Abstract
Free full text

Primary rat muscle progenitor cells have decreased proliferation and myotube formation during passages
Abstract
Abstract. Adult skeletal muscle contains populations of satellite cells and muscle‐derived stem cells that are capable of forming multinucleate myotubes. The purpose of this study was to determine the phenotype of cells isolated from a common satellite cell isolation and passaging procedure from whole skeletal muscle. To ascertain the characteristics of the cellular phenotype, the myogenic markers MyoD and desmin, the satellite‐cell‐specific marker Pax7, and the haemopoietic stem cell markers CD34 and CD45 were examined by immunohistochemical analysis. Immediately after isolation, > 90% myogenic marker‐positive cells were positive for desmin, MyoD and Pax7. In contrast, ~10% of the isolated cells expressed only CD34 or CD45. After three passages, the percentage of cells that were positive for the myogenic markers desmin, MyoD and Pax7 was reduced to ~55%, while the population of CD34‐ or CD45‐positive cells increased to ~30% after the third passage. Immunohistochemical detection of bromodeoxyuridine demonstrated that the number of proliferating cells decreased progressively after each passaging. Finally, after the third passage the percentage of nuclei in myotubes decreased from 46.7% to 12.5%. Since passaging of muscle progenitor cells is common practice, the results of the current report suggest that characterization of cell heterogeneity needs to be made frequently.
INTRODUCTION
Satellite cells are a population of mononuclear myogenic precursors, first described by Mauro (1961) as wedged between the sarcolemma and the basal lamina of the skeletal muscle fibre. Satellite cells in adult skeletal muscle are mitotically quiescent; however, in response to stimuli such as injury or mechanical loads, satellite cells become activated, proliferate, and fuse to existing muscle fibres or fuse together to form new myofibres for the regeneration of damaged skeletal muscle (Schultz & McCormick 1994). Based on their ability to proliferate and fuse, satellite cells are now considered a subpopulation of muscle stem/progenitor cells (Hawke & Garry 2001). Although satellite cells are accepted as the major source of new myonuclei, recent data indicate that skeletal muscle tissue contains a subpopulation of muscle progenitor cells that express markers that are typically associated with haemopoietic stem cells (Seale & Rudnicki 2000). Most large‐scale isolation techniques from whole skeletal muscle for culturing of skeletal muscle progenitor cells result in a heterogeneous population of cells. One commonly used method to enhance the number of cells obtained from skeletal muscle that express the muscle‐specific marker desmin, is by pre‐plating the isolated cells in non‐collagen‐coated and collagen‐coated flasks (Yaffe & Saxel 1977). Qu et al. (1998) used serial pre‐plating to enrich the heterogeneous isolated population for muscle progenitor cells. The technique described by Qu et al. (1998) employed multiple time‐dependent pre‐plates with PrePlate 1 (PP1) representing a population of skeletal muscle‐derived cells that adhered in the first hour after isolation from muscle, PP2 representing those that adhered in the next 2 h, PP3 representing those that adhered in the next 18 h, and the subsequent pre‐plates being obtained at 24‐h intervals (PP4–PP6) with a resultant enhancement of the percentage of desmin‐positive cells from PP2 = 14%; PP3 = 25%; PP4 = 72%; PP5 = 75%; to PP6 = 78%. If the goal of pre‐plating is ex vivo gene therapy, a desirable outcome may be to amplify cell number by passaging to obtain sufficient cells for biological significance after injection back into the muscle. For example, Deasy et al. (2001) state that ex vivo expansion of a muscle‐derived stem cell population by passaging is essential to obtain a suitable number of cells for engraftment. Others have passaged cells to achieve sufficient protein for multiple experimental assays (Rando & Blau 1994; Edom‐Vovard et al. 1999; Sabourin et al. 1999; Chakravarthy et al. 2000; Gaster et al. 2001; Michal et al. 2002; Spangenburg & Booth 2002; Machida et al. 2003). Because of the wide use of passage techniques to study cellular and molecular mechanisms involved in adult myogenesis, the whole muscle approach allows a more representative estimate of the entire muscle than does a single‐fibre source. However, no data exist describing the cellular phenotype of primary satellite cells after multiple passages. Thus, primary muscle progenitor cells were isolated from whole skeletal muscle and the cell phenotype was assessed during three passages using several markers for satellite cell or stem cell phenotype. The goal was to discover whether cells isolated from a commonly employed method of satellite cell isolation would retain the initial phenotype after passaging, and whether also a phenotype of a highly proliferative cell was maintained, with myogenic potential for gene transfer to muscle. The hypothesis posed was that passaging of the isolated rat primary progenitor muscle cells would not alter their heterogeneous composition, proliferation, or ability to form myotubes.
