Abstract
Free full text

Cryptococcal Glucuronoxylomannan Inhibits Adhesion of Neutrophils to Stimulated Endothelium In Vitro by Affecting Both Neutrophils and Endothelial Cells
Abstract
Cryptococcal infections are often characterized by a paucity of leukocytes in the infected tissues. Previous research has shown that the capsular polysaccharide glucuronoxylomannan (GXM) inhibits leukocyte migration. In this study we investigated whether the capsular polysaccharide GXM affects the migration of neutrophils (polymorphonuclear leukocytes [PMN]) through the endothelium by interfering with adhesion in a static adhesion model. Pretreatment of PMN with GXM inhibited PMN adhesion to tumor necrosis factor alpha (TNF-α)-stimulated endothelium up to 44%. Treatment of TNF-α-stimulated endothelium with GXM led to a 27% decrease in PMN adhesion. GXM treatment of both PMN and endothelium did not have an additive inhibitory effect. We demonstrated that GXM-induced L-selectin shedding does not play an important role in the detected inhibition of adhesion. L-selectin was still present on PMN in sufficient amounts after GXM treatment, since it could be further inhibited by blocking antibodies. Furthermore, blocking of GXM-related L-selectin shedding did not abolish the GXM-related inhibition of adhesion. GXM most likely exerts its effect on PMN by interfering with E-selectin-mediated binding. The use of blocking monoclonal antibodies against E-selectin, which was shown to decrease adhesion in the absence of GXM, did not cause additive inhibition of PMN adhesion after GXM pretreatment. The use of blocking antibodies also demonstrated that the inhibiting effect found after GXM treatment of endothelium probably involves interference with both intercellular adhesion molecule-1 and E-selectin binding.
Cryptococcus neoformans is an encapsulated yeast that can cause life-threatening meningitis in immunocompromised patients. Compared to bacterial meningitis, cryptococcal meningitis is usually characterized by a relative paucity of leukocytes in the cerobrospinal fluid and infected tissues (4, 6), despite adequate stimulation of cytokine production (6, 7, 12, 46, 60, 65, 67). Previous research has shown that the immunomodulatory effects of cryptococcal capsular polysaccharides contribute to the scant leukocyte infiltrates often observed. Cryptococcal culture filtrate, the isolated capsular polysaccharide glucuronoxylomannan (GXM), and mannoprotein 4 (10) all inhibit the influx of leukocytes into inflammation sites (10, 17, 48). Since GXM and mannoprotein 4 have intrinsic chemoattracting properties (10, 15, 16) and high titers of both are found in the bloodstream during infections (10, 13, 25), it has been hypothesized that these compounds, by cross-desensitization, prevent leukocytes from properly responding to chemoattractants, thereby contributing to the scant infiltrate often reported in cryptococcal infections. This has already been demonstrated for GXM in humans as well as in experimental infections in animal models (17, 48, 49, 50). Another mechanism for the diminished leukocyte transendothelial migration, one not yet analyzed in detail in the literature, could be the interference with leukocyte adhesion to the endothelium at the site of infection. This is the subject of the present study.
Leukocyte adhesion to the endothelium is initiated by the activation of endothelium by cytokines already present at the site (e.g., interleukin-1 [IL-1] and tumor necrosis factor alpha [TNF-α]). This leads to endothelial cytokine production and the upregulation of adhesion molecules. The first step of adhesion is the margination and rolling of leukocytes on the activated endothelium and depends on interactions between selectins (E-selectin on endothelium, P-selectin on platelets and endothelium, and L-selectin on leukocytes) and their counterligands. The leukocytes are then activated by cytokines, which results in the shedding of L-selectin from the leukocyte surface and the increased expression of integrins (e.g., CD11b/CD18 and VLA-4) thereon. The integrins are involved in the subsequent firm binding of the leukocytes to the ligands (e.g., the intercellular adhesion molecules [ICAM] and vascular cell adhesion molecule-1) expressed on endothelium, after which the cells finally transmigrate (see references 5, 30, and 56 for reviews). Interference with these binding processes might lead to a diminished efflux of leukocytes in the direction of the inflamed tissues and might involve inhibition of endothelial cytokine production, the blocking or shedding of adhesion molecules from the surface of leukocytes or endothelium, or the inhibition of protein expression.
Although various research groups have shown that cryptococcal culture filtrate and GXM adequately stimulate in vitro production of monocytic cytokines (IL-1β, IL-6, IL-8, TNF-α, and gamma interferon) (7, 12, 46, 57, 65, 67), whole cryptococci appear to have an inhibiting effect on the production of chemokines by the endothelium (57). Impairment of this trigger, leading to the extravasation of leukocytes, could hypothetically lead to interference with the adhesion process. However, since both capsular and acapsular cryptococci demonstrate this inhibiting effect (57) and GXM is part of the capsule, GXM is probably not responsible for the interference.
With regard to leukocyte adhesion, the effects of GXM on endothelial cells have not yet been studied. Much better documented are its effects on neutrophils (polymorphonuclear leukocytes [PMN]). GXM has been shown to induce L-selectin shedding from the surfaces of PMN and lymphocytes (18, 20), and since this shedding occurs prematurely, an inhibiting effect on leukocyte rolling can be expected. L-selectin shedding caused by GXM coincides with the upregulation of the integrin CD11b/CD18 (18), which might favor the subsequent firm binding of PMN to endothelium. Dong and Murphy, however, showed that GXM also binds to CD18 (19), which might in turn have a negative effect on firm binding. Thus, despite present knowledge of the effects of GXM on leukocyte adhesion molecules, the actual effects of GXM on the process of firm leukocyte binding are not well known.
In this study, we investigated the effect of GXM on the process of PMN adhesion to TNF-α-stimulated endothelium by using a static adhesion assay. Stimulation of endothelium by TNF-α leads to an increase in the expression of adhesion molecules and thus to increased binding of leukocytes (5, 31, 34, 44, 62). The static adhesion assay mainly involves binding processes that contribute to firm leukocyte adhesion. It has been extensively demonstrated that neutrophil CD11/CD18 binding to ICAM on the endothelium and E-selectin binding to its counterligands on PMN play comparable roles in this adhesion assay (33, 43, 54, 55). Their roles are partly codependent, since E-selectin binding mediates CD18 activation and thus promotes CD11b/18 binding to ICAM (30, 43, 47). The role of L-selectin in the static adhesion assay is probably restricted to serving as one of the ligands of E-selectin (5, 41, 68), since L-selectin participates mainly in leukocyte rolling and a threshold dynamic shear force is required for its binding (28).
