Abstract
Free full text

Phenotypic variation of Plasmodium falciparum merozoite proteins directs receptor targeting for invasion of human erythrocytes
Abstract
The members of the phylum Apicomplexa parasitize a wide range of eukaryotic host cells. Plasmodium falciparum, responsible for the most virulent form of malaria, invades human erythrocytes using several specific and high affinity ligand–receptor interactions that define invasion pathways. We find that members of the P.falciparum reticulocyte-binding homolog protein family, PfRh2a and PfRh2b, are expressed variantly in different lines. Targeted gene disruption shows that PfRh2b mediates a novel invasion pathway and that it functions independently of other related proteins. Phenotypic variation of the PfRh protein family allows P.falciparum to exploit different patterns of receptors on the erythrocyte surface and thereby respond to polymorphisms in erythrocyte receptors and to evade the host immune system.
Introduction
Plasmodium falciparum belongs to the phylum Apicomplexa, a group characterized by a highly specialized apical complex, including pedunculate organelles known as rhoptries that play a central role in the invasion of host cells. Plasmodium species infect a very restricted host range in the bloodstream; this specificity is determined by specific receptor–ligand interactions that occur between invasive forms of the parasite and the host erythrocyte (Butcher et al., 1973; Gratzer and Dluzewski, 1993). This is in contrast to some other members of Apicomplexa, such as Toxoplasma gondii, that invade a wide range of cell types from many different species. Despite the host cell specificity of P.falciparum, it has developed the ability to invade human erythrocytes using multiple parasite ligand–receptor interactions that have become known as alternative invasion pathways (Dolan et al., 1994; Okoyeh et al., 1999).
Erythrocyte invasion is a multistep process involving specific ligand–receptor interactions that mediate apical reorientation and adhesion of the parasite to the host cell (Gratzer and Dluzewski, 1993). A well-defined ligand– receptor interaction is erythrocyte-binding antigen 175 (EBA-175) and glycophorin A on the red blood cell surface (Dolan et al., 1994; Sim et al., 1994). Other invasion pathways have been defined using mutant erythrocytes and enzyme treatments, that use receptors such as glycophorin B and an unknown receptor termed ‘X’ (Dolan et al., 1994). The interactions of parasite ligands with glycophorin A and B depend on sialic acid present on these receptors (Miller et al., 1977; Howard et al., 1982; Pasvol et al., 1982; Pasvol, 1984). Truncation of EBA-175 in a parasite line highly dependent on sialic acid residues was associated with a switch towards invasion via a sialic acid-independent receptor of unknown identity (Reed et al., 2000). Recently, a number of EBA-175 homologs have been identified, and some bind to the erythrocyte surface in a sialic acid-dependent manner (Mayer et al., 2001; Thompson et al., 2001; Narum et al., 2002). The functions of these proteins in merozoite invasion are yet to be determined.
A second family of high molecular weight proteins that bind to erythrocytes with high affinity has been identified in the related human malaria Plasmodium vivax (Galinski et al., 1992) and the rodent malaria Plasmodium yoelii (Ogun and Holder, 1996). The P.vivax proteins bind selectively to reticulocytes and have been termed reticulocyte-binding protein-1 and -2 (RBP-1 and RBP-2) (Galinski et al., 1992). They are located at the apical end of merozoites and have been hypothesized to play a role in the recognition of reticulocytes by merozoites. Merozoite proteins in other species of Plasmodium, that are related to the RBPs of P.vivax, have been designated as members of a reticulocyte binding-like or RBL family of adhesive invasion proteins (Rayner et al., 2001; Miller et al., 2002). The P.falciparum RBL homologs (PfRh) of the P.vivax RBPs and the P.yoelii Py235 family have been identified and characterized (Rayner et al., 2000, 2001; Taylor et al., 2001; Triglia et al., 2001; Kaneko et al., 2002). There are four members of the PfRh or NBP protein family, encoded by separate genes, and these have been named PfRh1, PfRh2a, PfRh2b and PfRh4, or NBP-1, NBP-2a, NBP-2b and NBP-4 (Rayner et al., 2000, 2001; Triglia et al., 2001; Kaneko et al., 2002). A fifth gene, PfRh3 (Taylor et al., 2001), has been identified, and it has been suggested to be a pseudogene; however, proteomic analysis shows that it may be expressed in sporozoites (Florens et al., 2002). PfRh1/NBP-1 binds to erythrocytes via a neuraminidase-sensitive and trypsin-resistant receptor, and some evidence suggests that PfRh2b may form a complex with PfRh1 (Rayner et al., 2001).
The P.yoelii Py235 family is encoded by perhaps up to 14 genes, and individual merozoites originating from a single schizont transcribe distinct members of this gene family (Preiser et al., 1999). This clonal phenotypic variation has been suggested to provide this murine malaria with a survival strategy in the host by expression of a single but different Py235 protein in each merozoite, allowing the parasite to respond efficiently to variations in the host environment. However, the functional consequence of this transcriptional variation remains unclear.
Here we have analyzed the P.falciparum PfRh proteins and have found a remarkable degree of variant expression in different parasite lines. Using gene disruptions, we have identified a novel erythrocyte invasion pathway, and show that variation in expression of these proteins alters the pattern of receptor usage for invasion of human erythrocytes. This provides a unique strategy for parasite invasion in the face of erythrocyte receptor polymorphism and host immune responses.
Results
Variant expression of PfRh2a and PfRh2b proteins in multiple P.falciparum isolates
The PfRh family members in P.falciparum are large molecular weight proteins, homologous to P.vivax PvRBP-1 and -2 (Galinski et al., 2000) and the P.yoelii Py235 family (Ogun and Holder, 1996; Preiser et al., 1999). To address the role of PfRh2a and PfRh2b in merozoite invasion of P.falciparum into erythrocytes, we analyzed expression of these proteins in isolates of P.falciparum taken from different geographic areas. Immunoblots of supernatants were probed with antibodies for PfRh2a or PfRh2b (Figure 1A) (Rayner et al., 2000). Evidence for variation in expression of these proteins was obtained as PfRh2a was detectable in 3D7, T996, HB3, D10, 7G8, K1, Pf120 and W2mef, but was not apparent in MCAMP, FCB1, T994 or FCR3. PfRh2b showed a similar pattern of expression but was absent in the D10 isolate. The SERA5 protein was used as a loading control. These results show that PfRh2a and PfRh2b are expressed variably in different isolates, and are in agreement with recent data showing some variation in expression of PfRh2b in three P.falciparum isolates (Taylor et al., 2002).
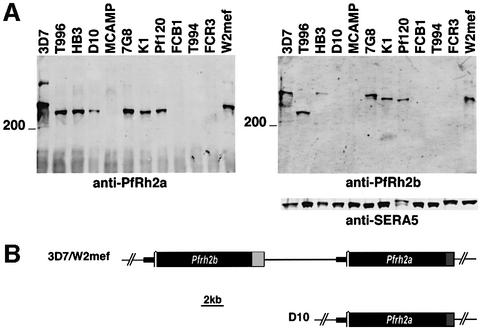
Fig. 1. Variant expression, subcellular localization and structure of the PfRh2a and PfRh2b genes and proteins in P.falciparum. (A) Supernatants from 3D7, T996, HB3, D10, MCAMP, 7G8, K1, Pf120, FCB1, T994, FCR3 and W2mef were probed with anti-PfRh2a, anti-PfRh2b or anti-SERA5 antibodies. The position of the 200 kDa molecular weight marker is shown. (B) Structure of the PfRh2a and PfRh2b locus in 3D7, W2mef and D10. The regions in black are identical for the PfRh2b and PfRh2a genes. The light gray (PfRh2b) and darker gray (PfRh2a) sections are non-homologous.
PCR analysis of genomic DNA from the different isolates 3D7, T996, HB3, 7G8, K1, Pf120, W2mef, MCAMP, FCB1, T994 and FCR3 detected PfRh2a and PfRh2b genes in all isolates, with the exception of D10, which lacks PfRh2b (Triglia et al., 2001). The P.falciparum isolates MCAMP, FCB1, T994 and FCR3 do not express PfRh2a or PfRh2b protein despite having both intact genes within their genomes (data not shown). To determine whether the variability in expression had any effect on merozoite invasion, we constructed gene disruptions in parasite lines in which they normally are expressed.
