Abstract
Free full text

Resistance to Pentamidine in Leishmania mexicana Involves Exclusion of the Drug from the Mitochondrion
Abstract
The uptake of [3H]pentamidine into wild-type and drug-resistant strains of Leishmania mexicana was compared. Uptake was carrier mediated. Pentamidine-resistant parasites showed cross-resistance to other toxic diamidine derivatives. A substantial decrease in accumulation of the drug accompanied the resistance phenotype, although the apparent affinity for pentamidine by its carrier was not altered when initial uptake velocity was measured. The apparent Vmax, however, was reduced. An efflux of pentamidine could be measured in both wild-type and resistant cells. Only a relatively small proportion of the total accumulated pentamidine was available for efflux in wild-type cells, while in resistant cells the majority of loaded pentamidine was available for release. Pharmacological reagents which diminish the mitochondrial membrane potential reduced pentamidine uptake in wild-type parasites, and the mitochondrial membrane potential was shown to be reduced in resistant cells. A fluorescent analogue of pentamidine, 4′,6′-diamidino-2-phenylindole, accumulated in the kinetoplast of wild-type but not resistant parasites. These data together indicate that diamidine drugs accumulate in the Leishmania mitochondrion and that the development of the resistance phenotype is accompanied by lack of mitochondrial accumulation of the drug and its exclusion from the parasites.
Leishmania mexicana, a flagellated protozoan parasite, is the causative agent of localized and diffuse cutaneous leishmaniasis. Sandflies transmit promastigote forms of the parasite to the mammalian host, where they invade macrophages and transform into amastigotes. Pentavalent antimonial drugs are the standard first-line treatment for leishmaniasis (1, 18), although resistance is a growing problem (42, 44, 56, 57). The aromatic diamidine pentamidine, initially developed for use against Trypanosoma brucei, is considered an alternative to pentavalent antimony in treatment of leishmaniasis (2, 14, 52, 55).
Previous work on pentamidine uptake has indicated that the drug is accumulated by saturable, transport-mediated processes in African trypanosomes (23, 27-29) and in Leishmania spp. (7, 17). The P2 adenosine transporter of Trypanosoma brucei (23) appears to play some part in the transport of this drug, although the situation is complex (24, 47), with at least two additional transporters also capable of carrying the drug (29). In Leishmania donovani, pentamidine was found to be a competitive inhibitor of arginine transport (40, 41) and a noncompetitive inhibitor of putrescine and spermidine transport in Leishmania infantum (51), L. donovani, and L. mexicana (12). With the exception of the P2 nucleoside transporter in T. brucei, the physiological roles of the carrier proteins which accumulate pentamidine have yet to be identified.
Resistance to pentamidine has been described for trypanosomes (15, 16), L. donovani (42, 56, 59), and other Leishmania species (8-11, 32, 53, 54). The mechanism(s) of resistance in trypanosomatids is poorly understood. However, yeasts are also susceptible to pentamidine (45), and a gene called PNT1, when overexpressed, confers resistance to the drug in Saccharomyces cerevisiae (46). The protein encoded by this gene localizes to the inner membrane of the mitochondrion and appears to play a role in secretion of proteins from the mitochondrion (36). Disruption of mitochondrial function has been proposed as a likely toxic effect of pentamidine in S. cerevisiae (36, 47). The mitochondrion has also been implicated in the action of pentamidine against trypanosomatids. Electron microscopy revealed that treatment of Leishmania spp. with pentamidine leads to disintegration of the kinetoplast and mitochondrion (26, 37), and a collapse in mitochondrial membrane potential (58) is one of the first manifestations of treatment of these parasites with the drug.
Mechanisms of drug resistance have been extensively studied in trypanosomatid parasites (19). In African trypanosomes, uptake of diamidines, including pentamidine (22) and berenil, appears to involve the P2 nucleoside transporter (23), and loss of this transporter can mediate resistance to diamidines (5, 6, 22). However, P2-deficient T. brucei strains remain sensitive to pentamidine, probably because this diamidine continues to accumulate through alternative transporters (29).
The aim of the present work was to study how the accumulation of pentamidine in L. mexicana and its efflux relate to pentamidine resistance in these parasites.
MATERIALS AND METHODS
Materials.
Pentamidine isethionate was a generous gift from Roger Bellon, Rhône Poulenc Laboratories, Neuilly sur Seine, France. [Ring-3H]pentamidine (98 Ci/mmol, 5 mCi/ml) was custom synthesized by Amersham Pharmacia Biotech (Buckinghamshire, United Kingdom) from dibromopentamidine as described previously (15). l-[U-14C]arginine (313 mCi/mmol) was purchased from Dupont NEN (Herts, United Kingdom). All other compounds were purchased from Sigma (Gillingham, United Kingdom). Depending on solubility, compounds were dissolved in phosphate-buffered saline (PBS), water, ethanol, or dimethyl sulfoxide.
Strains and culture conditions.
