Abstract
Free full text

N-methylpurine DNA glycosylase overexpression increases alkylation sensitivity by rapidly removing non-toxic 7-methylguanine adducts
Abstract
Previous studies indicate that overexpression of N-methylpurine DNA glycosylase (MPG) dramatically sensitizes cells to alkylating agent-induced cytotoxicity. We recently demonstrated that this sensitivity is preceded by an increased production of AP sites and strand breaks, confirming that overexpression of MPG disrupts normal base excision repair and causes cell death through overproduction of toxic repair intermediates. Here we establish through site-directed mutagenesis that MPG-induced sensitivity to alkylation is dependent on enzyme glycosylase activity. However, in contrast to the sensitivity seen to heterogeneous alkylating agents, MPG overexpression generates no cellular sensitivity to MeOSO2(CH2)2-lexitropsin, an alkylator which exclusively induces 3-meA lesions. Indeed, MPG overexpression has been shown to increase the toxicity of alkylating agents that produce 7-meG adducts, and here we demonstrate that MPG-overexpressing cells have dramatically increased removal of 7-meG from their DNA. These data suggest that the mechanism of MPG-induced cytotoxicity involves the conversion of non-toxic 7-meG lesions into highly toxic repair intermediates. This study establishes a mechanism by which a benign DNA modification can be made toxic through the overexpression of an otherwise well-tolerated gene product, and the application of this principle could lead to improved chemotherapeutic strategies that reduce the peripheral toxicity of alkylating agents.
INTRODUCTION
Many cancer chemotherapeutics achieve their medicinal effects by producing DNA lesions that trigger the interruption of cell division and ultimately lead to cell death. However, because chemotherapy does not exclusively target tumor cells, the adverse effects on normal tissues limit the doses that can be administered to patients (1). Because peripheral side effects limit treatment, tumor cells are often inadequately eliminated and the outgrowth of drug resistant clones is allowed. In order to advance cancer treatment toward a cure, chemotherapy needs to be made both more specific and more effective.
Chemotherapeutic drugs frequently modify DNA through oxidation and alkylation reactions (2), producing DNA adducts that are identified and replaced by the base excision repair (BER) pathway (3). BER involves the recognition and removal of damaged bases by a DNA glycosylase, followed by incision of the resulting abasic (AP) site by AP endonuclease (Ape 1), DNA synthesis by polymerase and strand ligation by DNA ligase (4). This pathway, therefore, involves the processing of modest structural DNA damage through toxic baseless and strand-interrupted species (5–7), which may transiently replace less toxic or non-toxic DNA lesions in the course of restoring the DNA sequence.
We have been interested in intervening into the BER pathway to improve the efficacy of cancer chemotherapy. Recent studies show that transient overexpression of N-methylpurine DNA glycosylase (MPG) increases the cytotoxicity of alkylating agents such as temozolomide in breast cancer cells (8). Cells that overexpress MPG also have higher numbers of alkylating agent-induced AP sites and/or single-stranded DNA breaks as well as double-stranded DNA breaks, which are the likely cause of this increased toxicity. These data suggest that overexpression of MPG creates an ‘imbalance’ in the BER pathway which leads to saturation of downstream repair enzymes and accumulation of toxic intermediates (8,9). Implicit is the idea that the intermediates produced are more detrimental to the cell that the alkylated base lesions from which they originate. Having established the accumulation of toxic BER intermediates, we turned our attention toward identifying the products of alkylation that lead to MPG overexpression-induced sensitivity.
The major site of alkylation for all methylating agents is the highly nucleophilic N7 nitrogen of guanine. N7-methylguanine (7-meG) makes up 70–90% of the adducts produced by methylation, with far fewer modifications occurring at the N3 position of adenine (3-meA) and fewest at the O6 position of guanine (O6-meG). Though quantitatively the most significant of these principal lesions, 7-meG has little, if any, effect on the cell. DNA duplex structure is unaltered by the addition of this methyl group, and 7-meG has the same coding properties as unmodified guanine. In fact, 7-meG is neither mutagenic nor toxic and appears to be in all ways harmless, as it is tolerated by the cell for generations. In contrast, the minor lesions 3-meA and O6-meG are primarily responsible for the cellular effects of methylation: 3-meA inhibits DNA polymerase and arrests DNA synthesis, leading to sister chromatid exchange, chromosome breaks and cell death; O6-meG is highly mutagenic and may, under certain circumstances, block DNA replication.
MPG recognizes and removes 3-meA and 7-meG (10), the most common methylated DNA lesions (11). Increased alkylation-induced cytotoxicity in MPG-overexpressing cells probably reflects an increased load of intermediates produced by incomplete repair of 3-meA adducts, 7-meG adducts or both. We decided to investigate the mechanism of MPG-induced alkylation sensitivity, with particular attention on the inciting lesions.
