Abstract
Free full text

Novel Carbapenem-Hydrolyzing β-Lactamase, KPC-1, from a Carbapenem-Resistant Strain of Klebsiella pneumoniae
Abstract
A Klebsiella pneumoniae isolate showing moderate to high-level imipenem and meropenem resistance was investigated. The MICs of both drugs were 16 μg/ml. The β-lactamase activity against imipenem and meropenem was inhibited in the presence of clavulanic acid. The strain was also resistant to extended-spectrum cephalosporins and aztreonam. Isoelectric focusing studies demonstrated three β-lactamases, with pIs of 7.2 (SHV-29), 6.7 (KPC-1), and 5.4 (TEM-1). The presence of blaSHV and blaTEM genes was confirmed by specific PCRs and DNA sequence analysis. Transformation and conjugation studies with Escherichia coli showed that the β-lactamase with a pI of 6.7, KPC-1 (K. pneumoniae carbapenemase-1), was encoded on an approximately 50-kb nonconjugative plasmid. The gene, blaKPC-1, was cloned in E. coli and shown to confer resistance to imipenem, meropenem, extended-spectrum cephalosporins, and aztreonam. The amino acid sequence of the novel carbapenem-hydrolyzing β-lactamase, KPC-1, showed 45% identity to the pI 9.7 carbapenem-hydrolyzing β-lactamase, Sme-1, from Serratia marcescens S6. Hydrolysis studies showed that purified KPC-1 hydrolyzed not only carbapenems but also penicillins, cephalosporins, and monobactams. KPC-1 had the highest affinity for meropenem. The kinetic studies also revealed that clavulanic acid and tazobactam inhibited KPC-1. An examination of the outer membrane proteins of the parent K. pneumoniae strain demonstrated that the strain does not express detectable levels of OmpK35 and OmpK37, although OmpK36 is present. We concluded that carbapenem resistance in K. pneumoniae strain 1534 is mainly due to production of a novel Bush group 2f, class A, carbapenem-hydrolyzing β-lactamase, KPC-1, although alterations in porin expression may also play a role.
The carbapenems, such as imipenem and meropenem, are used with increasing frequency in the United States and elsewhere for the treatment of multiresistant gram-negative nosocomial pathogens (21, 29, 30). Resistance to carbapenems is uncommon in enteric organisms; however, resistance can arise by three known mechanisms. First, high-level production of a chromosomal AmpC cephalosporinase combined with decreased outer membrane permeability due to loss or alteration of porins can result in carbapenem resistance. This has been shown for Enterobacter cloacae (28, 54), Enterobacter aerogenes (9, 10, 13, 23), Proteus rettgeri (54), Citrobacter freundii (32), Escherichia coli (11, 64), and Klebsiella pneumoniae (5, 7, 16). The second mechanism is production of a β-lactamase that is capable of hydrolyzing carbapenems (8, 30, 58) (e.g., IMI-1 [57], IMP-1 [3, 48], Nmc-A [42, 46], Sme-1 [41], and CfiA [69]). The third mechanism of resistance involves changes in the affinity of the target enzymes, the penicillin binding proteins, for carbapenems (15, 70).
In this study, a K. pneumoniae strain manifesting carbapenem resistance was collected through project ICARE (Intensive Care Antimicrobial Resistance Epidemiology) (4, 20) and analyzed for its mechanism(s) of carbapenem resistance. The results presented suggest that the carbapenem resistance phenotype of the strain is mainly caused by the production of a novel class A β-lactamase, KPC-1.
MATERIALS AND METHODS
Bacterial strains.
The carbapenem-resistant strain K. pneumoniae 1534 was collected from a hospital in North Carolina participating in project ICARE (4, 20). Identification of the isolate was confirmed using standard biochemical tests (17). E. coli HB101 [F− supE44 lacY1 ara-14 galK2 xyl-5 mtl-1 leuB6 Δ(mcrC-mrr) recA13 rpsL20 thi-1 Δ(gpt-proA)62 hsdSB20 λ−] (60) was used for electroporation of plasmid DNA isolated from K. pneumoniae 1534 and as a recipient in conjugal mating experiments (38). E. coli DH5α [supE44 ΔlacU169 (80 lacZΔM15) hsdR17 recA1 gyrA96 thi-1 relA1] was used for cloning the β-lactamase and for plasmid DNA preparation of the clone for DNA sequence analysis (60). K. pneumoniae ATCC 13883 (type strain) and K. pneumoniae 37, a carbapenem-susceptible clinical isolate from the Centers for Disease Control and Prevention collection, were used as controls for porin profiles.
Antimicrobial susceptibility testing.
Organisms were tested by broth microdilution using Mueller-Hinton broth (BD Biosciences, Sparks, Md.) as described by NCCLS (43) and by disk diffusion using Mueller-Hinton agar (Difco Laboratories, Detroit, Mich.) as described by NCCLS (44). Antimicrobial agent powders were obtained from the following sources: amikacin, amoxicillin, ampicillin, cefotaxime, ceftriaxone, chloramphenicol, gentamicin, piperacillin, trimethoprim-sulfamethoxazole, and tetracycline from Sigma Chemical Co., St. Louis, Mo.; aztreonam from Bristol-Myers Squibb, Princeton, N.J.; ceftazidime and tobramycin from Eli Lilly, Indianapolis, Ind.; cefoxitin from Merck, Rahway, N.J.; cefpodoxime from Pharmacia-Upjohn, Kalamazoo, Mich.; clavulanic acid from Smith-Kline Beecham, King of Prussia, Pa.; and tazobactam from Lederle, Pearl River, N.Y. All antimicrobial agent-containing disks were obtained from Fisher Scientific. E. coli ATCC 25922, Enterococcus faecalis ATCC 29212, Pseudomonas aeruginosa ATCC 27853 (45), E. coli HB101, and E. coli DH5α were used for quality control.
Isoelectric focusing of β-lactamases.
Crude cell lysates were prepared by a previously described freeze-thaw procedure (68). Isoelectric focusing was performed as described by Matthew and Harris (37). Cell extracts were loaded onto commercially prepared polyacrylamide gel plates (pH 3.5 to 9.5; Pharmacia LKB, Piscataway, N.J.) and electrophoresed to equilibrium by using an LKB Multiphor II apparatus (Pharmacia LKB). β-Lactamases were visualized by staining the isoelectric focusing gel with a 0.05% solution of nitrocefin (BD Biosciences). The isoelectric points of SHV-29 (7.2), TEM-1 (5.4), and KPC-1 (6.7) were calculated by comparison to TEM-12 (5.25), TEM-3 (6.3), SHV-2 (7.6), and SHV-4 (7.8).
Examination of porin genes and porin expression.
PCR amplifications were performed in a Thermoline Amplitron 1 thermal cycler using Taq polymerase (Pharmacia) with 30 cycles of amplification (1 min at 94°C, 1 min at 55°C, and 1 min at 72°C). The primers used to amplify porin genes were U681 and L1316 (Table (Table1).1). U681 and L1316 anneal to sequences conserved in porin genes located 215 and 850 bp downstream of the ompK36 start codon, respectively (14).
