endosomes, or between endosomes and lysosomes, can be assayed in vitro. Donor endosomes are isolated from cells exposed to b-HRP, whereas recipient vesicles, endosomes or lysosomes, are isolated from cells exposed to avidin (Ward et al., 1997). Fusion is identified through the formation of an avidin–b-HRP complex. Isolation of donor endosomes at specific times after release of the hypo-K+ block permits analysis of the fusion capabilities of differently aged endosome populations. All endosome populations have a similar density on Percoll gradients, which is quite different from that of lysosomes (Ward et al., 1990). Endosome populations obtained from Percoll gradients were tested for fusion in vitro. Early endosomes, formed within 4 min of internalization (E4), were capable of homotypic fusion but could not fuse with lysosomes (Figure (Figure1A).1A). Endosomes isolated 8 min after release of the hypo-K+ block (E8) were also unable to fuse with lysosomes but were capable of homotypic fusion (Figure (Figure1B).1B). There was a gradient in heterotypic fusion capacity, in that E4 had a greater ability to fuse with E8 than with 12-min endosomes (E12). Endosomes internalized for less than 12 min were unable to fuse with lysosomes. Later endosomes (E12) gained the ability to fuse with lysosomes (Figure (Figure1C).1C). These endosomes fused poorly with early endosomes (E4). Lysosomes were also capable of homotypic fusion (Figure (Figure1D),1D), as demonstrated previously (Ward et al., 1997). These results indicate that each endosome population is capable of homotypic fusion and that endosomes show a change in the selectivity of heterotypic vesicle fusion after 8 min of maturation.

Endosome and lysosome (Lyso) fusion in vitro. Isolated vesicle populations were combined in the in vitro fusion system as described in MATERIALS AND METHODS. Cells were incubated in hypo-K+ buffer for 30 min to stop endocytosis. Cells were then incubated in iso-Na+ buffers containing either b-HRP or avidin for 4 min, washed, and chased in hypo-K+ buffer for additional times. E4 (A) represents endosomes isolated from cells that had internalized avidin or b-HRP for 4 min. E8 (B) represents endosomes isolated from cells that had internalized ligand for 4 min followed by 4 min of chase time. E12 (C) represents endosomes isolated from cells that had internalized ligand for 4 min followed by 8 min of chase time. Lysosomes (D) were isolated from cells allowed to internalize ligand for 30 min followed by a 60-min chase period. Lysosome fusion with E4, E8, and E12 was measured (A–C), as was lysosome–lysosome fusion (D). The percentage of in vitro fusion was calculated with the use of the maximum avidin–b-HRP complex formation in the absence of biotinylated insulin as the denominator.
Homotypic Lysosome Fusion Is Affected by Syntaxin 7
A “candidate” protein approach was adapted to define the SNAREs responsible for selective vesicle fusion. We focused first on homotypic lysosome fusion, because we had previously defined some of the biochemical parameters for this event (Ward et al., 1997). Using information from studies on mammals and yeast, we identified and cloned specific mammalian SNAREs and VPS homologues involved in vesicle fusion and protein trafficking, including h-Syntaxin 4, 5, and 7, h-VAMP-7, h-VPS28 and -45, and rVPS33a and -33b. These proteins were expressed in bacteria as GST fusion proteins, and those containing a transmembrane domain were expressed without their transmembrane domain. Proteins were purified and examined for their ability to inhibit homotypic endocytic fusion in the in vitro assay. Syntaxin 4 inhibited early endosome fusion but had no effect on lysosome fusion. Alternatively, Syntaxin 7 affected lysosome fusion but not early endosome fusion (Table (Table1).1). The addition of recombinant h-VPS28, h-VPS45, r-VPS33a, or r-VPS33b did not affect endosome or lysosome fusion (our unpublished results).