Here we demonstrate, through the use of inactive site-directed mutants, that MPG-driven alkylation sensitivity is dependent on the enzyme's glycosylase activity. We additionally found that, although MPG overexpression causes sensitivity to classic monofunctional alkylating agents, it imparts no sensitivity to the 3-meA specific agent MeOSO2(CH2)2-lexitropsin (Me-lex), arguing against a role for 3-meA removal in generating alkylating agent sensitivity. Specific adduct analysis revealed that MPG overexpression causes rapid, near-total removal of 7-meG from the DNA. This strongly implicates the conversion of non-toxic 7-meG adducts to toxic repair intermediates in explaining MPG-induced alkylation sensitivity. The exchange of harmless base changes for toxic genetic interruptions represents a novel approach to cancer treatment that could decrease side effects and increase the likelihood of a cure.
MATERIALS AND METHODS
Cell lines and culture conditions
The human mammary gland adenocarcinoma cell line MDA-MB231 was purchased at passage number 29 from the American Type Culture Collection (ATCC, Manassas, VA, USA). Cells were grown at 37°C in a 5% CO2 water-humidified chamber (Forma Scientific, Marietta, OH, USA), and cultured in RPMI 1640 media (Invitrogen Corporation, Carlsbad, CA, USA) supplemented with 10% fetal bovine serum (Hyclone Laboratories, Logan, UT, USA). A 1% penicillin/streptomycin antimicrobial solution (Invitrogen) was included in stable stock culture but not in experimental cultures, and cells were replaced by fresh frozen stocks before 25 passages in our lab.
Site-directed mutagenesis
Two inactive site-directed mutants of HA-MPG were constructed using a QuikChange Site-Directed Mutagenesis kit (Stratagene, La Jolla, CA, USA), according to the manufacturer's protocol. An alanine was substituted for glutamic acid residue 125 (E125A), using a mutagenic primer (5′-CGCATCGTGGAGACCGCGGCATACCTGGGGCC-3′) and its complement, both containing a point mutation (underlined) that would change the glutamic acid codon to an alanine (bold). An additional MPG mutant was generated by substituting tyrosine 162 with alanine, using the following primer and its complement: 5′-CCTGTACGTGTACATCATTGCCGGCATGTACTTCTGC-3′ (mutation underlined; codon change in bold). These two mutants have minimal glycosylase activity (12). Glu-125 deprotonates a bound water molecule that is required for glycosylic bond cleavage. However, the E125A mutation eliminates glysosylase activity. Tyr-162 intercalates into the minor groove, flipping a damaged nucleotide into the enzyme's active site, where cleavage can occur. Y162A mutants have minimal glycosylase activity. Site-directed mutagenesis with these mutagenic primers was performed on HA-MPG in the pCR2.1 vector, and the resulting constructs were sequenced to verify that the desired mutations had been introduced into the HA-MPG sequence. Both mutants were then moved into the modified adenoviral shuttle vector pacAd5 IRES EGFP, using BamHI and XhoI restriction sites.
Adenoviral infection
The HA-MPG-, HA-E125A- and HA-Y162A-containing adenoviral shuttle vectors and empty vector control (pacAd5 IRES2EGFP) were sent to the University of Iowa Gene Transfer Vector Core Laboratory for production and titering of replication-defective recombinant adenovirus (13). Adenovirus stocks (Ad5 IRES2EGFP, Ad5 E125A, Ad5 Y162A and Ad5 HA-MPG) were aliquoted and stored at −80°C until the time of infection. Adenoviral infection was performed as previously reported (8), using MDA-MB231 breast cancer cells at 60–90% confluence that were washed with phosphate-buffered saline (PBS), trypsinized for 10–15 min and resuspended in a known volume of medium. Identical cell numbers ranging from 5 × 106 to 6 × 106 cells were pelleted and resuspended in 50 μl of PBS and purified adenovirus at a multiplicity of infection of 15 IU/cell. Infections were carried out for 3 h at 37°C in a 5% CO2 water-humidified chamber with gentle agitation every 20–30 min. Infected cells were resuspended in 10 ml 10% RPMI, mixed thoroughly and plated for cell survival assays. Infection efficiencies of transduced cells were determined 24 h after infection by measuring EGFP fluorescence intensities of 104 cells using a FACSCalibur fluorescence activated cell sorter (Becton-Dickinson, San Jose, CA, USA). Results were analyzed with Cell-Quest 3.3 software (Becton-Dickinson). EGFP expression levels were also visualized by fluorescence microscopy using a Nikon TS-100F microscope fitted with a Nikon fluorescence system (Nikon, Tokyo, Japan).
Western blot analysis
Western blots were performed according to the Invitrogen NuPage protocol (Invitrogen) as outlined previously (8). Briefly, 20 μg protein was analyzed on a 10% Bis-Tris NuPAGE gel and transferred to a nitrocellulose (NC) membrane. Membranes were probed using monoclonal MPG antibody (kindly provided by Dr Rabindra Roy, University of Texas Medical Branch, Galveston, TX, USA), followed by horseradish peroxidase-conjugated rabbit anti-mouse IgG (Chemicon International, Temecula, CA, USA). Antibody binding was detected using chemiluminescence (Roche Diagnostics Corp., Indianapolis, IN, USA), and equal loading was confirmed by probing with pan Actin monoclonal Ab (NeoMarkers Inc., Fremont, CA, USA).