MATERIALS AND METHODS
Materials
Cell culture medium and reagents were purchased from Life Technologies, Inc. (Rockville, MD). 5‐bromo‐2′‐deoxyuridine‐5′‐monophosphate (BrdUrd) was obtained from Sigma (St Louis, MO). Mouse monoclonal antibody to BrdUrd was obtained from Roche Diagnostics (Indianapolis, IN). The antibody against monoclonal anti‐CD34 (sc‐7324) was obtained from Santa Cruz (Santa Cruz, CA), and monoclonal anti‐MyoD (MoAb5.8A) was purchased from Pharmingen (San Diego, CA). Monoclonal anti‐CD45 (550566) was obtained from Transduction Laboratories (Lexington, KY) and D3 mouse monoclonal anti‐desmin, monoclonal anti‐Pax7 and MF20 mouse monoclonal anti‐sarcomeric myosin antibodies were obtained from the Developmental Studies Hybridoma Bank (Iowa City, IA). Matrigel™ Basement Membrane Matrix was purchased from BD Biosciences (Bedford, MA); normal goat serum, mouse immunoglobulin G (IgG), and rabbit IgG were purchased from Jackson (West Grove, PA); and Vectastain Elite ABC kit and peroxidase substrate kit (VECTOR VIP) were obtained from Vector Laboratories (Burlingame, CA).
Animals
Pathogen‐free, F1 generation of Fisher 344 × Brown Norway male rats (n = 5) were obtained from the National Institutes of Health ageing program (Harlan, Indianapolis, IN) at the age of 2 months. All animals were housed at 21 °C in a 12‐h light/12‐h dark cycle. Rat chow and water were provided ad libitum. The animals were allowed to acclimatize to their new surroundings for 2 weeks before the cell isolation procedures were performed.
Isolation of satellite cells
Satellite cells were isolated from the major hind‐limb muscles of the rats according to previously described methods modified from Allen et al. (1997). Briefly, animals were anaesthetized with a single intraperitoneal injection of a cocktail consisting of 75 mg/ml ketamine, 3 mg/ml xylazine, and 5 mg/ml acepromazine (2.0 ml/kg body weight). The muscles were removed, trimmed of excess connective tissue and fat, hand minced, and digested for 1 h at 37 °C with 1.25 mg/ml pronase (Sigma). Cells were separated from muscle fibre fragments and tissue debris by differential centrifugation and were plated on an uncoated dish in Dulbecco's modified Eagle's minimal essential medium (DMEM) containing 10% horse serum and 1% penicillin/streptomycin antibiotic mixture for 2 h. Unattached cells floating in the medium after 2 h incubation were then transferred onto a fresh uncoated dish. After 24 h, the floating cells were collected by centrifugation at 2000 g for 3 min, and the resultant pellet was resuspended in high serum medium [Ham's F‐10 nutrient mixture (F‐10) containing 20% fetal bovine serum, 1% chicken embryo extract, 1% penicillin/streptomycin antibiotic mixture, and 1%l‐glutamine] on a rat‐tail collagen (Sigma)‐coated dish. The growth medium (Ham's F‐10 nutrient mix) was selected based on the fact that this, compared to DMEM, provided optimal growth conditions as well as ensuring myogenic enrichment. Ham's F‐10 medium prevented myoblast differentiation and minimized fibroblast overgrowth (data not shown), which was consistent with the observation of Rando & Blau (1994). To further ensure high myogenic content in all cultures, a pre‐plating technique was used (Richler & Yaffe 1970) in which these cells were temporarily placed for 30 min on an uncoated dish at each passaging step to further enrich for myoblasts. All cultures were maintained at 37 °C in a humid air atmosphere containing 6% CO2.
Immunohistochemistry
To determine percentages of cells expressing specific marker proteins, cells were seeded at 10 000 cells/well on collagen‐coated glass chamber slides (Laboratory‐Tek II, Nalgene, Nunc Rochester, NY, UK) in 500 µl F‐10 with high serum, for 48 h. After this incubation, cells were rinsed three times with phosphate‐buffered saline (PBS), and then fixed in methanol containing 0.6% hydrogen peroxide for 20 min at 4 °C. The cells were then incubated sequentially in PBS followed by the appropriate blocking solution for each antibody (also in PBS) for 30 min (Table 1). Specimens were then incubated with diluted primary antibodies at 4 °C for 16 h at the appropriate dilution in PBS containing 2% bovine serum albumin (Table 1). Following thorough rinsing with PBS, cells were incubated with either biotinylated goat anti‐mouse IgG, or biotinylated goat anti‐rabbit IgG secondary antibodies (1 : 250, Vector Laboratories) for 30 min. Vectastain Elite ABC kit (Vector Laboratories) was used for detection of primary antibodies. Negative control staining was performed using an identical procedure, with non‐specific mouse IgG or rabbit IgG instead of each primary antibody. Nuclear staining for DNA was performed with methyl green solution. To identify the population of cells which expressed several markers for the isolated muscle progenitor cells, immunohistochemical experiments were carried out using cells evaluated at identical time‐points, from seeding to time of fixation, to control for differences in expression that may have arisen with time in culture. Three independent experiments in each condition were performed and a total of at least 100 cells was counted for each specimen.