TABLE 1
Primers used in this study
Primer | Sequence |
---|---|
omp37, omp36, omp35 | |
![]() | 5′-CGG TTA CGG CCA GTG GGA ATA-3′ |
![]() | 5′-GAC GCA GAC CGA AAT CGA ACT-3′ |
pBR322 | |
![]() | 5′-CAC TAT CGA CTA CGC GAT CA-3′ |
![]() | 5′-ACG ATA GTC ATG CCC CGC GC-3′ |
blaKPC-1 | |
![]() | 5′-CTG GAG GAC TAT GAC TTC-3′ |
![]() | 5′-ATA CCA CCC TGA CAG CCG-3′ |
![]() | 5′-TGT CAC TGT ATC GCC GTC-3′ |
![]() | 5′-CGG GTT GGA CTC AAG ACG-3′ |
![]() | 5′-TGA TGC GGT ATT TTC TCC-3′ |
![]() | 5′-ACT GAC ACT GGG CTC TGC-3′ |
![]() | 5′-GAG CTG AAC TCC GCC ATC-3′ |
![]() | 5′-CTC AGT GCT CTA CAG AAA ACC-3′ |
![]() | 5′-TAA CCT TCG CCC TCA CAG ATA C-3′ |
![]() | 5′-TAT TTT TCC GAG ATG GGT GAC-3′ |
![]() | 5′-AGC AGA ACT AGA CGG CGA TAC AGT GAC ATC-3′ |
Outer membrane proteins were isolated by sarcosyl extraction of total membrane preparations as described previously (22). Protein concentrations were determined with the bicinchoninic acid protein assay kit (Pierce, Rockford, Ill.) as described by the manufacturer. The proteins were examined on sodium dodecyl sulfate (SDS)–8 to 15% polyacrylamide linear gradient gels. For OmpK37 analysis, electrophoresis of outer membrane proteins (OMPs) was performed on 11% acrylamide–0.2% bisacrylamide–0.1% SDS gels (14). Samples were boiled for 5 min in Laemmli's sample buffer before electrophoresis. Gels were visualized by staining with Coomassie Blue R250.
Western blotting of OmpK37, OmpK36, and OmpK35 was performed as follows. Proteins from SDS-polyacrylamide gel electrophoresis (PAGE) gels (4 to 16% acrylamide–0.2% bisacrylamide–0.1% SDS) were transferred to Immobilon-P filters (Millipore) as described previously (14, 22). Filters were blocked in 1% bovine serum albumin in phosphate-buffered saline (PBS). After washing, the filters were incubated with diluted (1:100) anti-OmpK37, anti-OmpK36, or anti-OmpK35 antibody (14, 22) and then with alkaline phosphatase-labeled goat anti-rabbit immunoglobulin G (Sigma; 1:5,000). The filters were developed as described previously (14, 22). All the incubations were carried out at room temperature for 1 h in 1% bovine serum albumin–0.05% Tween 20–PBS, and after incubations with the antiserum, the filters were washed with 0.05% Tween 20–PBS (22).
Plasmid profile analysis and probing.
Plasmid DNA from K. pneumoniae 1534 was isolated using the method described by Portnoy et al. (52). The DNA preparations were electrophoresed on 0.85% agarose gels in the presence of 0.5× TBE buffer (45 mM Tris-HCl, 45 mM boric acid, and 1.25 mM EDTA, pH 8.3) at a constant voltage of 90 V for 15 h at 4°C. Supercoiled plasmid DNAs of pDK9 (165 kb) and R1 (97.6 kb) and the plasmids in E. coli V517 (56.7, 5.8, 4.09, 3.15, 2.83, and 2.2 kb) were used as size standards.
The DNA was transferred from the agarose gel to a positively charged nylon membrane as described by the manufacturer (Zeta-Probe; Bio-Rad Laboratories, Hercules, Calif.) and fixed by baking for 3 h at 80°C. The DNA on the filter was hybridized with a 1,010-bp digoxigenin-labeled blaKPC-1 DNA probe. Hybridization (at 65°C for 15 h) was performed using the Genius nonradioactive nucleic acid labeling and detection system (Boehringer Mannheim Biochemicals, Indianapolis, Ind.) according to the manufacturer's protocol. The plasmids pDK9 and the plasmids in E. coli V517 were used as negative controls. Purified K. pneumoniae 1534 DNA was the positive control.
Carbapenem inactivation assay.
In order to determine whether resistance to imipenem and meropenem was likely caused by production of a β-lactamase, a disk diffusion bioassay was performed. A suspension of E. coli DH5α equivalent to a 0.5 McFarland standard was inoculated on a Mueller-Hinton agar plate as for disk diffusion. Then, five imipenem or meropenem disks were applied evenly spaced on the plate, four on the periphery and one in the center of the plate. A suspension of the organism to be tested for the presence of carbapenemase was adjusted to the turbidity of a 0.5 McFarland standard, and a loop was used to make a 15-mm streak on each side of one imipenem or meropenem disk on the periphery of the plate (the center disk served as the control). Four different organism suspensions were used on each plate. The plates were incubated at 37°C for 18 to 20 h. Alterations in shape of the zones of inhibition around the test organism were considered to be indications of carbapenemase activity. Negative controls for carbapenemase production included E. coli HB101 and K. pneumoniae ATCC 13883.
Filter mating.
The filter mating protocol described by McDougal et al. (38) was used, except that incubation was performed at both 30 and 37°C. E. coli HB101 was used as the recipient.
Transformation.
Plasmid DNA prepared from K. pneumoniae 1534 via a Qiagen plasmid midi-prep kit (Qiagen, Chatsworth, Calif.) was electroporated into E. coli HB101 as described previously (60). Transformants were selected on Luria-Bertani agar containing 120 μg of streptomycin/ml and 1.5 μg of imipenem/ml.
Cloning of blaKPC-1.
Total cellular DNA was isolated from K. pneumoniae 1534 using a Qiagen plasmid midi-prep kit and was partially digested with BamHI and HindIII (Gibco BRL, Gaithersburg, Md.). A derivative of pBR322, pBR322-catI (in which blaTEM was replaced by catI), was constructed and used as the vector. The partially digested DNA was ligated into the corresponding sites of the vector by using T4 DNA ligase (Gibco BRL) and electroporated into E. coli DH5α. Clones were selected on Luria-Bertani agar plates containing 40 μg of chloramphenicol/ml and 1.5 μg of imipenem/ml. The initial clone, containing a 7.5-kb insert from K. pneumoniae 1534, was reduced in size to 3.4 kb via complete digestion with BamHI and religation into the same site of pBR322-catI by using T4 ligase. The plasmid DNA, pBR322-catI-blaKPC-1 containing the cloned blaKPC-1, was prepared with a QIAprep Spin plasmid kit (Qiagen) and used in the DNA sequencing reactions. The cloned fragment was sequenced as described below by using pBR322-derived primers (Table (Table1,1, primers 1 and 2). Other primers used to complete the sequencing of the 3.4-kb insert are listed in Table Table11 (primers 3 to 12).
blaSHV-, blaTEM-, and blaKPC-1-specific PCR.
The primers and the PCR conditions used for amplification of blaSHV and blaTEM were those described by Rasheed et al. (56). The novel β-lactamase gene, blaKPC-1, was amplified from the parent strain, K. pneumoniae 1534, by using primers 5 and 10 (Table (Table1).1). The PCRs (total volume, 100 μl) contained 0.5 μM (each) primers, 250 μM deoxynucleoside triphosphates, 2 mM MgCl2, and 2.5 U of Taq DNA polymerase prepared in 1× reaction buffer supplied by the manufacturer (Perkin-Elmer, Applied Biosystems Division [PE-ABI], Foster City, Calif.). The reactions were amplified in a GeneAmp PCR System 9600 thermal cycler (PE-ABI). Cycling parameters were 5 min at 95°C, followed by 35 cycles of denaturation at 95°C for 1 min, annealing at 58°C for 30 s, and extension at 72°C for 1 min 30 s. The PCR amplification was ended by a final extension cycle at 72°C for 10 min.
