Abstract
Free full text

Organ-specific distribution of ACE2 mRNA and correlating peptidase activity in rodents
Abstract
Biochemical analysis revealed that angiotensin-converting enzyme related carboxy-peptidase (ACE2) cleaves angiotensin (Ang) II to Ang-(1–7), a heptapeptide identified as an endogenous ligand for the G protein-coupled receptor Mas. No data are currently available that systematically describe ACE2 distribution and activity in rodents. Therefore, we analyzed the ACE2 expression in different tissues of mice and rats on mRNA (RNase protection assay) and protein levels (immunohistochemistry, ACE2 activity, western blot). Although ACE2 mRNA in both investigated species showed the highest expression in the ileum, the mouse organ exceeded rat ACE2, as also demonstrated in the kidney and colon. Corresponding to mRNA, ACE2 activity was highest in the ileum and mouse kidney but weak in the rat kidney, which was also confirmed by immunohistochemistry. Contrary to mRNA, we found weak activity in the lung of both species. Our data demonstrate a tissue- and species-specific pattern for ACE2 under physiological conditions.
1. Introduction
In the regulation of heart function and blood pressure, different peptide systems are involved, e.g. the renin–angiotensin system (RAS), the kallikrein–kinin system, and the natriuretic peptide system. In these systems, proteases like angiotensin-converting enzyme (ACE) or neutral endopeptidase (NEP) have the distinction of generating or catabolizing biologically active peptides [10], [39], [42]. The newly discovered angiotensin-converting enzyme-related carboxypeptidase (ACE2) has considerable sequence homology to ACE (40% identity and 61% similarity), contains a HEXXH zinc-binding domain, and conserves other critical residues typical of the ACE family [12], [37]. The first step in generating angiotensin peptides is the cleavage of angiotensinogen to angiotensin (Ang) I by renin. Ang I is hydrolyzed by either ACE or chymase to Ang II, which mediates its biological actions via the AT1 and AT2 receptors [15], [21]. Ang I is also metabolized by NEP to Ang-(1–7) [15], which mediates distinct effects through its receptor Mas [35]. Importantly, Ang-(1–7) can also be directly metabolized from Ang II by ACE2, whereas aminopeptidase A converts Ang II to Ang III [18]. ACE2 also hydrolyzes Ang I to Ang-(1–9), although there is no hydrolysis of Ang-(1–9), Ang-(1–7), and Ang-(1–5). Moreover, ACE2 hydrolysis is also specific for des-Arg [9] bradykinin and its shorter fragments, although it cleaves neither bradykinin nor bradykinin-(1–7) [40]. ACE2 mRNA is expressed in many tissues but shows a less ubiquitous profile than ACE. First studies in mice detected the highest expression in the ileum by quantitative reverse transcriptase polymerase chain reaction (QRT-PCR) [23].
ACE2 is an important part of the RAS, which counteracts the function of ACE. It was also shown that ACE2 expression can be upregulated by blockade of AT1-receptors [27]. The importance of ACE2 in cardiovascular regulation was confirmed by targeted disruption of ACE2 in mice. The absence of ACE2 in mice leads to a severe cardiac contractility defect, increased Ang II levels, and upregulation of hypoxia-induced genes in the heart [11]. In addition to its peptidolytic function, recent investigations have discovered that ACE2 is a functional receptor for the coronavirus, which causes the severe acute respiratory syndrome (SARS) [30].
In this investigation, we (i) measured the mRNA distribution of ACE2 through different tissues in both species. Moreover, we (ii) quantified ACE2 protein by western blot using a commercial polyclonal antibody to ACE2. We (iii) measured ACE2 activity in different tissues of mice and rats. We (iv) established a monoclonal antibody against ACE2 to complete the investigation of tissue distribution by immunohistochemistry. Finally, we compared (v) the distribution of ACE2 in both species on the mRNA and protein level.
2. Materials and methods
2.1. Animals
All experiments were done according to the guidelines of the Federal Law on the Use of Experimental Animals in Germany and were approved by the local authorities. For this investigation we used C57Bl/6 mice and Sprague-Dawley (SD) rats in an age of 3–5 months. Animals were killed by cervical dislocation. For RNase protection assay (RPA), ACE2 activity assay and western blot, the tissues were snap frozen in liquid nitrogen. The samples were stored at −80 °C until further processing (all organs in total, heart divided into atria and ventricles). The tissues for immunohistochemistry were put in 4% formalin. After 24 h they were embedded and processed to paraffin sections.