DNA isolation
DNA was isolated from cultured cells using the PureGene DNA extraction kit (Gentra Systems Inc., Minneapolis, MN, USA). Briefly, cell pellets were thawed and lysed in lysis buffer supplemented with 20 mM 2,2,6,6-tetramethylpiperidinoxyl (TEMPO; Aldrich). Protein was precipitated and removed by centrifugation, and the DNA/RNA in the supernatant was precipitated with isopropyl alcohol. The DNA/RNA pellet was resuspended in lysis buffer with 20 mM TEMPO and incubated with RNase A (100 mg/ml) at 37°C for 30 min, followed by protein and DNA precipitation. The DNA pellet was resuspended in sterilized distilled water with 2 mM TEMPO, and DNA samples were stored at −80°C until analysis.
Drug treatment
Infected cells were treated with DNA-damaging agents 24 h after adenoviral exposure (8). Infected cells were treated with Me-lex (a kind gift from Dr Barry Gold, University of Nebraska, Omaha, NE, USA), temozolomide (a kind gift from Dr Robert Bishop, Schering Corp., Kenilworth, NJ, USA), methylmethane sulfonate (MMS), ethyl nitrosourea (ENU), 1,3-bis(2-chloroethyl)-1-nitrosourea (BCNU), mitomycin C (MMC), Etoposide (all from Sigma Chemical Co., St Louis, MO, USA), thiotepa (Immunex Corp., Seattle, WA, USA) or cisplatin (Bristol Myers Squibb Co., Princeton, NJ, USA) for 1 h at 37°C in 5% CO2. Drug-containing media was removed and replaced with fresh growth medium, and cells were incubated for 24–48 h.
Cell survival assay
Survival of HA-MPG-overexpressing cells was measured 24 and 48 h after drug treatment using the tetrazolium-based MTS [3-(4,5-dimethylthiazol-2-yl)-5-(3-carboxymeyhoxyphenyl)-2-(4-sulfophenyl)-2H-tetrazolium] dye reduction assay (8,14,15) (Promega, Madison, WI, USA). Infected cells were incubated with MTS/PMS solution for 1–4 h at 37°C in a 5% CO2 humidified incubator, and absorbance at 490 nm was measured in a tunable microplate reader (Molecular Devices, Sunnyvale, CA, USA).
Measurement of N7-methylguanine adducts in cells treated with MMS
Two independent techniques were used to measure 7-meG adducts Infected cells expressing IRES EGFP vector control or HA-MPG were treated with MMS for 1 h starting 24 h after adenoviral infection. MMS-containing medium was removed and replaced with fresh growth medium. Cells were harvested 0, 2, 5 and 25 h after drug treatment, washed three times with PBS and collected by scraping. Cell pellets were frozen at −80°C until DNA isolation and adduct analysis.
N7-meG HPLC-ECD
Quantification of 7-meG in extracted cellular DNA was performed by high-performance liquid chromatography (HPLC) with electrochemical detection (ECD) using a modification of the method published by Park and Ames (16). Between 12 and 55 μg of extracted DNA was adjusted to a final volume of 100 μl with ddH2O. The DNA samples were subjected to neutral thermal hydrolysis at 100°C for 30 min to quantitatively release 7-meG. Depurinated DNA was precipitated by the addition of 10 μl of cold 1 M HCl and removed by centrifugation. To the supernatant, 100 μl of HPLC mobile phase buffer and 9 μl of 1 M NaOH were added. Bases released into the supernatant were separated by reversed phase chromatography (Beckman Ultrasphere C18 column, 25 cm × 4.6 mm, 5 μm particle size) using 50 mM potassium phosphate buffer, pH 5.5/methanol [98:2 (v/v)] as mobile phase at a flow rate of 1 ml/min. The 7-meG adduct was detected by a CoulArray electrochemical detector (ESA, Chelmsford, MA, USA) monitoring oxidation at 500, 600, 700 and 800 mV. The concentration of 7-meG was determined by comparing the peak areas resulting from the ECD of cell samples with those of a series of standards.
Immuno-slot-blot for N7-meG
Experimental samples (1 μg) or a reference standard containing a known amount of N7-meG were denatured in 200 μl TE buffer plus 20 μl 2N NaOH at 37°C for 15 min. This treatment generates 2,6-diamino-4-hydroxy-5-N-methylformamidopyrimidine (imidazole ring-opened 7-meG) from 7-meG. After addition of an equal volume of 2 M ammonium acetate, samples were applied to NC filters using a Minifold II (Schleicher & Schuell). The filters were soaked in 5× SSC (0.75 M NaCl, 75 mM sodium citrate, pH 7.0) for 15 min, dried and baked in a vacuum oven for 2 h at 80°C. Filters were incubated for 2 h at 37°C with blocking buffer [20 mM Tris–HCl (pH 7.5), 0.1 M NaCl, 1 mM EDTA, 0.5% casein and 0.1% deoxycholic acid], followed by a 2 h incubation at 37°C in blocking buffer containing antibody (1:10 000) to imidazole ring-opened 7-methylguanosine (17). The filters were washed extensively in washing buffer [20 mM Tris–HCl (pH 7.5), 0.26 M NaCl, 1 mM EDTA, 0.1% Tween-20] and treated with a labeled polymer (peroxidase-labeled polymer conjugated to goat anti-rabbit and goat anti-mouse immunoglobulins) (Daco) diluted 1:1000 in hybrid buffer. After rinsing the NC membrane, the enzymatic activity on the membrane was visualized by enhanced chemiluminescence reagents (Amersham). The NC filter was exposed to X-ray film, and the developed film was analyzed using a Kodak image analysis system.