Sequencing.
Sequencing of the PCR products for blaSHV, blaTEM, and blaKPC-1 was performed after purification of the PCR products with a QIAquick PCR purification kit (Qiagen). blaKPC-1 was initially sequenced from plasmid DNA (pBR322-catI-blaKPC-1). Cycle sequencing reactions were performed in a GeneAmp PCR System 9600 thermal cycler with an ABI Prism dRhodamine Terminator cycle sequencing ready reaction kit according to instructions provided by the vendor (PE-ABI). Sequencing reaction products were purified on Centri-Sep spin columns (Princeton Separations, Adelphia, N.J.) and analyzed on an ABI Prism 377 DNA sequencer (PE-ABI).
To eliminate errors due to PCR amplification, leading and lagging strands were sequenced from two independent PCR products for all three β-lactamase genes. The sequences obtained from PCR products were also compared to the sequences obtained from two independent clones (both leading and lagging strands) of blaKPC-1.
DNA sequencing data were analyzed by DNASIS for Windows (Hitachi Software Genetic Systems, San Francisco, Calif.). The DNA and protein sequences of other β-lactamases were from the European Molecular Biology Laboratory and the Swiss-Prot data banks. BLAST and BLASTX programs from the web site of the National Center for Biotechnology Information (www.ncbi.nlm.nih.gov /BLAST/) were used to identify blaKPC-1. The amino acid sequences of known class A β-lactamases (Nmc-A [42], IMI-1 [57], and Sme-1 [41]) were aligned by using the multiple alignment (Higgins-Sharp) option of DNASIS for Windows. The restriction map of the 3.4-kb cloned fragment was determined from the sequencing data by using DNASIS. The dendrogram presented in Fig. Fig.66 was generated by DNASIS from the alignment of the amino acid sequence of KPC-1 with known β-lactamases (Higgins-Sharp) representative of class A β-lactamases, including CARB-3 (27), PSE-1 (24), SHV-1 (39), LEN-1 (2), TEM-1 (65), MEN-1 (6), OXY1 (19), CITDI (50), YENT (61), Nmc-A (42), IMI-1 (57), Sme-1 (41), L2 (71), ROB-1 (31), and BRO-1 (D. Beaulieu, L. Piche, T. R. Parr, Jr., K. Roeger-Lawry, P. Rosteck, and P. H. Roy, β-lactamase BRO-1 precursor [penicillinase], gi:24975813, GenBank, 1996); representative of class B β-lactamases, including IMP-1 (48) and CfiA (69); representative of class C β-lactamases (ACT-1 [7]); and representative of class D β-lactamases (OXA-1 [49]).
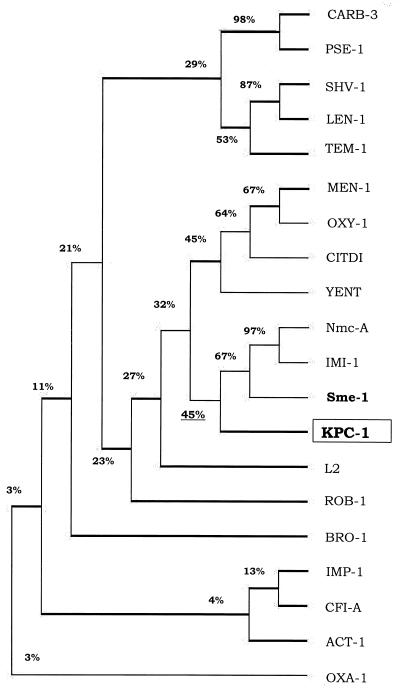
Dendrogram showing similarity of 20 β-lactamases. The dendrogram was constructed by using DNASIS for Window's multiple alignment option (Higgins-Sharp). Sixteen of the β-lactamases are class A enzymes, CARB-3 from P. aeruginosa (27), PSE-1 from P. aeruginosa (24), SHV-1 from E. coli (39), LEN-1 from K. pneumoniae (2), TEM-1 from E. coli (65), MEN-1 from E. coli (6), OXY-1 from Klebsiella oxytoca (19), CITDI from C. diversus (50), YENT from Y. enterocolitica (61), Nmc-A from E. cloacae (42), IMI-1 from E. cloacae (57), Sme-1 from S. marcescens (41), L2 from Stenotrophomonas maltophilia (71), ROB -1 from Haemophilus influenzae (31), and BRO-1 from Moraxella catarrhalis (D. Beaulieu, L. Piche, T. R. Parr, Jr., K. Roeger-Lawry, P. Rosteck, and P. H. Roy, β-lactamase BRO-1 precursor [penicillinase], gi:2497581, Gen Bank, 1996); IMP-1 from S. marcescens (48) and Cfi-A from Bacteroides fragilis (69) were included as representatives of class B (metallo-β-lactamases); ACT-1 from K. pneumoniae (7) represents class C, AmpC β-lactamases; and OXA-1 from E. coli (49) represents class D enzymes.
Transcriptional start site of blaKPC-1.
The transcriptional start site of blaKPC-1 was mapped by primer extension. Total RNA was isolated from parent strain K. pneumoniae 1534 and E. coli DH5α harboring the plasmid DNA encoding KPC-1 by using the SV total RNA isolation system (Promega Corporation, Madison, Wis.) as described by the manufacturer. Primer number 13 (Table (Table1)1) was labeled at the 5′ end by [γ-32P]ATP (3,000 Ci/mmol, 10 mCi/ml). The primer labeling and the primer extension reactions were carried out by using primer extension system avian myeloblastosis virus reverse transcriptase (Promega) as described by the manufacturer. The sequencing reactions were performed by using the same primer with Promega's fmol DNA sequencing system as described by the manufacturer. The plasmid DNA encoding KPC-1 was used as template in the sequencing reactions. The primer extension and sequencing reaction products were run on an 8% denaturing polyacrylamide gel containing 7 M urea in 1× TBE (60). The gel was dried and exposed to a PhosphorImager intensifying screen for 5 h and analyzed by using the PhosphorImager system (Molecular Dynamics) with ImageQuant software.
β-lactamase purification.
The cloned KPC-1 β-lactamase was purified for kinetic analysis from E. coli strain HY122 (DH5α/pBR322-catI-blaKPC-1). Three 1-liter cultures of trypticase soy agar supplemented with 1 μg of imipenem/ml and 40 μg of chloramphenicol/ml were grown overnight at 37°C. Bacteria were harvested by centrifugation and washed with 50 mM phosphate buffer, pH 7.0. The pellets were resuspended in 10 ml of 0.2 M sodium acetate, pH 5.5, and subjected to five freeze-thaw cycles (68). The lysate was centrifuged at 20,000 × g, and the β-lactamase activity of the supernatant was separated by column chromatography through Sephadex G-100 in 50 mM phosphate buffer, pH 7.0. Protein in peak fractions containing nitrocefin-hydrolyzing activity was precipitated with 90% ammonium sulfate; pellets were resuspended and dialyzed in 20 mM Tris, pH 7.8, at 4°C. The KPC-1 β-lactamase was eluted from a Q-Sepharose anion-exchange column in 20 mM Tris, pH 7.8, with a 0 to 0.5 M NaCl gradient. The protein concentration of the Q-Sepharose fractions was determined with the Pierce BCA protein assay. The purity of the KPC-1 preparation was determined by scanning densitometry of a colloidal blue-stained NuPAGE 10% bisacrylamide–Tris gel.