The PfRh2a and PfRh2b genes are in a head to tail configuration on chromosome 13
To facilitate gene disruptions of the PfRh2a and PfRh2b genes, we characterized their chromosomal organization. The PfRh2a and PfRh2b genes are identical for their first 7.5 kb, but diverge greatly at their C-termini, sharing only a putative membrane-spanning region and a cytoplasmic tail (Figure 1B) (Rayner et al., 2000; Triglia et al., 2001). Using Southern blots and PCR experiments, we showed that PfRh2b and PfRh2a are arranged head to tail in both 3D7 and W2mef, with PfRh2b at the 5′ end (Figure 1B). They are separated by ~7.0 kb of sequence, with no intervening coding regions. Southern blots of the D10 PfRh2a gene showed that it is present in a single copy, with the 5′-untranslated region identical to the equivalent region of the 3D7 PfRh2b gene. This suggests that PfRh2b was deleted from the D10 parasite line by an intergenic recombination between the two highly similar 5′ ends of PfRh2a and PfRh2b.
The PfRh2a and b proteins are located in the neck of the rhoptries of merozoites
To define the subcellular localization of the PfRh2a/b proteins, we performed immunogold-electron microscopy with the anti-PfRh2a/b antibodies (Figure 2). First we determined the subcellular localization of PfRh2a/b proteins in schizont stages of 3D7 parasites. Previous immunofluorescence experiments co-localizing the PfRh2a/b proteins with rhoptry proteins such as rhoptry-associated protein 1 (RAP1), Pf140 and Pf240/225 suggested that they are located towards the apical end of the merozoite, and co-localization with Pf240/225 but not exactly with RAP-1 or Pf140 suggested perhaps a location in the peduncle of the rhoptries (Rayner et al., 2000, 2001; Triglia et al., 2001).
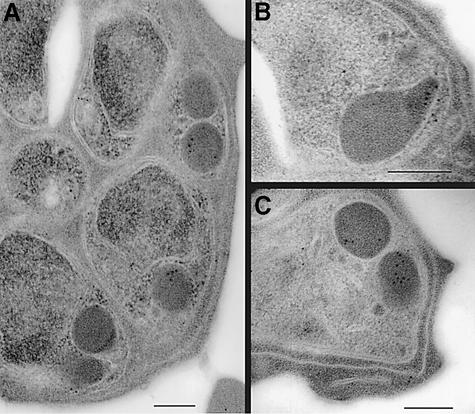
Fig. 2. Subcellular localization of PfRh2a/b in 3D7 parasites by immuno electron microscopy. (A–C) Cross-sections through merozoites. Scale bars are 200 nm in all panels.
Immunoelectron microscopy with anti-PfRh2a/b antibodies showed labeling in the electron-dense rhoptry organelles of the parasite (Figure 2A and C), with label evident in the neck of the rhoptry in some parasites (Figure 2B). The invasion proteins AMA-1 and EBA-175 were found to be present predominantly in the micronemes of parasites from the same preparation (Healer et al., 2002). Thus it appears that PfRh2b is positioned more apically than micronemal proteins. In 3D7Δ2b parasite lines lacking PfRh2b expression (described below), PfRh2a localization was unaltered (data not shown). Immunofluorescence experiments with anti-PfRh2a/b antibodies in 3D7Δ2a (described below, lacks PfRh2a) and 3D7Δ2b (lacks PfRh2b) parasites showed that localization of PfRh2a and PfRh2b in the corresponding PfRh2a-null or PfRh2b-null parasites remained unchanged relative to the RAP1 protein (Baldi et al., 2000) (data not shown). These data show definitively that the subcellular localizations of the PfRh2a and b proteins are not dependent on each other and that they are both localized predominantly in the body and neck of the rhoptries in P.falciparum merozoites.
PfRh2a and PfRh2b genes can be disrupted
In order to derive 3D7 parasite lines that lack expression of either PfRh2a or PfRh2b proteins, we constructed a plasmid (pHTkΔrh) that would integrate into either gene by double recombination crossover (Figure 3A) (Duraisingh et al., 2002). Integration of pHTkΔrh into 3D7 chromosomes was confirmed by Southern blotting, and four cloned lines were derived in which either PfRh2a or PfRh2b was disrupted (Figure 3B). Hybridization of a PrC probe (Figure 3A) to genomic DNA from 3D7 revealed a hybridizing fragment of 3.0 kb corresponding to the endogenous PfRh2a and PfRh2b genes. In parasites that maintained the pHTkΔrh vector episomally (called 3D7/pHTk parasites), a corresponding 9.6 kb plasmid fragment was evident in addition to the endogenous 3.0 kb fragment. Two clones were derived, 3D7Δ2a/b1 and 3D7Δ2a/b2, that retained the 3.0 kb fragment but also showed a second band of 4.9 kb. This indicated that the transfection plasmid had integrated into either the PfRh2a or the PfRh2b genes. Hybridization with PrB or PrA (which are specific to the PfRh2b or PfRh2a genes, respectively) to genomic DNA from 3D7 and the four cloned lines showed that PfRh2b was disrupted in 3D7Δ2b1 and 3D7Δ2b2, whereas PfRh2a was disrupted in 3D7Δ2a1 and 3D7Δ2a2.
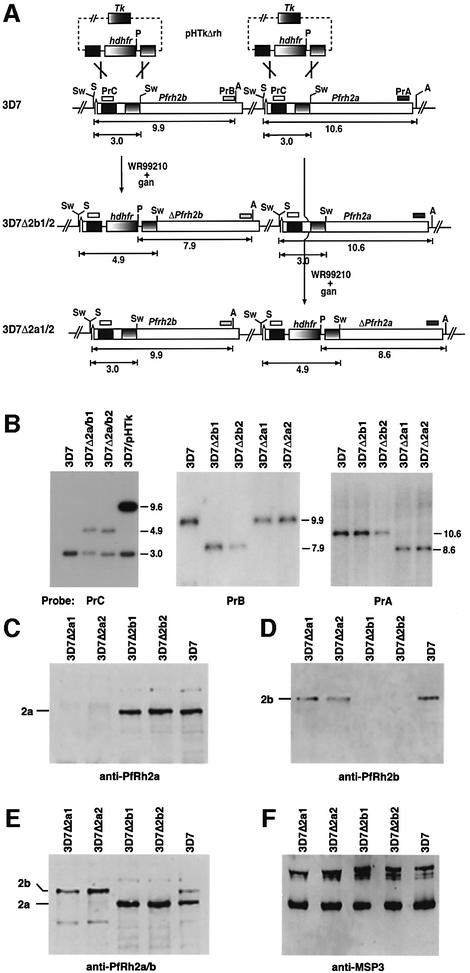
Fig. 3. Disruption of the PfRh2b and PfRh2a genes in 3D7 parasites. (A) The pHTkΔrh plasmid has the human dhfr gene (hdhfr) flanked by two target sequences for homologous recombination within the PfRh2a and PfRh2b genes. It also includes the thymidine kinase gene (Tk) for negative selection with ganciclovir. 3D7Δ2b1/2 and 3D7Δ2a1/2 are the cloned transfected lines showing the structure of the double recombination crossovers. The PfRh2a and PfRh2b genes are shown with relevant restriction enzyme sites: Sw, SwaI; S, SacI; A, AvaII; P, PvuII. The sizes are in kbp. (B) Southern blotting of genomic DNA from 3D7, 3D7Δ2a/b1 and 3D7Δ2a/b2 (uncloned population transfected with pHTkΔrh), 3D7/pHTk (uncycled parasites transfected with pHTkΔrh), 3D7Δ2b1 and 3D7Δ2b2 (cloned lines), 3D7Δ2a1 and 3D7Δ2a1/a2 (cloned lines). The left panel was digested with PstI and SwaI, whilst the other panels were digested with SacI, AvaII and PvuII. (C–F) PfRh2a and PfRh2b are not expressed in the parasites with the PfRh2a and PfRh2b genes, respectively, disrupted. Supernatants from 3D7Δ2a1, 3D7Δ2a2, 3D7Δ2b1, 3D7Δ2b2 and 3D7 were probed with the relevant antisera: (C) anti-PfRh2a sera; (D) anti-PfRh2b sera; (E) affinity-purified anti-PfRh2a/2b antibodies; (F) anti-MSP3 antibodies.