Table 1
Antibodies used in this study
Antibody | Monoclonal/ Polyclonal | Blocking | Primary dilution | Secondary | Phenotype | Reference |
---|---|---|---|---|---|---|
Desmin | Mono | NGS | 1 : 3 | anti‐mouse IgG | myogenic cell | Allen et al. 1991 |
MyoD | Mono | NGS | 1 : 100 | anti‐mouse IgG | myogenic cell | Sabourin et al. 1999 |
Pax7 | Mono | NGS | 1 : 1 | anti‐mouse IgG | satellite cell | Seale et al. 2000 |
CD34 | Mono | NGS | 1 : 50 | anti‐rabbit IgG | haemopoietic stem cell | Krause et al. 1994 |
CD45 | Mono | NGS | 1 : 50 | anti‐rabbit IgG | haemopoietic stem cell | Woollett et al. 1985 |
Mono., monoclonal.
Blocking: NGS, 10% normal goat serum + 0.1% Triton‐X in 2% bovine serum albumin.
BrdUrd assay
Cultures were pulse‐labelled for 1 h with 10 µm BrdUrd, followed by immunocytochemistry for detection of BrdUrd according to the manufacturer's directions. The percentage of BrdUrd‐labelled cells was used as an indicator of activating and proliferating cells.
Differentiation assay
To induce differentiation, unpassaged cells and cells passaged three times were plated at 25 000 cells/cm2 on Matrigel (1 : 50 dilution)‐coated glass chamber slides (Laboratory‐Tek II, Nunc) in F‐10 medium at high serum concentration. After 48 h, the cells were washed twice with DMEM and then incubated with DMEM containing 2% horse serum (differentiation medium) for 5 days, with the medium changed daily. Differentiation of the isolated cells was determined by sarcomeric myosin expression (Allen et al. 1991). Efficiency of differentiation was quantified by counting the total number of nuclei in myosin‐expressing myotubes as a percentage of the total number of nuclei present on the plate. At least 600 cells were counted for each sample.
Statistics
Data are shown as means ± SE and were analysed using a one‐way analysis of variance (anova), with the statistical significance of differences between group means being assessed by Tukey's multiple comparison test. P < 0.05 was considered to denote statistical significance.
RESULTS
At the time of their isolation, most muscle progenitor cells had myogenic cell markers. Representative immunostaining for each marker at the time of isolation is shown in Fig. 1. Immunohistochemical detection clearly revealed numerous myogenic cells positive for desmin (Fig. 1a,a′), MyoD (Fig. 1b,b′), and Pax7 (Fig. 1c,c′) in freshly isolated cells. MyoD and Pax7 were present in the nuclei of the cells just isolated (Fig. 1b′c′). As a percentage, > 90% of the freshly isolated cells contained both myogenic and satellite cell markers (Table 2). Cells that expressed myogenic and satellite markers exhibited either bipolar morphology or non‐bipolar morphology. In contrast, cells that were CD34‐ or CD45‐positive (Fig. d,e) were found to be smaller, circular, non‐bipolar cells after isolation and comprised ~10% of the total isolate (Table 2). At no time did cells that exhibited bipolar morphology express CD34 or CD45.
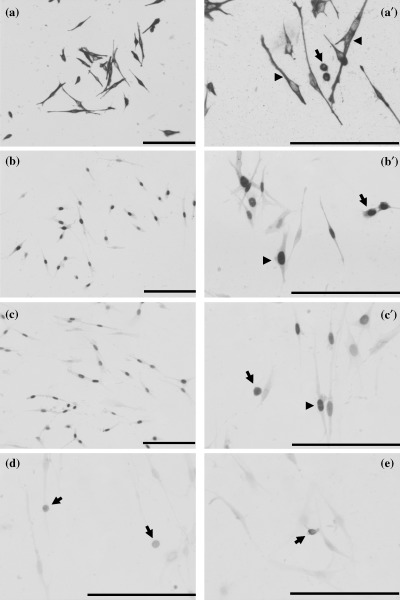
Immunohistochemical analyses with markers for myogenic, satellite cell and haemopoietic stem cells in primary muscle progenitor cells isolated from adult rats at the time of isolation. Desmin (a,a′) and MyoD (b,b′) were used as myogenic markers, Pax7 (c,c′) is shown as a satellite cell marker, CD34 (d) and CD45 (e) were used as haemopoietic stem cell markers. Desmin is expressed in the cytoplasm of isolated bipolar cells (a′, arrowheads) and the smaller, circular, non‐bipolar cells (a′, arrows). MyoD and Pax7 are present in the nuclei of the bipolar cells (b′,c′, arrowheads) and the smaller, circular, non‐bipolar cells (b′,c′, arrows). CD34 or CD45 are found in the smaller, circular, non‐bipolar cells (d,e, arrows), but not in the isolated bipolar cells. Myotubes were never observed, verifying that these markers were associated with muscle precursor cells and not with differentiating cells. Bars indicate 500 µm.