Kinetic studies.
Initial hydrolysis rates were measured in 50 mM phosphate buffer (pH 7.0) on a Shimadzu UV-1601 spectrophotometer at 25°C (68). Km and Vmax values were obtained by averaging results from Lineweaver-Burk, Eadie-Hofstee, Hanes-Woolf, and Cornish-Bowden direct linear plot analyses. Substrates were assayed on at least two separate days, with cephaloridine included as a reference each day. Inhibition of hydrolysis of 100 μM nitrocefin was measured after a 5-min preincubation of enzyme with inhibitor in 100 μl of phosphate buffer (pH 7.0). Fifty percent inhibitory concentrations were determined from inhibition graphs of percent control activity versus concentration of inhibitor.
Nucleotide sequence accession numbers.
The nucleotide sequence of blaKPC-1 reported in this study will appear under GenBank accession number AF297554. The nucleotide sequence of blaSHV-29 reported here will appear under GenBank accession number AF301532.
RESULTS
Antimicrobial susceptibility patterns of K. pneumoniae 1534.
The MICs of a variety of antimicrobial agents tested against K. pneumoniae 1534 are shown in Table Table2.2. The isolate was resistant to imipenem and meropenem which had MICs of 16 μg/ml. The isolate was also resistant to extended-spectrum cephalosporins and aztreonam. Although the MIC of amoxicillin did not decrease when it was tested in combination with clavulanic acid, the MIC of imipenem was reduced from 16 to 2 μg/ml when tested in the presence of clavulanic acid (4 μg/ml). Similarly, the MIC of meropenem was reduced from 16 to 1 μg/ml in the presence of clavulanic acid (Table (Table2).2).
TABLE 2
Antimicrobial susceptibility patterns of K. pneumoniae 1534, E. coli DH5α clone, and E. coli HB101 transformant
Antimicrobial agent(s) | MIC (μg/ml)
| ||||
---|---|---|---|---|---|
K. pneumoniae 1534 (parent) | E. coli DH5α | E. coli DH5α (pBR322-catI-blaKPC-1) | E. coli HB101 transformant containing blaKPC-1 | E. coli HB101 | |
Imipenem | 16 | ≤0.25 | 8 | 8 | ≤0.25 |
Imipenem-clavulanic acida | 2 | ≤0.25 | 0.5 | 0.5 | ≤0.25 |
Meropenem | 16 | ≤0.25 | 4 | 4 | ≤0.25 |
Meropenem-clavulanic acid | 1 | ≤0.25 | ≤0.25 | ≤0.25 | ≤0.25 |
Ampicillin | >64 | 2 | >64 | >64 | 4 |
Amoxicillin-clavulanic acid | >32/16 | 2/1 | >32/16 | >32/16 | 2/1 |
Piperacillin-tazobactam | >128/4 | ≤1/4 | >128/4 | >128/4 | ≤1/4 |
Ceftazidime | 32 | ≤2 | 8 | 8 | ≤2 |
Cefoxitin | 32 | ≤2 | 16 | 16 | 4 |
Cefpodoxime | >16 | 0.5 | >16 | >16 | ≤0.25 |
Cefotaxime | 64 | ≤1 | 16 | 8 | ≤1 |
Ceftriaxone | >64 | ≤1 | 32 | 32 | ≤1 |
Aztreonam | >64 | ≤1 | >64 | >64 | ≤1 |
Gentamicin | >16 | ≤0.25 | ≤0.25 | ≤0.25 | ≤0.25 |
Tobramycin | >16 | ≤0.25 | ≤0.25 | ≤0.25 | ≤0.25 |
Trimethoprim-sulfamethoxazole | >8 | ≤0.12 | ≤0.12 | ≤0.12 | ≤0.12 |
Chloramphenicol | 32 | 4 | >32 | 4 | 4 |
Imipenem and meropenem resistance involves production of β-lactamase.
Isoelectric focusing of K. pneumoniae 1534 revealed three β-lactamases, with pIs of 7.2, 6.7, and 5.4 (Fig. (Fig.1,1, lane 3) (the extra bands between pIs 6.7 and 5.4 in lanes 1 to 3 are presumably degradation products of the pI 6.7 β-lactamase). To determine whether resistance to carbapenems could be attributed to the production of a β-lactamase, a disk diffusion carbapenem inactivation assay was performed. The assay was positive (Fig. (Fig.2),2), suggesting that a β-lactamase was involved in hydrolysis of imipenem and meropenem in K. pneumoniae 1534 and in the E. coli HB101 transformant (Fig. (Fig.2,2, disks 1 and 5, respectively). This β-lactamase was named K. pneumoniae carbapenemase 1, or KPC-1. The presence of EDTA did not inhibit the activity of the β-lactamase, nor did the addition of ZnCl2 enhance the β-lactamase activity against imipenem or meropenem (data not shown).
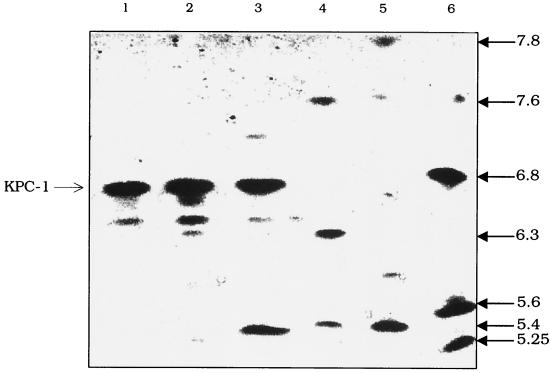
Isoelectric focusing patterns of carbapenem-resistant K. pneumoniae 1534. The gel was stained with nitrocefin, which is specific for β-lactamases. Lane 1, cell lysate prepared from the imipenem-resistant E. coli DH5α containing the blaKPC-1 gene on pBR322-catI; lane 2, cell lysate prepared from imipenem-resistant E. coli HB101 that was transformed with K. pneumoniae 1534 DNA; lane 3, cell lysate prepared from K. pneumoniae 1534; lane 4, cell lysates prepared from strains producing SHV-2 (pI of 7.6), TEM-3 (pI of 6.3), and TEM-1 (pI of 5.4); lane 5, cell lysates prepared from strains producing TEM-1 (pI of 5.4) and SHV-4 (pI of 7.8); lane 6, cell lysates prepared from strains producing TEM-12 (pI of 5.25), TEM-10 (pI of 5.6), SHV-3 (pI of 6.8), and SHV-2 (pI of 7.6). The extra bands between pIs 6.7 and 5.4 are presumably degradation products of KPC-1 (lanes 1 to 3). The pIs of the β-lactamases were calculated by using the known pIs of TEM-12 (pI of 5.25), TEM-1 (pI of 5.4), TEM-10 (pI of 5.6), TEM-3 (pI of 6.3), SHV-3 (pI of 6.8), SHV-2 (pI of 7.6), and SHV-4 (pI of 7.8).