L. mexicana (MNYC/BZ/62/M379) promastigotes were grown in vitro at 25°C in HOMEM medium supplemented with 10% (vol/vol) heat-inactivated fetal calf serum (35). L. mexicana (MNYC/BZ/62/M379) amastigotes were grown at pH 5.5 in Schneider's Drosophila medium (Gibco) supplemented with 20% (vol/vol) heat-inactivated fetal calf serum and gentamicin sulfate at 25 μg/ml and 32°C (13). L. mexicana promastigotes resistant to pentamidine were obtained in vitro from sensitive L. mexicana cells by stepwise exposure to the drug with final concentrations of 2.5 μM, 5 μM, 10 μM, and 30 μM until they had no effect on parasite growth, as previously described for other Leishmania species (9). L. mexicana amastigotes resistant to pentamidine were obtained axenically by transformation of promastigotes resistant to 30 μM pentamidine at 32°C without drug pressure (13). Once transformed, parasites were cultivated with 30 μM pentamidine. The stability of pentamidine resistance in vitro was determined as described previously (9).
Cross-resistance to other drugs was determined by measuring the 50% inhibitory concentration (IC50) and the resistance factor (IC50 ratio between resistant and wild-type cells) with the colorimetric MTT dye reduction assay (48), in which the tetrazolium salt MTT is converted to a colored formazan product by enzymes active only in living cells. The IC50 value corresponds to the concentration of a given compound provoking 50% inhibition of cell proliferation compared with untreated cells after 3 days. The effect on pentamidine resistance of 5 μM trifluoperazine (TFP), 5 μM prochlorperazine (PCP), 15 μM verapamil, and 1 mM buthionine sulfoximine (BSO) was studied by incubating the parasites with these compounds and different concentrations of pentamidine for 72 h, with the inhibitory effect on growth being measured as described above.
Transport studies.
Parasites were harvested during the mid-logarithmic phase of growth by centrifugation at 2,100 × g for 10 min at room temperature. Cells were then washed three times with phosphate-buffered saline supplemented with 1% d-glucose (PBSG) at pH 7.4 for promastigotes and pH 5.5 for amastigotes. Cells were resuspended in this buffer at densities appropriate for the procedure to be used, as indicated in the text and figure legends. Parasite suspensions (160 μl, containing 2 × 107 cells for promastigotes and 3 × 107 cells for amastigotes) were warmed to 25°C (for promastigotes) or 32°C (for amastigotes) and mixed with 20 μl of assay buffer containing labeled molecule plus or minus other test compounds at the concentrations indicated in the text. Transport was terminated by the rapid separation of parasites from the buffer components by centrifugation through a 9:1 mixture of dibutyl phthalate (specific gravity, 1.04) and mineral oil (specific gravity, 0.875 to 0.885). The sample tubes were immediately flash frozen in liquid nitrogen, and the tubes were cut to separate the pellet from the transport medium. The pellet was dissolved in 2% sodium dodecyl sulfate (250 μl) and 3 ml of scintillation fluid (EcoscintA; National Diagnostics, Hessle, United Kingdom). These were left overnight to remove the effects of chemiluminescence, and then incorporated radioactivity was counted. The protein content was determined by the dye-binding method (21) and calculated as 6.1 and 2.0 μg of protein/106 cells for L. mexicana promastigotes and amastigotes, respectively. Cell volumes were previously determined as 100 μm3 for promastigotes and 27 μm3 for amastigotes (17).
The effects of various pharmacological reagents on pentamidine accumulation in wild-type and resistant cells was investigated by resuspending cells in PBSG containing inhibitors at the concentrations indicated in the text and figure legends for 10 min at 25°C. After this treatment, 0.25 μM [3H]pentamidine was added to the mixture, and uptake over 10 s was measured.
In order to determine efflux of pentamidine, cells were loaded with 0.25 μM [3H]pentamidine for 10 min either at room temperature or on ice. After exposure to the drug, an aliquot was immediately centrifuged through oil and frozen in liquid nitrogen. Retained pentamidine was determined as described above. The remaining cells were centrifuged, washed three times with PBSG, resuspended in drug-free PBSG at 108 cells/ml, and incubated at 25°C or 32°C. Aliquots of cells were removed, and intracellular pentamidine was determined over a range of time points. To evaluate the effect of energy, mitochondrial membrane potential, proton motive forces, and multidrug resistance-reversing agents on pentamidine efflux, inhibitors were added simultaneously with the [3H]pentamidine. Subsequently, cells were centrifuged, washed three times with PBSG containing inhibitors, and then resuspended in PBSG supplemented with the inhibitor but no pentamidine.
Analysis of kinetic data.
The net uptake of pentamidine into leishmanias probably involves several components, including a plasma membrane uptake system, a plasma membrane efflux system, and a mitochondrial uptake system. Technical limitations prevented us from dissecting out each of these components, and hence we measured net uptake and expressed the values from plots of net uptake against velocity as apparent kinetic parameters. Apparent Km and Vmax values for pentamidine uptake were determined by measuring apparent initial uptake rates over various concentrations of pentamidine between 1 μM and 1 mM. Nonlinear regression fits to the Michaelis-Menten equation with the GraFit version 4.09 for Microsoft Windows (Erithacus Software) were used to determine apparent Km and Vmax values. The type of inhibition and Ki values for pentamidine, arginine, and other molecules were determined with the enzyme inhibition program of GraFit 4.09.
Fluorescence microscopy.
Cells were incubated with 2 μM 4′,6′-diamidino-2-phenylindole (DAPI) for the times marked in the text. Cells were then transferred to a microscope slide and viewed by fluorescence microscopy (excitation at 330 nm, emission at 400 nm). To permeabilize the cells, 1 μM digitonin was added to the cells for 1 to 2 min before DAPI was added.
Flow cytometry.
