Abstract
Free full text

The Fas-Associated Death Domain Protein (FADD) is Required in Apoptosis and TLR-induced Proliferative Responses in B Cells1
Abstract
The Fas-associated death domain protein FADD/Mort1 is a signaling adaptor protein which mediates the activation of caspase 8 during death receptor-induced apoptosis. Disruption of FADD in germ cells results in death receptor-independent embryonic lethality in mice. Previous studies indicated that in addition to its function in apoptosis, FADD is also required in peripheral T cell homeostasis and TCR-induced proliferative responses. In this report, we generated B cell-specific FADD-deficient mice and showed that deletion of FADD at the pro-B cell stage had minor effects on B cell development in the bone marrow, and resulted in increased splenic and lymph node B cell numbers and decreased peritoneal B1 cell numbers. As in T cells, a FADD deficiency inhibited Fas-induced apoptosis in B cells. However, B cell proliferative responses induced by stimulation of the BCR and CD40 using anti-IgM or anti-CD40 antibodies were unaffected by the absence of FADD. Further analyses revealed that FADD-deficient B cells were defective in proliferative responses induced by treatments with dsRNA and LPS which stimulate TLR3 and TLR4 respectively. Therefore, in addition to its apoptotic function, FADD also plays a role in TLR3- and TLR4-induced proliferative responses in B cells.
Introduction
In mammals, T cells are generated in the thymus from bone marrow-derived progenitor cells, whereas conventional B cells develop in the bone marrow and mature in the periphery. During lymphocyte development, random DNA rearrangements required for antigen receptor gene expression give rise not only to antigen-specific lymphocytes but also to those recognizing self. In order to avoid autoimmune diseases, self-reactive lymphocytes must be eliminated through mechanisms such as apoptosis (1-3). In an immune response, signaling through antigen receptors induces lymphocyte proliferation and differentiation, and in the subsequent contraction phase, effector lymphocytes are removed by apoptosis in order to prepare the immune system for future responses to distinct antigens (2, 4). The Fas (or Apo-1) receptor can induce lymphocyte apoptosis, and mutations in the Fas gene cause an autoimmune-lymphoproliferative syndrome (ALPS) (5-7). Upon triggering of apoptosis by Fas ligand engagement, the intracellular death domain (DD) of Fas binds to the DD of FADD (8-10), and the death effector domain (DED) of FADD interacts with the DED present in pro-caspase 8 (FLICE or MACH) (11, 12). In addition to Fas, other death receptors such as TNF receptor I (TNF-R1) and TNF-related apoptosis-inducing ligand receptors (TRAIL-Rs) also require FADD as an adaptor for apoptotic signal transduction (13-16). Regulation of death receptor-induced apoptotic signaling involves the cellular FLICE-like inhibitory protein (c-FLIP, Casper, IFLICE, CASH, FLAME-1, MRIT, CLARP, or usurpin), which is homologous to caspase 8 but lacks a protease activity (17-19).
Aside from ALPS, Fas mutant mice otherwise show normal embryonic and postnatal development. Mice lacking either TNF-R1 or TRAIL-R also develop normally and show no sign of ALPS (20, 21). Surprisingly, deletion of FADD, caspase 8, or c-FLIP results in early embryonic lethality in mice (22-25). T cells lacking FADD or expressing a FADD dominant negative mutant are defective in TCR-induced proliferation and Fas-induced apoptotic responses (22, 26-29). T cell-specific deletion of caspase 8 or c-FLIP resulted in similar proliferation defects in peripheral T cells (30-33). Importantly, mutations in the human caspase 8 gene caused both impaired apoptosis and immunodeficiency (34, 35). Therefore, it appears that FADD, caspase 8, and c-FLIP constitute a novel signaling complex with a dual function in both apoptosis and TCR-induced proliferation signaling.
An absence of FADD or c-FLIP in embryonic stem cells in FADD-/-→RAG-1-/- chimeric mice severely impaired T cell development (22, 33), whereas the deletion of FADD, caspase 8 or c-FLIP after T lineage commitment had no obvious effect on intrathymic development (26, 30, 32). Neither FADD-/-→RAG-1-/- nor c-FLIP-/-→RAG-1-/- chimeras contain detectable B cells, indicating that FADD is essential for B cell lymphopoiesis (22, 33). However, the temporal requirement of FADD during B lineage development has not yet been determined. There are at least three subsets of mature B cells in mice (36, 37). The recirculating follicular B cells are usually found in lymphoid follicles of the spleen and lymph nodes. Marginal zone B cells are derived from transitional B cells (38), and reside primarily around the periphery of the splenic lymphoid nodules. The development of these conventional (or B2) B cells is initiated in the bone marrow and continues in peripheral lymphoid organs such as the spleen (39). Finally, B1 B cells are believed to be fetal liver-derived, long-lived and present mainly in the peritoneal and pleural cavities (40, 41).