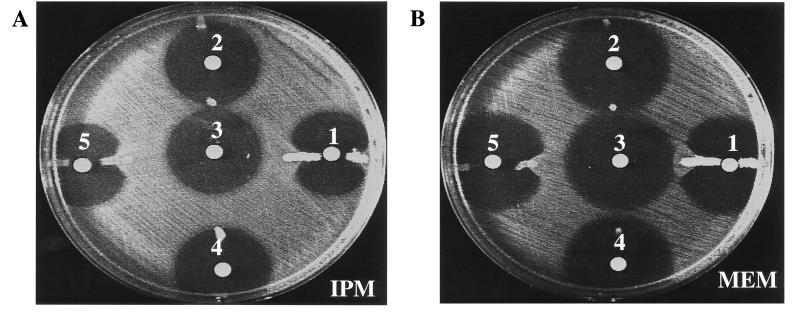
(A) Inhibition assay with imipenem (IPM); (B) inhibition assay with meropenem (MEM). Disks: 1, lysate of K. pneumoniae 1534 (parent strain); 2, lysate of E. coli HB101/pBR322-catI; 3, disk control with imipenem (A) or meropenem (B); 4, lysate of K. pneumoniae ATCC 13883; 5, lysate of E. coli HB101/pBR322-catI-blaKPC-1 clone.
PCR and DNA sequence analysis detected the presence of blaSHV and blaTEM.
Isoelectric focusing results suggested the presence of blaSHV (pI 7.2) and blaTEM (pI 5.4) derivatives (Fig. (Fig.1,1, lane 3). PCR analysis using blaSHV- and blaTEM-specific primers confirmed the presence of these genes in K. pneumoniae 1534 (data not shown). DNA sequencing results identified the genes as blaTEM-1 and a novel blaSHV-29 (H. Yigit, G. J. Anderson, and F. C. Tenover, unpublished data).
Cloning of the blaKPC-1 gene from the E. coli DH5α transformant.
The filter mating results showed that the carbapenem resistance determinant in K. pneumoniae 1534 was not encoded by a conjugative plasmid. However, electroporation results demonstrated that the gene encoding resistance to carbapenems, extended-spectrum cephalosporins, and aztreonam was located on an approximately 50-kb plasmid (Fig. (Fig.3A,3A, lane 4).
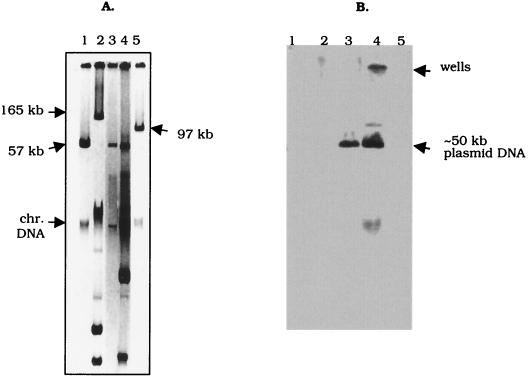
(A) Plasmid profiles of K. pneumoniae 1534 and an E. coli transformant on 0.85% agarose gel. Lane 1, plasmid RP4 (57-kb); lane 2, plasmid pDK9 (165-kb); lane 3, total DNA prepared from an E. coli HB101 transformant; lane 4, total DNA isolated from K. pneumoniae 1534; lane 5, plasmid R1 (97-kb). Chr., chromosomal. (B) Southern blot of the gel shown in panel A after hybridization with a 1,010-bp blaKPC-1-specific probe. Lane 1, RP4 DNA; lane 2, pDK9 DNA; and lane 5, R1 DNA served as negative controls for the probe. DNA isolated from K. pneumoniae 1534 (lane 4) was used as a positive control. Lane 3, DNA isolated from an E. coli HB101 transformant of K. pneumoniae 1534 DNA that was imipenem resistant.
A blaKPC-1-specific DNA probe confirmed the location of blaKPC-1 on the 50-kb plasmid (Fig. (Fig.3B,3B, lanes 3 and 4). Resistance to chloramphenicol, gentamicin, tobramycin, and trimethoprim-sulfamethoxazole was not linked to imipenem and meropenem resistance (Table (Table2,2, see HB101 transformant).
Neither blaSHV-29 nor blaTEM-1 was present in the transformants examined by PCR analysis. However, blaKPC-1-specific products were generated from the E. coli transformants (data not shown), which was consistent with the isoelectric focusing data showing only one β-lactamase with a pI of 6.7 (Fig. (Fig.1,1, lane 2).
The antibiogram of the E. coli DH5α blaKPC-1 clone (which harbors the plasmid pBR322-catI-blaKPC-1, which contains the 3.4-kb cloned insert from K. pneumoniae 1534) is shown in Table Table2.2. The MICs are consistent with those for E. coli HB101 transformants containing the 50-kb plasmid encoding KPC-1 (Table (Table2).2). This demonstrates that the β-lactamase gene located on the 3.4-kb fragment is responsible for the resistance to carbapenems, extended-spectrum cephalosporins, and aztreonam. E. coli DH5α(pBR322-catI-blaKPC-1) encoded a single β-lactamase with a pI of 6.7, as shown by isoelectric focusing (Fig. (Fig.1,1, lane 1).
Sequence analysis of blaKPC-1.
The nucleotide sequence of the carbapenemase gene was determined from pBR322-catI-blaKPC-1. The nucleotide sequence of blaKPC-1 (Fig. (Fig.4)4) did not show significant similarity to those of any other β-lactamase genes or other sequences in GenBank.

Nucleotide and deduced amino acid sequences of the novel class A carbapenemase KPC-1 isolated from K. pneumoniae 1534. The −10 and −35 regions of the putative promoter are underlined. The transcription start site indicated by the mRNA primer extension study is marked as +1. RBS indicates a potential ribosome-binding site (62). The HindIII recognition site and the conserved amino acid residues for class A carbapenemases are underlined (26, 35, 36). The start and stop codons for blaKPC-1 are marked with asterisks (59).
The location of the blaKPC-1 open reading frame (ORF) was verified by HindIII subcloning of the 3.4-kb cloned fragment. The fragment contained one HindIII site that cleaves in the predicted ORF of blaKPC-1 (Fig. (Fig.4).4). Both of the HindIII sub-fragments of the original 3.4-kb fragment were subcloned, but neither encoded a functional β-lactamase. Therefore, this region was proven to represent the ORF for blaKPC-1.
blaKPC-1 contained an 879-bp coding region which encoded a 32,230-Da protein containing 293 amino acids (Fig. (Fig.4).4). The protein contains a serine-serine-phenylalanine-lysine (S-S-F-K) and a lysine-threonine-glycine (K-T-G) motif. These sequences, S-X-X-K and K-T-G, are characteristic of class A serine β-lactamases (25, 26). Other conserved residues among class A carbapenemases (55, 63) are shown in bold and underlined in Fig. Fig.44.
A six-nucleotide sequence located eight nucleotides upstream from the ATG initiation codon (AAGGAA) was identified as a possible ribosome-binding site (59, 62) for blaKPC-1. mRNA primer extension results identified the GATTAC sequence as the −10 region and determined the mRNA starting site for blaKPC-1 (the mRNA start site is denoted +1 in Fig. Fig.4).4). The primer extension results also suggested GATAAT as the putative −35 region (Fig. (Fig.44).