Table 1
Effect of recombinant SNARE proteins on in vitro fusion
SNARE
| ||||
---|---|---|---|---|
h-Syntaxin 4 | h-Syntaxin 5 | h-Syntaxin 7 | h-VAMP-7 | |
Lysosome–lysosome fusion | 101.1![]() ![]() | 93.6![]() ![]() | 32.1![]() ![]() | 69.8![]() ![]() |
E4–E4 fusion | 68.3![]() ![]() | 98.7![]() ![]() | 99.3![]() ![]() | 96.7![]() ![]() |
In vitro lysosome–lysosome or endosome–endosome fusion was performed as described in MATERIALS AND METHODS. Recombinant SNAREs were added to the fusion reaction at 20 μg/ml before the fusion incubation. The data are expressed as the percentage of fusion observed, where 100% is the maximum amount of fusion observed for each vesicle population. The data represent the average of three to five different experiments.
Syntaxin 7 was a likely candidate because one of its homologues in yeast, Vam3p, was shown to mediate homotypic vacuole fusion (Sato et al., 1998). The bacterially expressed Syntaxin 7 lacking the transmembrane domain inhibited lysosomal fusion in a dose-dependent manner (Figure (Figure2).2). GST or thrombin (used to cleave the GST) had no inhibitory affect in the fusion assay (our unpublished results). GST-Syntaxin 7 inhibited fusion to the same extent as Syntaxin 7 lacking GST. The addition of an affinity-purified rabbit polyclonal anti-Syntaxin 7 antibody to the fusion assay resulted in inhibition of lysosome fusion in a concentration-dependent manner (Figure (Figure3).3). Early endosome fusion was unaffected by the addition of anti-Syntaxin 7 (our unpublished results). No inhibitory activity in lysosome–lysosome fusion was seen with the use of either irrelevant immunoglobulin G (our unpublished results) or preimmune sera. The addition of recombinant Syntaxin 7 to the specific antiserum before incubation in the fusion assay partially abrogated the antibody-inhibitory activity (our unpublished results).

Syntaxin 7 concentration-dependent inhibition of homotypic vesicle fusion in vitro. Purified endosomes (E4, E8, and E12) and lysosomes (Lyso) containing avidin or b-HRP were incubated in the in vitro fusion system. Specified amounts (0–20 μg/ml) of purified recombinant Syntaxin 7, minus the transmembrane domain, were added before incubation at 37°C. The data are expressed as the percentage of fusion measured, where 100% represents the maximum fusion observed.

Anti-Syntaxin 7 inhibition of homotypic lysosome (Lyso) fusion in vitro. Purified lysosomes containing avidin or b-HRP were incubated in the in vitro fusion system containing affinity-purified rabbit polyclonal anti-Syntaxin 7 antibody () or preimmune serum (
). The data are expressed as the percentage of fusion, where 100% represents the maximum fusion observed.
Syntaxin 7 Is Responsible for Late Endosome Vesicle Fusion Events but Not for Early Endosome Fusion Events
Recently, Syntaxin 7 was identified as a component of early endosomes in cultured fibroblasts (Prekeris et al., 1999). In our in vitro system, however, we found that h-Syntaxin 7 affected only the fusion of the late components of the endocytic pathway. Although affinity-purified anti-Syntaxin 7 inhibited the fusion of lysosomes or late endosomes, the antibody was unable to detect a rabbit Syntaxin 7 by Western blot analysis, immunoprecipitation followed by silver staining, or immunofluorescence. The antibody could specifically detect either a human or murine protein of 36–38 kDa by either Western analysis or immunoprecipitation. If Syntaxin 7 had a cognate partner on lysosomes and not early endosomes, the protein should form a complex with lysosomes. To test this hypothesis, GST-Syntaxin 7 was added to a fusion reaction containing either lysosomes or early endosomes. As observed previously (Table (Table1),1), Syntaxin 7 inhibited homotypic lysosome but not homotypic early endosome fusion. The remainder of the endosome or lysosome fusion reaction was centrifuged to pellet vesicles. The fusion reaction supernatant was removed, vesicles were washed and solubilized with detergent, and the extract was incubated with GST-agarose beads. The beads were then eluted with glutathione, and the eluate was examined by Western blot analysis with the use of the anti-Syntaxin 7 antibody. Even though equal amounts of vesicles were used in the assay, there was no evidence of GST-Syntaxin 7 bound to early endosomes, whereas there was clear evidence that GST-Syntaxin 7 was bound to lysosomes (Figure (Figure4).4).