RESULTS
The role of MPG glycosylase activity in sensitivity to DNA alkylation damage
In order to determine whether MPG-driven alkylator sensitivity depends on the enzyme's glycosylase activity, two MPG mutants, Y162A and E125A, were constructed to be selectively inactive in their ability to bind substrate bases or excise them, respectively. Tyrosine 162 plays a role in flipping nucleotides into the enzyme active site, which is required for lesion recognition and DNA binding; glutamic acid 125 then activates a water molecule that is required for hydrolysis of the glycosyl bond (12).
MDA-MB231 breast cancer cells were infected with recombinant adenovirus carrying HA-tagged wild-type, Y162A or E125A MPG or vector control (Figure 1). Western blot analysis at 24 h post-infection showed that these three MPG variants were equivalently overexpressed (data not shown). Cells were treated with the monofunctional alkylating agent MMS or the clinical chemotherapeutic temozolomide 24 h post-infection and incubation was continued for 24 h. Cell survival was measured using the MTS assay. The results showed that overexpression of wild-type but neither mutant MPG increased the toxicity of MMS and temozolomide relative to the vector control (Figure 1). This proves that the sensitizing effect of MPG overexpression is a result of the enzyme's glycosylase activity, and further indicates that both binding and excisional components of MPG glycosylase activity are required for the increased alkylation toxicity created by high levels of wild-type MPG.
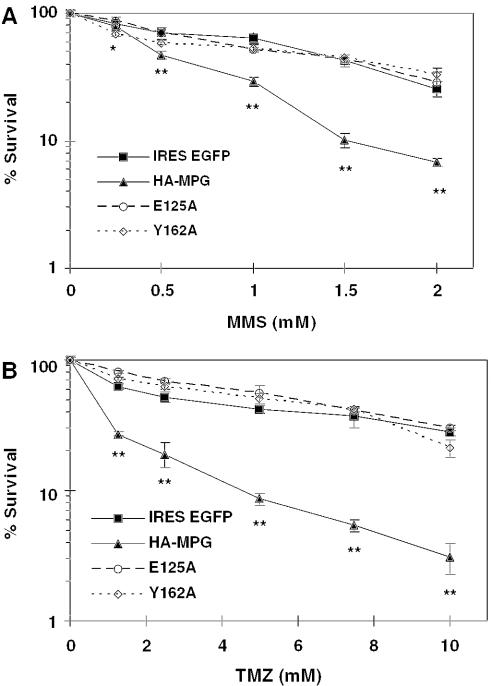
Survival of drug-treated MDA-MB231 cells overexpressing wild-type or mutant MPG. Vector control (IRES EGFP), wild-type MPG (HA-MPG), E125A mutant MPG (E125A) or Y162A mutant MPG (Y162A) overexpressing cells were treated with either MMS (A) or temozolomide (TMZ) (B) at the indicated concentrations and survival was determined as described in Materials and Methods. Data points are the average of >3 independent experiments. Two-way ANOVA analysis was used to evaluate statistical significance. The significance is indicated as *P < 0.05 or **P < 0.01.
Lesion specificity of MPG-dependent sensitivity to DNA alkylation damage
Alkylating agents react with nucleophilic centers to form several distinct covalent adducts with DNA bases. The most common sites of alkylation include (in order of prevalence) N7-guanine, N3-adenine and O6-guanine (11). Most simple methylating agents generate predominantly 7-meG adducts and a smaller number of 3-meA and O6-meG adducts.
MPG has a relatively broad substrate specificity and it excises many DNA adducts including 7-meG (11), 3-meA (10), 3-methylguanine (18,19), hypoxanthine (20), 1,N6-ethenoadenine (21), 3,N2-ethenoguanine and 8-oxoguanine (22). Because of their prevalence, 7-meG and 3-meA are principal alkylation substrates of MPG. However, the lesions responsible for increased alkylating agent-induced cytotoxicity in MPG-overexpressing cells remain unknown.