Amino acid sequence similarity to other β-lactamases.
KPC-1 had the highest similarity to the class A carbapenem-hydrolyzing β-lactamases, in particular to Sme-1 (45% identical) from Serratia marcescens (41). The amino acid sequences of three known class A carbapenemases were aligned with the amino acid sequence of KPC-1 (Fig. (Fig.5).5). The regions critical for catalysis are shown in Fig. Fig.55 (25, 26, 35, 36, 55). The residues known or suggested to be important for class A carbapenemase activity by Raquet et al. (55) are marked by asterisks (C69, S70, K73, H105, S130, R164, E166, N170, D179, R220, K234, S237, and C238). Interestingly, H105 and S237 were not conserved in KPC-1. KPC-1 contained a tryptophan at position 105 and a threonine at position 237. KPC-1 also showed similarity to some other class A β-lactamases, including Nmc-A (44%) (42), IMI-1 (43%) (57), OXY-1 (39%) (19), and MEN-1 (34%) (6). These results, in combination with the kinetic data presented in the next section, place KPC-1 in Bush functional group 2f (8), together with other class A carbapenemases.
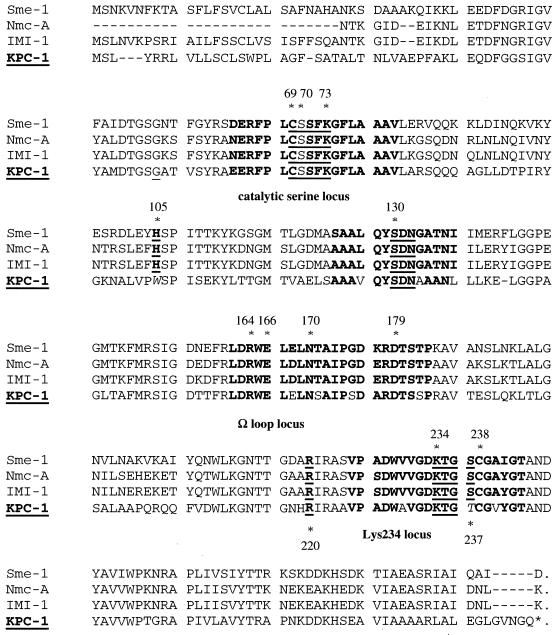
Alignment of the amino acid sequence of KPC-1 with that of Sme-1 from S. marcescens S6 (41), Nmc-A from E. cloacae NOR1 (42), and IMI-1 from E. cloacae (57). Dashes indicate the gaps that were inserted to optimize the alignment. The numbering is from Ambler et al. and Sykes (1, 67). The conserved domains of class A β-lactamases are underlined (12, 26, 35, 36, 55, 63, 66). The residues suggested to play a critical role for carbapenemase activity are marked by asterisks and underlined (63, 66). The positions where the KPC-1 sequence diverges from these conserved residues (positions 105 and 237) are indicated in italics.
A dendrogram was generated (Fig. (Fig.6)6) from the amino acid sequence alignment of KPC-1 with other class A β-lactamases. This shows that KPC-1 is more closely related to the subgroup including YENT (61), CITDI (50), OXY-1 (19), and MEN-1 (6) than to TEM-1 (65) and SHV-1 (39). This finding is in agreement with the results presented for Sme-1 by T. Nass et al. (41). KPC-1 had low amino acid sequence similarity to β-lactamases of class B, e.g., IMP-1 (48) and Cfi-A (69); class C, e.g., AmpC and ACT-1 (7); and class D, e.g., OXA-1 (49) (Fig. (Fig.6).6).
Kinetic parameters.
The kinetic parameters for KPC-1 are summarized in Table Table3.3. The KPC-1 enzyme used in these studies was approximately 90% pure. KPC-1 hydrolyzed β-lactams from the penicillin, cephalosporin, carbapenem, and monobactam groups. The highest kcat values were obtained with cephaloridine, which demonstrated a kcat value approximately four times higher than that for cephalothin or nitrocefin and three times higher than that for ampicillin. Piperacillin, benzylpenicillin, and cloxacillin, the other penicillins tested, had similar kcat values approximately 10 to 14 times lower than that of cephaloridine. KPC-1 showed hydrolytic activity against the carbapenems; hydrolysis of imipenem occurred at a rate approximately 25 times slower than that of cephaloridine. Meropenem had a kcat value four times lower than that for imipenem. Hydrolysis rates for cefotaxime and ceftazidime were 24 and 3,370 times lower than the value obtained for cephaloridine. Between the two extended-spectrum cephalosporins tested, cefotaxime had the highest kcat value, approximately 140 times higher than the kcat value for ceftazidime. Of the 13 substrates used in these experiments, cefoxitin and ceftazidime had the lowest hydrolysis rates.
TABLE 3
Substrate profile for KPC-1
Substrate | kcat (s−1) | Relative kcat | Km (μM) | Relative kcat/Km |
---|---|---|---|---|
Cephaloridine | 340![]() ![]() | 100 | 560![]() ![]() | 100 |
Cephalothin | 75![]() ![]() | 22 | 53![]() ![]() | 240 |
Nitrocefin | 78![]() ![]() | 23 | 24![]() ![]() | 530 |
Cefotaxime | 14![]() ![]() | 4.2 | 160![]() ![]() | 15 |
Cefoxitin | 0.26![]() ![]() | 0.08 | 120![]() ![]() | 0.4 |
Ceftazidime | 0.10![]() ![]() | 0.03 | 94![]() ![]() | 0.2 |
Benzylpenicillin | 32![]() ![]() | 9.6 | 23![]() ![]() | 230 |
Ampicillin | 110![]() ![]() | 33 | 130![]() ![]() | 140 |
Cloxacillin | 25![]() ![]() | 7.4 | 100![]() ![]() | 41 |
Piperacillin | 24![]() ![]() | 7.2 | 18![]() ![]() | 230 |
Imipenem | 12.4![]() ![]() | 3.7 | 81![]() ![]() | 26 |
Meropenem | 3.0![]() ![]() | 0.9 | 12![]() ![]() | 41.5 |
Aztreonam | 20![]() ![]() | 5.9 | 310![]() ![]() | 11 |
KPC-1 had the highest affinity for meropenem, with a Km of 12 μM. Other substrates with low Kms were piperacillin, penicillin, and nitrocefin, with Kms that ranged from 18 to 24 μM. Cephaloridine had the highest Km, 560 μM.
Hydrolytic efficiencies, measured by kcat/Km, revealed that penicillin was hydrolyzed by KPC-1 approximately two times more efficiently than cephaloridine. Nitrocefin had the highest catalytic efficiency of the substrates tested, with a value five times that of cephaloridine. The hydrolytic efficiencies of KPC-1 for imipenem and meropenem were substantially less than that of cephaloridine (26 and 42%, respectively). Cefotaxime was hydrolyzed the most efficiently of the three extended-spectrum cephalosporins tested, with a kcat/Km value of 15% that for cephaloridine. The hydrolytic efficiencies for cefoxitin and ceftazidime were 250 and 500 times lower than that for cephaloridine, respectively.
Clavulanic acid and tazobactam both inhibited the KPC-1 β-lactamase, with 50% inhibitory concentrations of 10.5 ± 1.3 μM for clavulanic acid and 0.374 ± 0.034 μM for tazobactam (data not shown). Tazobactam was a better inhibitor of KPC-1 than was clavulanic acid. No inhibition was observed when the enzyme was tested with 5 mM EDTA at pH 7.0.