The objectives of this study were (i) to demonstrate whether GXM inhibits PMN adhesion to endothelium and (ii) to establish whether GXM exerts this action by affecting PMN, endothelial cells, or both. Furthermore, we wanted to establish which adhesion molecules are affected by GXM. Using the static adhesion assay, we observed that GXM inhibits PMN adhesion to activated endothelium in a concentration-dependent manner and exerts this effect by an effect on PMN as well as on the endothelium.
MATERIALS AND METHODS
MAbs and chemicals.
The following blocking monoclonal antibodies (MAbs) were used: DREG 56 (anti-L-selectin, immunoglobulin G1 [IgG1]) (41) from the American Type Culture Collection (ATCC), BBA2 (anti-E-selectin, IgG1) from R&D systems (Oxon, United Kingdom), and RR1/1 (anti-ICAM-1, IgG1) (33, 34, 44) from the Fourth Leukocyte Typing Workshop. MAb IB4 (anti-CD18, IgG2a) (61, 64) and the control MAb W6/32 (anti-HLA A/B/C/, IgG2a) (34, 55) were raised in our own laboratory from mouse hybridomas (HB-10164 and HB-95, respectively) obtained from the ATCC. Except for MAb DREG 56 (anti-L-selectin), which was used at a concentration of 20 μg/ml (41), all blocking antibodies were used at a concentration of 10 μg/ml. TNF-α was purchased from Roche (Almere, The Netherlands). The tissue inhibitor TAPI-0 [KD-IX-73-4; HO-NH-CO-CH2-CH(CH2-CH(CH3)2)-CO-Nal-Ala-NH-CH2-CH2-NH2], an inhibitor of L-selectin shedding (26) from Peptides International (Louisville, Ky.) was dissolved at 25 mg/ml in dimethyl sulfoxide and further diluted with phosphate-buffered saline (PBS) to 1 mg/ml. Fibronectin from human plasma was obtained from Micronic (Lelystad, The Netherlands), and polymyxin B sulfate was obtained from Sigma (St. Louis, Mo.). A Superose 6 HR gel filtration column was purchased from Pharmacia (Uppsala, Sweden). The fluorescent probe 2′,7′-bis-(2-carboxyethyl)-5-(6)-carboxyfluorescein acetoxymethyl ester (BC-ECF AM) was purchased from Molecular Probes Europe (Leiden, The Netherlands). Human pooled serum, obtained from a pool of healthy donors, was heat inactivated at 56°C for 30 min and subsequently filtered. GXM, purified from C. neoformans (NIH 37, serotype A, chemotype 5, ATCC 62066), was a generous gift from R. Cherniak, Georgia State University, Atlanta (8). Specific primer pairs for E-selectin and ICAM-1 were synthesized by Amersham Pharmacia Biotech (Uppsala, Sweden).
Isolation and culture of HUVEC.
Primary human umbilical vein endothelial cells (HUVEC) were obtained according to the method described by Jaffe et al. (37) and cultured in sterile RPMI 1640 (RPMI) supplemented with 10 μg of gentamicin per ml and 20% inactivated pooled human serum. Only passages 2 to 4 were used for the experiments.
Isolation, labeling, and GXM treatment of human PMN.
Human PMN were isolated as described before (63). Briefly, blood from healthy volunteers was collected in Vacuette tubes containing sodium heparin as an anticoagulant (Greiner), diluted with an equal volume of pyrogen-free PBS, and layered on top of a Ficoll (Pharmacia)-Histopaque (Sigma) gradient. After centrifugation for 20 min at 230 × g, PMN were collected from the Histopaque phase. They were subsequently subjected to a brief hypotonic shock with mQ water, washed, and suspended at 2 × 106 cells/ml in sterile RPMI supplemented with 0.1% human serum albumin (RPMI-HSA). The neutrophils were then labeled with 3.3 μM BC-ECF AM for 20 min at 4°C. After one washing step, the cells were incubated with either GXM dissolved in RPMI-HSA or RPMI-HSA alone for 1 h at 37°C.
Pyrogen-free conditions.
To ensure lipopolysaccharide (LPS)-free conditions, all of the materials were kept under pyrogen-free conditions. GXM was tested for the presence of LPS by using a Limulus amoebocyte lysate assay (Coatest Endotoxin Diagnostica, Mölndal, Sweden) with a sensitivity of 25 pg of Escherichia coli LPS per ml. Although the LPS concentration was always less than 0.6 ng/ml, all experiments were carried out in the presence of 10 μg of polymyxin B sulfate per ml to neutralize any undetected LPS contamination (21). Polymyxin B sulfate had neither a stimulating nor an inhibiting effect on the adhesion of PMN to the endothelium when tested in the static adhesion assay (as described below).
Static adhesion assay and GXM treatment of HUVEC (51).
HUVEC were plated on fibronectin-coated Nunc 72-well microtiter plates (Life Technologies, Breda, The Netherlands) and grown to confluency in 1 to 2 days. The cells were washed twice with RPMI-gentamicin and stimulated with 10 ng of TNF-α per ml (corresponding to 200 to 300 U/ml) in culture medium for 6 h at 37°C (31, 51). In some experiments HUVEC were treated with GXM suspended in culture medium for 1 h at 37°C prior to, simultaneously with, or after stimulation with TNF-α. After three washes with RPMI-gentamicin, donor PMN were added to the wells at a concentration of 106/ml (PMN/endothelial cell ratio = 5:1) and left to adhere for 15 min at 37°C. After the endothelium was carefully washed, the adherent cells were fixed with 2% paraformaldehyde (pH 7.4) and counted within a fixed frame of 1 mm2 in the center of each well by fluorescence microscopy (Leitz Fluovert inverted microscope). Measurements were performed in quadruple wells for each variable. All tests were repeated using different batches of donor cells. Following the GXM incubation, in some experiments, PMN and endothelial cells were also incubated with blocking MAbs for 15 min at room temperature. This was then followed by the adhesion experiment.