Table 2
Phenotype of primary rat muscle precursor cells at the time of isolation
Antibody | % Positive cell |
---|---|
Desmin | 92.8 (2.2) |
MyoD | 90.6 (0.6) |
Pax7 | 90.1 (0.9) |
CD34 | 10.6 (0.3) |
CD45 | 12.4 (3.6) |
The data are mean (SE). The SE were calculated from three independent experiments. A total of at least 100 cells were counted in each sample.
Passaging decreased the percentage of cells with myogenic and satellite cell markers. After each passage, the percentage of desmin‐, MyoD‐ and Pax7‐positive cells decreased gradually until ~55% of the myogenic cells were positive for myogenic and satellite cell markers at the third passage (Fig. 2). On the other hand, the percentage of CD34‐ or CD45‐positive cells gradually increased to ~30% after the third passage (Fig. 2).

The expression profiles of myogenic, satellite cell, and haemopoietic stem cell markers during passaging of rat primary muscle progenitor cells. A total of at least 100 cells was counted in each sample. The data are means ± SE. The SE was calculated from three independent experiments performed on separate sets of animals. The changes in the percentage of cells expressing desmin, MyoD, Pax7, CD34 and CD45 between unpassaged cells (#0 on the x‐axis) and the third passage group (#3 on the x‐axis) were significantly different (*P < 0.05).
Proliferation of the primary rat muscle precursor cells decreased during passaging. To evaluate cell proliferation at each passage, BrdUrd incorporation was examined by immunohistochemical analysis. Immunohistochemical detection of BrdUrd‐positive cells demonstrated that the percentage of proliferating cells decreased progressively after each passage (Fig. 3).
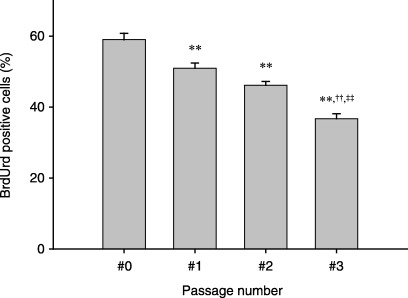
Effect of passaging on rat primary muscle progenitor cell proliferation. Muscle progenitor cell activation was assayed by BrdUrd incorporation in the adult rat muscle precursor cell culture. Cells were pulse‐labelled for 1 h with 10 µm BrdUrd before staining. The percentage of BrdUrd‐labelled cells was used as an indicator of activation and proliferating cells. Error bars represent the SE from three independent experiments performed on separate sets of animals. A total of at least 100 cells was counted in each sample. Significantly (P < 0.01) different from **unpassaged cells (#0 on the x‐axis), ††one passage (#1 on the x‐axis), and ‡‡two passage (#2 on the x‐axis) groups.
The myogenic potential in culture of isolated progenitor cells decreases during passaging. To evaluate myogenic potential in the isolate at the third passage, sarcomeric myosin protein (a marker of the differentiated state) expression was examined by immunohistochemical analysis. Myotubes derived from unpassaged cells were large and contained numerous nuclei (Fig. 4a); percentage of nuclei in the myotubes with no passaging was 46.7%. In contrast, small myotubes formed from the cells after three passages, with a significant reduction in the number of myonuclei present (Fig. 4b). Only 12.5% of total nuclei were present within the myosin‐expressing myotubes. These data indicate that the myogenic potential of isolated progenitor cells was decreased by the third passage compared to myogenic potential of cells immediately after isolation.

Differentiation potential of isolated progenitor cells decreases during passaging. Representative photograph of myotubes derived from unpassaged cells (a) and cells passaged three times (b) stained with a monoclonal antibody specific for sarcomeric myosin (MF20). The myotubes derived from unpassaged cells were large and contained numerous nuclei (a). In contrast, myotubes derived from three‐passaged cells were small with fewer incorporated nuclei (b).
DISCUSSION
A number of workers (Rando & Blau 1994; Edom‐Vovard et al. 1999; Sabourin et al. 1999; Gaster et al. 2001; Spangenburg et al. 2002; Michal et al. 2002; Machida et al. 2003) have employed multiple passages of muscle progenitor cells for their assays. Consequently in the current experiment, we wished to determine whether the characteristics of a heterogeneous population of muscle progenitor cells changed as they were passaged. The results indicated that from isolation through to the third passage, there was a decline in the percentage of cells with myogenic/satellite cell markers, proliferation rates, and differentiation potential.