A total of 106 parasites were washed three times and resuspended in 1 ml of PBSG containing 250 nM rhodamine-123. The fluorescence of 105 cells was measured immediately after addition and 30 min later. The procedure was carried out for wild-type and resistant parasites and repeated in the presence of 10 mM pentamidine. Data were gathered with a FACSCalibur (Becton Dickinson, Oxford, United Kingdom) flow cytometer equipped with an argon-ion laser (15 mW) tuned to 488 nm. Rhodamine-123 fluorescence was collected in the photomultiplier tube designated FL1, which is equipped with a 530/30-nm band pass filter. Data analysis was carried out with CellQuest (Becton Dickinson, Oxford, United Kingdom) software.
RESULTS
Characterization of pentamidine-resistant parasites.
Four cloned lines of L. mexicana promastigotes resistant to pentamidine (Pentr) were selected in vitro by increasing drug pressure. Pentr2.5, Pentr5, Pentr10, and Pentr30 promastigotes displayed 14-, 30-, 50-, and 100-fold resistance to pentamidine, respectively (Table (Table1).1). These resistant cells expressed cross-resistance to the diamidines propamidine, stilbamidine, and berenil. Resistant promastigotes did not show any cross-resistance to sodium arsenite or to inhibitors of multidrug resistance and multidrug resistance-associated protein, which are toxic to cells in the assay conditions used (Table (Table11).
TABLE 1.
Cross-resistance profile of wild-type and pentamidine-resistant L. mexicana promastigotesa
Drug | Mean IC50 (μM) ± SEM (n = 4)
| ||||
---|---|---|---|---|---|
Wild type | Pentr2.5 | Pentr5 | Pentr10 | Pentr30 | |
Pentamidine | 0.5 ± 0.1 | 7 ± 2 | 15 ± 3 | 25 ± 2 | 50 ± 5 |
Propamidine | 10 ± 2 | 25 ± 6 | 42 ± 5 | 64 ± 7 | 120 ± 7 |
Berenil | 1.2 ± 0.1 | 4.0 ± 0.5 | 11 ± 2 | 18 ± 4 | 45 ± 3 |
Stilbamidine | 9 ± 3 | 21 ± 4 | 45 ± 4 | 90 ± 5 | 161 ± 8 |
DAPI | 3.1 ± 0.4 | ND | ND | ND | 49 ± 6 |
Promastigotes of the resistant line Pentr30 were transformed in vitro to amastigotes without drug pressure because pentamidine inhibits the transformation of promastigotes to amastigotes (54). It has previously been shown that while low-level resistance to pentamidine in L. mexicana is rapidly lost once drug selection is removed, high-level resistance is stable (54). Here we too show that high-level pentamidine resistance was maintained for at least 6 months without pressure for promastigotes and 2 months for amastigotes (data not shown). The IC50 of pentamidine for amastigote derivatives of Pentr30 was 70 ± 5 μM, compared with 0.30 ± 0.05 μM for sensitive amastigotes.
The ability of typical P-glycoprotein inhibitors previously used to identify at least three separate efflux activities in Leishmania spp. (30) were tested for their ability to reverse pentamidine resistance. TFP (5 μM), PCP (5 μM), and verapamil (15 μM) had no significant toxic effects on either wild-type or resistant promastigotes themselves, nor did BSO (1 mM), which inhibits glutathione biosynthesis (data not shown). However, at these concentrations, each compound provoked a reversion towards wild-type pentamidine sensitivity. The IC50s for L. mexicana promastigotes of the Pentr30 strain were 50 ± 5 μM for pentamidine, 3.3 ± 0.9 μM for pentamidine plus 5 μM TFP, 4.0 ± 0.8 μM for pentamidine plus 5 μM PCP, 5.1 ± 0.9 μM for pentamidine plus 15 μM verapamil, and 3.7 ± 0.04 μM for pentamidine plus 1 mM BSO (mean ± standard error of the mean, n = 4).
Pentamidine uptake by wild-type and resistant parasites.
Uptake of 0.25 μM pentamidine was measured over 3 h in pentamidine-resistant L. mexicana and compared with that in wild-type cells. The four pentamidine-resistant promastigote strains showed a reduced accumulation of drug in comparison with sensitive cells, with all of the resistant lines accumulating very little (Fig. (Fig.1).1). After 3 h of incubation, the intracellular pentamidine concentration was 10 μM for Pentr2.5, 9 μM for Pentr5, 7 μM for Pentr10, and 5 μM for Pentr30, compared with 55 μM in the wild-type cell line. Similar results were obtained with amastigotes, where an intracellular concentration of 35 μM was obtained for the resistant line Pentr30 and 230 μM for the sensitive amastigotes (data not shown).
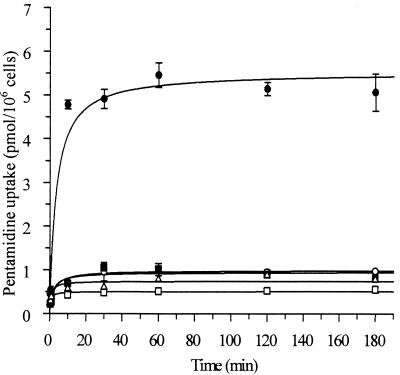
Time course of pentamidine uptake by wild-type and pentamidine-resistant L. mexicana promastigotes. Parasites were incubated with 0.25 μM pentamidine at 25°C, and pentamidine uptake was measured between 5 s and 3 h. Wild-type (solid circles) and resistant Pentr2.5 (solid squares), Pentr5 (open circles), Pentr10 (open triangles), and Pentr30 (open square) strains were tested. Results are the mean ± standard error of the mean of at least three experiments.