Western analysis of GST-Syntaxin 7 binding to endosomes and lysosomes in vitro. Purified lysosomes or endosomes (0.5 mg/ml) were incubated in the in vitro fusion system in the presence of 20 μg/ml GST-Syntaxin 7. After incubation at 37°C, fusion was assessed as described in MATERIALS AND METHODS. Samples of endosomes or lysosomes from the fusion assay were pelleted and washed with fusion buffer. Vesicle pellets were solubilized by the addition of 0.05% Triton X-100 containing 10 mM Tris-HCl, pH 7.5, and 50 mM NaCl at 0°C for 30 min followed by centrifugation at 40,000 × g for 30 min. Supernatants were incubated with glutathione–agarose beads at 0°C for 2 h or overnight, beads were pelleted and washed, and GST protein was eluted with 20 mM glutathione according to the Pierce protocol. Eluted samples were run on 4–20% SDS-PAGE, and Western analysis was performed with the use of affinity-purified rabbit anti-Syntaxin 7 (1:1000) as the primary antibody followed by HRP-conjugated goat anti-rabbit secondary antibody (1:10,000). A represents samples from lysosomes and B represents endosomal samples. Lanes A represent purified GST-Syntaxin 7 eluted from glutathione–agarose. Lanes B represent the supernatant from pelleted fusion reactions. Lanes C represent lysates after incubation with glutathione–agarose. Lanes D represent a glutathione–agarose bead wash after lysate incubation. Lanes E represent glutathione eluates from the lysosome or endosome glutathione–agarose beads. The arrow represents GST-Syntaxin 7 eluted from only purified lysosomes.
VAMP-7 Specifically Inhibits Late Fusion Events
A recent report demonstrated that antibodies against a human homologue of VAMP-7, when added to semipermeabilized cells, inhibited the transfer of internalized EGF to lysosomes (Advani et al., 1999). The authors concluded that human VAMP-7 was involved in late endosome–lysosome trafficking. We have confirmed this conclusion with the use of our in vitro assay. The addition of recombinant h-VAMP-7 inhibited the fusion of E12 vesicles with lysosomes and homotypic lysosome fusion in a dose-dependent manner (Figure (Figure5).5). The fact that higher concentrations of h-VAMP-7 are required for inhibitory activity, compared with Syntaxin 7, may reflect the state of the bacterially expressed proteins rather than an intrinsic difference between the proteins. h-VAMP-7 did not inhibit homotypic fusion of early endosomes (E4 and E8), indicating that its effect on late endosome–lysosome fusion is quite specific.
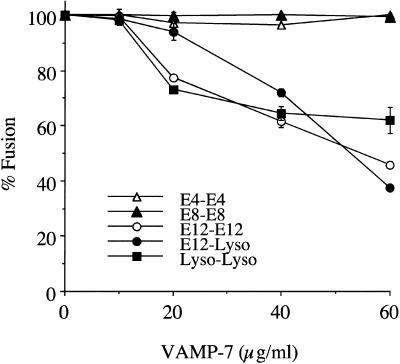
Addition of VAMP-7 to in vitro homotypic/heterotypic endosome and lysosome (Lyso) fusion. Specified amounts of recombinant VAMP-7 (minus the transmembrane domain) were added to the in vitro fusion reaction of E4–E4, E8–E8, E12–E12, Lyso–Lyso (all homotypic), and E12–Lyso (heterotypic). The data are expressed as the percentage of fusion, with 100% fusion representing the maximum fusion observed for each vesicle population.