Using the procedures of the current experiment, ~90% of freshly isolated muscle progenitor cells were classified as satellite cells, based on the expression of known markers (desmin, MyoD and Pax‐7), while ~10% of the cells expressed markers consistent with haemopoietic cell types (CD34 and CD45). However, after three passages, the percentage of satellite cells progressively declined to ~55% (based on the reduced percentage of cells expressing the known myogenic/satellite cell markers), while the percentage of cells expressing the canonical haemopoietic markers rose to ~30%. These observations suggest two things. First, the heterogeneity of the cell population should be characterized at the point of utilization rather than only immediately after isolation and second, if the purpose of the experiment is to examine cells with satellite cell markers, then freshly isolated cells, in our hands, provide a heterogeneous population composed more predominantly of satellite cells with fewer haemopoietic cells.
Cells with haemopoietic markers
CD34 and CD45 are established markers of haemopoietic stem cells (Gratama et al. 1998). Cells expressing CD34 or CD45 in skeletal muscle may possess the ability to differentiate into haemopoietic, myogenic, osteogenic, adipogenic, chondrogenic, neural, or endothelial cells (Seale & Rudnicki 2000; Torrente et al. 2001; Asakura et al. 2002; Deasy & Huard 2002). The percentage of cells expressing CD34 and CD45 progressively rose from ~10% to ~30% from isolation to passage 3. Qu‐Petersen et al. (2002) noted that mouse‐muscle‐derived stem cells, which express CD34, might divide many more times than satellite cells and thus it is possible that the CD34 and CD45 cells proliferate more than cells not expressing these proteins.
Cells with markers for satellite cells
Seale et al. (2000) identified that the Paired box transcription factor, Pax7, was expressed selectively in quiescent and proliferating satellite cells. In addition, Pax7 knock‐out skeletal muscle had no satellite cells, which Seale et al. (2000) interpreted to mean that the induction of Pax7 in muscle‐derived pluripotent stem cells induced satellite cell specification by restricting alternate developmental programmes. In the current study, although there were > 90% of cells positive for Pax7 at the time of isolation, the population of Pax7‐positive cells decreased gradually to ~55% during passaging.
Cells with myogenic markers
At the time of isolation (48 h after cells were seeded), > 90% of the cells were positive for MyoD and desmin, which are considered to be markers of cells with myogenic potential (Allen et al. 1991; Sabourin et al. 1999), but not to be indicators of terminal differentiation whereas proteins such as myogenin or myosin heavy chain are utilized (Smith et al. 1994; Cornelison & Wold 1997; Sabourin et al. 1999). While desmin does not distinguish between different types of muscle [skeletal, cardiac, and smooth muscle (Kubin et al. 2003; Stiebellehner et al. 2003)], two of which may be in the culture, the similar percentages of desmin, MyoD, and Pax7 (Fig. 2) suggest that at least in the current experiment, contamination by smooth muscle cells was not significant. On the other hand, MyoD is specific to proliferating skeletal myoblasts (Molkentin & Olson 1996). The decrease in the percentage of cells expressing MyoD protein suggests that a smaller proportion of cells at the third passage were proliferating. Indeed, this was confirmed by decreases in BrdUrd measured with each passage. Proliferating satellite cells as measured by [3H]thymidine or BrdUrd incorporation, have been identified immunohistochemically to have coexpression of MyoD and desmin (Kaufman & Foster 1988; Allen et al. 1991; Bockhold et al. 1998; Lawson‐Smith & McGeachie 1998). In addition, Yablonka‐Reuveni & Rivera (1994) reported that the temporal appearance and number of cells positive for proliferating cell nuclear antigen expression or for MyoD proteins were similar, suggesting that MyoD was present in detectable amounts in proliferating, but not in quiescent, satellite cells. Decline in differentiation into myotubes from cell isolation to passage 3 was unexpected. The only previous study, located in the literature after the completion of the current study, showed a decrease in cell division and myotube formation from the 5th to the 7th passage of cloned porcine myogenic satellite cells (Fligger et al. 1998). One potential explanation for the these findings is that CD34‐ or CD45‐positive cells are not able to differentiate into myotubes in culture (yet retain the ability to differentiate into skeletal muscle fibres after transplantation) (Torrente et al. 2001; Asakura et al. 2002; Jankowski et al. 2002; Tamaki et al. 2002). Since the CD34 or CD45 cells increased to 30% of the population during the first three passages, it can be speculated that this increase may have contributed, in part, to the decrease in myogenic differentiation. However, CD34+/45− cells have been shown to have the potential to differentiate into adipocytes, or endothelial or myogenic cells in culture (Tamaki et al. 2002).