2.2. RNase protection assay
2.2.1. Probe generation
The polymerase chain reaction (PCR) amplified a 358 bp fragment (probe: MMACE2) from mouse kidney cDNA using the 5′-primer CTC AGT GGA TGG GAT CTT GG (MMACE25) and the 3′-primer TGT AGC CAT CTG CTC CCT CT (MMACE23), respectively a 342 bp fragment (probe: RNACE2) from rat lung cDNA using the 5′-primer CGG GGA AAG ATG TCA AGC TCC TGC (RNACE25) and the 3′-primer CTT GTC TGG TGA CAG CGC (RNACE23), which were subcloned in a T-vector (Promega GmbH, Mannheim, Germany). A SP6 polymerase transcribed a radioactive probe complementary to MMACE2 (resp. RNACE2) mRNA, and a RNA complementary to 127 nucleotides of the rL32 mRNA was used as positive control [2]. ACE2-specific mRNA for mouse and rat were identified by RNase protection assay (RPA) using the Ambion RPA II kit (Ambion (Europe) Ltd., Huntingdon, UK).
2.2.2. RNase protection assay
Total RNA was isolated from tissues using the TRIzol reagent (Invitrogen GmbH, Karlsruhe, Germany) with subsequent chloroform–isopropanol extraction according to the manufacturer's instructions. A 15 μg total RNA fraction of each sample was hybridized with approximately 50 000 cpm for ACE2 and 50 000 cpm for rL32 of the radiolabeled antisense probes in the same assay. Equal loading has been insured by mRNA measurements and mRNA gel electrophoresis using 1 μg of each sample (not shown). The hybridized fragments protected from RNase A + T1 digestion were separated by electrophoresis on a denaturing gel (5%, w/v polyacrylamide, 8 M urea) and analyzed using a FUJIX BAS 2000 Phospho-Imager system (Raytest GmbH, Straubenhardt, Germany) to perform quantitative analysis by measuring the intensity of the ACE2 bands. The blots of each species were calculated to ACE2 mRNA expression in kidney, which was present on both blots of each species. The expression level in the lung was set to 100%.
2.3. ACE2 activity assay
ACE2 activity was measured similar to the method by Vickers et al. [40]. Tissue was homogenized in assay buffer (50 mM 2-morpholinoethanesulfonic acid, 300 mM NaCl, 10 μM ZnCl2, 0.01% Brij-35, pH 6.5). Protein concentration was determined using Roti-Quant (Carl Roth GmbH and Co. KG, Karlsruhe, Germany) by the manufacturers instruction. We used Mca-APK(Dnp) (Biosynthan GmbH, Berlin, Germany) dissolved in DMSO (50 μM, final concentration) as the ACE2 substrate. The assay was performed in assay buffer and was started by adding 10 μl of tissue homogenate. After 2 h incubation at ambient temperature (24 °C), the reaction was suppressed by adding 100 μM o-phenanthrolin (final concentration). Parallel control tests were performed in the presence of 1 μM DX 600 (data not shown) [25]. After centrifugation (10 min, 10 000 × g) the fluorescence was measured at 320 nm (excitation) and 405 nm (emission) with the Perkin-Elmer fluorescence reader Lambda 5 (Perkin-Elmer LAS GmbH, Rodgau, Germany). The molecular standardization was performed with Mca-AP (Biosynthan GmbH, Berlin, Germany) and calculated per mg protein. The functionality of the assay was proven by a standardized solution with defined, recombinant ACE2 activity (R&D Systems GmbH, Wiesbaden, Germany).