L-selectin ELISA.
The quantification of L-selectin levels in the supernatants of PMN treated with GXM was performed with a commercial enzyme-linked immunosorbent assay (ELISA) kit (R&D Systems) according to the manufacturer's guidelines. The sensitivity of the ELISA was less than 0.3 ng of L-selectin per ml.
Reverse transcription-PCR.
RNA expression of endothelial adhesion molecules was measured by reverse transcription-PCR. Briefly, HUVEC in 25-ml culture flasks were exposed to either GXM or culture medium for 2 h, followed by stimulation with TNF-α for another 6 h. After washing, total endothelial RNA was extracted using guanidium isothiocyanate, followed by reverse transcription of 1 μg of RNA per sample with oligo(dT) priming. Amplification by PCR of the target cDNA was conducted using specific primer pairs for E-selectin (sense primer 5′-GCTACAATTCTTCCTGCTC-3′ and antisense primer 5′-GGAGAGTCCAGCAGCAGAAAGTC-3′) or for ICAM-1 (sense primer 5′-CAAGAGGAAGGAGCAAGACT-3′ and antisense primer 5′-ACAAGAGGACAAGGCATAGC-3′). A primer pair for the housekeeping protein GAPDH (glyceraldehyde-3-phosphate dehydrogenase) (sense primer 5′-CACCATGGAGAAGGCTGGGG-3′ and antisense primer 5′-ACCAAAGTTGTCATGGATGACC-3′) was used to equalize the amounts of RNA present in each sample. PCR-amplified products were separated by agarose gel electrophoresis.
Preparation of FITC-labeled GXM and detection of GXM binding to HUVEC.
Fluorescein isothiocyanate (FITC)-labeled GXM was prepared by activation of GXM with cyanogen bromide and subsequent reaction with FITC (32). HUVEC monolayers were stimulated with TNF-α for 6 h, after which the cells were detached with 1.5 mM EDTA in a calcium-free buffer. After being washed, cells were incubated with FITC-labeled GXM in calcium-containing Hanks balanced salt solution medium for 1 h, followed by flow cytometric analysis of the immunofluorescently stained cells.
Gel filtration chromatography and cryptococcal antigen ELISA.
GXM samples (in 0.5 ml PBS) of two different concentrations (2 mg/ml and 2 μg/ml) were applied to a Superose 6.0 column (1.5 by 30 cm; Pharmacia) and eluted with PBS at a flow rate of 12 ml/h. Dextran blue (2,000 kDa), apoferritin (440 kDa), and HSA (67 kDa) were used as molecular mass markers and detected at 280 nm. Fractions of 0.5 ml were collected and assayed for GXM by using a commercial ELISA (Premier Cryptococcal Antigen ELISA; Meridian Bioscience, Boxtel, The Netherlands) according to the manufacturer's guidelines. The sensitivity of the ELISA was 0.1 ng/ml.
Statistics.
All adhesion experiments were repeated using different donors of both PMN and endothelial cells. Per experiment, each variable was tested in quadruple wells and the counts of adherent PMN were averaged. The average cell counts from multiple experiments were statistically analyzed. Since the level of PMN binding usually varies considerably from experiment to experiment due to donor-to-donor variability, all data were analyzed by a univariate analysis of variance (ANOVA) test with a two-factor ANOVA, analyzing only main effects (in which the donor was set as a blocking factor). To compare multiple treatment groups to each other or to the control, the ANOVA was then followed by a post hoc analysis by Bonferroni's test. P values of <0.05 were considered significant. In Results the adherence data are expressed as adherence percentages followed by a 95% confidence interval (95% CI).
RESULTS
Preincubation of PMN with GXM inhibits the adhesion of PMN to stimulated HUVEC.
We first investigated the effect of preincubation of donor PMN with GXM on PMN adhesion to the endothelium. PMN were incubated with GXM at different concentrations for 1 h at 37°C, after which a static adhesion assay was performed with confluent monolayers of TNF-α-stimulated HUVEC. Figure Figure1A1A shows an example of the variation in the level of PMN binding from experiment to experiment due to donor-to-donor variability. The results of two representative experiments are presented. Despite the variation, the results of all experiments were averaged and analyzed by a variance test using a two-factor ANOVA. Figure Figure1B1B shows the averaged adhesions from seven experiments expressed as adhesion percentages. When GXM was not washed off before the adhesion process, PMN adhesion was inhibited by 39% (Fig. (Fig.1B)1B) (P < 0.01; 95% CI, 19 to 52%). Significant inhibition was detected at GXM concentrations of between 10 ng/ml and 100 μg/ml. The optimal inhibiting effect was reached at a concentration of 100 ng/ml, after which it gradually diminished at higher concentrations. Washing the PMN twice after incubation with GXM and prior to the adhesion process did not affect the level of inhibition (Fig. (Fig.1C)1C) (inhibition of 44% after washing; P = 0.005), indicating that the effect is not merely due to an aspecific mechanical impairment of adhesion by the presence of large polysaccharide molecules.

Preincubation of PMN with GXM inhibits adhesion to TNF-α-stimulated HUVEC. BC-ECF AM-labeled PMN were incubated with GXM for 1 h at 37°C, followed by adhesion to TNF-α-stimulated HUVEC monolayers (see Materials and Methods). Adherent PMN were counted by fluorescence microscopy, and observations were made in quadruplicate. The negative control consisted of the adhesion of untreated PMN to unstimulated endothelium, and the positive control was the adhesion of untreated PMN to TNF-α-stimulated endothelium. (A) Results of two separate representative experiments performed with cells from different healthy donors (squares and circles). The data are presented as the mean absolute counts of adherent PMN. (B) The results of seven experiments were averaged and subsequently expressed as the percentage of adhesion compared to the positive control (i.e., adhesion of untreated PMN to stimulated endothelium = 100%). (C) To evaluate the effect of washing, PMN were washed twice with medium after PMN-GXM incubation. Adhesion is expressed as the percentage of adhesion of the positive control (either washed or unwashed PMN). The results of seven experiments were averaged. , P < 0.05. The error bars represent standard deviations.