To determine whether disruption of PfRh2a and PfRh2b resulted in lack of expression of the corresponding protein, we used western blots of supernatants probed with antibodies against each protein (Figure 3C–E). Antibodies to the C-terminus of PfRh2a (Rayner et al., 2000) revealed two proteins >200 kDa in 3D7, 3D7Δ2b1 and 3D7Δ2b2, whereas these proteins were absent in 3D7Δ2a1 and 3D7Δ2a2 (Figure 3C). This is consistent with disruption of the PfRh2a gene in both 3D7Δ2a1 and 2. Similarly, antibodies to the C-terminus of PfRh2b (Rayner et al., 2000) showed a major band that was also >200 kDa in 3D7, 3D7Δ2a1 and 2, whereas it was absent from 3D7Δ2b1 and 2 (Figure 3D). Using the anti-PfRh2a/b (2A9) antibodies that detect both PfRh2a and PfRh2b (Triglia et al., 2001), we confirmed that the corresponding proteins were absent from the recombinant parasite lines (Figure 3E). These results clearly show that PfRh2a and PfRh2b proteins are not expressed in 3D7Δ2a1/2 or 3D7Δ2b1/2, respectively. Western blots with schizont pellets of each parasite line gave similar results and confirmed the lack of expression of each protein in the parasite cloned lines (data not shown). The level of expression of the intact PfRh gene in each knockout line appeared unchanged when tested in multiple western blot experiments compared with the 3D7 wild-type strain and the control protein MSP3 (Figure 3F). This suggested that integration of the transfection cassette into the neighboring gene had no apparent effect on expression of the adjacent gene.
PfRh2b function is required for a novel invasion pathway in P.falciparum
Having constructed 3D7 recombinant parasite lines that lack expression of PfRh2a or PfRh2b, we initially tested the effect of loss of these proteins on the ability of merozoites to invade untreated human erythrocytes (Dolan et al., 1990, 1994). The growth and merozoite invasion rate of 3D7ΔRh2a1 and 2, and 3D7ΔRh2b1 and 2 parasites was unchanged relative to their 3D7 parent (data not shown). This suggested either that the PfRh2a and PfRh2b proteins are not required for merozoite invasion or that the function of PfRh2a and PfRh2b is compensated for by other parasite ligands in the knockout parasite lines. This has been described previously for the parasite ligand EBA-175 which binds to glycophorin A and mediates an invasion pathway via this receptor (Reed et al., 2000). Loss of function of EBA-175 results in a switch in merozoite invasion to a sialic acid-independent pathway, and this parasite invades and grows as well as parental parasites.
To examine the role of the PfRh2a and PfRh2b proteins in erythrocyte invasion further, we determined the effect of different enzyme treatments on the ability of the parental parasites (3D7) to invade protease- or neuraminidase-treated erythrocytes (Table I). Neuraminidase removes sialic acid residues from the erythrocyte surface and blocks invasion pathways dependent on sialic acid present on both glycophorin A and other receptors (Miller et al., 1977). Trypsin treatment cleaves proteins such as glycophorin A and C, but does not affect glycophorin B (Thompson et al., 2001). Chymotrypsin cleaves a non-overlapping set of proteins including glycophorin B and band 3 on the erythrocyte surface. The different enzyme treatments have the effect of limiting the repertoire of receptors on the erythrocyte, allowing dissection of specific ligand–receptor interactions. This is evident from the effect of different enzyme treatments on erythrocytes and the decreased ability of 3D7 to invade many of these treated cells (Table I).
Table I.
Erythrocyte treatmenta | Parasiteb | |||||
---|---|---|---|---|---|---|
3D7 | 3D7Δ2b1 | 3D7Δ2b2 | 3D7Δ2a1 | 3D7Δ2a2 | D10 | |
Low trypsin/chymotrypsin | 22.8 ± 7.6 | 70.5 ± 7.7c | 65.5 ± 12.9c | 18.1 ± 4.8 | 14.1 ± 2.6 | 58.0 ± 5.8c |
Neuraminidase/low trypsin | 23.0 ± 6.9 | 3.9 ± 0.6c | 3.3 ± 0.4c | 24.5 ± 6.0 | 21.9 ± 4.9 | 10.26 ± 2.3c |
Chymotrypsin | 67.0 ± 9.1 | 105.3 ± 9.4c | 113.9 ± 14.3c | 70.1 ± 10.1 | 65.3 ± 15.1 | 99.8 ± 5.9c |
Low trypsin | 93.5 ± 9.0 | 63.3 ± 7.8c | 61.7 ± 9.7c | 80.8 ± 6.7 | 93.0 ± 14.0 | 85.9 ± 7.3 |
Neuraminidase | 79.2 ± 8.5 | 58.2 ± 8.7c | 47.5 ± 6.3c | 73.9 ± 7.1 | 82.7 ± 14.9 | 74.3 ± 11.4 |
aRed blood cells were treated with the enzymes indicated, as described in Materials and methods, before testing in merozoite invasion assays.
bFigures are the percentage invasion compared with invasion of 3D7 into untreated blood cells, and errors are 95% confidence limits. The data were from 6–14 independent experiments all carried out in triplicate. In the experiments, the starting parasitemia was 0.5% and the final parasitemia was between 3 and 6% for untreated erythrocytes.
cSignificantly different invasion when compared with 3D7.
The 3D7Δ2b1 and 3D7Δ2b2 parasites, lacking expression of PfRh2b, show a dramatically altered invasion phenotype when compared with 3D7 (Table I). The 3D7Δ2b parasites invade both neuraminidase- and trypsin-treated erythrocytes at a significantly lower efficiency than the 3D7 parent. This reflects a shift in dependency in the 3D7Δ2b parasites from 3D7 parasites using neuraminidase-resistant (sialic acid-independent) and trypsin-resistant receptors, towards using neuraminidase-sensitive (sialic acid-dependent) and trypsin-sensitive receptors in 3D7Δ2b parasites, upon disruption of PfRH2b. Moreover, when double combinations of enzyme treatments of erythrocytes were used, it was found that the 3D7Δ2b1/2 parasites invade erythrocytes treated with neuraminidase/trypsin at a much lower level compared with 3D7. This treatment appears to limit the erythrocyte receptors available to the parasite, leaving a residual neuraminidase-resistant/trypsin-resistant receptor that can be utilized by a PfRh2b-dependent invasion pathway in 3D7 (23% invasion) but can no longer be used by 3D7Δ2b1/2 parasites that lack PfRh2b (3.6% invasion) (Table I; Figure 6A). Thus, it can be inferred that the receptor for PfRh2b is neuraminidase resistant (sialic acid independent) and trypsin resistant from the PfRh2b-dependent 3D7 invasion of neuraminidase/trypsin-treated cells.
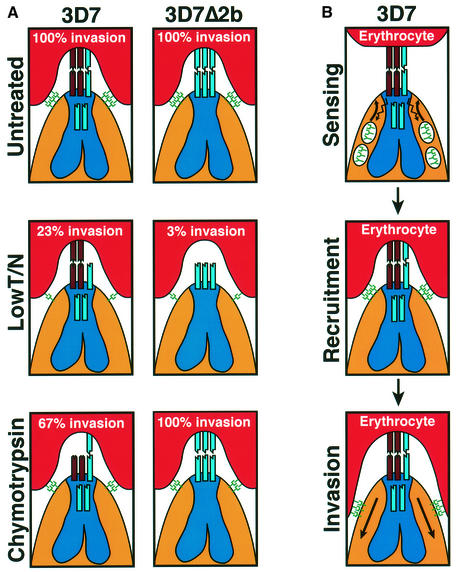
Fig. 6. A simplified model of merozoite invasion into erythrocytes by 3D7 and 3D7Δ2b parasites and the role of the PfRh protein family. (A) A model explaining the enzyme sensitivity of receptor Z as trypsin resistant, neuraminidase resistant and chymotrypsin sensitive. The invasion data are from Table I. Top panels: both 3D7 and 3D7Δ2b parasites show 100% invasion of untreated erythrocytes. The PfRh proteins (shown at the apical end of the merozoites and in the neck of the rhoptries) are responsible for sensing the apical end interaction and signaling to the micronemes for release of high affinity ligands (see B). The eythrocyte-binding antigens (EBAs) and their receptors are shown as green symbols. In 3D7, PfRh2b is responsible for the dominant sensing pathway for invasion via the chymotrypsin-sensitive receptor Z (brown). Disruption of the PfRh2b gene in 3D7 results in replacement of PfRh2b with an alternative pathway specified by another protein [shown as a blue ligand in the neck of the 3D7 rhoptries and interacting with a receptor (blue) on the erythrocyte in 3D7Δ2b] that binds to a chymotrypsin-resistant receptor. Middle panels: following erythrocyte treatment with trypsin and neuraminidase, 3D7 and 3D7Δ2b give 23 and 3% invasion, respectively. The trypsin and neuraminidase resistance of receptor Z is shown. Bottom panels: following erythrocyte treatment with chymotrypsin, 3D7 and 3D7Δ2b give 67 and 100% invasion, respectively. Some invasion can still be achieved using the alternative parasite ligand shown in the rhoptries that binds to a chymotrypsin-resistant receptor. The chymotrypsin sensitivity of receptor Z is shown. (B) Model for the role of the PfRh proteins in sensing the erythrocyte. This would activate release of proteins from micronemes and recruitment at the tight junction, followed by invasion.