In contrast, the mixture of DNA lesions resulting from most alkylating agents, Me-lex, selectively generates 3-meA adducts and was used here to investigate their role in MPG-dependent cytotoxicity. Me-lex is a methyl sulfonate ester tethered to a neutral N-methylpyrrolecarboxamide-based dipeptide (lex), which confers sequence-selective minor groove DNA binding (23,24). Of the adducts formed by Me-lex, >99% are 3-meA, and a very small fraction are 7-meG (25) or O6-meG (26), making Me-lex extremely useful for determining the role of 3-meA lesions in MPG-dependent cytotoxicity. When wild-type cells or cells overexpressing MPG were treated with Me-lex, the results clearly showed that overexpression of MPG had no effect on cell survival (Figure 2), demonstrating that 3-meA is not the lesion involved in MPG-induced alkylator sensitivity.
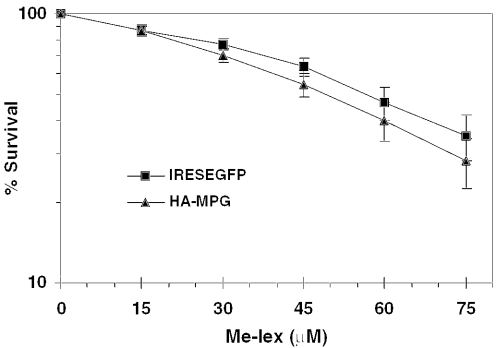
Survival of Me-lex-treated MDA-MB231 cells overexpressing MPG. MPG-overexpressing cells were treated with Me-lex at the indicated concentrations and survival was determined as described in Materials and Methods. Data points are the average of >10 independent experiments. Two-way ANOVA analysis was used to evaluate statistical significance. There were no statistically significant differences between the vector and MPG-expressing cells.
Quantitative analysis of 7-meG adducts in MMS-treated wild-type and MPG-overexpressing cells
DNA adducts at the highly nucleophilic N7 position of guanine account for 70–90% of the base modifications generated by monofunctional methylating agents such as MMS (11). Despite its abundance, 7-meG is a relatively innocuous DNA modification, because it does not alter DNA structure, is not mutagenic and does not lead to cell death (27–30). Still, its relative electron deficiency causes 7-meG to be recognized and excised by MPG, even though it presents no threat to the cell. No compounds are currently known to generate 7-meG exclusively in the DNA, but all commonly used methylating agents generate a preponderance of this adduct. A quantitative analytical approach was developed to establish the possible role of 7-meG adducts in MPG-dependent alkylation-induced cytotoxicity.
Wild-type and MPG-overexpressing cells were treated with sensitizing doses of MMS and cells were then harvested 0, 2, 5 and 25 h post-treatment. 7-meG adducts in the DNA were quantified by two independent methods: HPLC with ECD and immuno-slot-blotting. The results shown in Figure 3 indicate that a large number of 7-meG adducts accumulate in wild-type cells following MMS treatment. In contrast, very few 7-meG adducts are observed at any time point in cells overexpressing MPG. In fact, the level of 7-meG in alkylating agent-treated MPG-overexpressing cells was reduced to at or near baseline at every time point, and in the more quantitative HPLC assay, levels were below the limit of detection except immediately following the highest drug exposure. These data suggest that MPG rapidly and efficiently converts 7-meG adducts into the downstream repair intermediates previously demonstrated (8). This striking, near-total exchange of benign 7-meG bases for toxic baseless and strand-broken DNA establishes a role for 7-meG removal in the sensitizing effect of MPG overexpression.
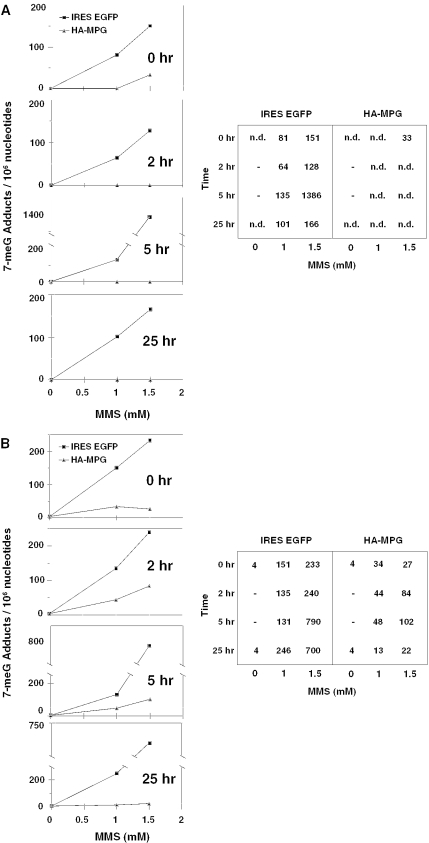
High-performance liquid chromatography (HPLC) analysis of 7-meG lesions per 106 nt in the DNA of adenovirally infected MDA-MB231 cells at various time points following treatment with MMS. (A) 7-meG DNA adducts were quantified by HPLC with electrochemical detection in MPG-overexpressing cells or in vector control cells exposed to MMS as indicated. n.d. = below detectable limit. (B) same as (A), except 7-meG was quantified by immuno-slot-blot analysis.