DISCUSSION
We developed an in vitro system to identify molecules required for endocytic vesicle fusion. We focused our efforts on alveolar macrophages, which are professional phagocytes, because we previously demonstrated the ability to “synchronize” the endocytic apparatus (Ward et al., 1995). The ability to selectively regulate endocytosis results in the synchronization of endosome development/maturation, permitting the isolation of “reagent-grade” endosomes with differing biochemical properties. Although each endosomal age cohort is capable of homotypic fusion, they are restricted in their ability to undergo heterotypic fusion with either older or younger endosomes. A 4-min endosome can fuse with a 4- or 8-min endosome but shows a markedly diminished ability to fuse with either 12-min endosomes or lysosomes. Conversely, a 12-min endosome, which is capable of fusing with lysosomes, has a markedly reduced ability to fuse with endosomes earlier than 8 min. These results demonstrate that a change in endosome fusion properties occurs between 8 and 12 min after internalization. It is clear that this change in fusion activity must reflect some corresponding biochemical change in the molecules that either regulate fusion or specify vesicle interactions.
The genetic information encoding proteins required for many cellular processes is conserved in yeast and human, leading us to search for mammalian homologues of yeast proteins required for fusion events. Vam3p was initially identified as a vacuolar SNARE through genetic studies that demonstrated its role in vacuolar protein sorting (Darsow et al., 1997; Gotte and Gallwitz, 1997; Wada et al., 1997). Vam3p was also found to be required for vacuolar inheritance, which results from homotypic fusion of newly formed vacuolar vesicles present in budding cells (Ungermann et al., 1999). Pep12p was identified as being required for transport to the prevacuole (Becherer et al., 1996; Burd et al., 1997). Genetic and biochemical studies have demonstrated that in addition to Vam3p, Vam7p and the vacuolar SNAREs Nyv1p, Vti1p, and Ykt6p are implicated in homotypic vacuole fusion, perhaps as a fusion complex (Ungermann et al., 1999). Database searches identified Syntaxin 7 as a homologue of Vam3p and Pep12p (Wang et al., 1997). These studies demonstrate that Syntaxin 7 is a SNARE involved in late vesicle fusion in the endocytic pathway of alveolar macrophages.
Our studies demonstrating that Syntaxin 7 is a lysosomal SNARE are based on the ability of recombinant Syntaxin 7 to inhibit homotypic lysosome fusion in a dose-dependent manner. Syntaxin 7 was one of only two SNAREs or VPS proteins we tested that showed inhibitory activity in the late endocytic pathway. An affinity-purified antibody directed against recombinant Syntaxin 7 also inhibited homotypic fusion, and this inhibition could be partially prevented by previous addition of the Syntaxin 7 protein. We suspect that the recombinant protein only partially prevented inhibition of fusion because the protein, albeit at higher levels, could itself prevent fusion. Evidence for specificity is demonstrated by the facts that nonimmune or preimmune serum did not inhibit fusion and affinity-purified antibody affected the fusion of lysosomes but not early endosomes. Although the antibody inhibited lysosome fusion, it was unable to detect a rabbit protein by either Western analysis or immunoprecipitation. However, the antibody could detect an appropriately sized antigen in human cells, and this detection was ablated by the addition of recombinant GST-Syntaxin 7. A physical interaction between Syntaxin 7 and cognate molecules was shown by the binding of recombinant GST-Syntaxin 7 to lysosomes but not early endosomes.
Further evidence that our assay faithfully identifies vesicle-unique SNAREs is demonstrated by the specificity of Syntaxin 7. Syntaxin 7 inhibits lysosomal/late endosomal fusion events but not early endosome fusion events. Advani et al. (1999) demonstrated that h-VAMP-7 was required for late endosome–lysosome fusion. We have extended those studies to show that VAMP-7 is also involved in homotypic lysosome fusion but not early endosome fusion. Thus, two vesicle-specific SNAREs were identified by our assay. Are VAMP-7 and Syntaxin 7 cognate SNAREs? Studies are currently under way to address this question.