In contrast, the 3D7Δ2b1/2 parasites invade erythrocytes treated with chymotrypsin better than the 3D7 parent, indicating that the loss of the PfRh2b ligand in the null parasites has led to an increased dependency on ligands that utilize chymotrypsin-resistant receptors for invasion. It can therefore be inferred that the cognate receptor for PfRh2b that is used for invasion in wild-type 3D7 is chymotrypsin sensitive (67% invasion compared with 100% for 3D7Δ2b1/2, which cannot use PfRh2b) (Table I; Figure 6A). The reliance of merozoite invasion on this chymotrypsin-sensitive receptor can be increased by limiting the available receptors on the erythrocyte surface as a result of additional treatment with trypsin. 3D7Δ2b parasites invade trypsin/chymotrypsin-treated erythrocytes at least 3-fold better than 3D7 (Table I). To confirm this, an independent 3D7 transfectant with PfRh2b disrupted was obtained, and a clone derived from this population gave the same merozoite invasion phenotype as that seen for 3D7Δ2b1/2 (data not shown). Taken together, these data show that PfRh2b functions in a dominant invasion pathway in 3D7 that utilizes an erythrocyte receptor that is chymotrypsin sensitive and neuraminidase/trypsin resistant (Figure 6A). The loss of PfRh2b function leads to an increased reliance on other invasion pathways.
As further evidence that loss of PfRh2b expression in 3D7Δ2b1 and 2 was responsible for the invasion phenotype observed, we tested the ability of D10 merozoites that lack the PfRh2b gene (Triglia et al., 2001) to invade enzyme-treated erythrocytes (Table I). Comparison of D10 invasion with 3D7 showed striking similarities to that observed with 3D7Δ2b1 and 2 that also lack PfRh2b. In particular, both the observed ability of D10 merozoites to invade chymotrypsin/trypsin-treated erythrocytes and the impaired invasion of neuraminidase/trypsin-treated cells by D10 lend support to the role of PfRh2b in a merozoite invasion pathway involving a chymotrypsin-sensitive and neuraminidase/trypsin-resistant receptor, in multiple parasite lines from different geographical locations.
Next we tested the effect of loss of expression of PfRh2a on merozoite invasion. A comparison of the ability of 3D7 and 3D7Δ2a merozoites to invade the different enzyme-treated erythrocytes showed no apparent differences (Table I). This was in contrast to the dramatic changes seen with loss of PfRh2b expression. PfRh2a is expressed in 3D7, but its loss of expression was not associated with a measurable shift in reliance to other invasion pathways. Interestingly, attempts to disrupt the PfRh2a gene in D10, a parasite that lacks the PfRh2b gene, were not successful, suggesting that the PfRh2a protein fulfills an important function in this parasite line, which lacks PfRh2b protein (data not shown).
Inhibition of PfRh2b function using specific antibodies impedes merozoite invasion via the PfRh2b invasion pathway
To dissect the function of PfRh2a/b in merozoite invasion further, we used antibodies specific for the conserved regions of PfRh2a/b to test their ability to inhibit invasion into enzyme-treated erythrocytes in parasites in which the different genes were disrupted (Figure 4). Invasion of 3D7, 3D7Δ2a, 3D7Δ2b and D10 parasites into untreated erythrocytes showed no significant inhibition using these antibodies (Figure 4A). This result was not consistent with previous data suggesting that antibodies to either PfRh2a or PfRh2b can inhibit 3D7 merozoite invasion of untreated erythrocytes (Rayner et al., 2001; Triglia et al., 2001). Our results using parasites with specific PfRh2a/b gene disruptions allow specificity controls (Figure 4) that have not been possible previously.
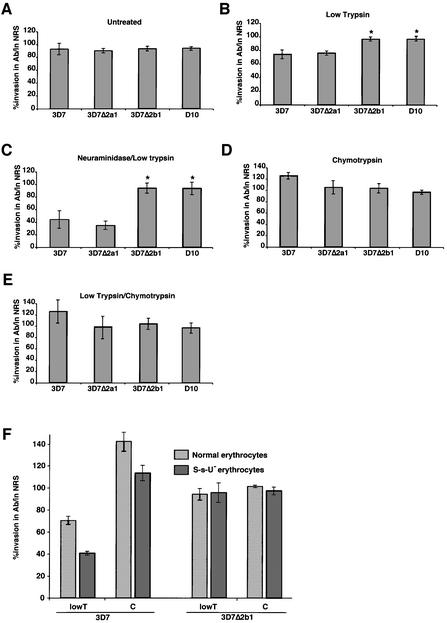
Fig. 4. Specific antibody inhibition of PfRh2b function in 3D7, 3D7Δ2a1, 3D7Δ2b1 and D10. Assays measure the percentage merozoite invasion in the presence or absence of anti-PfRh2a/b IgG antibodies relative to controls with IgG from normal rabbit sera (NRS). The erythrocytes in each panel are: (A) untreated; (B) trypsin treated; (C) neuraminidase/trypsin treated; (D) chymotrypsin treated; and (E) trypsin/chymotrypsin treated. (F) Assays measure the percentage merozoite invasion into S-s-U– erythrocytes that lack glycophorin B. Error bars in all cases show 95% confidence limits from 4–10 experiments in triplicate.
The anti-PfRh2a/b antibodies, however, did show significant inhibition when the potential receptors available on the erythrocyte surface for merozoite invasion were restricted using enzyme-treated erythrocytes. Merozoite invasion of 3D7 into trypsin- and neuraminidase/trypsin-treated erythrocytes was significantly decreased in the presence of anti-PfRh2a/b antibodies (Figure 4B and C). This antibody inhibition was due specifically to blockage of PfRh2b function, as 3D7Δ2b1 parasites, that did not express the PfRh2b protein, showed no antibody-mediated inhibition of invasion into either untreated or enzyme-treated erythrocytes (Figure 4A–C). The level of invasion in the presence of anti-PfRh2b antibodies by 3D7 (43%) versus 3D7Δ2b1 (95%) into neuraminidase/trypsin-treated erythrocytes is consistent with the conclusion that the PfRh2b receptor is neuraminidase and trypsin resistant.
To confirm that PfRh2b functions via a chymotrypsin-sensitive receptor, we tested direct inhibition of this parasite protein using the anti-PfRh2a/b antibodies in a merozoite invasion assay (Figure 4). These antibodies showed no inhibition of merozoite invasion of either 3D7 or 3D7Δ2b1 into chymotrypsin-treated erythrocytes (Figure 4D). Significantly, no inhibition of invasion was seen of either 3D7 or 3D7Δ2b1 into trypsin/chymotrypsin-treated erythrocytes, suggesting that chymotrypsin treatment had removed the PfRh2b receptor, blocking all function of the PfRh2b protein (Figure 4D and E). This also confirms that the antibodies are directly inhibiting the function of the PfRh2b protein rather than acting by non-specific steric hindrance. These results strongly support our data showing that PfRh2b acts in a merozoite invasion pathway using a chymotrypsin-sensitive receptor on the erythrocyte surface.