Apparent kinetic constants for pentamidine uptake into both resistant promastigotes and amastigotes were determined with a range of substrate concentrations between 1 μM and 1 mM and compared with values obtained for wild-type cells (Table (Table2).2). The apparent Km value was similar for both wild-type amastigotes and promastigotes. The apparent Vmax value was, however, 19-fold higher in amastigotes than in promastigotes (Table (Table2).2). Apparent Km values for pentamidine in resistant cells were similar to the mean values obtained for wild-type cells. Apparent Vmax values, however, were substantially lower in resistant cells and inversely correlated with the degree of resistance. The apparent Vmax value of Pentr30 amastigotes was 17-fold lower than the value obtained for wild-type cells.
TABLE 2.
Kinetic constants of pentamidine uptake by wild-type and pentamidine-resistant L. mexicana strains
Cells | Mean apparent Km (μM) ± SEM (n = 4) | Mean apparent Vmax (fmol/s/μg of protein) ± SEM (n = 4) |
---|---|---|
Promastigotes | ||
![]() ![]() ![]() ![]() | 7.4 ± 1.8 | 452 ± 42 |
![]() ![]() ![]() ![]() | 6.2 ± 2.4 | 196 ± 23 |
![]() ![]() ![]() ![]() | 5.8 ± 1.6 | 148 ± 12 |
![]() ![]() ![]() ![]() | 5.4 ± 0.6 | 68 ± 3 |
![]() ![]() ![]() ![]() | 5.9 ± 2.1 | 57 ± 5 |
Amastigotes | ||
![]() ![]() ![]() ![]() | 13.9 ± 2.9 | 8,540 ± 726 |
![]() ![]() ![]() ![]() | 13.2 ± 5.6 | 491 ± 85 |
Effects of pharmacological inhibitors of cellular homeostasis.
Pentamidine uptake was investigated in the presence and absence of different inhibitors to investigate the nature of the force driving uptake. The results indicate that classical inhibitors of mitochondrial metabolism, potassium cyanide, sodium azide, and 2,4-dinitrophenol (Table (Table3;3; data not shown for amastigotes), led to significant reductions in net pentamidine uptake in wild-type parasites. Ouabain, an inhibitor of the plasma membrane Na+/K+-ATPase (31), and monensin, an Na+ ionophore, did not significantly decrease pentamidine uptake in either promastigotes or amastigotes. These data indicate that pentamidine uptake is independent of Na+ ions. The K+ ionophore valinomycin and the protonophore carbonyl cyanide (33, 34) reduced pentamidine uptake.
TABLE 3.
Relative accumulation of pentamidine in wild-type and pentamidine-resistant L. mexicana promastigotes in the absence and presence of different inhibitorsa
Inhibitor (concn) | Mean % of wild-type control accumulation ± SEM (n = 4)
| |
---|---|---|
Wild type | Pentr50 | |
None (control) | 100 | 16 ± 4 |
KCN (1 mM) | 35 ± 6 | 27 ± 3 |
2,4-Dinitrophenol (2 mM) | 30 ± 7 | 25 ± 5 |
Azide (5 mM) | 29 ± 3 | 30 ± 2 |
Monensin (50 μM) | 86 ± 8 | 14 ± 3 |
Ouabain (1 mM) | 88 ± 4 | 14 ± 5 |
Valinomycin (10 μM) | 52 ± 5 | 23 ± 4 |
Carbonyl cyanide (10 μM) | 37 ± 2 | 22 ± 3 |
N, N′-Dicyclohexylcarbodiimide (50 μM) | 29 ± 3 | 20 ± 2 |
Oligomycin A (12 μg/ml) | 44 ± 4 | 32 ± 5 |
N-Ethylmaleimide (1 mM) | 48 ± 4 | 21 ± 2 |
Orthovanadate (10 μM) | 57 ± 4 | 17 ± 2 |
Bafilomicin A1 (1 μM) | 103 ± 2 | 14 ± 3 |
Verapamil (50 μM) | 32 ± 5 | 20 ± 3 |
The nonspecific H+-ATPase inhibitor N,N′-dicyclohexylcarbodiimide, which reduced the plasma membrane pH gradient and the membrane potential in Leishmania spp. (33, 34), oligomycin A, a mitochondrial H+-ATPase inhibitor (3), and N-ethylmaleimide all had inhibitory effects on pentamidine uptake. Orthovanadate, a plasma membrane-type H+-ATPase inhibitor (61), also decreased pentamidine uptake, whereas bafilomycin A1, a specific vacuolar-type H+-ATPase inhibitor (20), had no effect. Verapamil, a Ca2+ channel blocker and a P-glycoprotein inhibitor, inhibited pentamidine uptake. Overall, while the agents used could affect the permeability of cells independently of their effect on the proton motive force, the data are consistent with inhibition of proton motive forces associated with mitochondrial metabolism having a profound effect on the net accumulation of pentamidine into L. mexicana.
Uptake in the presence of these inhibitors was also studied in Pentr30 promastigotes. Net pentamidine uptake in the resistant line was increased in the presence of dinitrophenol, potassium cyanide, and sodium azide compared with uptake into the resistant cells themselves (Table (Table3).3). Valinomycin, carbonyl cyanide, N,N′-dicyclohexylcarbodiimide, N-ethylmaleimide, oligomycin A, and verapamil also increased pentamidine accumulation in resistant cells. These results indicate that the mitochondrial membrane potential is not involved in the accumulation of pentamidine in resistant parasites. Moreover, since most of these inhibitors also deenergize cells, they also indicate that the drug may be actively effluxed from energized cells.