There is a discrepancy among the published studies on the location of Syntaxin 7. Two groups suggested that Syntaxin 7 was associated with early endosomes (Wong et al., 1998; Prekeris et al., 1999), whereas a third group suggested that Syntaxin 7 was localized to late endocytic compartments (Nakamura et al., 2000). Each study used different cell types and different methods for organelle identification. In the study by Wong et al. (1998), early endosomes in A431 cells were defined by the addition of a mAb to surface transferrin receptors, which were then internalized and subsequently localized by indirect immunofluorescence. The secondary antibody was directed against the anti-transferrin receptor antibody. Because the antibody is multivalent, the intracellular distribution of transferrin receptors may not represent the native distribution and may not reflect early endosomes. Furthermore, the fluorescence observed reflects the localization of the antibody, not necessarily that of the receptor. The antibody may be localized to late endosomal compartments where it may be degraded, as suggested by older studies (Lesley et al., 1989). Prekeris et al. (1999) colocalized Syntaxin 7 and anti-transferrin receptor antibodies by both fluorescence and electron microscopy with the use of several different cell types. More recently, Nakamura et al. (2000), again with the use of fluorescence and electron microscopy, localized Syntaxin 7 in NIH 3T3 and NRK cells to Lamp 2–positive compartments, suggesting a late endosomal/lysosomal location. Our studies used freshly obtained alveolar macrophages, whereas the other studies used cultured cell types. Alveolar macrophages are highly endocytic, and the localization of Syntaxin 7 on lysosomes may represent some adaptation for high-efficiency endocytosis. It is possible that our results, which rely on a functional assay for SNARE identification, reflect SNARE promiscuity. Recent studies postulate that SNAREs can be promiscuous and bind to a spectrum of cognates (Fasshauer et al., 1999). Thus, our finding of inhibition of lysosome fusion by Syntaxin 7 may reflect an inherent lack of specificity in cognate receptors. We do not favor this explanation because the inhibitory effect of Syntaxin 7 is specific for a restricted population of endocytic vesicles. Finally, we think that the identification of Syntaxin 7 as a lysosomal SNARE is consistent with the strong biochemical and genetic data demonstrating that the yeast homologue Vam3p is a vacuolar SNARE involved in both vacuolar traffic and homotypic fusion (Becherer et al., 1996; Darsow et al., 1997; Peterson et al., 1999; Ungermann et al., 1999). Nakamura et al. (2000) demonstrated that expression of Syntaxin 7 in yeast complemented vam3 and pep12 mutants. This observation reinforces the idea that Syntaxin 7 functions in late endocytic compartments.
This study clearly shows a dramatic change in endosomal fusion specificity at a defined maturation stage, in that 8-min endosomes could not fuse with lysosomes but 12-min endosomes could. The converse was also true with respect to early endosome fusion. The ability to isolate enriched populations of endosomes with different fusion specificities will permit the determination of the biochemical basis for these changes in vesicle fusion properties.
ACKNOWLEDGMENTS
We express our appreciation to Drs. Jim P. Kushner and Richard Ajioka and the members of the Kaplan laboratory for critical reading of the manuscript. This work was supported by National Institutes of Health grants HL26922 to J.K. and NS36670 to J.P. D.M.W. was supported by National Institutes of Health Hematology Postdoctoral Training Grant T32DK07115.
Abbreviations used:
b-HRP | biotinylated HRP |
E4 | 4-min endosome |
E8 | 8-min endosome |
E12 | 12-min endosome |
hypo-K+ | hypoosmotic K+ |
NSF | N-ethylmaleimide–sensitive factor |
SNARE | soluble N-ethylmaleimide–sensitive factor attachment protein receptor |
VAMP | vesicle-associated membrane protein |
VPS | vacuolar protein sorting |
REFERENCES