PfRh2b functions in a novel merozoite invasion pathway
The receptor utilized by PfRh2b is trypsin resistant and chymotrypsin sensitive, and a potential candidate on the erythrocyte was glycophorin B. To test whether PfRh2b utilizes glycophorin B, we used erythrocytes from an S-s-U– individual who lacks this protein on their red blood cells (Figure 4F). The PfRh2a/b antibodies significantly inhibited invasion by 3D7 merozoites into tryspin-treated erythrocytes; however, as expected, there was no inhibition of 3D7Δ2b parasites. Inhibition of 3D7 invasion into trypsin-treated erythrocytes by the antibodies was ablated by pre-treatment with chymotrypsin, consistent with removal of the receptor. These results show that PfRh2b function during merozoite invasion is not via glycophorin B as a receptor. The physical properties of the receptor have not been described previously for other defined erythrocyte receptors (Dolan et al., 1994; Rayner et al., 2001), and therefore represent a novel merozoite invasion pathway, which we refer to as receptor Z.
The function of PfRh2b is independent of PfRh1
The PfRh2a and PfRh2b proteins are part of a family that includes PfRh1, and it has been suggested previously that PfRh2b forms a complex with PfRh1 that functions in merozoite invasion (Rayner et al., 2001). To analyze the role of PfRh1, we raised antibodies to different regions of this protein and used them in western blots to detect the protein (Figure 5C). No specific protein bands were observed in 3D7 with antibodies to PfRh1, athough a major protein of ~190 kDa was observed in supernatants from another P.falciparum strain W2mef. This contrasted with EBA-175 that was detected in both parasite lines. Identical experiments with schizont pellets gave similar results. These results show that the amount of PfRh1 expressed in 3D7 is below detectable levels, suggesting that the PfRh1 parasite ligand is not functional in merozoite invasion of this parasite line.
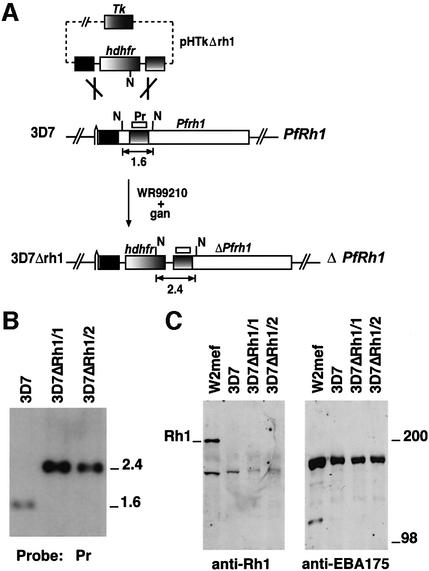
Fig. 5. Disruption of the PfRh1 gene in 3D7. (A) Pr represents the probe used for Southern hybridization in (B). The restriction enzyme shown as N is NsiI. pHTkΔrh1 has integrated into PfRh1 by a double recombination crossover event to yield 3D7ΔRh1. (B) Southern hybridization of genomic DNA digested with NsiI from 3D7, 3D7ΔRh1/1 and 3D7ΔRh1/2. Sizes on the right are in kb. (C) Western blot of supernatants from W2mef, 3D7, 3D7ΔRh1/1, 3D7ΔRh1/2 with antibodies to PfRh1 (first panel) and EBA175 (second panel).
To exclude the possibility that PfRh1 was expressed at low levels in 3D7 and still played a role in merozoite invasion of erythrocytes, we made constructs for transfection of 3D7 to disrupt the PfRh1 gene. The pHTkΔrh1 vector was transfected into 3D7 and, after selection on WR99210 and ganciclovir, parasites were obtained that had integrated the vector (Duraisingh et al., 2002). Southern blot experiments confirmed that the PfRh1 gene had been disrupted by integration of the pHTkΔrh1 vector in a double recombination crossover event (Figure 5B) (Duraisingh et al., 2002). Restriction mapping of this locus in two cloned lines 3D7ΔRh1/1 and 2 confirmed the structure of the integration event and disruption of the PfRh1 gene.
Western blot analysis of 3D7ΔRh1/1 and 2 with antibodies to the PfRh1 protein showed identical patterns to those seen in the 3D7 parental line, suggesting that this protein was not expressed (Figure 5C). Additionally, we determined whether the PfRh1 protein could be detected in the 3D7Δ2b parasites using western blots. No PfRh1 protein could be detected in these parasites, suggesting that the altered invasion phenotypes of the 3D7Δ2b were not due to increased expression of PfRh1 (data not shown). To confirm further that disruption of PfRh1 had no effect on merozoite invasion, we analyzed the ability of 3D7, 3D7ΔRh1/1 and 2 merozoites to invade untreated and enzyme-treated erythrocytes. The three parasite lines showed identical abilities to invade these cells, suggesting that PfRh1 was not functional in 3D7, 3D7ΔRh1 or 2 (data not shown). Therefore, the PfRh2b protein functions in a merozoite invasion pathway independent of PfRh1 expression and function.
Discussion
The availability of the P.falciparum genome sequence has allowed the identification of two adhesive families of parasite proteins, namely the EBA (Adams et al., 2001) and PfRh proteins (Rayner et al., 2000, 2001; Taylor et al., 2001; Triglia et al., 2001; Kaneko et al., 2002), that may play an important role in determining the pattern of receptor usage on the erythrocyte surface. Here we have used specific gene knockouts to reveal that a member of the PfRh protein family, PfRh2b, functions to define an alternative invasion pathway (Figure 6). Moreover, we find that the PfRh proteins vary in their expression in different parasite lines to provide a mechanism of phenotypic variation utilizing alternative receptors for invasion of merozoites into human erythrocytes. The availability of different parasite ligands for merozoite invasion alters the pattern of receptor utilization, enabling P.falciparum parasites to increase the chance of successful invasion in the face of erythrocyte receptor polymorphism and host immune responses.
Nature of the receptor for the PfRh2b invasion pathway
The PfRh2b ligand mediates invasion through a chymotrypsin-sensitive, trypsin/neuraminidase-resistant receptor, which we refer to as receptor Z (Figure 6A). Plasmodium falciparum is known to utilize several receptors on the erythrocyte surface for merozoite invasion, including glycophorins A, B, C and an unknown receptor X (Dolan et al., 1990, 1994; Mayer et al., 2001; Thompson et al., 2001; Narum et al., 2002). Since receptor Z is trypsin resistant, it cannot correspond to glycophorins A, C or receptor X, all of which are trypsin sensitive. The putative receptor Y, which binds to the ligand PfRh1, is trypsin resistant but neuraminidase sensitive (Rayner et al., 2001), so is also distinct from receptor Z. Glycophorin B possesses the enzyme sensitivities of receptor Z, but PfRh2b function can be inhibited in merozoite invasion of S-s-U– erythrocytes lacking this receptor, demonstrating that it is not utilized by this invasion pathway. This suggests that PfRh2b functions in a merozoite invasion pathway distinct from any described previously.
The PfRh1 protein binds to receptor Y on the erythrocyte surface (Rayner et al., 2001); however, there is no direct evidence that PfRh2b binds directly to receptor Z (Rayner et al., 2000; Triglia et al., 2001). Failure to detect binding in previous assays could be due to either the absence of the binding domain in the processed protein released into the supernatant or low affinity binding that is not detectable by these methods. Since the PvRBP and Py235 homologs of PfRh2b from P.vivax (Galinski et al., 1992) and P.yoelii (Ogun and Holder, 1996) bind to reticulocytes or normalocytes, respectively, it is therefore likely that PfRh2b also binds directly to receptor Z. However, we cannot rule out the possibility that PfRh2b binds indirectly to receptor Z through an intermediate ligand (Baldi et al., 2000). Importantly, disruption of the PfRh2b gene and demonstration of protein function in merozoite invasion by inhibition with antibody have both provided direct evidence for the role of PfRh2b in a novel invasion pathway.
The location of the very large PfRh2a/b proteins at the apical end of the merozoite, together with the pivotal role of PfRh2b in an invasion pathway, suggest that the PfRh proteins may be involved in sensing the apical interaction and signaling for subsequent events to form the tight junction (Figure 6) (Galinski et al., 1992; Barnwell and Galinski, 1998). This has also been suggested for the role of the Py235 protein family in P.yoelii invasion of erythrocytes (Khan et al., 2001). We speculate that once apical interaction is established between the merozoite and erythrocyte, the PfRh proteins may recruit high affinity receptors such as EBA-175 by release of micronemal components. Disruption of interactions at the apical end or subsequent recruitment of high affinity receptors by enzyme treatment of erythrocytes and removal of some receptors would have a similar effect on the pattern of merozoite invasion due to blockage of sequential steps in the same process (Figure 6).