MPG-dependent cytotoxicity in the presence of N7-guanine reactive compounds
To determine whether methylation at the N7 position of guanine is specifically responsible for MPG-induced alkylation sensitivity, a number of drugs that append other forms of damage to guanine were analyzed for their impact on MPG-overexpressing cell survival. These compounds were ENU, BCNU, thiotepa (N,N′,N′,-triethylenethiophosphoramide), 4-hydroxycyclophosphamide (4-HC), MMC and cisplatin. ENU is a monofunctional alkylating agent that generates ethylation damage at the same N7G, O6G and N3A positions as its methylating counterpart, MNU, which was previously shown to sensitize MPG-overexpressing cells. BCNU is a monofunctional alkylating agent that has some selectivity for chloroethylating guanine at the O6 position (11,31). Thiotepa is a clinical bifunctional alkylating agent that generates N7-guanine- and N3-adenine-targeted aminoethyl adducts (32), which can undergo depurination or imidazole ring-opening to AP sites or 2,6-diamino-4-hydroxy-5-formamidopyrimidine (FaPyGua) and 4,6-diamino-5-formamidopyrimidine (FaPyAde) (33). 4-HC, the activated form of the clinical chemotherapeutic cyclophosphamide and its structural isomer ifosphamide, is a bifunctional alkylating agent with bischloroethyl side chains that generates N7-guanine-linked interstrand crosslinks in G–X–C-containing sequences (34). Similarly, MMC, an aziridin ring-containing DNA cross-linking agent (35), forms N7-guanine-linked interstrand crosslinks. Cisplatin is a square planar platinum-containing complex with two cis-oriented chloride leaving groups that preferentially generates intrastrand DNA crosslinks in dGpdG and dApdG motifs (34,36). MPG recognizes and binds to cisplatin-DNA adducts, but not does not excise them in vitro (37).
Wild-type and MPG-overexpressing cells were treated with the DNA-damaging agents discussed above and survival curves were determined 24 or 48 h post-treatment using the MTS survival assay (see legend to Figure 4 for details). The results are shown in Figures 4A–F. These data show that overexpression of MPG in MDA-MB231 cells does not alter the cytotoxicity of N7-ethyl-adducted lesions, N7-aminoethylguanine or N3-aminoethyladenine adducts, O6-chloroethylguanine adducts, N7-adducted nitrogen mustard monoadducts or crosslinks, extracyclic guanine monoadducts or crosslinks, or cisplatin crosslinks. MPG-dependent cytotoxicity in MDA-MB231 cells appears to be specific to 7-meG adducts generated by monofunctional methylating agents.
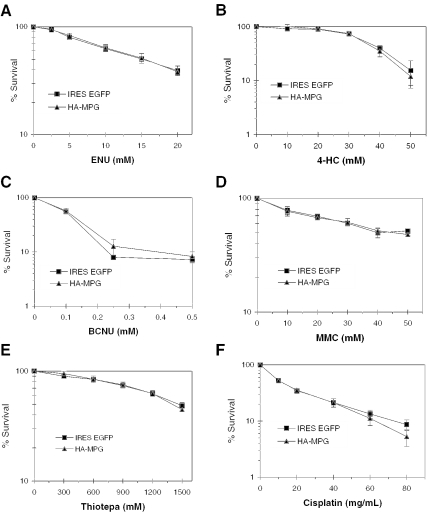
Survival of MPG-overexpressing MDA-MB231 cells exposed to diverse DNA-damaging agents. Wild-type and MPG-overexpressing MDA-MB231 cells were treated with (A) ENU, (B) BCNU, (C) Thiotepa, (D) 4-HC, (E) MMC or (F) Cisplatin. Survival was measured 24 (A and B) or 48 h (C–F) after drug treatment. Two-way ANOVA analysis was used to evaluate statistical significance. There were no statistically significant differences between the vector and MPG-expressing cells for any of the agents shown in this figure.
DISCUSSION
We have previously shown that MPG overexpression sensitizes breast cancer cells to the effects of DNA alkylation, manifested as high levels of baseless and strand-broken DNA that ultimately lead to considerable increases in cell death (8). The goal of this study was to understand the mechanism by which overexpression of MPG increases sensitivity to alkylating agents in MDA-MB231 cancer cells. The data presented here support the hypothesis that the observed cytotoxicity is caused by rapid conversion of 7-meG adducts into toxic repair intermediates sites by MPG. 7-meG is not a cytotoxic lesion and overexpression of MPG, in the absence of exposure to DNA alkylation damage, is also not cytotoxic; thus, this study reveals a novel synthetic cytotoxicity uniquely observed in MPG-overexpressing cells exposed to alkylation damage.
Two MPG mutants deficient in either substrate binding or excision were used in this study to examine the requirement for MPG glycosylase activity in generating alkylation sensitivity. Overexpression of these two inactive mutants did not in either case confer the sensitivity seen with wild-type MPG (Figure 1), demonstrating that both MPG binding and excision of substrate bases are necessary to confer the dramatic sensitivity to alkylation previously seen (8). MPG lesion binding alone is insufficient. The obvious implication is that the products of MPG excisional activity are directly responsible for increasing toxicity, providing further evidence that unrepaired AP sites and their downstream strand-broken counterparts lead to the increased toxicity observed in MPG-overexpressing cells.