- Abeliovich H, Grote E, Novick P, Ferro-Novick S. Tlg2p, a yeast syntaxin homolog that resides on the Golgi and endocytic structures. J Biol Chem. 1998;273:11719–11727. [Abstract] [Google Scholar]
- Advani RJ, Yang B, Prekeris R, Lee KC, Klumperman J, Scheller RH. VAMP-7 mediates vesicular transport from endosomes to lysosomes. J Cell Biol. 1999;146:765–776. [Europe PMC free article] [Abstract] [Google Scholar]
- Becherer KA, Rieder SE, Emr SD, Jones EW. Novel syntaxin homologue, Pep12p, required for the sorting of lumenal hydrolases to the lysosome-like vacuole in yeast. Mol Biol Cell. 1996;7:579–594. [Europe PMC free article] [Abstract] [Google Scholar]
- Burd CG, Peterson M, Cowles CR, Emr SD. A novel Sec18p/NSF-dependent complex required for Golgi-to-endosome transport in yeast. Mol Biol Cell. 1997;8:1089–1104. [Europe PMC free article] [Abstract] [Google Scholar]
- Carr CM, Grote E, Munson M, Hughson FM, Novick PJ. Sec1p binds to SNARE complexes and concentrates at sites of secretion. J Cell Biol. 1999;146:333–344. [Europe PMC free article] [Abstract] [Google Scholar]
- Coorssen JR, Blank PS, Tahara M, Zimmerberg J. Biochemical and functional studies of cortical vesicle fusion: the SNARE complex and Ca2+ sensitivity. J Cell Biol. 1998;143:1845–1857. [Europe PMC free article] [Abstract] [Google Scholar]
- Darsow T, Rieder SE, Emr SD. A multispecificity syntaxin homologue, Vam3p, essential for autophagic and biosynthetic protein transport to the vacuole. J Cell Biol. 1997;138:517–529. [Europe PMC free article] [Abstract] [Google Scholar]
- D'Esposito M, Ciccodicola A, Gianfrancesco F, Esposito T, Flagiello L, Mazzarella R, Schlessinger D, D'Urso M. A synaptobrevin-like gene in the Xq28 pseudoautosomal region undergoes X inactivation. Nat Genet. 1996;13:227–229. [Abstract] [Google Scholar]
- Fasshauer D, Antonin W, Margittai M, Pabst S, Jahn R. Mixed and non-cognate SNARE complexes: characterization of assembly and biophysical properties. J Biol Chem. 1999;274:15440–15446. [Abstract] [Google Scholar]
- Ferro-Novick S, Jahn R. Vesicle fusion from yeast to man. Nature. 1994;370:191–193. [Abstract] [Google Scholar]
- Fischer von Mollard G, Stevens TH. A human homolog can functionally replace the yeast vesicle-associated SNARE Vti1p in two vesicle transport pathways. J Biol Chem. 1998;273:2624–2630. [Abstract] [Google Scholar]
- Fischer von Mollard G, Stevens TH. The Saccharomyces cerevisiae v-SNARE Vti1p is required for multiple membrane transport pathways to the vacuole. Mol Biol Cell. 1999;10:1719–1732. [Europe PMC free article] [Abstract] [Google Scholar]
- Gerst JE. SNAREs and SNARE regulators in membrane fusion and exocytosis. Cell Mol Life Sci. 1999;55:707–734. [Europe PMC free article] [Abstract] [Google Scholar]
- Gotte M, Gallwitz D. High expression of the yeast syntaxin-related Vam3 protein suppresses the protein transport defects of a pep12 null mutant. FEBS Lett. 1997;411:48–52. [Abstract] [Google Scholar]
- Hackam DJ, Rotstein OD, Sjolin C, Schreiber AD, Trimble WS, Grinstein S. v-SNARE-dependent secretion is required for phagocytosis. Proc Natl Acad Sci USA. 1998;95:11691–11696. [Europe PMC free article] [Abstract] [Google Scholar]
- Huang X, Wheeler MB, Kang YH, Sheu L, Lukacs GL, Trimble WS, Gaisano HY. Truncated SNAP-25 (1–197), like botulinum neurotoxin A, can inhibit insulin secretion from HIT-T15 insulinoma cells. Mol Endocrinol. 1998;12:1060–1070. [Abstract] [Google Scholar]
- Kaplan J. Evidence for reutilization of surface receptors for alpha-macroglobulin protease complexes in rabbit alveolar macrophages. Cell. 1980;19:197–205. [Abstract] [Google Scholar]
- Lesley J, Schulte R, Woods J. Modulation of transferrin receptor expression and function by anti-transferrin receptor antibodies and antibody fragments. Exp Cell Res. 1989;182:215–233. [Abstract] [Google Scholar]
- Myrvik QN, Leake ES, Fariss B. Studies on alveolar macrophages from the normal rabbit: a technique to procure them in a high state of purity. J Immunol. 1961;86:133–136. [Abstract] [Google Scholar]