In summary, the hypothesis posed in the current study was not upheld, in that passaging of isolated rat primary progenitor muscle cells did reduce the number of myogenic cells and the differentiation potential of these cells in culture. However, in our hands, the percentage of cells expressing CD34 and CD45 progressively increased from 10% to 30% over three passages, which was associated with a stepwise decrease in cell proliferation and in the proportion of cells expressing markers for satellite cells, suggesting that these cells were becoming more quiescent with passaging. These observations suggest that characterization of passaged cells should not be made immediately upon their isolation, but at the passage employed for assay, or that freshly isolated cells should be used.
ACKNOWLEDGEMENTS
The authors wish to thank Dr Yablonka‐Reuveni for helpful discussion and advice with this study. This research was supported by NIH grant AG‐18780 (F.W.B.) and fellowship AR‐48514 (E.E.S.).
Notes
‡ Present address: Exercise Biology Program, UC‐Davis, One Shields Ave, Davis, CA 95616, USA.
REFERENCES
- Allen RE, Rankin LL, Greene EA, Boxhorn LK, Johnson SE, Taylor RG, Pierce PR (1991) Desmin is present in proliferating rat muscle satellite cells but not in bovine muscle satellite cells. J. Cell. Physiol. 149, 525. [Abstract] [Google Scholar]
- Allen RE, Temm‐Grove CJ, Sheehan SM, Rice G (1997) Skeletal muscle satellite cell cultures. Meth. Cell Biol. 52, 155. [Abstract] [Google Scholar]
- Asakura A, Seale P, Girgis‐Gabardo A, Rudnicki MA (2002) Myogenic specification of side population cells in skeletal muscle. J. Cell Biol. 159, 123. [Europe PMC free article] [Abstract] [Google Scholar]
- Bockhold KJ, Rosenblatt JD, Partridge TA (1998) Aging normal and dystrophic mouse muscle: analysis of myogenicity in cultures of living single fibres. Muscle Nerve 21, 173. [Abstract] [Google Scholar]
- Chakravarthy MV, Davis BS, Booth FW (2000) IGF‐I restores satellite cell proliferative potential in immobilized old skeletal muscle. J. Appl. Physiol. 89, 1365. [Abstract] [Google Scholar]
- Cornelison DD, Wold BJ (1997) Single‐cell analysis of regulatory gene expression in quiescent and activated mouse skeletal muscle satellite cells. Dev. Biol. 191, 270. [Abstract] [Google Scholar]
- Deasy BM, Huard J (2002) Gene therapy and tissue engineering based on muscle‐derived stem cells. Curr. Opin. Mol. Ther. 4, 382. [Abstract] [Google Scholar]
- Deasy BM, Jankowski RJ, Huard J (2001) Muscle‐derived stem cells: characterization and potential for cell‐mediated therapy. Blood Cell Mol. Dis. 27, 924. [Abstract] [Google Scholar]
- Edom‐Vovard F, Mouly V, Barbet JP, Butler‐Browne GS (1999) The four populations of myoblasts involved in human limb muscle formation are present from the onset of primary myotube formation. J. Cell Sci. 112, 191. [Abstract] [Google Scholar]
- Fligger JM, Malven PV, Doumit ME, Merkel RA, Grant AL (1998) Increases in insulin‐like growth factor binding protein‐2 accompany decreases in proliferation and differentiation when porcine muscle satellite cells undergo multiple passages. J. Anim. Sci. 76, 2086. [Abstract] [Google Scholar]
- Gaster M, Beck‐Nielsen H, Schroder HD (2001) Proliferation conditions for human satellite cells. The fractional content of satellite cells. APMIS 109, 726. [Abstract] [Google Scholar]
- Gratama JW, Orfao A, Barnett D, Brando B, Huber A, Janossy G, Johnsen HE, Keeney M, Marti GE, Preijers F, Rothe G, Serke S, Sutherland DR, Van Der Schoot CE, Schmitz G, Papa S (1998) Flow cytometric enumeration of CD34+ haematopoietic stem and progenitor cells. European Working Group on Clinical Cell Analysis. Cytometry 34, 128. [Abstract] [Google Scholar]
- Hawke TJ, Garry DJ (2001) Myogenic satellite cells: physiology to molecular biology. J. Appl. Physiol. 91, 534. [Abstract] [Google Scholar]
- Jankowski RJ, Deasy BM, Cao B, Gates C, Huard J (2002) The role of CD34 expression and cellular fusion in the regeneration capacity of myogenic progenitor cells. J. Cell Sci. 115, 4361. [Abstract] [Google Scholar]