2.4. Western blot
Tissue was homogenized in phosphate-buffered solution (PBS) containing protease inhibitor mixture (Complete, Roche Diagnostics GmbH, Mannheim, Germany). Protein concentration was determined with BCA Protein Assay Kit (Perbio Science GmbH, Bonn, Germany). Sample proteins (10 μg/lane) and a prestained protein-weight marker (Amersham Biosciences GmbH, Freiburg, Germany) were size fractionated by SDS-polyacrylamide gels (10%) and transferred to PVDF membranes with a Pegasus semi-dry-blotter (Phase GmbH, Lübeck, Germany). Equal loading has been insured by staining control gels with SimplyBlue Safe Stain (Invitrogen GmbH, Karlsruhe, Germany) using 10 μg of each sample (not shown). The membranes were blocked at room temperature in 5% dry milk powder (blotting grade, non-fat dry milk, Bio-Rad Laboratories GmbH, Munich, Germany) prepared with Tris-buffered saline containing 0.1% Tween 20 (TTBS) for 1 h, incubated with goat polyclonal antibody against ACE2 (Santa Cruz Biotechnology Inc., Heidelberg, Germany, 1:250 diluted in 5% dry milk powder TTBS, 1 h), and then washed three times with TTBS (15 min each). Subsequently, the membranes were incubated with horseradish peroxidase-conjugated anti-goat IgG (DakoCytomation A/S, Glostrup, Denmark, 1:1000, 1 h) and washed three times. Specific immunoreactive proteins were detected by enhanced chemiluminescence (Amersham Biosciences GmbH, Freiburg, Germany). The bands on the X-ray film were quantified by densitometry scanning and expressed as percentage of the kidney protein signal.
2.5. Immunohistochemistry
2.5.1. Generation of monoclonal antibodies against ACE2 (clones: 7E7 and 1D3)
Monoclonal antibodies against the synthetic peptide AVGEIMSLSAAT (AA 403-414 of murine ACE2) have been raised. For immunization of the mice peptide was cross-linked with glutaraldehyde to albumin fraction V from bovine serum. BALB/cJ female mice were injected with the conjugate. Following four booster injections the spleen lymphocytes were fused with FO myeloma cells by using polyethylene glycol 1500 (Roche Diagnostics GmbH, Mannheim, Germany) following the manufacturers instructions.
The different hybridoma supernatants were screened for specific antibodies by using the synthetic peptide in the NC-test [26]. For production of monoclonal antibodies, positive hybridoma cells were grown in CELLine incubators (Integra Biosciences GmbH, Fernwald, Germany). The mouse monoclonal antibodies were affinity purified on a MabTrap G II column (Amersham-Pharmacia GmbH, Otelfingen, Switzerland) from cell culture supernatants. Immunoglobulin class and subclasses were determined with the Immuno Type Kit (Sigma–Aldrich Chemie GmbH, Taufkirchen, Germany).
2.5.2. Immunohistochemistry
Paraffin sections of mouse tissues were prepared and stained using standard histology procedures. For immunostainings, deparaffinized and rehydrated tissue slides were first treated for 30 min with 30% H2O2 to block the endogenous peroxidase. After rinsing in ddH2O and soaking in PBS for 5–10 min, slides were treated with 10% (w/v) BSA in PBS to eliminate non-specific protein binding sites. The slides were then exposed (overnight, 4 °C) to the monoclonal ACE 2 antibodies (clone 7E7, 1D3) at concentrations of 1 and 4 μg/ml, respectively. After removing excess antibody, slides were treated with biotin-labeled anti-mouse (Dianova GmbH, Hamburg, Germany) antibody for 30 min at 37 °C and finally with horse-radish peroxidase (HRP) labeled streptavidine (Zymed Laboratories Inc., San Francisco, USA) for 20 min at 37 °C. After washing, slides were incubated in aminoethylcarbazol (Sigma–Aldrich Co., St. Louis, USA) for 10 min at room temperature. Slides were counterstained with hematoxylin, and cover slipped according to conventional procedures. Slides were examined under a conventional microscope after removing the excess substrate in ddH2O. Negative controls were performed without the primary antibody, just applying dilution buffer of the primary antibody.
2.6. Statistics
Data were analyzed by t-test using SPSS11 software (SPSS Benelux BV, Gorinchem, The Netherlands). Each value was expressed as the mean ± S.E.M., and statistical significance was accepted for P < 0.05.
3. Results
3.1. RNase protection assay
ACE2 mRNA could be detected in all investigated organs, but with profound distinction between different organs. In both species, only a low amount was found in ventricle, liver, testis, forebrain, and spleen (Fig. 1, Fig. 2 ), whereas in the lungs a moderate and comparable expression of ACE2 mRNA was found and set to 100%. The highest levels were found in the ileum of both species (Fig. 3 ). Between the species several differences in tissue specific expression of ACE2 mRNA were found. The expression in mouse was most pronounced higher than in rat in kidney (~31.9-fold), colon (~18.6-fold), and ileum (~12.0-fold) (Fig. 3), whereas in bladder (~2.5-fold) and ventricle (~2.1-fold) ACE2 expression in rat exceeded the mouse.