- Nakamura N, Yamamoto A, Wada Y, Futai M. Syntaxin 7 mediates endocytic trafficking to late endosomes. J Biol Chem. 2000;275:6523–6529. [Abstract] [Google Scholar]
- Novak JM, Ward DM, Buys SS, Kaplan J. Effect of hypo-osmotic incubation on membrane recycling. J Cell Physiol. 1988;137:235–242. [Abstract] [Google Scholar]
- Pelham HR. SNAREs and the secretory pathway: lessons from yeast. Exp Cell Res. 1999;247:1–8. [Abstract] [Google Scholar]
- Peterson MR, Burd CG, Emr SD. Vac1p coordinates Rab and phosphatidylinositol 3-kinase signaling in Vps45p-dependent vesicle docking/fusion at the endosome. Curr Biol. 1999;9:159–162. [Abstract] [Google Scholar]
- Pevsner J, Hsu SC, Hyde PS, Scheller RH. Mammalian homologues of yeast vacuolar protein sorting (vps) genes implicated in Golgi-to-lysosome trafficking. Gene. 1996;183:7–14. [Abstract] [Google Scholar]
- Prekeris R, Yang B, Oorschot V, Klumperman J, Scheller RH. Differential roles of syntaxin 7 and syntaxin 8 in endosomal trafficking. Mol Biol Cell. 1999;10:3891–3908. [Europe PMC free article] [Abstract] [Google Scholar]
- Rothman JE, Warren G. Implications of the SNARE hypothesis for intracellular membrane topology and dynamics. Curr Biol. 1994;4:220–233. [Abstract] [Google Scholar]
- Sato TK, Darsow T, Emr SD. Vam7p, a SNAP-25-like molecule, and Vam3p, a syntaxin homolog, function together in yeast vacuolar protein trafficking. Mol Cell Biol. 1998;18:5308–5319. [Europe PMC free article] [Abstract] [Google Scholar]
- Sollner C, Mentele R, Eckerskorn C, Fritz H, Sommerhoff CP. Isolation and characterization of hirustasin, an antistasin-type serine-proteinase inhibitor from the medical leech Hirudo medicinalis. Eur J Biochem. 1994;219:937–943. [Abstract] [Google Scholar]
- Sollner T, Bennett MK, Whiteheart SW, Scheller RH, Rothman JE. A protein assembly-disassembly pathway in vitro that may correspond to sequential steps of synaptic vesicle docking, activation, and fusion. Cell. 1993;75:409–418. [Abstract] [Google Scholar]
- Ungermann C, von Mollard GF, Jensen ON, Margolis N, Stevens TH, Wickner W. Three v-SNAREs and two t-SNAREs, present in a pentameric cis-SNARE complex on isolated vacuoles, are essential for homotypic fusion. J Cell Biol. 1999;145:1435–1442. [Europe PMC free article] [Abstract] [Google Scholar]
- Wada Y, Nakamura N, Ohsumi Y, Hirata A. Vam3p, a new member of syntaxin related protein, is required for vacuolar assembly in the yeast Saccharomyces cerevisiae. J Cell Sci. 1997;110:1299–1306. [Abstract] [Google Scholar]
- Wang H, Frelin L, Pevsner J. Human syntaxin 7: a Pep12p/Vps6p homologue implicated in vesicle trafficking to lysosomes. Gene. 1997;199:39–48. [Abstract] [Google Scholar]
- Ward DM, Hackenyos DP, Kaplan J. Fusion of sequentially internalized vesicles in alveolar macrophages. J Cell Biol. 1990;110:1013–1022. [Europe PMC free article] [Abstract] [Google Scholar]
- Ward DM, Leslie JD, Kaplan J. Homotypic lysosome fusion in macrophages: analysis using an in vitro assay. J Cell Biol. 1997;139:665–673. [Europe PMC free article] [Abstract] [Google Scholar]
- Ward DM, Perou CM, Lloyd M, Kaplan J. “Synchronized” endocytosis and intracellular sorting in alveolar macrophages: the early sorting endosome is a transient organelle. J Cell Biol. 1995;129:1229–1240. [Europe PMC free article] [Abstract] [Google Scholar]
- Wong SH, Xu Y, Zhang T, Hong W. Syntaxin 7, a novel syntaxin member associated with the early endosomal compartment. J Biol Chem. 1998;273:375–380. [Abstract] [Google Scholar]
Articles from Molecular Biology of the Cell are provided here courtesy of American Society for Cell Biology
Full text links
Read article at publisher's site: https://doi.org/10.1091/mbc.11.7.2327
Read article for free, from open access legal sources, via Unpaywall:
https://europepmc.org/articles/pmc14922?pdf=render
Citations & impact
Impact metrics
Citations of article over time
Alternative metrics
Smart citations by scite.ai
Explore citation contexts and check if this article has been
supported or disputed.