The B cell antigen receptor (BCR) induces intracellular signaling processes analogous to those induced by the TCR. Given the proliferation defect present in FADD-deficient T cells, it was of interest to determine whether FADD is required for proliferative responses induced by BCR signaling. B cells can also be induced to proliferate by stimulation of CD40 and by certain macromolecules present in microbial pathogens which can trigger intracellular signaling through Toll-like receptors (TLRs). These evolutionarily conserved “pattern recognition” receptors play a critical role in innate immune responses (42, 43). TLRs contain an intracellular TIR (TLR/IL-1 receptor) domain which interacts with the TIR of the adaptor protein MyD88. The DD of MyD88 binds that of IRAK serine/threonine kinases (44, 45). Although MyD88 is believed to be utilized by all TLRs during signaling, other adaptor proteins, such as TRIF, mediate alternative pathways, particularly those induced by TLR3 and TLR4 (46).
In this study, we generated B cell-specific FADD-deficient mice using the Cre-LoxP system to determine the temporal requirement of FADD in B cell lymphopoiesis and the FADD function in apoptosis and proliferation responses in B cells. Analysis of these mice revealed that FADD is required for Fas-induced apoptosis in B cells in order to maintain homeostasis in the spleen and lymph nodes. Furthermore, FADD plays a role in B cell proliferative responses induced by TLR3 and TLR4.
Materials and Methods
Generation of B cell-specific FADD-/- mice
FADD+/- and FADD:GFPflox mice were reported previously (22, 26) and crossed to obtain FADD+/- FADD:GFPflox mice, which were then backcrossed to FADD+/- mice to produce viable FADD-/- FADD:GFPflox mice. To generate B cell-specific FADD-deficient mice, CD19-Cre transgenic mice, kindly provided by Dr. Klaus Rajewsky (Harvard Medical School, Boston, MA), were crossed with FADD+/- mice. The resulting FADD+/- CD19-Cre mice were backcrossed to B6 mice. Co-segregation of the FADD knockout allele and CD19-Cre allele in the offspring indicates that crossovers on the chromosome 7 resulted in linkage of these two gene alleles on the same chromatid in the parental mice. These were then crossed with FADD-/- FADD:GFPflox mice to generate B cell-specific FADD-deficient FADD-/- FADD:GFPflox CD19-Cre mice. Mouse genotypes with regard to FADD alleles were determined by Southern blot analyses using mouse tail DNA and a 0.4-kb probe to detect the endogenous and knockout FADD alleles and FADD:GFP as different sizes of EcoR I fragments as described elsewhere (26). The CD19-Cre gene was detected by PCR using oligo primer DNA sequences (GTCTGAAGCATTCCACCGGAA and CTGCGTGCAATCCATCTTGTT) and a protocol (1 min at 94°C, 1 min at 63°C, and 2.5 min at 72°C for 35 cycles) provided by Dr. R. Richert (Burnham Institute, San Diego, CA). All animal studies were approved by the Institutional Review Board at Thomas Jefferson University.
Flow cytometry
Single cell suspensions were prepared from the bone marrow, spleen, lymph nodes, or peripheral blood. Red blood cells were depleted by hypotonic lysis. To determine GFP expression, cells were subjected to flow cytometric analysis using a Coulter Epics XL analyzer (Beckman Coulter, Fullerton, CA). For cell surface protein staining, appropriate fluorochrome-conjugated Abs were added to single cell suspensions in PBS with 1% FBS and 0.1% sodium azide, followed by incubation on ice for 20-30 min and two washes with PBS. Peritoneal cavity cells were harvested from individual mice by flushing two times with 5 ml each of RPMI 1640 medium (Cellgro, Herndon, VA). After counting, cells were resuspended in staining buffer (3% BSA, 0.5 mM EDTA, 0.02% sodium azide) and stained with appropriate antibodies. The following Abs were used: Tri-Color-conjugated anti-CD4, CD8, B220, and biotinylated anti-CD23 Abs (Caltag, Burlingame, CA); PE-conjugated anti-AA4.1, and TLR-4 Abs (eBioscience, San Diego, CA); PE-conjugated anti-CD3, CD21, CD25, c-Kit, and biotinylated anti-CD19 (clone 1D3), CD5 (clone 53-7.3), CD11b (Mac-1, clone M1/70) Abs, streptavidin-CyChrome (BD Bioscience, San Diego, CA); PE-conjugated anti-mouse IgM Abs (clone II/41, Jackson Immunoresearch Laboratories); Biotinylated anti-IgD (clone 11-26, Southern Biotech, Burmingham, AL). Cells were analyzed using a Coulter Epics XL cytometer (Beckman Coulter, Fullerton, CA), and data analyzed with WinMDI (C. J. Trotter, The Scripps Institute, La Jolla, CA) and FlowJo softwares (Treestar, San Carlos, CA). To purify B cells by sorting, single cell suspensions were prepared as described above and stained with Tri-color-anti-B220 or PE-anti-CD19 Abs. CD19+ or B220+GFP- cells were isolated using a MoFlo high-speed cell sorter (Dako Cytomation, Glostrup, Denmark).