Specificity of pentamidine transporter.
Pentamidine was shown to be a competitive inhibitor of arginine uptake for promastigotes and amastigotes, with Ki values of 12.5 ±3.8 μM and 16.3 ±2.8 μM, respectively. However, arginine had no inhibitory activity against pentamidine uptake, even at concentrations as high as 10 mM (data not shown). Km and Vmax values of arginine uptake were essentially identical in wild-type and pentamidine-resistant parasites (Km values were 2.3 ± 0.7 μM for the wild-type and 3.3 ± 2.7 μM for the resistant line, and Vmax values were 6.6 ± 0.5 nmol s−1 [106 cells]−1 and 5.1 ± 0.6 nmol s−1 [106 cells]−1 for the two lines, respectively). This would indicate that pentamidine does not enter cells via an arginine transporter.
The aromatic diamidines propamidine, stilbamidine, DAPI, and berenil and the phenanthridine isometamidium (Samorin) were found to be potent competitive inhibitors of pentamidine uptake, suggesting that the nature of the lateral chain is not important in recognition (Table (Table4).4). para-Aminobenzoic acid, benzamide, and benzylamine did not affect pentamidine uptake, whereas benzamidine, 3-aminobenzamidine, and 4-aminobenzamidine all inhibited uptake, but noncompetitively.
TABLE 4.
Effect of different aromatic diamidines on growth and pentamidine uptake by L. mexicana promastigotesa
Drug | Mean uptake (% of control) ± SEM (n = 4) | Mean Ki (μM) ± SEM (n = 4) |
---|---|---|
Pentamidine | 25 ± 3 | 7.5 ± 0.7 |
Propamidine | 26 ± 5 | 12.4 ± 1.2 |
Stilbamidine | 40 ± 7 | 21 ± 5 |
Berenil | 35 ± 4 | 27 ± 3 |
DAPI | 32 ± 3 | ND |
Isometamidium | 25 ± 2 | 8 ± 1 |
Benzamidine | 84 ± 17 | 252 ± 23 |
3-Aminobenzamidine | 75 ± 15 | 180 ± 20 |
4-Aminobenzamidine | 70 ± 11 | 173 ± 11 |
In order to establish the specificity of the transporter, uptake of 4 μM pentamidine was studied in the presence of a vast excess (1,000-fold or more) of potential competitive substrates. Polyamines (putrescine, spermidine, and spermine), amino acids (all 20 naturally occurring l-amino acids), nucleobases (adenine, guanine, cytosine, and uracil), nucleosides (adenosine, inosine, guanosine, thymidine, and cytidine), sugars (d-glucose, d-fructose, and d-galactose), and a variety of other metabolites (folic acid, biotin, choline, panthenoic acid, ethanolamine, thiamine, pterine, pyridoxal, taurine, linoleic acid, sphingosine, guanidine, urea, reduced glutathione, oxidized glutathione, ascorbic acid, and imidazole) all failed to inhibit pentamidine uptake. Two peptides rich in basic amino acids (Arg-Ser-Arg and Lys-Lys-Lys-Lys) did give modest inhibition (around 50% when used at 5 mM and 10 mM, respectively).
Inhibition was also seen with some inorganic divalent cations (74% inhibition with 5 mM Ca2+, 47% with 0.1 mM Cd2+, 45% with 0.1 mM Co2+, and 69% with 5 mM Ni2+). Taurocholate also decreased pentamidine uptake by 50% when used at 10 mM. Taurocholate was a noncompetitive inhibitor, with a Ki of 10.5 ± 1.9 mM (data not shown). Pentamidine uptake was sensitive to several compounds often used as P-glycoprotein inhibitors, including TFP, PCP, and verapamil (the three drugs were competitive inhibitors, with a Ki of 10.70 ± 0.04 μM for TFP, 8.01 ± 0.09 μM for PCP, and 53.2 ± 8.1 μM for verapamil [data not shown]).
Efflux of pentamidine.
Examining pentamidine uptake in the presence of metabolic inhibitors, including dinitrophenol, azide, and KCN, revealed significant decreases in accumulated drug in the wild-type parasites. However, these compounds increased net uptake in resistant cells, indicating that an energy-dependent efflux system for pentamidine could influence the resistance phenotype. This is in agreement with the observation that P-glycoprotein inhibitors reverse the resistance (Table (Table33).
To study this more, parasites were loaded to steady state over 10 min with radiolabeled pentamidine at room temperature, and retained drug was measured for 1 h in both wild-type and resistant cells. Accumulated pentamidine reached substantially higher levels in wild-type parasites during the loading phase. An efflux of pentamidine was then observed in both wild-type and resistant cells. This represented a significant proportion (about 70%) of total accumulated drug in resistant parasites but only a small fraction (about 14%) of the total in wild-type cells (Fig. (Fig.2A2A).