- Kaufman SJ, Foster RF (1988) Replicating myoblasts express a muscle‐specific phenotype. Proc. Natl. Acad. Sci. USA 85, 9606. [Europe PMC free article] [Abstract] [Google Scholar]
- Krause DS, Ito T, Fackler MJ, Smith OM, Collector MI, Sharkis SJ, May WS (1994) Characterization of murine CD34, a marker for hematopoietic progenitor and stem cells. Blood 84, 691. [Abstract] [Google Scholar]
- Kubin T, Vogel S, Wetzel J, Hein S, Pipp F, Herold J, Heil M, Kampmann A, Hehlgans S, Von Der Ahe D, Schaper W, Zimmermann R (2003) Porcine aortic endothelial cells show little effects on smooth muscle cells but are potent stimulators of cardiomyocyte growth. Mol. Cell Biochem. 242, 39. [Abstract] [Google Scholar]
- Lawson‐Smith MJ, McGeachie JK (1998) The identification of myogenic cells in skeletal muscle, with emphasis on the use of tritiated thymidine autoradiography and desmin antibodies. J. Anat. 192, 161. [Abstract] [Google Scholar]
- Machida S, Spangenburg EE, Booth FW (2003) Forkhead transcription factor FoxO1 transduces insulin‐like growth factor's signal to p27Kip1 in primary skeletal muscle satellite cells. J. Cell Physiol. 196, 523. [Abstract] [Google Scholar]
- Mauro A (1961) Satellite cell of skeletal muscle fibres. J. Biophys. Biochem. Cytol. 9, 493. [Europe PMC free article] [Abstract] [Google Scholar]
- Michal J, Xiang Z, Davenport G, Hayek M, Dodson MV, Byrne KM (2002) Isolation and characterization of canine satellite cells. In Vitro Cell Dev. Biol. Anim. 38, 467. [Abstract] [Google Scholar]
- Molkentin JD, Olson EN (1996) Defining the regulatory networks for muscle development. Curr. Opin. Genet. Dev. 6, 445. [Abstract] [Google Scholar]
- Qu Z, Balkir L, Van Deutekom JC, Robbins PD, Pruchnic R, Huard J (1998) Development of approaches to improve cell survival in myoblast transfer therapy. J. Cell Biol. 142, 1257. [Europe PMC free article] [Abstract] [Google Scholar]
- Qu‐Petersen Z, Deasy B, Jankowski R, Ikezawa M, Cummins J, Pruchnic R, Mytinger J, Cao B, Gates C, Wernig A, Huard J (2002) Identification of a novel population of muscle stem cells in mice: potential for muscle regeneration. J. Cell Biol. 157, 851. [Europe PMC free article] [Abstract] [Google Scholar]
- Rando TA, Blau HM (1994) Primary mouse myoblast purification, characterization, and transplantation for cell‐mediated gene therapy. J. Cell Biol. 125, 1275. [Europe PMC free article] [Abstract] [Google Scholar]
- Richler C, Yaffe D (1970) The in vitro cultivation and differentiation capacities of myogenic cell lines. Dev. Biol. 23, 1. [Abstract] [Google Scholar]
- Sabourin LA, Girgis‐Gabardo A, Seale P, Asakura A, Rudnicki MA (1999) Reduced differentiation potential of primary MyoD–/– myogenic cells derived from adult skeletal muscle. J. Cell Biol. 144, 631. [Europe PMC free article] [Abstract] [Google Scholar]
- Schultz E, Mccormick KM (1994) Skeletal muscle satellite cells. Rev. Physiol. Biochem. Pharmacol. 123, 213. [Abstract] [Google Scholar]
- Seale P, Rudnicki MA (2000) A new look at the origin, function, and ‘stem‐cell’ status of muscle satellite cells. Dev. Biol. 218, 115. [Abstract] [Google Scholar]
- Seale P, Sabourin LA, Girgis‐Gabardo A, Mansouri A, Gruss P, Rudnicki MA (2000) Pax7 is required for the specification of myogenic satellite cells. Cell 102, 777. [Abstract] [Google Scholar]
- Smith CK, Janney MJ, Allen RE (1994) Temporal expression of myogenic regulatory genes during activation, proliferation, and differentiation of rat skeletal muscle satellite cells. J. Cell Physiol. 159, 379. [Abstract] [Google Scholar]
- Spangenburg EE, Booth FW (2002) Multiple signaling pathways mediate LIF‐induced skeletal muscle satellite cell proliferation. Am. J. Physiol. Cell Physiol. 283, C204. [Abstract] [Google Scholar]
- Stiebellehner L, Frid MG, Reeves JT, Low RB, Gnanasekharan M, Stenmark KR (2003) Bovine distal pulmonary arterial media is composed of a uniform population of well‐differentiated smooth muscle cells with low proliferative capabilities. Am. J. Physiol. Lung Cell Mol. Physiol. 285, L819. [Abstract] [Google Scholar]