Compensation for loss of PfRh2b function by other parasite ligands?
Binding and entry of P.falciparum invasive merozoites into human erythrocytes require specific ligand–receptor interactions. The observed strain variation in the utilization of these invasion pathways is very stable and does not change in the course of routine culture (Dolan et al., 1994; Okoyeh et al., 1999). Merozoites require sufficient affinity of ligand binding to cognate receptors to activate subsequent steps in the invasion process (see Barnwell and Galinski, 1998). It is likely that the area available for interaction between the apical end of the merozoite and the erythrocyte is limited, and therefore the number of ligands occupying that space would also be limited (Figure 6). Even in the absence of PfRh2b expression, no difference in invasion and growth rates is observed, implying that a sufficient affinity still exists between the remaining ligands and their cognate receptors for efficient invasion of normal erythrocytes. Nevertheless, a large change in receptor usage does result from the loss of PfRh2b expression, indicating that a significant shift in dependency to other ligands with different erythrocyte receptor specificities has occurred. This shift in receptor usage may either result from the upregulation of ligands, existing or novel, to compensate for the missing Rh2b ligand or, alternatively, be due to the redeployment of existing ligands from a pool containing an excess of ligands. In either case, the apparent shift in the usage of pathways implies that loss of PfRh2b expression necessitated an increased reliance on other ligands to provide sufficient affinity for efficient invasion (Figure 6).
PfRh proteins function independently of each other
Previous data has suggested that PfRh2b and PfRh1 might form a functional complex (Rayner et al., 2001), and this would be consistent with the suggested association of PvRBP1 and PvRBP2 in P.vivax (Galinski et al., 1992). However, our data show that PfRh2b functions in merozoite invasion in the 3D7 parasite line in the absence of PfRh1 and PfRh2a expression. Furthermore, immunoprecipitation experiments did not co-precipitate other PfRh proteins using antibodies to PfRh2b, PfRh2a and PfRh1 (data not shown). The functional independence of the PfRh proteins is consistent with the expression of only one Py235 gene from a large gene family in a single merozoite of P.yoelii (Preiser et al., 1999). The role of a fourth member of the PfRh family, PfRh4 (Kaneko et al., 2002), in merozoite invasion remains to be determined, but it is likely that this protein is involved in specifying a different invasion pathway.
Although PfRh2b functions in merozoite invasion, we found no invasion role for the closely related protein PfRh2a in the 3D7 parasite line. PfRh2a and PfRh2b are virtually identical for a large part of the N-terminus, and differ at the C-terminus that includes a transmembrane region and a short cytoplasmic tail. Although PfRh2a does not play an important role in 3D7 parasites, it does not exclude a role in other parasite lines in a different genetic background. Indeed, attempts to disrupt the PfRh2a gene in D10 parasites, that lack PfRh2b, were unsuccessful, suggesting that the encoded protein plays an important role in this parasite line. The differences in function between PfRh2b and PfRh2a must be specified by the coding divergence in their C-termini. It is possible that the cytoplasmic tail of PfRh2b is responsible for activating signals for release of micronemes onto the erythrocyte and subsequent steps in tight junction formation (Galinski et al., 1992; Barnwell and Galinski, 1998). Alternatively, the unique region in PfRh2b N-terminal to the transmembrane domain may be responsible for interacting with a second protein that is involved in receptor binding on the erythrocyte.
Variation in expression of PfRh proteins: a selective advantage for P.falciparum
Different patterns of invasion phenotypes between isolates of P.falciparum can be explained in part by variation in expression of the PfRh protein family. This study has identified many parasite isolates that do not express PfRh2a or PfRh2b, and, similarly, 3D7 does not express detectable levels of PfRh1. We have evidence suggesting that there are many parasite isolates that do not express PfRh1 (data not shown). Loss of expression of the PfRh proteins in any merozoite is compensated by other parasite ligands interacting with available receptors. The presence of non-functional parasite ligands at the apical end of some merozoites, because of blockade by either antibodies or receptor polymorphism, decreases the efficiency of merozoite invasion. It follows that variation in expression of different parasite ligands across parasite populations would overcome this for individual merozoites, since they would lack expression of these non-functional ligands. By concentrating functional ligands at the apical end in place of PfRh2b, these merozoites could achieve the required affinity for efficient invasion. Obviously, suppression of expression of proteins such as PfRh2b or PfRh1 could result in the permanent loss of these proteins within the parasite population. However, genetic recombination during meiosis between frequent mixed infections would constantly generate a pool of parasites that either express or do not express particular PfRh proteins and invasion pathways. Hence, the parasites appear to maintain a varied repertoire of ligands, but probably concentrate on expressing a limited suite at any one time in order to achieve a threshold of binding necessary for invasion.
A number of P.falciparum isolates do not express the PfRh2a or PfRh2b proteins despite having apparently intact genes within their genomes. The mechanism for lack of expression of these proteins is not yet understood, but there are several possibilities. First, there may be mutations that block transcription or translation. Secondly, it is possible that the genes are silenced, and this would provide a mechanism to switch the expression of these genes on and off, allowing the parasite access to different invasion pathways when required. This would be analogous to the antigenic switching of the var gene family of P.falciparum (Smith et al., 1995; Su et al., 1995). Expression of the equivalent of the PfRh family in P.yoelii (Py235) is limited to only one gene per merozoite, suggesting some form of regulated control of this gene family (Preiser et al., 1999).
Biological significance for P.falciparum invasion of human erythrocytes
Multiple parasite ligands and phenotypic variation in expression of the PfRh family in P.falciparum have important implications for the parasite. Merozoite invasion requires sufficient ligand–receptor interactions on the erythrocyte surface, and there are circumstances where receptor availability is limited. First, the molecules on the erythrocyte are highly polymorphic in the human population, and this heterogeneity has probably been selected by P.falciparum and other infectious agents to protect against severe disease (Miller et al., 2002). The absence or mutation of receptors would limit those available on the erythrocyte for interaction with parasite ligands, and this would decrease invasion efficiency. Examples are populations in East Africa that lack glycophorin B, and Melanesian populations that express a mutant form of glycophorin C on the erythrocyte surface (Patel et al., 2001). Secondly, during development and aging of the human erythrocyte, different receptors such as glycophorin A are not present or vary in their quantity and presentation on the surface of this cell (Issitt and Anstee, 1998). Thirdly, host immune responses to one or more of the parasite ligands involved in merozoite invasion would block utilization of particular alternative invasion pathways, thus limiting the pathways available. Multiple parasite ligands and phenotypic variation of PfRh protein expression provide a mechanism for the parasite to evade these situations by the presence of merozoites within the population that have different dominant invasion pathway specificities, increasing the ability of the parasite to maintain infection and its potential for transmission to subsequent hosts. It will be important to consider the ability of the parasite to vary expression of parasite ligands for merozoite invasion when developing vaccine strategies directed against invasion-related proteins.
Materials and methods
Plasmids and parasites
Genomic DNA from the 3D7 parasite line was used as template in the PCR of two ~1 kb fragments corresponding to a region conserved between the PfRh2a and PfRh2b genes: Flank 1 (5′-GGACCGCCGCGGAGTCATGAGCATTTTGTTGGACAA-3′) and Flank 2n (5′-GGACCGGTTAACTTCGTCATGTATATATCCAATAGA-3′) using the primers 5′-GGACCGGGCGCCCGGGTGATGTATTATGTACAG CTAAAGC-3′ and 5′-GGACCGCCTAGGGAAAAAGCCTCTATGG ATGAAATG-3′. Similarly, two flanks from the PfRh1 gene were cloned into a vector derived from pHTk, which had a modified multiple cloning site. Flank 1 was amplified as above using the primers 5′-GATCAC TAGTTTATGAACCTACCCCTTCAT and 5′-GATCAGATCTATGA AAGTAGGTTTAATGTA, and Flank 2 was amplifed using the primers 5′-GATCGAATTCGATTTACAAGATCTCTTACT and 5′-GATCCC ATGGTTTGGATATATTTTCCCTTA.