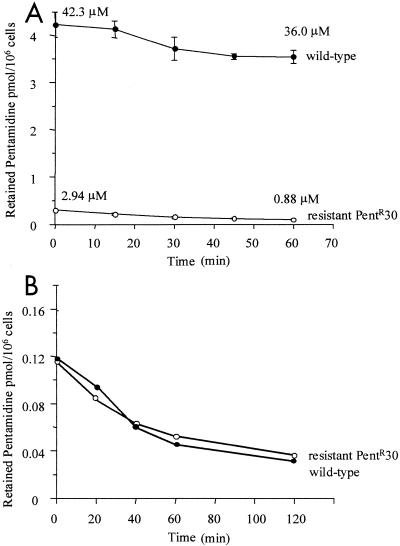
Efflux of pentamidine by L. mexicana promastigotes. Wild-type and pentamidine-resistant parasites were incubated with 0.25 μM pentamidine at 25°C (A) or on ice (B) for 10 min, centrifuged, washed three times with PBSG, and resuspended in drug-free PBSG. Retained pentamidine was measured between 1 and 60 min. Results are the mean ± standard error of the mean of at least three experiments.
When cells were loaded with pentamidine on ice, both wild-type and resistant lines accumulated similar levels. When these were then transferred to room temperature and the rate of efflux was measured in both cell lines, it was shown to occur at a similar rate (Fig. (Fig.2B),2B), indicating that increased efflux could not explain the resistance phenotype.
Pentamidine efflux was also assayed in the presence of various metabolic inhibitors and protonophores, and this also diminished efflux (Fig. (Fig.3).3). Typical inhibitors of the multidrug resistance phenotype in mammalian cells which have also been used to distinguish at least three efflux systems in Leishmania spp. (30), including verapamil (used at 50 μM), TFP, and PCP (both used at 20 μM), inhibited pentamidine efflux (Fig. (Fig.33 and and4).4). Treatment of the cells with 1 mM BSO for 24 h also inhibited efflux (Fig. (Fig.44).
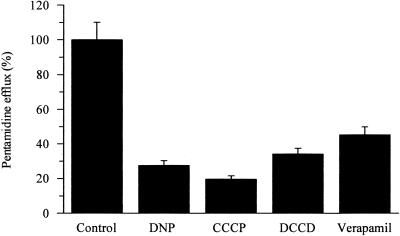
Pentamidine efflux by pentamidine-resistant promastigotes under energy- and proton-depleting conditions. Retained pentamidine was measured after 30 min in the absence (control) and presence of inhibitors: 1 mM dinitrophenol (DNP), 10 μM carbonyl cyanide (CCCP), 50 μM N,N′-dicyclohexylcarbodiimide (DCCD), and 50 μM verapamil. Results are the mean ± standard error of the mean of at least three experiments.
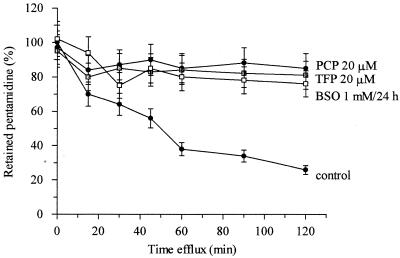
Inhibition effects of different multidrug resistance-associated protein inhibitors on pentamidine efflux by pentamidine-resistant promastigotes. Time course of pentamidine efflux was measured in the presence and absence of 20 μM PCP and 20 μM TFP and after 24 h of treatment with 1 mM BSO. Results are the mean ± standard error of the mean of at least four experiments.
Pentamidine accumulation over a 6-h period was also investigated in wild-type and resistant promastigotes with or without PCP and TFP. In the presence of these inhibitors, resistant cells accumulated drug to level similar to those in the wild type by 6 h (Fig. (Fig.55).
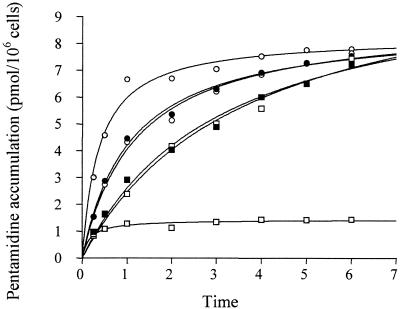
Effect of multidrug resistance-associated protein inhibitors on time course of pentamidine accumulation by wild-type and pentamidine-resistant promastigotes. The effect of 10 μM PCP and 10 μM TFP in sensitive wild-type cells and 20 μM PCP and 20 μM TFP in resistant cells on pentamidine influx was studied. The wild type (shaded circles), wild type plus 10 μM PCP (solid circles), wild type plus 10 μM TFP (open circles), resistant line Pentr30 (shaded squares), resistant line Pentr30 plus 20 μM PCP (solid squares), and resistant line Pentr30 plus 20 μM TFP (open squares) were tested. Results are means of at least three experiments.
Intracellular compartmentalization of diamidines.
Pentamidine-resistant promastigotes (Pentr30) were treated with 1 μM digitonin to permeabilize membranes without killing the cells in order to bypass membrane transport systems. Accumulation of pentamidine was similar in wild-type and resistant cells under these conditions (data not shown), while without digitonin the apparent rate of pentamidine uptake by resistant cells was only 10% of that of the sensitive cells (Fig. (Fig.11).