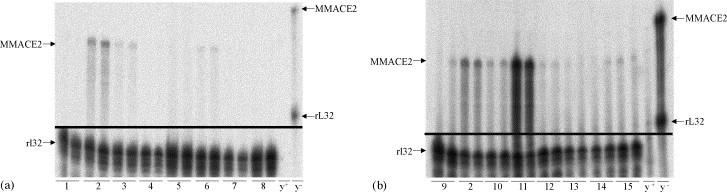
mRNA expression in different mouse tissues. Representative RPA of different tissues from C57Bl/6 mice. The specific bands for MMACE2 and the housekeeping mRNA rl32 are indicated with arrows on the left. The MMACE2 and rL32 probes are indicated with arrows on the right. (a) 1. ventricle, 2. kidney, 3. lung, 4. liver, 5. testis, 6. bladder, 7. forebrain, 8. spleen, y+, yeast plus RNase; y−, yeast without RNase; (b) 9. thymus, 2. kidney, 10. stomach, 11. ileum, 12. colon, 13. brainstem, 14. atrium, 15. adipose tissue; MM, Mus Musculus; y+, yeast plus RNase; y−, yeast without RNase.
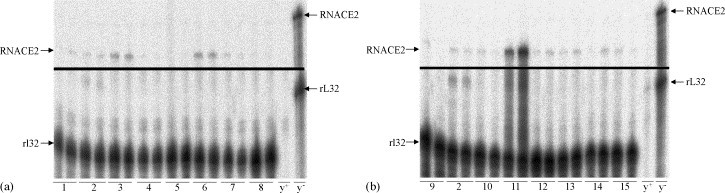
mRNA expression of different rat tissues. Representative RPA of different tissues from C57Bl/6 mice. The specific bands for RNACE2 and the housekeeping mRNA rl32 are indicated with arrows on the left. The RNACE2 and rL32 probes are indicated with arrows on the right. (a) 1. ventricle, 2. kidney, 3. lung, 4. liver, 5. testis, 6. bladder, 7. forebrain, 8. spleen, y+, yeast plus RNase; y−, yeast without RNase; (b) 9. thymus, 2. kidney, 10. stomach, 11. ileum, 12. colon, 13. brainstem, 14. atrium, 15. adipose tissue; RN, Rattus Norvegicus; y+, yeast plus RNase; y−, yeast without RNase.
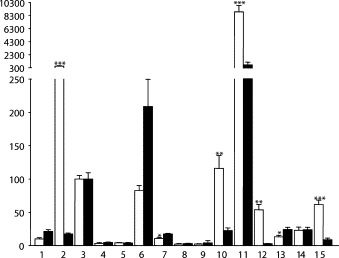
Quantification of the RPAs of mice (white columns) and rats (black columns). The mRNA amount of the lungs is set to 100% (n ≤ 4). The values are shown as mean + S.E.M. 1. ventricle, 2. kidney, 3. lung, 4. liver, 5. testis, 6. bladder, 7. forebrain, 8. spleen, 9. thymus, 10. stomach, 11. ileum, 12. colon, 13. brainstem, 14. atrium, 15. adipose tissue. *P < 0.05, **P < 0.01, ***P < 0.0001 compared mouse vs. rat.
3.2. ACE2 activity assay
In accordance with the RNA expression data, highest activity for ACE2 was found in the ileum of mouse and rat (Table 1 ), whereas the activity in the mouse was 3.2-fold higher. Lowest ACE2 activity was found for both species in spleen. Low activity was also found for liver of mice and thymus of rats. Corresponding to the differences on mRNA levels in the kidney the ACE2 activity was much higher in mice than in rats (~13.9-fold). The activity of ACE2 in the lung was different to mRNA and 2.6-fold higher in rats than in mice. In contrast to RPA data the activity in colon was comparable between both species.