Analysis of K. pneumoniae 1534 OMPs.
The MICs of meropenem, ceftazidime, and cefotaxime were lower for the E. coli HB101 transformants and E. coli DH5α(pBR322-catI-blaKPC-1) than for the parent strain. This may be due to alterations in porin expression, which are known to increase the MICs of these drugs for K. pneumoniae isolates (5, 7, 34). Thus, an examination of the porin profile of the parent strain K. pneumoniae 1534 was performed, and the results were compared to those for two extended-spectrum-cephalosporin-susceptible strains, K. pneumoniae ATCC 13883 (type strain) and K. pneumoniae strain 37, a clinical isolate.
PCR analysis showed that K. pneumoniae strains 1534, ATCC 13883, and 37 each encode all three porin genes, ompK35, ompK36, and ompK37. The expression of the porin genes was examined by purification of OMPs, separation on SDS-PAGE, and Western blotting with anti-OmpK36, anti-OmpK35, and anti-OmpK37 antisera. As reported by Hernandez-Alles et al. (22), the presence of OmpK35 and OmpK36 cannot be detected solely by their migration in gels, since in some strains OmpK36 migrates faster than OmpK35. As shown in Fig. Fig.7A,7A, this is the case for strains ATCC 13883 (lane 2) and 37 (lane 4), where OmpK35 apparently migrates slower than OmpK36. K. pneumoniae 1534, on the other hand, appears to lack OmpK35. A Western blot using anti-OmpK36-specific antisera (Fig. (Fig.7B)7B) confirmed the identity of OmpK36 bands for K. pneumoniae strains ATCC 13883 (lane 1), 1534 (lane 2), and 37 (data not shown). Anti-OmpK35 sera is known to cross-react with OmpK36 (22) and reacted, as predicted, with two bands for ATCC 13883 (OmpK35 and OmpK36) (Fig. (Fig.7C,7C, lane 1) but only one band with K. pneumoniae 1534 (OmpK36) (lane 2). These results suggest that OmpK35 was not expressed in K. pneumoniae 1534. None of the three K. pneumoniae strains appeared to express OmpK37 when tested with OmpK37-specific antisera (data not shown). (LamB and OmpA are not porins and do not contribute to antimicrobial resistance phenotypes.)
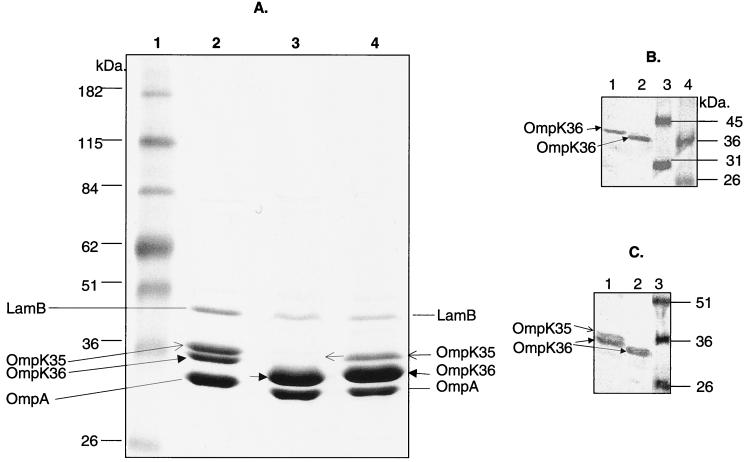
SDS-PAGE and Western blot analysis of OMPs of K. pneumoniae 1543 and two carbapenem-susceptible control strains. (A) SDS-PAGE analysis of OMPs. Lane 1, molecular mass markers; lane 2, OMPs prepared from K. pneumoniae ATCC 13883; lane 3, OMPs prepared from K. pneumoniae 1534; lane 4, OMPs prepared from K. pneumoniae 37. (B) Western blot analysis of OMPs using anti-OmpK36 antisera. Lane 1, OMPs prepared from ATCC 13883; lane 2, OMPs prepared from K. pneumoniae 1534; lanes 3 and 4, molecular mass markers in kilodaltons. (C) Western blot analysis of OMPs using anti-OmpK35 antisera. Lane 1, OMPs prepared from ATCC 13883; lane 2, OMPs prepared from K. pneumoniae 1534; lane 3, molecular mass markers in kilodaltons. (The OmpK35 antibody cross-reacts with OmpK36 [22].)
DISCUSSION
Carbapenems, such as imipenem and meropenem, are antibacterial agents with activity against many gram-negative, gram-positive, and anaerobic microorganisms. Carbapenems are often used to treat multidrug-resistant isolates, especially strains producing extended-spectrum β-lactamases (21, 29, 30, 47, 58). However, the recent appearance of β-lactamases capable of hydrolyzing carbapenems, in addition to other mechanisms of carbapenem resistance, creates an increasing therapeutic dilemma (21, 29, 30, 47, 58). Therefore, a better understanding of carbapenem resistance mechanisms is critical to optimizing therapy.
Here we describe the fourth class A β-lactamase with high carbapenem-hydrolyzing activity isolated from a strain of Enterobacteriaceae. The enzyme KPC-1 shows 45% amino acid identity to Sme-1 (41) from S. marcescens S6. Unlike KPC-1, the other three class A carbapenemases (Nmc-A [42], IMI-1 [57], and Sme-1 [41]) show >90% similarity to each other at the nucleotide level (41, 47). These findings suggest that KPC-1 might be derived from a different ancestor than the other three more closely related class A carbapenemases.
As Fig. Fig.66 shows, KPC-1 and other class A carbapenemases belong to a subgroup that also includes CITDI, a penicillinase from Citrobacter diversus (50), YENT from Yersinia enterocolitica (61), and the extended-spectrum β-lactamase MEN-1 from E. coli (6). The alignment of KPC-1 to the other class A β-lactamases demonstrated that KPC-1 contained cysteine residues at positions 69 and 238 that can form a disulfide bridge (55), but KPC-1 did not contain the C77 or C123 residues present in TEM and SHV derivatives and in PSE-4 (51, 55). However, the other well-conserved residues among class A carbapenemases, S70, K73, S130, R164, E166, N170, D179, R220, and K234 (12, 55, 63) were present in KPC-1. The histidine at position 105 in Sme-1 (41, 63) was not conserved in KPC-1, which had a tryptophan at that site. Although H105 was conserved in Sme-1 (41), Nmc-A (42), and IMI-1 (57) and has been suggested to be important for carbapenemase activity, studies by Raquet et al. (55) have shown that the H105 is not sufficient for the carbapenemase activity. However, it might be useful to generate a mutant version of KPC-1 that contained this residue and to change H105 to A in Sme-1 to determine the direct effect of the H105 residue on the carbapenem hydrolysis activity. Kinetic study and X-ray analysis of Nmc-A (33, 66) have suggested that N132, which is conserved in KPC-1, is critical for giving additional space for carbapenems and cephamycins.
The serine at position 237 is directly involved in imipenem hydrolysis in Sme-1 (63). An S237A substitution in Sme-1 resulted in a twofold reduction in the imipenem hydrolysis rate (63). Although an S-to-T change is not as dramatic, it may explain the lower carbapenem hydrolysis rate of KPC-1 in comparison to Sme-1. Sougakoff et al. (63) postulated that a hydroxyl residue (Ser or Thr) at position 237 of class A β-lactamases is generally associated with higher hydrolytic efficiency against cephalosporins. KPC-1 hydrolyzed cefotaxime better than does Sme-1, suggesting that the presence of threonine in KPC-1 extends the activity of the enzyme to a broader array of cephalosporins.