DAPI is a fluorescent analogue of pentamidine. The compound is toxic to L. mexicana promastigotes, and cells resistant to pentamidine are cross-resistant to DAPI (Table (Table1).1). Moreover, the compound inhibits pentamidine uptake into L. mexicana (Table (Table4),4), indicating that the two compounds share a route of internalization. Distribution of DAPI (2 μM) was analyzed by adding it to cells and viewing the cells under fluorescence microscopy. Within 1 min, bright blue fluorescence associated with the kinetoplast was observed in wild-type but not pentamidine-resistant (Pentr30) cells. The Pentr2.5 line, like the wild-type cells, continued to accumulate drug into the kinetoplast. Treating both wild-type and Pentr30 resistant cells with 1 μM digitonin, however, led to a clear fluorescence associated with the kinetoplast in both types of parasite within 3 to 5 min. This indicates that drug is bound to the kinetoplast DNA regardless of the resistance phenotype. However, the compound is apparently selectively accumulated across the mitochondrial membrane of wild-type but not high-level pentamidine-resistant parasites.
The ability of wild-type and resistant cells to accumulate the hydrophobic cation rhodamine-123, which is widely used as an indicator of mitochondrial membrane potential (25), was also studied. At time zero, both wild-type and resistant cells (Pentr30) had very similar rhodamine-123 fluorescence. However, 30 min later, a substantially greater fluorescence was evident in wild-type cells, while the fluorescence of the resistant cells was virtually unchanged since the initial time point (Fig. (Fig.6).6). This indicates that resistant cells had a reduced mitochondrial membrane potential compared with wild-type cells.
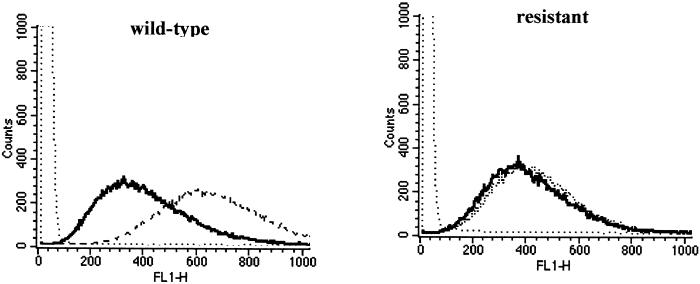
Flow cytometry analysis of wild-type and pentamidine-resistant L. mexicana promastigotes. Parasite fluorescence was measured immediately (black line) or 30 min (irregular dotted line) after addition of rhodamine-123. The level of fluorescence of the parasites in the absence of rhodamine-123 is represented for comparison (regular dotted line). Data from one representative experiment are presented.
DISCUSSION
Resistance to antimicrobial agents has become a serious issue in efforts to control disease caused by pathogenic microorganisms. Understanding of the mechanisms of resistance may have value in allowing reversal of drug resistance phenotypes and also in planning use of agents which circumvent operative resistance mechanisms. Pentamidine is a drug which is widely used in the treatment of early-stage sleeping sickness caused by the parasitic protozoan T. brucei. The same drug is also used for Pneumocystis carinii pneumonia, fungal infections, and antimony-refractory leishmaniasis. Pentamidine belongs to the diamidine class of drugs, which also contains berenil (diminazene aceturate), which is used against disease caused by African trypanosomes in animals.
Recently, a series of novel diamidine drugs have been shown to be effective against P. carinii, and prodrugs that can be delivered to the body through the oral route have become available (49, 50). Diamidines are substrates of the P2 nucleoside transporter operative in T. brucei (5, 23). Loss of this transporter can mediate resistance to drugs which enter cells via this route (5, 22). The situation regarding pentamidine uptake into T. brucei is complex (47) because in T. brucei, the drug also enters via at least two other transporters (29). Leishmania parasites are related to T. brucei, although we have shown here that pentamidine does not enter Leishmania parasites via a nucleoside transporter. Moreover, Leishmania spp. do not have a functional equivalent of P2 (38, 43). Thus, the mechanisms underpinning both sensitivity and resistance to pentamidine in Leishmania spp. must differ from those in trypanosomes.
The drug enters both promastigote and amastigote forms of the Leishmania cell via a carrier-mediated process which recognizes diamidines with high affinity. Efforts to identify a physiological substrate for the transporter were unsuccessful, although because arginine and other basic amino acids failed to inhibit uptake it appears unlikely that pentamidine enters these parasites with a basic amino acid transporter, as was previously suggested (40, 41). Polyamines also failed to inhibit uptake, ruling out polyamine transporters as the carriers of pentamidine into the cells. A wide variety of common metabolites also failed to inhibit pentamidine uptake. Three inhibitors of P-glycoproteins (PCP, TFP and verapamil) were competitive inhibitors of uptake, but the physiological role of the leishmanial transporter that carries pentamidine into the cell remains unknown.
The affinity for pentamidine shown by its transporter in L. mexicana was unaltered in resistant parasites. However, drug accumulated to a lower level, and the apparent Vmax for uptake was diminished in resistant parasites. A similar situation has been noted in resistance to isometamidium in Trypansoma congolense (60). Resistance in this case was concluded not to associate with alterations at the level of a plasma membrane transporter, but with changes in the mitochondrial membrane potential. Isometamidium rapidly enters the T. congolense mitochondrion, where it is binds tightly to kinetoplast DNA. The apparent decrease in Vmax in resistant cells correlated to diminished capacity to accumulate drug intracellularly (60).