When we varied the time of incubation of PMN with GXM between 15 min and 2 h, the inhibiting effect did not change. In contrast, when GXM was added simultaneously with PMN to the endothelium, no significant inhibiting effect was noted (data not shown). The latter finding supports the view that the inhibiting effect of GXM in these experiments is due to the effect of GXM on the PMN during the incubation period and not to its effect on the endothelium during the subsequent short adhesion period. Moreover, the generation of an inhibitory effect after 15 min of preincubation suggests that the mechanism of action by which GXM affects neutrophils does not involve de novo protein synthesis.
Effect of GXM on endothelium: incubation of TNF-α-stimulated endothelium with GXM leads to a decrease in PMN adhesion.
To investigate the effect of GXM on endothelial adhesiveness, nonstimulated endothelium was incubated with GXM at different concentrations and for different lengths of time. We found that GXM treatment did not affect the adhesiveness of resting endothelium (data not shown).
The effect of GXM on the adhesiveness of TNF-α-stimulated endothelium was then investigated. The incubation of HUVEC monolayers with GXM prior to or simultaneously with TNF-α inhibited PMN adhesion by 27% compared to endothelium treated with TNF-α alone (Fig. (Fig.2A)2A) (P < 0.05; 95% CI, 12 to 42%). The inhibitory effects on endothelium were detected at lower GXM concentrations (1 pg/ml to 1 μg/ml) than when PMN were incubated with GXM. To demonstrate that GXM is able to bind to and affect the endothelium, both unstimulated and stimulated HUVEC were incubated with FITC-labeled GXM, followed by fluorescence-activated cell sorter analysis of the fluorescently stained cells. Binding of FITC-labeled GXM to HUVEC could be demonstrated at concentrations of 0.1 mg/ml and 1 μg/ml, albeit at low levels (Fig. (Fig.2B).2B). GXM was found to bind more readily to stimulated HUVEC.
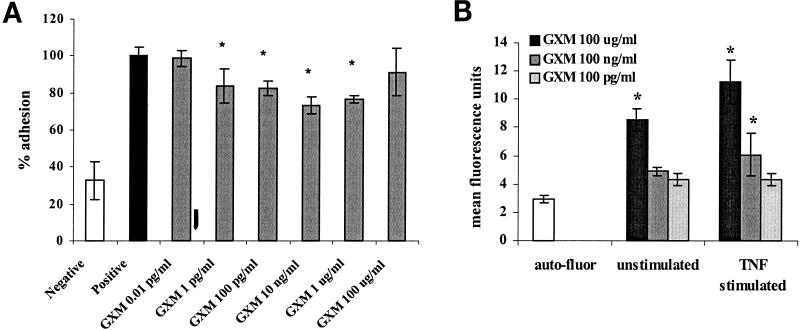
(A) Preincubation of endothelium with GXM prior to TNF-α stimulation leads to a decrease in PMN adhesion. HUVEC monolayers were incubated with GXM for 1 h at 37°C, after which TNF-α was added to a final concentration of 10 ng/ml and left for another 6 h. After three washes, fluorescent PMN were added and left for 15 min at 37°C. After washing, adherent cells were counted by means of fluorescence microscopy. The results of three experiments were averaged and expressed as percentages of the value for the positive control (i.e., adhesion of PMN to HUVEC treated with TNF-α alone = 100%). , P < 0.05. (B) GXM is able to bind to HUVEC. Unstimulated and TNF-α-stimulated HUVEC were incubated with FITC-labeled GXM for 20 min. After washing, fluorescently stained cells were evaluated by fluorescence-activated cell sorter analysis. The results of five experiments were averaged.
, P < 0.05. The error bars represent standard deviations.
Since the same degree of inhibition was also observed when GXM was added after TNF-α stimulation, inhibition of protein synthesis does not seem to be the underlying mechanism. The effect of GXM on TNF-α-related upregulation of endothelial adhesion molecules was examined by RNA analysis. HUVEC were incubated with GXM for various times, followed by stimulation with TNF-α. Untreated HUVEC expressed ICAM-1 but no E-selectin. TNF-α stimulation led to an increase in both ICAM-1 and E-selectin transcription, which was not influenced by GXM (results not shown).
Previous research has already demonstrated that GXM does not decrease the viability of the endothelium (36). Nevertheless, to ensure that the effect of GXM on adhesiveness was not due to a decrease in the viability of endothelial cells, HUVEC monolayers were incubated with GXM for different lengths of time. The viability of the endothelium proved to be >95% as evaluated by trypan blue exclusion and light microscopy examination.
Compared to treatment of either PMN or endothelium, treatment of both PMN and endothelium with GXM does not lead to an additive inhibiting effect.
To mimic the in vivo situation during cryptococcal infections, as well as to look at possible additive effects, we investigated the effect that the preincubation of both endothelium and PMN with GXM has on adhesion. GXM treatment of both the endothelium and PMN (as described in Material and Methods) led to a decrease in adhesion of at most 38% (results not shown) (P < 0.05; 95% CI, 27 to 49%); a 27 to 38% inhibition was found at GXM concentrations of between 10 pg/ml and 1 μg/ml, with diminishing inhibition at higher concentrations. Compared to the treatment of PMN or endothelium alone, no statistical significant additive inhibiting effect was found, even at those concentrations where both treatments separately had exerted an effect in earlier experiments.
GXM aggregation.
In the experiments described above, the effects of GXM on both PMN and endothelium diminished at higher GXM concentrations. Earlier, Dong and Murphy described this phenomenon for other effects of GXM (19). A possible mechanism might be aggregation of the polysaccharide molecules at higher concentrations, thereby lowering the actual numbers of reactive molecules. To support this hypothesis, we analyzed GXM preparations at two concentrations (2 mg/ml and 2 μg/ml) on a Superose 6 HR chromatography column. Elution was followed by detection of GXM with a commercial cryptococcal antigen ELISA. The eluted fractions from the highest concentration were diluted 1,000-fold in order to reach the detection range of the ELISA. Figure Figure33 shows that the elution curve of the 2-mg/ml sample is shifted towards the higher-molecular-mass range compared to the 2-μg/ml sample, supporting the theory that GXM tends to aggregate at higher concentrations. In addition, low-molecular-mass GXM (in the fractions with the higher numbers) is overrepresented in the lower-concentration curve.