https://scite.ai/reports/10.1091/mbc.11.7.2327
Article citations
Integrative Human Genetic and Cellular Analysis of the Pathophysiological Roles of AnxA2 in Alzheimer's Disease.
Antioxidants (Basel), 13(10):1274, 21 Oct 2024
Cited by: 0 articles | PMID: 39456526 | PMCID: PMC11504888
Spatial organization of lysosomal exocytosis relies on membrane tension gradients.
Proc Natl Acad Sci U S A, 120(8):e2207425120, 17 Feb 2023
Cited by: 7 articles | PMID: 36800388 | PMCID: PMC9974462
Regulation of exosome release by lysosomal acid ceramidase in coronary arterial endothelial cells: Role of TRPML1 channel.
Curr Top Membr, 90:37-63, 03 Oct 2022
Cited by: 1 article | PMID: 36368874 | PMCID: PMC9842397
Identification of Synaptic DGKθ Interactors That Stimulate DGKθ Activity.
Front Synaptic Neurosci, 14:855673, 27 Apr 2022
Cited by: 1 article | PMID: 35573662 | PMCID: PMC9095502
The endosomal Q-SNARE, Syntaxin 7, defines a rapidly replenishing synaptic vesicle recycling pool in hippocampal neurons.
Commun Biol, 4(1):981, 18 Aug 2021
Cited by: 9 articles | PMID: 34408265 | PMCID: PMC8373932
Go to all (79) article citations
Other citations
Wikipedia
Data
Data behind the article
This data has been text mined from the article, or deposited into data resources.
BioStudies: supplemental material and supporting data
Nucleotide Sequences (2)
- (1 citation) ENA - AF182844
- (1 citation) ENA - U77942
Similar Articles
To arrive at the top five similar articles we use a word-weighted algorithm to compare words from the Title and Abstract of each citation.
Syntaxin 7 is localized to late endosome compartments, associates with Vamp 8, and Is required for late endosome-lysosome fusion.
Mol Biol Cell, 11(9):3137-3153, 01 Sep 2000
Cited by: 93 articles | PMID: 10982406 | PMCID: PMC14981
The R-SNARE endobrevin/VAMP-8 mediates homotypic fusion of early endosomes and late endosomes.
Mol Biol Cell, 11(10):3289-3298, 01 Oct 2000
Cited by: 99 articles | PMID: 11029036 | PMCID: PMC14992
Hrs regulates early endosome fusion by inhibiting formation of an endosomal SNARE complex.
J Cell Biol, 162(1):125-137, 01 Jul 2003
Cited by: 56 articles | PMID: 12847087 | PMCID: PMC2172712
The delivery of endocytosed cargo to lysosomes.
Biochem Soc Trans, 37(pt 5):1019-1021, 01 Oct 2009
Cited by: 83 articles | PMID: 19754443
Review
Funding
Funders who supported this work.
NHLBI NIH HHS (3)
Grant ID: R37 HL026922
Grant ID: R01 HL026922
Grant ID: HL26922
NIDDK NIH HHS (2)
Grant ID: T32 DK007115
Grant ID: T32DK07115
NINDS NIH HHS (1)
Grant ID: NS36670