Plasmodium falciparum asexual stages were maintained in human 0+ erythrocytes. 3D7 is a cloned line derived from NF54 and was obtained from David Walliker at Edinburgh University. W2mef is a cloned line derived from the Indochina III/CDC strain. The parasites Tak996 and Tak994 from South-East Asia, and FCB1 of African origin were provided by David Baker from the London School of Hygiene and Tropical Medicine. The FCR3 cloned line is of African origin. HB3 and 7G8 are South American cloned lines, while D10 was cloned from a Papua New Guinean isolate. Malayan Camp, K1 and Pf120 are all SouthEast Asian lines. Transfection with 80 µg of purified plasmid DNA (Qiagen) and selection for stable transfectants with double recombination crossover were carried out as described previously (Duraisingh et al., 2002).
SDS–PAGE and immunoblot analysis
Parasite pellets were collected from parasites 40 h after synchronization. Parasite supernatants were obtained from cultures following treatment of the erythrocytes with trypsin (1.0 mg/ml) and neuraminidase (25 mU/ml). Proteins were separated on 6% SDS–polyacrylamide gels. Western blotting onto nitrocellulose (0.45 µm; Schleicher and Schuell, Germany) was performed according to standard protocols.
Erythrocyte invasion assay
Uninfected erythrocytes were treated with enzymes as described previously (Thompson et al., 2001). The invasion assay was carried out essentially as previously described (Reed et al., 2000). Erythrocytes infected with ring stages were synchronized twice, followed by pre-treatment with trypsin and neuraminidase to prevent reinvasion. Experiments were carried out with the addition of new erythrocytes treated with trypsin (66.7 µg/ml), neuraminidase (66.7 mU/ml) and chymotrypsin (1.0 mg/ml) or a combination of these enzyme treatments (Thompson et al., 2001). Enzyme-treated or normal erythrocytes at 4% hematocrit were inoculated with infected erythrocytes to give a parasitemia of 0.5% and hematocrit of 4% in a volume of 100 µl. Parasites were incubated for 48 h followed by addition of [3H]hypoxanthine (Amersham) to 1 µCi/well. After 16 h, the cells were harvested onto glass filters with a cell harvester (Packard). Incorporated [3H]hypoxanthine was determined in a scintillation counter. Percentage invasion was calculated in comparison with invasion of the same parasite line into untreated erythrocytes, which was set to 100%.
Antibody inhibition assay
Antibodies were purified using protein G. Antibody inhibition assays were performed in the same way as the erythrocyte invasion assay except that the hematocrit was kept at 2% with an initial parasitemia of 0.5%. Antibodies were added to a final concentration of 0.5 mg/ml 24 h following the setting up of the assay, prior to reinvasion. Invasion in the presence of specific anti-PfRh2a/b antibodies was compared with invasion in the presence of control antibodies from pre-immune serum.
Electron microscopy
Immunolabeling for electron microscopy was performed on ultrathin sections of glutaraldehyde-fixed parasites as described previously (Healer et al., 2002). Micrographs were obtained using a Philips CM120 BioTWIN transmission electron microscope at 120 kV.
Acknowledgements
We thank the Red Cross Blood Service (Melbourne, Australia) for supply of red cells and serum, and Dr Brendan Crabb and Dr Mark Wickham for critical reading of the manuscript. This work was supported by the National Health and Medical Research Council of Australia. M.T.D. is funded by a Wellcome Trust Advanced Training Fellowship (Tropical Medicine). S.A.R. is funded by a Melbourne Research Scholarship. G.I.M. and A.F.C. are Howard Hughes International Scholars.
References
- Adams J.H., Blair,P.L., Kaneko,O. and Peterson,D.S. (2001) An expanding ebl family of Plasmodium falciparum. Trends Parasitol., 17, 297–299. [Abstract] [Google Scholar]
- Baldi D.L., Andrews,K.T., Waller,R.S., Roos,D., Crabb,B.S. and Cowman,A.F. (2000) RAP1 controls rhoptry targeting of RAP2 in the malaria parasite Plasmodium falciparum. EMBO J., 19, 1–9. [Europe PMC free article] [Abstract] [Google Scholar]
- Barnwell J.W. and Galinski,M.R. (1998) Invasion of vertebrate cells: erythrocytes. In Sherman,I.W. (ed.), Malaria: Parasite Biology, Pathogenesis and Protection. ASM Press, Washington, DC, pp. 193–120.
- Butcher G.A., Mitchell,G.H. and Cohen,S. (1973) Mechanism of host specificity in malarial infection. Nature, 244, 40–41. [Abstract] [Google Scholar]
- Dolan S.A., Miller,L.H. and Wellems,T.E. (1990) Evidence for a switching mechanism in the invasion of erythrocytes by Plasmodium falciparum. J. Clin. Invest., 86, 618–624. [Europe PMC free article] [Abstract] [Google Scholar]
- Dolan S.A., Proctor,J.L., Alling,D.W., Okubo,Y., Wellems,T.E. and Miller,L.H. (1994) Glycophorin B as an EBA-175 independent Plasmodium falciparum receptor of human erythrocytes. Mol. Biochem. Parasitol., 64, 55–63. [Abstract] [Google Scholar]
- Duraisingh M.T., Triglia,T. and Cowman,A.F. (2002) Negative selection of Plasmodium falciparum reveals targeted gene deletion by double crossover recombination. Int. J. Parasitol., 32, 81–89. [Abstract] [Google Scholar]
- Florens L. et al. (2002) A proteomic view of the Plasmodium falciparum life cycle. Nature, 419, 520–526. [Abstract] [Google Scholar]
- Galinski M.R., Medina,C.C., Ingravallo,P. and Barnwell,J.W. (1992) A reticulocyte-binding protein complex of Plasmodium vivax merozoites. Cell, 69, 1213–1226. [Abstract] [Google Scholar]
- Galinski M.R., Mengyao,X. and Barnwell,J.W. (2000) Plasmodium vivax reticulocyte binding protein-2 (PvRBP-2) shares structural features with PvRBP-1 and the Plasmodium yoelii 235kDa rhoptry protein family. Mol. Biochem. Parasitol., 108, 257–262. [Abstract] [Google Scholar]
- Gratzer W.B. and Dluzewski,A.R. (1993) The red blood cell and malaria parasite invasion. Semin. Hematol., 30, 232–247. [Abstract] [Google Scholar]
- Healer J., Crawford,S., Ralph,S., McFadden,G. and Cowman,A.F. (2002) Independent translocation of two micronemal proteins in developing Plasmodium falciparum merozoites. Infect. Immun., 70, 5751–5758. [Europe PMC free article] [Abstract] [Google Scholar]
- Howard R.J., Haynes,J.D., McGinniss,M.H. and Miller,L.H. (1982) Studies on the role of red blood cell glycoproteins as receptors for invasion by Plasmodium falciparum merozoites. Mol. Biochem. Parasitol., 6, 303–315. [Abstract] [Google Scholar]
- Issitt P.D. and Anstee,D.J. (1998) Applied Blood Group Serology. Montgomery Scientific Publications, Durham.