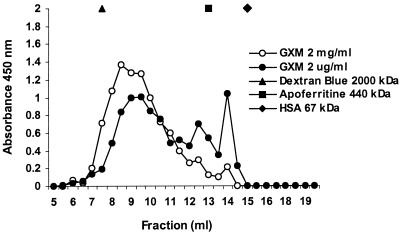
GXM size chromatography. Samples of GXM at a concentration of 2 mg/ml or 2 μg/ml (0.5 ml) were applied to a Superose 6 gel filtration column. The eluted fractions from the 2-mg/ml sample were diluted 1,000-fold, followed by GXM detection with a commercial cryptococcal antigen ELISA. The eluted fractions from the 2-μg/ml sample were tested undiluted. The y axis represents the spectrophotometric absorbance measured at 450/630 nm. The results are the averages from two ELISAs. The molecular mass markers dextran blue (2,000 kDa), apoferritin (440 kDa), and HSA (67 kDa) were directly detected during chromatography by spectrophotometry at 280 nm.
TAPI-0, a blocker of L-selectin shedding, does not affect GXM-related inhibition of PMN adhesion.
Since GXM is known to shed the adhesion molecule L-selectin from the surface of PMN (18), we investigated whether this mechanism could account for the observed effect of GXM on PMN, i.e., the inhibition of PMN adhesion to the endothelium. We evaluated the effect of an inhibitor of L-selectin shedding, TAPI-0 (26), on the GXM-related inhibition of PMN adhesion.
First, to verify the effect of TAPI-0 on L-selectin shedding, we incubated 106 PMN in Hanks balanced salt solution with 50 μg of TAPI-0 per ml at 37°C for 15 min and then added 0.1 mg of GXM per ml. The supernatants were subsequently analyzed for L-selectin with a commercial L-selectin ELISA kit, and the results of three experiments performed in duplicate were averaged. The mean L-selectin shedding caused by GXM (corrected for the L-selectin level present in the supernatants of untreated PMN) was 8.1 ng/ml (standard deviation, 2.9 ng/ml). Pretreatment of the PMN with TAPI-0 inhibited GXM-related L-selectin shedding by 80% (mean L-selectin concentration, 1.5 ng/ml [standard deviation, 0.9 ng/ml]).
Second, we compared the adhesions of GXM-treated and TAPI-0- and GXM-treated PMN to the endothelium. As Fig, 4 shows, the inhibition of adhesion caused by GXM (35%) persisted in the presence of TAPI-0, the blocker of L-selectin shedding (37%) (no significant difference; P = 0.4). Thus, L-selectin shedding does not seem to be the mechanism underlying the inhibition of PMN adhesion to the endothelium after preincubation of PMN with GXM.
GXM treatment of PMN affects E-selectin-mediated adhesion rather than CD11b/CD18-ICAM-1- or L-selectin-mediated adhesion.
Several investigators have shown that together, CD11b/CD18- and E-selectin-mediated binding bring about the adhesion in static adhesion models (33, 43, 54, 55). The relative contribution of these adhesion molecules to binding, however, depends on the expression of ICAM-1 and E-selectin on the endothelium, the amount of which differs over time. The expression of E-selectin has reached its maximum after a 6-h stimulation of the endothelium by cytokines (TNF-α and IL-1β), whereas ICAM-1 expression is only 70% (34, 44, 62). In this situation, both E-selectin and CD11b/CD18-ICAM binding play quantitatively comparable and partly independent roles in adhesion (54, 55). After a 24-h stimulation, nearly all of the E-selectin has been shed from the endothelium and ICAM-1 expression has reached its maximum, conferring a major role to CD11/CD18-ICAM binding (54, 55). To discriminate between the interference of GXM with E-selectin binding to its counterligand on neutrophils and GXM interference with CD11b/CD18-ICAM binding, we studied the adhesion of GXM-treated neutrophils to HUVEC stimulated for either 6 or 24 h with TNF-α. The blocking MAb IB4 (anti-CD18) was used as a control to quantify the role of CD11/CD18-ICAM binding to endothelium stimulated with TNF-α for 6 or 24 h. Since the level of PMN binding was usually considerably lower with 24-h-stimulated endothelium than with 6-h-stimulated endothelium, the results of the two time points could not be averaged and were thus statistically analyzed separately.
In our experiments, the MAb against CD18 caused an 28% inhibition of adhesion to endothelium stimulated with TNF-α for 6 h (Fig. (Fig.5)5) (95% CI, 18 to 39%) and a 74% inhibition of adhesion to endothelium stimulated for 24 h (95% CI, 56 to 93%). The MAb against E-selectin caused a 44% inhibition (95% CI, 33 to 55%) after a 6-h stimulation and no statistically significant inhibition after a 24-h stimulation. These results confirm the earlier findings of researchers (54, 55). We observed that GXM-related inhibition of adhesion was more pronounced with endothelium stimulated for 6 h than with that stimulated for 24 h. After a 6-h stimulation the maximal detected inhibition was 35% (P = 0.001; 95% CI, 20 to 43%,), whereas after a 24-h stimulation no statistically significant inhibition was found (Fig. (Fig.5)5) (mean inhibition, 15%; P = 1), suggesting that the mechanism of inhibition might be based on the interference of E-selectin binding, not CD11/18-ICAM binding, to its counterligand.
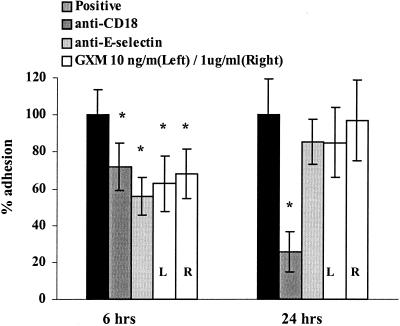
Inhibition of PMN adhesion to endothelium by GXM is stronger when endothelium is stimulated with TNF-α for 6 h than when it is stimulated for 24 h. HUVEC monolayers were stimulated with TNF-α for either 6 or 24 h and subsequently washed, and then either untreated or GXM-treated PMN were added and left for 15 min. To evaluate the relevance of both E-selectin- and CD18-mediated binding at either time point, the adhesion of PMN to endothelium was performed both after incubation of PMN with MAb IB4 (anti-CD18) and after incubation of stimulated HUVEC with MAb BBA2 (anti-E-selectin). Adhesion is expressed as the percentage of adhesion of untreated PMN (positive control) to 6- or 24-h-stimulated endothelium. The results are the averages from four experiments. , P < 0.05 (comparing adhesion to the positive control of either 6- or 24-h-stimulated HUVEC set at 100%). The error bars represent standard deviations.