All class A carbapenemase genes have promoter regions that contain well-conserved −10 and −35 regions (41, 42, 57). Since there was no DNA similarity between blaKPC-1 and other class A carbapenemase genes, mRNA primer extension was performed to determine the start site and putative promoter region of the blaKPC-1 gene. The results revealed that blaKPC-1 contains a reasonably conserved −10 region (18, 59, 62) but does not contain a−35 region that resembles the E. coli consensus sequence (62; W. S. Reznikoff, personal communication). Thus, a putative −35 region 17 bp away from the −10 region has been assigned (Fig. (Fig.4).4). This finding suggests involvement of alternative transcription factors (W. S. Reznikoff, personal communication) in the transcription of blaKPC-1.
The regions flanking blaSme-1 (40), blaIMI-1 (57), and blaNmc-A contained a second ORF in an orientation opposite to that of the β-lactamase structural gene. These ORFs encoded Lys-R-type DNA-binding proteins shown to be acting as positive regulators of the β-lactamase structural genes (40, 42, 57). Therefore, the regions upstream and downstream of the blaKPC-1 gene were examined for other potential ORFs. No homology to Lys-R-type DNA-binding proteins was found on either side of blaKPC-1.
Finally, we searched for alterations of one or more of the three porin proteins described for K. pneumoniae strains that are associated with increased MICs of extended-spectrum cephalosporins or carbapenems (14, 22, 53). K. pneumoniae 1534 encodes all three porin genes as shown by PCR analysis. However, SDS-PAGE analysis of K. pneumoniae 1534 porin profiles in combination with the Western blot analysis of purified OMP preparations with OmpK35, OmpK36, and OmpK37-specific antibodies showed that K. pneumoniae 1534 expresses only OmpK36 (Fig. (Fig.7).7). This is consistent with the observations of Hernandez-Alles et al., who reported that most extended-spectrum β-lactamase-producing K. pneumoniae strains produced only OmpK36 and not OmpK35 (22). Studies by Domenech-Sanchez et al. suggest that the newly identified porin, OmpK37, might be used by carbapenems to gain access to the cell (14); however, this porin is strongly down-regulated under standard laboratory conditions and is often only seen in the absence of OmpK35 and OmpK36 expression. Thus, its contribution to resistance in K. pneumoniae 1534 remains unclear.
In conclusion, we have isolated and characterized a novel class A carbapenemase from K. pneumoniae 1534, designated KPC-1. The data presented here show that KPC-1 is mainly responsible for the carbapenem resistance of this strain. Our data also show that the absence of OmpK35 in particular is consistent with K. pneumoniae clinical isolates harboring class A β-lactamases.
ACKNOWLEDGMENTS
H.Y. is a recipient of an American Society for Microbiology-National Center for Infectious Diseases postdoctoral fellowship.
We thank John E. McGowan, Jr., from Rollins School of Public Health of Emory University for providing the strains from project ICARE. We give special thanks to W. Reznikoff for his assistance with the primer extension studies and his suggestions on determination of the promoter region. Also, we thank A. Glasgow for her helpful suggestions on experimental design, J. K. Rasheed for his suggestions on the manuscript, N. Clark for suggestions regarding Southern blots, and J. Swenson for her help in obtaining the required media and antimicrobial agents for this study.
REFERENCES
Articles from Antimicrobial Agents and Chemotherapy are provided here courtesy of American Society for Microbiology (ASM)
Full text links
Read article at publisher's site: https://doi.org/10.1128/aac.45.4.1151-1161.2001
Read article for free, from open access legal sources, via Unpaywall:
https://europepmc.org/articles/pmc90438?pdf=render
Citations & impact
Impact metrics
Citations of article over time
Alternative metrics
Smart citations by scite.ai
Explore citation contexts and check if this article has been
supported or disputed.
https://scite.ai/reports/10.1128/aac.45.4.1151-1161.2001
Article citations
Isolation and characterization of lytic bacteriophage vB_KpnP_23: A promising antimicrobial candidate against carbapenem-resistant Klebsiella pneumoniae.
Virus Res, 350:199473, 01 Oct 2024
Cited by: 0 articles | PMID: 39332682 | PMCID: PMC11474366
Molecular epidemiology of extended-spectrum beta-lactamase-producing-<i>Klebsiella</i> species in East Tennessee dairy cattle farms.
Front Microbiol, 15:1439363, 24 Sep 2024
Cited by: 0 articles | PMID: 39380685 | PMCID: PMC11458399
Clinical outcomes and safety of polymyxin B versus tigecycline combination therapy for pneumonia of carbapenem-resistant Klebsiella pneumoniae: a retrospective cohort study.
Ann Med, 56(1):2397087, 06 Sep 2024
Cited by: 0 articles | PMID: 39239861 | PMCID: PMC11382689
Transmission Dynamics and Novel Treatments of High Risk Carbapenem-Resistant Klebsiella pneumoniae: The Lens of One Health.
Pharmaceuticals (Basel), 17(9):1206, 12 Sep 2024
Cited by: 0 articles | PMID: 39338368 | PMCID: PMC11434721
Review Free full text in Europe PMC
Prevalence and distribution of carbapenem-resistant Enterobacterales in companion animals: A nationwide study in the United States using commercial laboratory data.
J Vet Intern Med, 38(5):2642-2653, 17 Aug 2024
Cited by: 1 article | PMID: 39152797 | PMCID: PMC11423476
Go to all (942) article citations
Other citations
Data
Data behind the article
This data has been text mined from the article, or deposited into data resources.
BioStudies: supplemental material and supporting data
Nucleotide Sequences (2)
- (1 citation) ENA - AF297554
- (1 citation) ENA - AF301532
Similar Articles
To arrive at the top five similar articles we use a word-weighted algorithm to compare words from the Title and Abstract of each citation.
Carbapenem-resistant strain of Klebsiella oxytoca harboring carbapenem-hydrolyzing beta-lactamase KPC-2.
Antimicrob Agents Chemother, 47(12):3881-3889, 01 Dec 2003
Cited by: 128 articles | PMID: 14638498 | PMCID: PMC296202
Emergence of Serratia marcescens, Klebsiella pneumoniae, and Escherichia coli Isolates possessing the plasmid-mediated carbapenem-hydrolyzing beta-lactamase KPC-2 in intensive care units of a Chinese hospital.
Antimicrob Agents Chemother, 52(6):2014-2018, 10 Mar 2008
Cited by: 133 articles | PMID: 18332176 | PMCID: PMC2415814
Biochemical properties of a carbapenem-hydrolyzing beta-lactamase from Enterobacter cloacae and cloning of the gene into Escherichia coli.
Antimicrob Agents Chemother, 37(5):939-946, 01 May 1993
Cited by: 95 articles | PMID: 8517720 | PMCID: PMC187856
Imipenem-Relebactam and Meropenem-Vaborbactam: Two Novel Carbapenem-β-Lactamase Inhibitor Combinations.
Drugs, 78(1):65-98, 01 Jan 2018
Cited by: 167 articles | PMID: 29230684
Review