- Kaneko O., Mu,J., Tsuboi,T., Su,X. and Torii,M. (2002) Gene structure and expression of a Plasmodium falciparum 220-kDa protein homologous to the Plasmodium vivax reticulocyte binding proteins. Mol. Biochem. Parasitol., 121, 275–278. [Abstract] [Google Scholar]
- Khan S.M., Jarra,W. and Preiser,P.R. (2001) The 235 kDa rhoptry protein of Plasmodium (yoelii) yoelii: function at the junction. Mol. Biochem. Parasitol., 117, 1–10. [Abstract] [Google Scholar]
- Mayer D.C., Kaneko,O., Hudson-Taylor,D.E., Reid,M.E. and Miller,L.H. (2001) Characterization of a Plasmodium falciparum erythrocyte-binding protein paralogous to EBA-175. Proc. Natl Acad. Sci. USA, 98, 5222–5227. [Europe PMC free article] [Abstract] [Google Scholar]
- Miller L.H., McAuliffe,F.M. and Mason,S.J. (1977) Erythrocyte receptors for malaria merozoites. Am. J. Trop. Med. Hyg., 26, 204–208. [Abstract] [Google Scholar]
- Miller L.H., Baruch,D.I., Marsh,K. and Doumbo,O.K. (2002) The pathogenic basis of malaria. Nature, 415, 673–679. [Abstract] [Google Scholar]
- Narum D.L., Fuhrmann,S.R., Luu,T. and Sim,B.K. (2002) A novel Plasmodium falciparum erythrocyte binding protein-2 (EBP2/BAEBL) involved in erythrocyte receptor binding. Mol. Biochem. Parasitol., 119, 159–168. [Abstract] [Google Scholar]
- Ogun S.A. and Holder,A.A. (1996) A high molecular mass Plasmodium yoelii rhoptry protein binds to erythrocytes. Mol. Biochem. Parasitol., 76, 321–324. [Abstract] [Google Scholar]
- Okoyeh J.N., Pillai,C.R. and Chitnis,C.E. (1999) Plasmodium falciparum field isolates commonly use erythrocyte invasion pathways that are independent of sialic acid residues of glycophorin A. Infect. Immun., 67, 5784–5791. [Europe PMC free article] [Abstract] [Google Scholar]
- Pasvol G. (1984) Receptors on red cells for Plasmodium falciparum and their interaction with merozoites. Philos. Trans. R. Soc. Lond. B Biol. Sci., 307, 189–200. [Abstract] [Google Scholar]
- Pasvol G., Jungery,M., Weatherall,D.J., Parsons,S.F., Anstee,D.J. and Tanner,M.J.A. (1982) Glycophorin as a possible receptor for Plasmodium falciparum. Lancet, 2, 947–950. [Abstract] [Google Scholar]
- Patel S.S., Mehlotra,R.K., Kastens,W., Mgone,C.S., Kazura,J.W. and Zimmerman,P.A. (2001) The association of the glycophorin C exon 3 deletion with ovalocytosis and malaria susceptibility in the Wosera, Papua New Guinea. Blood, 98, 3489–3491. [Abstract] [Google Scholar]
- Preiser P.R., Jarra,W., Capiod,T. and Snounou,G. (1999) A rhoptry-protein-associated mechanism of clonal phenotypic variation in rodent malaria. Nature, 398, 618–622. [Abstract] [Google Scholar]
- Rayner J.C., Galinski,M.R., Ingravallo,P. and Barnwell,J.W. (2000) Two Plasmodium falciparum genes express merozoite proteins that are related to Plasmodium vivax and Plasmodium yoelii adhesive proteins involved in host cell selection and invasion. Proc. Natl Acad. Sci. USA, 97, 9648–9653. [Europe PMC free article] [Abstract] [Google Scholar]
- Rayner J.C., Vargas-Serrato,E., Huber,C.S., Galinski,M.R. and Barnwell,J.W. (2001) A Plasmodium falciparum homologue of Plasmodium vivax reticulocyte binding protein (PvRBP1) defines a trypsin-resistant erythrocyte invasion pathway. J. Exp. Med., 194, 1571–1581. [Europe PMC free article] [Abstract] [Google Scholar]
- Reed M.B., Caruana,S.R., Batchelor,A.H., Thompson,J.K., Crabb,B.S. and Cowman,A.F. (2000) Targeted disruption of an erythrocyte binding antigen in Plasmodium falciparum is associated with a switch toward a sialic acid independent pathway of invasion. Proc. Natl Acad. Sci. USA, 97, 7509–7514. [Europe PMC free article] [Abstract] [Google Scholar]
- Sim B.K.L., Chitnis,C.E., Wasniowska,K., Hadley,T.J. and Miller,L.H. (1994) Receptor and ligand domains for invasion of erythrocytes by Plasmodium falciparum. Science, 264, 1941–1944. [Abstract] [Google Scholar]
- Smith J.D., Chitnis,C.E., Craig,A.G., Roberts,D.J., Hudson-Taylor,D.E., Peterson,D.S., Pinches,R., Newbold,C.I. and Miller,L.H. (1995) Switches in expression of Plasmodium falciparum var genes correlate with changes in antigenic and cytoadherent phenotypes of infected erythrocytes. Cell, 82, 101–110. [Abstract] [Google Scholar]
- Su X.Z., Heatwole,V.M., Wertheimer,S.P., Guinet,F., Herrfeldt,J.A., Peterson,D.S., Ravetch,J.A. and Wellems,T.E. (1995) The large diverse gene family var encodes proteins involved in cytoadherence and antigenic variation of Plasmodium falciparum-infected erythrocytes. Cell, 82, 89–100. [Abstract] [Google Scholar]
- Taylor H.M., Triglia,T., Thompson,J., Sajid,M., Fowler,R., Wickham,M.E., Cowman,A.F. and Holder,A.A. (2001) Plasmodium falciparum homologue of the genes for Plasmodium vivax and Plasmodium yoelii adhesive proteins, which is transcribed but not translated. Infect. Immun., 69, 3635–3645. [Europe PMC free article] [Abstract] [Google Scholar]
- Taylor H.M., Grainger,M. and Holder,A.A. (2002) Variation in the expression of a Plasmodium falciparum protein family implicated in erythrocyte invasion. Infect. Immun., 70, 5779–5789. [Europe PMC free article] [Abstract] [Google Scholar]
- Thompson J.K., Triglia,T., Reed,M.B. and Cowman,A.F. (2001) A novel ligand from Plasmodium falciparum that binds to a sialic acid-containing receptor on the surface of human erythrocytes. Mol. Microbiol., 41, 47–58. [Abstract] [Google Scholar]
- Triglia T., Thompson,J., Caruana,S.R., Delorenzi,M., Speed,T. and Cowman,A.F. (2001) Identification of proteins from Plasmodium falciparum that are homologous to reticulocyte binding proteins in Plasmodium vivax. Infect. Immun., 69, 1084–1092. [Europe PMC free article] [Abstract] [Google Scholar]
Articles from The EMBO Journal are provided here courtesy of Nature Publishing Group
Full text links
Read article at publisher's site: https://doi.org/10.1093/emboj/cdg096
Read article for free, from open access legal sources, via Unpaywall:
https://europepmc.org/articles/pmc150330?pdf=render
Citations & impact
Impact metrics
Citations of article over time
Alternative metrics
Article citations
Application of optical tweezer technology reveals that PfEBA and PfRH ligands, not PfMSP1, play a central role in Plasmodium falciparum merozoite-erythrocyte attachment.
PLoS Pathog, 20(9):e1012041, 23 Sep 2024
Cited by: 0 articles | PMID: 39312588 | PMCID: PMC11449297
Patterns of Heterochromatin Transitions Linked to Changes in the Expression of Plasmodium falciparum Clonally Variant Genes.
Microbiol Spectr, 11(1):e0304922, 14 Dec 2022
Cited by: 5 articles | PMID: 36515553 | PMCID: PMC9927496
Epigenetic and Epitranscriptomic Gene Regulation in Plasmodium falciparum and How We Can Use It against Malaria.
Genes (Basel), 13(10):1734, 27 Sep 2022
Cited by: 8 articles | PMID: 36292619 | PMCID: PMC9601349
Review Free full text in Europe PMC
Phenotypic characterization of Ghanaian P. falciparum clinical isolates reveals a homogenous parasite population.
Front Immunol, 13:1009252, 23 Sep 2022
Cited by: 0 articles | PMID: 36211335 | PMCID: PMC9537689
The Cellular and Molecular Interaction Between Erythrocytes and Plasmodium falciparum Merozoites.
Front Cell Infect Microbiol, 12:816574, 31 Mar 2022
Cited by: 7 articles | PMID: 35433504 | PMCID: PMC9008539
Review Free full text in Europe PMC
Go to all (186) article citations
Data
Similar Articles
To arrive at the top five similar articles we use a word-weighted algorithm to compare words from the Title and Abstract of each citation.
Reticulocyte-binding protein homologue 1 is required for sialic acid-dependent invasion into human erythrocytes by Plasmodium falciparum.
Mol Microbiol, 55(1):162-174, 01 Jan 2005
Cited by: 113 articles | PMID: 15612925
Plasmodium falciparum reticulocyte binding-like homologue protein 2 (PfRH2) is a key adhesive molecule involved in erythrocyte invasion.
PLoS One, 6(2):e17102, 28 Feb 2011
Cited by: 49 articles | PMID: 21386888 | PMCID: PMC3046117
Identifying Plasmodium falciparum merozoite surface protein-10 human erythrocyte specific binding regions.
Biochimie, 87(5):461-472, 01 May 2005
Cited by: 16 articles | PMID: 15820753
Invasion of red blood cells by malaria parasites.
Cell, 124(4):755-766, 01 Feb 2006
Cited by: 539 articles | PMID: 16497586
Review