To further investigate whether GXM interferes specifically with the binding of one of the involved adhesion molecules, we used blocking antibodies against each molecule. After GXM treatment, the PMN were incubated with designated MAbs (directed against L-selectin [DREG56], CD18 [IB4], or HLA A/B/C [control]). TNF-α-stimulated HUVEC were incubated with either a control MAb or a blocking MAb against E-selectin (BBA2) or ICAM-1 (RR1/1). Adhesion was performed in the presence of the antibodies. The control MAb against HLA did not affect adhesion (results not shown). As Fig. Fig.6A6A shows, blocking MAbs against the adhesion molecules CD18 (IB4), ICAM (RR1/1), L-selectin (DREG56), and E-selectin (BBA2) inhibited the adhesion of control PMN to stimulated endothelium by, respectively, 31% (95% CI, 14 to 46%), 20% (95% CI, 2 to 37%), 21% (95% CI, 10 to 38%), and 42% (95% CI, 32 to 52%) compared to the adhesion of control PMN in the absence of MAb.
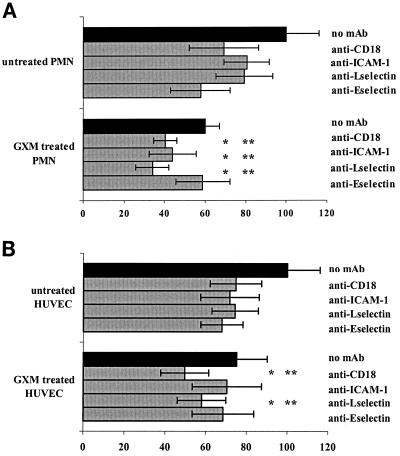
(A) Blocking antibodies against E-selectin do not have an additive inhibitory effect on the adhesion of PMN treated with GXM, while those against ICAM-1, CD18, and L-selectin do cause further inhibition of adhesion. PMN were treated with either medium or GXM at a concentration of 0.1 μg/ml for 1 h at 37°C. After washing, PMN were incubated with either medium or blocking MAb DREG 56 (anti-L-selectin) or IB4 (anti-CD18). HUVEC monolayers stimulated for 6 h with TNF-α were treated with either medium or blocking MAb BBA2 (anti-E-selectin) or RR1/1 (anti-ICAM-1). Adhesion of PMN to HUVEC occurred in the presence of the antibodies. Adhesion is expressed as the percentage of the adhesion of untreated PMN to stimulated endothelium (positive control), set at 100%. The results are averages from four experiments. , P < 0.05 compared to the inhibiting effect of GXM treatment only;
, P < 0.05 compared to the effect of MAb on adhesion of untreated PMN. The error bars represent standard deviations. (B) Effect of blocking MAbs against adhesion molecules on adhesion of PMN to stimulated endothelium pretreated with GXM. HUVEC were treated with either culture medium or GXM prior to stimulation with TNF-α (for another 6 h). After washing, the endothelium and PMN were incubated with various blocking MAbs for 20 min. Adhesion is expressed as the percentage of the value for the positive control (adhesion of PMN to TNF-α-stimulated endothelium). The results are the averages from five experiments.
, P < 0.05 compared to the inhibiting effect of GXM only;
, P < 0.05 compared to the effect of MAb only. The error bars represent standard deviations.
Treatment of PMN with GXM caused a 41% inhibition of adhesion (95% CI, 32 to 48%). MAbs IB4 (anti-CD18), RR1/1 (anti-ICAM-1), and DREG56 (anti-L-selectin) increased the inhibition of GXM-treated PMN to 60% (95% CI, 42 to 77%), 56% (95% CI, 38 to 74%), and 66% (95% CI, 59 to 73%), respectively. This further decrease in adhesion was statistically significant compared to GXM-related inhibition (adhesion of GXM-treated PMN to stimulated endothelium; P = 0.05, 0.003, and 0.005, respectively) and antibody-related inhibition (adhesion of non-GXM-treated PMN to stimulated endothelium in the presence of the antibodies; P = 0.03, 0.02, and 0.005, respectively) alone. In contrast, the blocking MAb against E-selectin (BBA2) was not capable of further decreasing the adhesion of GXM-treated PMN (inhibition caused by GXM, 40%; inhibition when BBA2 was added, 41% [95% CI, 37 to 46%; P = 0.65 for comparing both]). Similarly, the inhibition of adhesion caused by BBA2 and GXM together did not differ from that for treatment with the antibody alone (41 versus 42%; P = 0.9). Thus, GXM most likely interferes with the binding of PMN to endothelial E-selectin.
GXM treatment of the endothelium probably inhibits PMN adhesion by interfering with both ICAM-1 and E-selectin binding.
The mechanism underlying the decrease in adhesion caused by GXM treatment of the endothelium was evaluated by using blocking antibodies against adhesion molecules. Confluent HUVEC monolayers were treated with either medium or GXM for 1 h, followed by TNF-α stimulation for an additional 6 h. After washing, the endothelium and PMN were incubated with either blocking antibodies, control HLA antibodies, or medium. Again, the HLA-directed control MAbs did not affect adhesion (results not shown). Figure Figure6B6B shows that GXM treatment of the endothelium yielded a 25% inhibition (95% CI, 19 to 31%). The combined use of GXM and either MAb IB4 (anti-CD18) or DREG56 (anti-L-selectin) led to a stronger inhibition of adhesion (50 and 42%, respectively; P < 0.05) than the inhibition caused by either the MAbs (25% and 26%, respectively) or GXM (25%). These results indicate that the CD18 and L-selectin binding pathways are probably not affected by GXM. In contrast, the combined use of GXM and MAb RR1/1 (against ICAM-1) did not lead to a stronger inhibition of adhesion compared to treatment with either one separately (30 versus 25 and 28%, respectively; P = 0.5 and 0.8, respectively). This suggests that GXM might affect the endothelium by interfering with ICAM-1 binding pathways.