Table 1
ACE2 activity in different tissues
Tissue | mmol Mca-AP-OH/mg protein/h | |||
---|---|---|---|---|
Mouse | Rat | |||
Mean | SEM | Mean | SEM | |
Ventricle | 0.1035 | 0.0161 | 0.1238 | 0.0156 |
Kidney | 11.7588 | 0.6394 | 0.8585 | 0.1042 |
Lung | 0.2615 | 0.0111 | 0.6690 | 0.1327 |
Liver | 0.0772 | 0.0078 | 0.2065 | 0.0419 |
Testis | 0.1276 | 0.0123 | 0.1946 | 0.0341 |
Bladder | 0.5557 | 0.0758 | 0.7404 | 0.0876 |
Forebrain | 0.4265 | 0.0563 | 0.3729 | 0.0098 |
Spleen | 0.0273 | 0.0049 | 0.0236 | 0.0072 |
Thymus | 0.1380 | 0.0453 | 0.0555 | 0.0054 |
Stomach | 0.4447 | 0.0222 | 0.4999 | 0.0437 |
Ileum | 51.5659 | 14.6782 | 16.3141 | 1.4390 |
Colon | 0.1348 | 0.0419 | 0.1823 | 0.0339 |
Brainstem | 0.3519 | 0.1396 | 0.3507 | 0.0381 |
Atrium | 0.1076 | 0.0268 | 0.1617 | 0.0161 |
Adipose tissue | 0.3578 | 0.0905 | 0.9696 | 0.3500 |
It is shown that the amount of Mca-AP generated from Mca-APK(Dnp) by 1 mg of protein from different tissues of mice and rat within 1 h (n ≥ 4).
3.3. Western blot
Using a commercial polyclonal antibody in western blot for the quantification of protein levels in mouse and rat tissues (Fig. 4 ) a pattern completely different from RNA expression and ACE2 activity was found. A moderate and comparable expression could be detected in the kidney of both species and was set to 100%. Thus, the highest amount of protein could be detected in atrium of both species (mouse: 124.5%; rat: 131.5%) and ventricle (mouse: 131.7%; rat: 143.3%). For the mouse less ACE2 protein was found in lung (19.7%) and testis (28.7%), whereas no protein was detectable in these two tissues in rat. In thymus (mouse: 44.4%; rat: 50.6%) and forebrain (mouse: 87.9%; rat: 80.7%) of both species a moderate expression was detectable, whereas no ACE2 protein was found in spleen of mouse and rat.
3.4. Immunohistochemistry
To further clarify the discrepancy between RPA and activity on one side and western blot on the other, immunohistochemistry was performed in lung, kidney (Fig. 5 ), and testis (data not shown) of mice and rat with new monoclonal ACE2 antibodies (clones 7E7 and 1D3), we generated. The antibodies were determined to belong to the IgG1 subclass. In the lungs of both species alveolar macrophages and type 2 cells (Fig. 5, upper row) were stained with both monoclonal ACE2 antibodies (data for clone 1D3 not shown). The epithelium of the renal tubuli was strongly stained (Fig. 5, lower row, left) in the kidney of mice. In rats only a weak signal, but the same pattern as in mouse, was detected, what aligned with mRNA and ACE2 activity (Fig. 5, lower row, right).
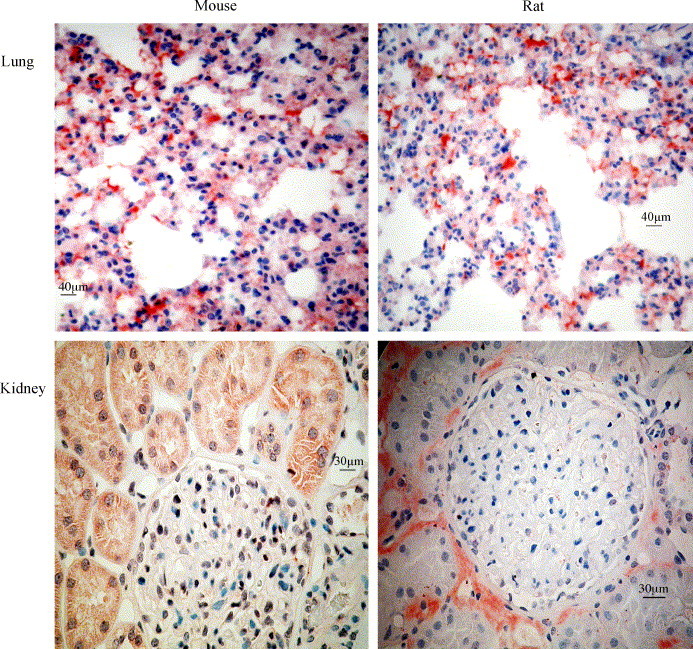
Immunohistochemical visualization of ACE2 positive cells. Sections of lungs (upper row) and kidneys (lower row) from mouse (left panel) and rat (right panel). In the lungs of both species alveolar macrophages and type 2 cells were stained positive. The tubulus epithelium in mouse kidney was stained positive, whereas in the rat kidney only weak staining was seen.