Previous studies have established that the E125A and Y162A MPG mutants employed here assume a near-native 3D structure, despite their aberrant enzymatic function (12). It is therefore unlikely that MPG-induced cytotoxicity results from any other function of the enzyme, including its proposed interaction with the nucleotide excision repair protein hHR23 (38), a function which should be conserved in E125A and Y162A.
MPG has broad specificity for a number of damaged DNA bases. We therefore proceeded to investigate the role of specific alkylated bases in MPG overexpression-induced alkylation sensitivity. The majority of base damage induced by methylation is removed by MPG, including the principal adducts 3-meA and 7-meG (10). Treatment of MPG-overexpressing cells with the minor groove methylating agent Me-lex (23), which selectively introduces >99% 3-meA adducts, was performed in order to discover the contribution of 3-meA to MPG-induced sensitivity. Results showed that increased exogenous expression of MPG caused no change in the survival response to Me-lex. These experiments clearly demonstrate that 3-meA removal is not involved in producing sensitivity to alkylation, despite the fact that MPG is able to recognize and excise 3-meA lesions from DNA. One explanation for this result is that the cytotoxicity of 3-meA may be roughly equivalent to the cytotoxicity of downstream intermediates generated during BER of 3-meA. Such an exchange, therefore, even at an increased rate, would not be expected to significantly alter cell survival.
The hypothesis that imbalanced BER has cytotoxic consequences is based on the assumption that downstream repair intermediates can be more cytotoxic than the DNA lesions that trigger repair. Thus, it is relevant to note that 3-meA is intrinsically cytotoxic, because it inhibits DNA polymerases (39,40), but 7-meG, a more abundant DNA lesion, is neither cytotoxic nor mutagenic (29,30). In fact, 7-meG does not appear to have any impact on cell viability. Thus, 7-meG is a likely candidate for the lesion associated with MPG-dependent alkylating agent-induced cytotoxicity because incomplete repair of 7-meG, in contrast to 3-meA, could potentially be more deleterious to the cell than no repair at all.
It is also important to note that MPG removes 3-meA and 7-meG adducts with very different enzyme kinetics. When present at its average endogenous level, MPG excises 7-meG much more slowly than it excises 3-meA (t1/2 = 18 h versus 1 h, respectively). For 3-meA, the rate-limiting step occurs late in the BER pathway, whereas for 7-meG, the rate-limiting step is MPG excision. Furthermore, because 7-meG is abundant and its repair is slow, excision could readily become saturated in wild-type cells with relatively low endogenous MPG (41), and this would not be expected to occur for 3-meA. Increased levels of MPG may therefore selectively increase the excision of 7-meG because of differential enzyme kinetics, supporting the hypothesis that MPG overexpression rapidly converts 7-meG into toxic baseless and strand-broken intermediates and leads to increased cytotoxicity following alkylating agent exposure.
This hypothesis was tested by quantitative HPLC-ECD and immuno-slot-blot analyses of 7-meG adducts in MPG-overexpressing cells (Figure 3). The results show that the removal of 7-meG adducts is much faster and more extensive in MPG-overexpressing cells than in wild-type cells. This confirms that heightened MPG activity is able to increase the normally slow rate of 7-meG excision, shifting the rate-limiting step downstream and leading to the accumulation of toxic repair intermediates. These results strongly implicate 7-meG removal as the mechanism by which MPG overexpression causes sensitivity to alkylating agents.
As demonstrated in this and previous work (8), alkylating agents that generate 7-meG DNA adducts have increased cytotoxicity in cells with heightened levels of MPG. We explored the specificity of this effect using a number of agents that append other forms of damage to guanine. No sensitivity was seen to any of the agents tested, providing further evidence that MPG overexpression exclusively generates sensitivity to compounds that produce 7-meG in the DNA. This further strengthens the hypothesis that rapid conversion of 7-meG adducts into toxic abasic sites and strand breaks is responsible for the increased toxicity of alkylating agents in MPG-overexpressing cells.
CONCLUSION
The results presented here provide insight into BER and the consequences of alkylation damage, and have important implications for clinical chemotherapy.
The rapid removal of 7-meG lesions by increased MPG converts innocuous base changes into toxic repair intermediates that cannot be adequately handled by downstream BER enzymes. The result is a dramatic increase in cell death. This mechanism confirms that uncoordinated handling of even harmless DNA changes can be highly detrimental to the cell, and it underscores the importance of repair pathway management.
These experiments demonstrate that cells risk extreme toxicity to reverse changes to their genetic material and that intervening into these repair mechanisms can alter cell survival. We believe that the results of this study have novel implications for cancer chemotherapy. Because MPG overexpression, which in and of itself is non-toxic, can convert a benign DNA adduct into a highly toxic one by saturating BER, two non-toxic therapies can be used to create cytotoxicity. Of course, the sensitizing effects of MPG overexpression toward 7-meG could be expected to occur in normal as well as cancer cells. However, if independent tumor-targeting strategies, each with distinct tissues of non-specificity, were used for both MPG gene delivery and 7-meG drug delivery, even imprecise targeting could be overcome as the two therapies coincide to produce tumor-specific cell death. The result would be more effective and more specific chemotherapy, leading to decreased peripheral toxicity and improved cancer elimination.