- Tamaki T, Akatsuka A, Ando K, Nakamura Y, Matsuzawa H, Hotta T, Roy RR, Edgerton VR (2002) Identification of myogenic‐endothelial progenitor cells in the interstitial spaces of skeletal muscle. J. Cell Biol. 157, 571. [Europe PMC free article] [Abstract] [Google Scholar]
- Torrente Y, Tremblay JP, Pisati F, Belicchi M, Rossi B, Sironi M, Fortunato F, El Fahime M, D’Angelo MG, Caron NJ, Constantin G, Paulin D, Scarlato G, Bresolin N (2001) Intraarterial injection of muscle‐derived CD34(+) Sca‐1(+) stem cells restores dystrophin in mdx mice. J. Cell Biol. 152, 335. [Europe PMC free article] [Abstract] [Google Scholar]
- Woollett GR, Williams AF, Shotton DM (1985) Visualisation by low‐angle shadowing of the leucocyte‐common antigen. A major cell surface glycoprotein of lymphocytes. EMBO J. 4, 2827. [Europe PMC free article] [Abstract] [Google Scholar]
- Yablonka‐Reuveni Z, Rivera AJ (1994) Temporal expression of regulatory and structural muscle proteins during myogenesis of satellite cells on isolated adult rat fibers. Dev. Biol. 164, 588. [Europe PMC free article] [Abstract] [Google Scholar]
- Yaffe D, Saxel O (1977) Serial passaging and differentiation of myogenic cells isolated from dystrophic mouse muscle. Nature 270, 725. [Abstract] [Google Scholar]
Articles from Cell Proliferation are provided here courtesy of Wiley
Full text links
Read article at publisher's site: https://doi.org/10.1111/j.1365-2184.2004.00311.x
Read article for free, from open access legal sources, via Unpaywall:
https://europepmc.org/articles/pmc6495777?pdf=render
Citations & impact
Impact metrics
Citations of article over time
Alternative metrics
Smart citations by scite.ai
Explore citation contexts and check if this article has been
supported or disputed.
https://scite.ai/reports/10.1111/j.1365-2184.2004.00311.x
Article citations
Bioengineered Model of Human LGMD2B Skeletal Muscle Reveals Roles of Intracellular Calcium Overload in Contractile and Metabolic Dysfunction in Dysferlinopathy.
Adv Sci (Weinh), 11(31):e2400188, 17 Jun 2024
Cited by: 1 article | PMID: 38887849 | PMCID: PMC11336985
Methods to Isolate Muscle Stem Cells for Cell-Based Cultured Meat Production: A Review.
Animals (Basel), 14(5):819, 06 Mar 2024
Cited by: 0 articles | PMID: 38473203 | PMCID: PMC10931118
Review Free full text in Europe PMC
Investigating proliferation and differentiation capacities of Hanwoo steer myosatellite cells at different passages for developing cell-cultured meat.
Sci Rep, 13(1):15614, 20 Sep 2023
Cited by: 1 article | PMID: 37730695 | PMCID: PMC10511522
Stem cells and extracellular vesicles to improve preclinical orofacial soft tissue healing.
Stem Cell Res Ther, 14(1):203, 15 Aug 2023
Cited by: 2 articles | PMID: 37580820 | PMCID: PMC10426149
Review Free full text in Europe PMC
The roles of growth factors and hormones in the regulation of muscle satellite cells for cultured meat production.
J Anim Sci Technol, 65(1):16-31, 31 Jan 2023
Cited by: 8 articles | PMID: 37093925 | PMCID: PMC10119461
Review Free full text in Europe PMC
Go to all (72) article citations
Similar Articles
To arrive at the top five similar articles we use a word-weighted algorithm to compare words from the Title and Abstract of each citation.
Myogenic specification of side population cells in skeletal muscle.
J Cell Biol, 159(1):123-134, 14 Oct 2002
Cited by: 407 articles | PMID: 12379804 | PMCID: PMC2173497
Origin and hierarchy of basal lamina-forming and -non-forming myogenic cells in mouse skeletal muscle in relation to adhesive capacity and Pax7 expression in vitro.
Cell Tissue Res, 344(1):147-168, 29 Jan 2011
Cited by: 4 articles | PMID: 21274567
Functional analysis of homeodomain-containing transcription factor Lbx1 in satellite cells of mouse skeletal muscle.
J Cell Sci, 120(pt 23):4178-4187, 14 Nov 2007
Cited by: 24 articles | PMID: 18003701
The molecular regulation of muscle stem cell function.
Cold Spring Harb Symp Quant Biol, 73:323-331, 01 Jan 2008
Cited by: 154 articles | PMID: 19329572
Review
Funding
Funders who supported this work.
NIA NIH HHS (1)
Grant ID: AG-18780
NIAMS NIH HHS (1)
Grant ID: AR-48514