4. Discussion
In recent investigations it was shown that peptidases like ACE and NEP are important regulators of cardiovascular and endothelial function as well as myocardial remodelling [1], [7], [36], [41]. Consequently, after its discovery in 2000, ACE2 became an enzyme of interest for scientific investigation of its impact in cardiovascular physiology and pathophysiology [11], [12], [37]. To elucidate some of its physiological functions we investigated the tissue distribution of mRNA and protein in a variety of tissues of C57Bl/6 mice and Sprague-Dawley rats. While we could see correlating patterns of mRNA and ACE2 activity in most of the examined tissues, we also found significant divergences between the investigated species. The huge difference between mRNA and protein levels in the lung may be due to shedding as demonstrated for ACE [4], [12], [32]. This shedding leads to an increased secretion of ACE2 and lowered its protein content in the lung by even high mRNA expression. The significant differences that we found between the species on ACE2 protein and mRNA levels in kidney could be explained by the varying interspecies regulation and expression of peptidases, as shown in the literature for NEP activity in rat and rabbit kidneys [14].
Comparing our mRNA and activity data with the western blot pattern, we have to conclude that the commercial polyclonal antibody is not detecting ACE2 protein in organ homogenates and is not suitable for ACE2 staining. In contrast, using immunohistochemistry our new monoclonal ACE2 antibodies produce staining patterns comparable to our mRNA and activity data.
We have shown that ACE2 expression in rodents is highest in ileum among the examined organs. It was shown for other peptidases of RAS like ACE and NEP that they are also present at high levels in the intestine [29]. However, the distinct function of these peptidases in the ileum is not yet known. Further investigations have to clarify the physiological and pathophysiological functions of the peptidases in the gastrointestinal tract.
Beside its physiological function as a peptidase, ACE2 is used by coronavirus as a co-receptor in severe acute respiratory syndrome (SARS) [30]. It was shown that the SARS coronavirus only can enter cells which express ACE2 [24]. ACE2 distribution in the small intestine, lung and vascular endothelium may offer a point of entry for the SARS coronavirus, but does not reflect its basic function [22], [30], [38].
Interestingly, the distribution patterns we found for mRNA and ACE2 activity contradict investigations using a commercial northern blot for detecting mRNA [12], [37] but have been confirmed by recent papers using RT-PCR [23]. This discrepancy may be a species-specific alteration of tissue distribution, since they used human tissue for northern blot, or it may be due to technique differences (commercial northern versus RPA and activity assay). The first possibility is at least supported by our finding that significant differences in ACE2 expression patterns exist between the close relatives mouse and rat.
Recent investigations revealed biological activity for angiotensin peptides other than AngII, like Ang-(1–7) [16], [33], [34]. ACE2 can generate Ang-(1–7) by cleaving the C-terminal amino acid from AngII [40]. ACE2 is also involved in another pathway leading to the generation of Ang-(1–7). It cleaves AngI to Ang-(1–9) [12]. Ang-(1–9) is then hydrolyzed by ACE to Ang-(1–7). We demonstrated that Ang-(1–7) is an endogenous ligand for the G protein-coupled receptor (GPCR) Mas [35]. mRNA of the GPCR Mas was found at high levels in testis and certain brain regions and at moderate levels in kidney and heart [2], [3], [31]. It was shown that high concentrations of Ang-(1–7) were present in heart, kidney, and brain [5], [6], [8], [28]. In recent investigations, it was demonstrated that ACE2, Mas, and its endogenous ligand Ang-(1–7) are present in the same cells of the kidney [9]. As we recently postulated, this indicates a relevant impact of the ACE2/Ang-(1–7)/Mas axis on blood pressure regulation and cardioprotection.