Acknowledgments
This work was supported by grants from the National Institutes of Health CA106298, CA094025, ES05865, ES03456 and a P30 DK49218 grant to M.R.K. M.L.R was supported by Department of Defense/Congressionally Directed Mandated Research Program training grant BC011075. J.N. was supported by National Institutes of Health grant R42ES11746, and B.F.P. was supported by National Cancer Institute training grant T32-CA72319. The Riley Children's Foundation provided additional support. We would also like to heartily thank Dr Rabindra Roy, Georgetown University Medical School, Washington, DC, USA, for the MPG antibody, Dr Robert Bishop for the temozolomide and Dr Rhoderick Elder for the polyclonal antibody against ring-opened N7-meG. We would also like to thank Dr Barry Gold, Eppley Institute for Research in Cancer and Allied Disease and Department of Pharmaceutical Sciences, University of Nebraska Medical Center, Omaha, NE, USA for the Me-lex and for reviewing this manuscript. Funding to pay the Open Access publication charges for this article was provided by Riley Children's Foundation.
Conflict of interest statement. None declared.
REFERENCES
Articles from Nucleic Acids Research are provided here courtesy of Oxford University Press
Full text links
Read article at publisher's site: https://doi.org/10.1093/nar/gki601
Read article for free, from open access legal sources, via Unpaywall:
https://academic.oup.com/nar/article-pdf/33/9/2859/7127732/gki601.pdf
Citations & impact
Impact metrics
Citations of article over time
Smart citations by scite.ai
Explore citation contexts and check if this article has been
supported or disputed.
https://scite.ai/reports/10.1093/nar/gki601
Article citations
Anthracyclines React with Apurinic/Apyrimidinic Sites in DNA.
ACS Chem Biol, 18(6):1315-1323, 18 May 2023
Cited by: 2 articles | PMID: 37200590 | PMCID: PMC10391585
Effect of Polyphenols and Zinc Co-Supplementation on the Development of Neoplasms in Rats with Breast Cancer.
Foods, 12(2):356, 12 Jan 2023
Cited by: 0 articles | PMID: 36673448 | PMCID: PMC9857727
Exploratory Metabolomics Underscores the Folate Enzyme ALDH1L1 as a Regulator of Glycine and Methylation Reactions.
Molecules, 27(23):8394, 01 Dec 2022
Cited by: 2 articles | PMID: 36500483 | PMCID: PMC9740053
Effect of Genistein Supplementation on the Progression of Neoplasms and the Level of the Modified Nucleosides in Rats With Mammary Cancer.
In Vivo, 35(4):2059-2072, 01 Jul 2021
Cited by: 4 articles | PMID: 34182481 | PMCID: PMC8286469
Urinary biomarkers for secondhand smoke and heated tobacco products exposure.
J Clin Biochem Nutr, 69(1):37-43, 09 Mar 2021
Cited by: 5 articles | PMID: 34376912 | PMCID: PMC8325761
Go to all (53) article citations
Similar Articles
To arrive at the top five similar articles we use a word-weighted algorithm to compare words from the Title and Abstract of each citation.
Transient adenoviral N-methylpurine DNA glycosylase overexpression imparts chemotherapeutic sensitivity to human breast cancer cells.
Mol Cancer Ther, 3(8):955-967, 01 Aug 2004
Cited by: 57 articles | PMID: 15299078
Effects of substrate specificity on initiating the base excision repair of N-methylpurines by variant human 3-methyladenine DNA glycosylases.
Chem Res Toxicol, 18(1):87-94, 01 Jan 2005
Cited by: 13 articles | PMID: 15651853
N-methylpurine DNA glycosylase inhibits p53-mediated cell cycle arrest and coordinates with p53 to determine sensitivity to alkylating agents.
Cell Res, 22(8):1285-1303, 17 Jul 2012
Cited by: 13 articles | PMID: 22801474 | PMCID: PMC3411163
MGMT: key node in the battle against genotoxicity, carcinogenicity and apoptosis induced by alkylating agents.
DNA Repair (Amst), 6(8):1079-1099, 07 May 2007
Cited by: 369 articles | PMID: 17485253
Review
Funding
Funders who supported this work.
NCI NIH HHS (6)
Grant ID: T32 CA072319
Grant ID: CA094025
Grant ID: CA106298
Grant ID: R01 CA106298
Grant ID: R01 CA094025
Grant ID: T32-CA72319
NIDDK NIH HHS (2)
Grant ID: P30 DK049218
Grant ID: P30 DK49218
NIEHS NIH HHS (7)
Grant ID: R01 ES003456
Grant ID: ES05865
Grant ID: R42ES11746
Grant ID: ES03456
Grant ID: F32 ES005865
Grant ID: R01 ES005865
Grant ID: R42 ES011746