We also found that MAb BBA2 (anti-E-selectin) in combination with GXM did not increase inhibition compared to the inhibiting effect of either GXM or the MAb alone (32 versus 25% [P = 0.1] and 32% [P = 0.4], respectively). These results suggest that GXM treatment of endothelium also interferes with E-selectin binding.
DISCUSSION
The biological properties of the polysaccharides present in the capsule of C. neoformans that contribute to the pathogenesis of cryptococcosis include inhibition of phagocytosis, induction of immune unresponsiveness, cross-desensitization, alteration of cytokine production, enhanced infectivity of human immunodeficiency virus (59), and inhibition of neutrophil influx into potential sites of inflammation. (4, 15, 16). Although capsular GXM might not be the sole causative compound of the impaired leukocyte migration (other cryptococcus-derived products such as prostaglandins and mannoproteins may also contribute [10, 58]), it is certainly an important virulence factor (4). It has been hypothesized that the intrinsic chemoattracting properties of GXM, which circulates in the bloodstreams of infected individuals, might be responsible for the observed inhibition of leukocyte migration towards infected tissues (50). In this paper, we propose an additional mechanism leading to impaired transmigration, namely, the inhibition of neutrophil adhesion to the endothelium by GXM.
Although many microorganisms are capable of promoting adhesion by stimulating cytokine production and the expression of adhesion molecules (14, 22, 27, 35, 38, 39, 42) and some even use adhesion molecules on leukocytes and the endothelium for invasion (40), there are few examples of microorganisms that negatively affect the process of leukocyte adhesion to the endothelium (e.g., Leishmania, Porphyromonas gingivalis, E. coli, and Staphylococcus aureus) (11, 23, 53, 66). In this paper we show that cryptococcal GXM is able to inhibit adhesion of PMN to stimulated endothelium. All of our experiments showed that these inhibitory effects gradually diminish at higher concentrations, which has also been documented for other immunological effects of GXM (19). We have two possible explanations for this paradoxical result. First, we were able to demonstrate that this molecule tends to aggregate at higher concentrations, thereby possibly lowering the actual numbers of reactive molecules. Second, the phenomenon might be explained by the ability of GXM to activate neutrophils at higher concentrations (i.e., to cause L-selectin shedding and upregulation of CD11b/CD18) (10, 18). The latter explanation, however, could explain the effect of GXM on PMN only and not on endothelium.
We also demonstrated that GXM inhibits the adhesion of neutrophils to the endothelium by affecting both types of cells. Notably, the range of concentrations of GXM at which these effects were found is much lower (1 pg/ml to 10 μg/ml) than that described in the literature for other GXM effects (25 μg/ml to 1 mg/ml) (7, 12, 15, 18, 49, 57, 65). The lower concentration range is, however, more in line with physiological GXM levels. Although levels of up to several milligrams per milliliter have been measured in sera of AIDS patients, they are usually 100-fold lower in individuals who are not infected with human immunodeficiency virus (13, 24, 25). Interestingly, the optimal effect of GXM on PMN occurred at concentrations of 10 to 100 ng/ml, while such an effect on endothelium was found at much lower concentrations (1 pg/ml to 10 ng/ml). This suggests that there are different mechanisms underlying the inhibiting effects of GXM on either PMN or endothelial cells. The reported molecular mass of GXM varies between 10 and 1,400 kDa depending on the method of isolation and the degree of sonication (3, 9). Assuming, for calculation purposes, a molecular mass of 500 kDa for GXM, the average number of GXM molecules per endothelial cell would be three at a concentration of 1 pg/ml. This might indicate that GXM affects endothelial cells via some sort of signaling pathway rather than via a receptor or ligand blockade. We demonstrated that FITC-labeled GXM is able to bind the endothelium, endorsing the proposed mechanism. The absence of a cumulative inhibitory effect when treating both PMN and the endothelium with GXM suggests interference with the same binding pathway. The absence of a cumulative effect might also be explained by redundancy; the participation of certain adhesion pathways might increase when other pathways are blocked. Further research should allow discrimination between both possible explanations.
Since GXM is known to cause L-selectin shedding from the surface of PMN (18, 20), it seemed likely that this mechanism contributes to the inhibition of PMN adhesion to endothelium. We were able to prove, however, that the observed effects of GXM on PMN in the static adhesion model are not based on L-selectin shedding. First, we showed that blocking of GXM-related L-selectin shedding (by TAPI-0) does not abolish the GXM-related inhibition of PMN adhesion. This indicates that surface release of L-selectin is not involved in the GXM-related inhibition of adhesion. Second, we showed that blocking antibodies against L-selectin were still able to inhibit adhesion of GXM-treated PMN considerably. Both observations make it unlikely that L-selectin shedding is the principal mechanism underlying the GXM-related inhibition of adhesion.
In our experiments the average detected level of L-selectin shed from PMN by GXM was lower (8 ng/ml in the supernatant of 106 PMN) than previously described. Dong and Murphy detected 20 ng of L-selectin per ml, which accounted for 42% L-selectin loss (18). The ability of blocking anti-L-selectin antibodies to inhibit adhesion further after GXM treatment of PMN might therefore be explained by a higher percentage of L-selectin remaining on the surface of PMN after GXM treatment. Furthermore, although in the static adhesion assay L-selectin might serve as one of the several ligands of E-selectin, L-selectin binding is more important for the initial rolling of neutrophils on the endothelium than for the later phase of firm binding and transmigration (5, 28). This might be a second explanation for the absence of an effect of GXM-related L-selectin shedding on static adhesion.