Like isometamidium, diamidines are organic cations, and they also bind to the kinetoplast. Ultrastructural changes to kinetoplast DNA and the mitochondrion are observed in parasites exposed to these drugs (26, 37, 39). DAPI, a fluorescent analogue of pentamidine, enters cells and accumulates rapidly in the mitochondrion, binding to the kinetoplast in wild-type but not high-level pentamidine-resistant Leishmania strains. Treating cells with digitonin to render their membranes permeable revealed that the fluorescent diamidine bound equally well to wild-type and resistant kinetoplasts, indicating that it is access to the kinetoplast rather than the ability to bind to the DNA that differentiates wild-type and resistant cells. Previous studies have pointed to a diminution of mitochondrial membrane potential in pentamidine-resistant Leishmania spp. (11), and accumulation of rhodamine-123 also indicates that mitochondrial membrane potential is diminished in the drug-resistant lines analyzed in this study.
The data presented here are consistent with a model similar to that proposed for isometamidium resistance in T. congolense. Pentamidine appears to enter both resistant and wild-type cells via the same plasma membrane carrier, which is unaltered in the resistant lines. However, in wild-type but not resistant parasites, the drug is rapidly accumulated in the mitochondrion, with uptake being driven by the greater mitochondrial membrane potential of wild-type cells. In contrast, in resistant parasites, the drug does not accumulate rapidly in the mitochondrion but remains free in the cytosol. Efflux pumps appear to be operative in removing cytosolic and membrane-associated pentamidine from the cell, and it is likely that the pumps can remove the majority of the pentamidine from the nonmitochondrial compartment of both cell types.
This pentamidine efflux system is operative in both cell types, but in the wild-type cells, most of the pentamidine becomes bound to the kinetoplast, within the mitochondrion, and so is not available for efflux, thus remaining in the cell. This is not the case in the resistant parasites. A balance involving rate of uptake into the cell, efflux from the cell at the plasma membrane, and accumulation in the mitochondrion would then underlie the events reported here. Agents which inhibit efflux may lead to reversal of resistance by allowing pentamidine to accumulate in the cytosol to levels which then drive accumulation in the mitochondrion. In S. cerevisiae, a gene, PNT1, whose overexpression mediates pentamidine resistance, encodes a product that is targeted to the mitochondrion and is involved in the biogenesis of that organelle as a protein translocator (36). The mitochondrion appears to be the target of pentamidine action in S. cerevisiae, and based on the data presented here, it would appear that the mitochondrion also plays a crucial role in the mode of action for pentamidine in Leishmania parasites.
Acknowledgments
This work was supported by a Wellcome Trust Traveling Fellowship to M. Basselin.
We are grateful to Janice Brock for technical assistance.
REFERENCES
Articles from Antimicrobial Agents and Chemotherapy are provided here courtesy of American Society for Microbiology (ASM)
Full text links
Read article at publisher's site: https://doi.org/10.1128/aac.46.12.3731-3738.2002
Read article for free, from open access legal sources, via Unpaywall:
https://europepmc.org/articles/pmc132791?pdf=render
Citations & impact
Impact metrics
Citations of article over time
Smart citations by scite.ai
Explore citation contexts and check if this article has been
supported or disputed.
https://scite.ai/reports/10.1128/aac.46.12.3731-3738.2002
Article citations
Evaluation of the Leishmania Inositol Phosphorylceramide Synthase as a Drug Target Using a Chemical and Genetic Approach.
ACS Infect Dis, 10(8):2913-2928, 18 Jul 2024
Cited by: 0 articles | PMID: 39023360 | PMCID: PMC11320567
Amidine containing compounds: Antimicrobial activity and its potential in combating antimicrobial resistance.
Heliyon, 10(15):e32010, 31 May 2024
Cited by: 0 articles | PMID: 39170404 | PMCID: PMC11336351
Review Free full text in Europe PMC
ABC transporter inhibition by beauvericin partially overcomes drug resistance in Leishmania tropica.
Antimicrob Agents Chemother, 68(5):e0136823, 04 Apr 2024
Cited by: 1 article | PMID: 38572959 | PMCID: PMC11064568
Synthesis and Biophysical and Biological Studies of N-Phenylbenzamide Derivatives Targeting Kinetoplastid Parasites.
J Med Chem, 66(19):13452-13480, 20 Sep 2023
Cited by: 1 article | PMID: 37729094 | PMCID: PMC10578353
Recent Advances in Chemotherapeutics for Leishmaniasis: Importance of the Cellular Biochemistry of the Parasite and Its Molecular Interaction with the Host.
Pathogens, 12(5):706, 12 May 2023
Cited by: 5 articles | PMID: 37242374 | PMCID: PMC10222285
Review Free full text in Europe PMC
Go to all (73) article citations
Similar Articles
To arrive at the top five similar articles we use a word-weighted algorithm to compare words from the Title and Abstract of each citation.
Roles for mitochondria in pentamidine susceptibility and resistance in Leishmania donovani.
Mol Biochem Parasitol, 145(1):1-10, 15 Sep 2005
Cited by: 46 articles | PMID: 16219371
Pentamidine uptake in Leishmania donovani and Leishmania amazonensis promastigotes and axenic amastigotes.
Biochem J, 315 ( Pt 2):631-634, 01 Apr 1996
Cited by: 40 articles | PMID: 8615840 | PMCID: PMC1217243
Phenotypic characterization of Leishmania mexicana pentamidine-resistant promastigotes. Modulation of the resistance during in-vitro developmental life cycle.
C R Acad Sci III, 320(12):981-987, 01 Dec 1997
Cited by: 9 articles | PMID: 9587476
Pentamidine uptake and resistance in pathogenic protozoa: past, present and future.
Trends Parasitol, 19(5):232-239, 01 May 2003
Cited by: 123 articles | PMID: 12763430
Review
Funding
Funders who supported this work.