Actual investigations indicate an upregulation of ACE2 in heart failure, pointing to the relevance of ACE2 in cardiac function [20], [32], [43]. However, there was a high incidence of sudden death in animals overexpressing ACE2. Electrophysiology revealed severe, progressive conduction and rhythm disturbances with sustained ventricular tachycardia that progressed to fibrillation and death [13]. While anti-arrhythmic actions were demonstrated for Ang-(1–7) in low concentrations (0.22 nM) by stimulating its own receptor, 100-fold higher concentrations of Ang-(1–7) lead to arrhythmias by stimulating the AT1 receptor [17], [19]. Therefore, the overexpression of ACE2 may lead to a high increase in the production of Ang-(1–7), turning its cardioprotective actions into effects causing arrhythmias by unspecific AT1 stimulation. In future studies, the actions of Ang-(1–7) and its concentration-dependency on ACE2 expression on heart rhythm have to be proven in in vivo experiments with AT1- and Mas-deficient animals.
Our data on tissue and species-specific ACE2 expression point to the fact that the RAS becomes increasingly complex. Since we identified an expression pattern markedly different from ACE, we conclude that the expression levels of the involved peptidases like ACE, ACE2, and NEP that generate and/or degrade the bioactive peptides of the RAS are predictive of either the occurrence of vasoconstriction or dilatation or the dominance of pathophysiological stimuli over beneficial conditions.
Acknowledgements
Florian Gembardt is paid by a grant from the “Deutsche Forschungsgemeinschaft” (German Research Foundation) [GRK865]. This study was also supported by the “Stiftung zur Förderung der wissenschaftlichen Forschung an der Universität Bern”. We thank Helmut Würdemann and Susanne Gygax for their technical assistance.
References
Citations & impact
Impact metrics
Citations of article over time
Alternative metrics
Article citations
Causal linkage between angiotensin-converting enzyme 2 and risk of lung cancer: a bidirectional two-sample Mendelian randomization study.
Front Med (Lausanne), 11:1419612, 08 Jul 2024
Cited by: 0 articles | PMID: 39040892 | PMCID: PMC11260785
Alternative Renin-Angiotensin System.
Hypertension, 81(5):964-976, 16 Feb 2024
Cited by: 3 articles | PMID: 38362781
Review
Neurological manifestations of SARS-CoV-2: complexity, mechanism and associated disorders.
Eur J Med Res, 28(1):307, 30 Aug 2023
Cited by: 11 articles | PMID: 37649125 | PMCID: PMC10469568
Review Free full text in Europe PMC
ACE2, ACE, DPPIV, PREP and CAT L enzymatic activities in COVID-19: imbalance of ACE2/ACE ratio and potential RAAS dysregulation in severe cases.
Inflamm Res, 72(8):1719-1731, 03 Aug 2023
Cited by: 1 article | PMID: 37537367
Renin-Angiotensin System: Updated Understanding and Role in Physiological and Pathophysiological States.
Cureus, 15(6):e40725, 21 Jun 2023
Cited by: 6 articles | PMID: 37350982 | PMCID: PMC10283427
Review Free full text in Europe PMC
Go to all (138) article citations
Data
Similar Articles
To arrive at the top five similar articles we use a word-weighted algorithm to compare words from the Title and Abstract of each citation.
Characterization of renal angiotensin-converting enzyme 2 in diabetic nephropathy.
Hypertension, 41(3):392-397, 24 Feb 2003
Cited by: 238 articles | PMID: 12623933
Distinct roles for ANG II and ANG-(1-7) in the regulation of angiotensin-converting enzyme 2 in rat astrocytes.
Am J Physiol Cell Physiol, 290(2):C420-6, 21 Sep 2005
Cited by: 128 articles | PMID: 16176966
Effect of angiotensin-converting enzyme inhibition and angiotensin II receptor blockers on cardiac angiotensin-converting enzyme 2.
Circulation, 111(20):2605-2610, 16 May 2005
Cited by: 1049 articles | PMID: 15897343
ACE2, a new regulator of the renin-angiotensin system.
Trends Endocrinol Metab, 15(4):166-169, 01 May 2004
Cited by: 211 articles | PMID: 15109615 | PMCID: PMC7128798
Review Free full text in Europe PMC