To further unravel the mechanism underlying the GXM-related inhibition of adhesion, we analyzed the impact of blocking MAbs against different adhesion molecules on the inhibition of adhesion caused by GXM. We were able to show that the effect of GXM on both PMN and the endothelium probably involves interference with the E-selectin binding pathway, since blocking antibodies against E-selectin did not cause an increase of the GXM-related inhibition of adhesion after either treatment. It has been shown that E-selectin is expressed on stimulated endothelium (34, 44, 56, 62) and that it binds preferentially to sialyl Lewis X carbohydrate structures expressed on the surface of PMN (29). A few of these ligands have been identified, namely, ESL-1 (45), PSGL-1 (the ligand for P-selectin) (2), and L-selectin (41, 68). We also know that E-selectin binding is involved in both the initial phase of leukocyte rolling (5) and firm binding, the latter by contributing to leukocyte activation (5, 47, 52, 56). The interference of GXM with these binding pathways might, therefore, impair both phases of neutrophil adhesion, thus contributing to the observed diminution of leukocyte migration toward the inflamed tissues in cryptococcal infections. Since we already showed that L-selectin binding is not inhibited by GXM, it remains to be investigated which of the other known ligands of E-selectin is affected by GXM (i.e., ESL-1 or PSGL-1). Since relatively short incubation periods with GXM are required to attain its inhibitory effects, impairment of protein expression seems unlikely. GXM might bind to or shed E-selectin receptors on PMN. As noted earlier, GXM causes PMN to shed L-selectin. Our experiments, however, show that this phenomenon does not underlie the observed effects of GXM on PMN. Dong and Murphy showed that GXM does not affect the expression of CD15, a Lewis X carbohydrate antigen, negatively on the surface of PMN (18). The effects on other E-selectin specific ligands remain to be investigated.
Interestingly, Dong and Murphy showed that GXM is able to bind to PMN and even binds specifically to CD18 on PMN, and not to L-selectin, CD11a, or CD11b (19). In our adhesion experiments, however, CD18-related binding seems not to be impaired by GXM.
Furthermore, by using blocking antibodies, we showed that GXM probably also inhibits E-selectin-mediated adhesion by affecting the endothelium, thus possibly affecting E-selectin itself. We further demonstrated that treatment of the endothelium with GXM may also affect ICAM-1, a ligand of CD11/CD18, expressed on the endothelium. Interference with CD18 binding might therefore be expected. Our experiments, however, did not show that GXM interferes with the binding of CD11/CD18 to the endothelium, since antibodies against CD18 were still able to significantly inhibit adhesion to GXM-treated endothelium. CD11/CD18, which usually binds preferentially to ICAM-1, might bind to other ligands (e.g., ICAM-2) when binding to ICAM-1 is impaired (30), since much overlap and redundancy exist in the binding of integrins to a large variety of ligands (1).
We are currently studying the effects of GXM on the separate ligands on PMN and endothelium involved in E-selectin binding as well as its effect on ICAM-1.

TAPI-0, a blocker of L-selectin shedding, does not affect GXM-related inhibition of PMN adhesion. PMN were incubated with either buffer or 50 μg of TAPI-0 per ml at 37°C for 15 min, after which GXM was added for a 1-h incubation. Adhesion to TNF-α-stimulated HUVEC occurred in the presence of both GXM and TAPI-0. The results are the averages from three experiments. Adhesion is expressed as the percentage of the value for the positive control (i.e., adhesion of untreated PMN to stimulated endothelium = 100%). The error bars represent standard deviations.
Acknowledgments
We thank P. G de Groot and Glenda J. Heijnen-Snyder from the Department of Hematology, University Medical Centre Utrecht, Utrecht, The Netherlands, for their contributions.
Notes
Editor: B. B. Finlay
REFERENCES
Articles from Infection and Immunity are provided here courtesy of American Society for Microbiology (ASM)
Full text links
Read article at publisher's site: https://doi.org/10.1128/iai.70.9.4762-4771.2002
Read article for free, from open access legal sources, via Unpaywall:
https://europepmc.org/articles/pmc128235?pdf=render
Citations & impact
Impact metrics
Citations of article over time
Alternative metrics
Smart citations by scite.ai
Explore citation contexts and check if this article has been
supported or disputed.
https://scite.ai/reports/10.1128/iai.70.9.4762-4771.2002
Article citations
Inhibition of RhoA Prevents Cryptococcus neoformans Capsule Glucuronoxylomannan-Stimulated Brain Endothelial Barrier Disruption.
J Infect Dis, 230(4):1042-1051, 01 Oct 2024
Cited by: 0 articles | PMID: 38622836
Glucuronoxylomannan intranasal challenge prior to Cryptococcus neoformans pulmonary infection enhances cerebral cryptococcosis in rodents.
PLoS Pathog, 19(4):e1010941, 28 Apr 2023
Cited by: 5 articles | PMID: 37115795 | PMCID: PMC10171644
Clinical and pathological characterization of Central Nervous System cryptococcosis in an experimental mouse model of stereotaxic intracerebral infection.
PLoS Negl Trop Dis, 17(1):e0011068, 19 Jan 2023
Cited by: 7 articles | PMID: 36656900 | PMCID: PMC9888703
Cell Wall Integrity Pathway Involved in Morphogenesis, Virulence and Antifungal Susceptibility in Cryptococcus neoformans.
J Fungi (Basel), 7(10):831, 05 Oct 2021
Cited by: 9 articles | PMID: 34682253 | PMCID: PMC8540506
Review Free full text in Europe PMC
Proteinase 3 contributes to endothelial dysfunction in an experimental model of sepsis.
Exp Biol Med (Maywood), 246(21):2338-2345, 22 Jul 2021
Cited by: 3 articles | PMID: 34292081 | PMCID: PMC8581824
Go to all (23) article citations
Similar Articles
To arrive at the top five similar articles we use a word-weighted algorithm to compare words from the Title and Abstract of each citation.
Cryptococcal polysaccharides bind to CD18 on human neutrophils.
Infect Immun, 65(2):557-563, 01 Feb 1997
Cited by: 68 articles | PMID: 9009313 | PMCID: PMC176096
Cryptococcal glucuronoxylomannan interferes with neutrophil rolling on the endothelium.
Cell Microbiol, 6(6):581-592, 01 Jun 2004
Cited by: 24 articles | PMID: 15104598
Cryptococcal polysaccharides induce L-selectin shedding and tumor necrosis factor receptor loss from the surface of human neutrophils.
J Clin Invest, 97(3):689-698, 01 Feb 1996
Cited by: 61 articles | PMID: 8609224 | PMCID: PMC507105