Immunohistology
Spleen and lymph nodes cryostat sections (5-6 μm) were prepared and immunohistology was performed as previously described (47). Abs were detected using the Vector Blue Alkaline-phosphatase Substrate kit III and the Vector NovaRed kit for peroxidase (Vector Laboratories). HRP-anti-CD4 (clone GK1.5) Abs were made in house. The stained sections were analyzed using a light microscope (Leitz Diaplan; Axioplan Universal Microscope; Carl Zeiss MicroImaging, Inc.), and digital images were captured using an Eastman Kodak Co. camera.
Cell Culture
B cells were cultured at 37°C in a 5% CO2 incubator in RPMI 1640 (Mediatech, Herndon, VA) supplemented with 100 U/ml penicillin, 100 μg/ml streptomycin, 1 mM glutamine, 50 μM β-mercaptoethanol (Sigma, St. Louis, MO), and 10% FBS (HyClone Laboratories, Logan, UT).
Fas-induced apoptosis assay
Sorted mutant and control B cells were seeded to 96-well plates (105/well) in 100 μl RPMI medium. sFasL (Alexis Biochemical, San Diego, CA) was added at various concentrations in triplicates. Anti-FLAG Abs M2 (Sigma, St.Louis, MO) were then added (1 μg/ml) to the culture. After a 16 h-incubation, cells were transferred to flow tubes and subject to analysis using a flow cytometer after addition of PI (1 μg/ml).
Serum Igs
Concentrations of serum Igs were determined by ELISA. 96-well flat-bottom plates (Thermo Labsystems, Franklin, MA) were coated with 10 μg/ml of goat anti-mouse capture antibody (Southern Biotech, Burmingham, AL) in PBS overnight at 4°C. Plates were washed with 0.05% Tween-20 in PBS and blocked with 1% BSA in PBS for at least 1 h at room temperature. After washing, serum dilutions of 1:10,000, 1:30,000, 1:90,000 and 1:270,000 were made and added to appropriate wells for 1 h at room temperature. After washing, HRP-conjugated goat anti-mouse Igs were added for 1 hour at room temperature, then thoroughly washed. Substrate solution was prepared by mixing 10 ml citrate substrate buffer (525 mg citric acid in 50 ml H2O) with 0.2 ml of 2,2'-azino-bis(3-ethylbenzthiazoline-6-sulfonic acid) stock solution and 100 μl of 3% H2O2. Substrate solution was added to the wells and absorbance was measured at 405 nm at 10 min and 20 min. Ig concentrations were determined by using a standard curve obtained from every ELISA plate.
B cell proliferation and activation marker assays
For [3H] thymidine incorporation assays, sorted B cells were plated in triplicates in 96-well round-bottom plates (105/well) in 100 μl RPMI 1640-10% FBS, and cultured for 40 h in the presence of LPS, poly (I:C) (Sigma-Aldrich, St. Louis, MO), CpG ODN 1826 (Coley Pharmaceutical, Wellesley, MA), F(ab’)2 of goat-anti- mouse IgM (μ chain, Jackson ImmunoResearch, West Grove, PA), or anti-CD40 Abs (Clone FGK45, Alexis Biochemical, San Diego, CA) at indicated concentrations. 0.5 μCi [3H] thymidine (ICN Biochemicals, Irvine, CA) was added into each well after 36 h, followed by additional 8 h incubation. Incorporation of [3H] thymidine was determined using a Wallac beta counter (PerkinElmer, Wellesley, MA).
To analyze cell division by CFSE labeling, B220+GFP- B cells were sorted from the spleen and lymph nodes, labeled with 5 μM CFSE (Molecular Probe, Eugene, OR) in PBS plus 5% FBS for 5 min at 37°C in dark, and washed three times with 5 ml of RPMI 1640-10% FBS. These labeled cells were then stimulated with LPS (10 μg/ml) or anti-IgM Abs (10 μg/ml) for 72, 96 and 120 h, and analyzed using a flow cytometer. 7-aminoactinomycin D (7-AAD, BD Pharmingen) was added (0.83 μg/ml) to CFSE-labeled B cells at 72 h after stimulation with LPS, to detect cell death by two-color flow cytometry.
To detect CD54, CD86, and MHC class II up-regulation, sorted mutant and control B cells were cultured with LPS (10 μg/ml) in 96-well round-bottom plates as indicated above. After16 h, cells were washed once with 1 ml of staining buffer, and then stained on ice for 30 min with CD86-PE (GL1), CD54-PE (YN1/1.7.4; eBioSciences, San Diego, CA), or I-Ab-FITC (AF6-120.1; BD Bioscience, San Diego, CA). The cells were then washed once in PBS, resuspended in 200 μl PBS and analyzed by flow cytometry.
B cell survival/death assays
B cells were isolated from the spleen and lymph nodes by high speed sorting, resuspended in complete medium with or without LPS, and seeded (105 cell/well) into 96-well round-bottom plates. At indicated times, live cells were detected as those excluding PI (1 μg/ml) in flow cytometry assays.
Western blot analysis
To detect NF-κB, Erk, Jnk, and Akt activation, sorted B cells (2-5 × 106) were stimulated at 37°C in 0.5 ml RPMI medium-10% FBS and LPS (10 μg/ml) for the times indicated. Cells were washed once with ice-cold PBS and lysed for 3 min in an ice-cold buffer (1% Titon X-100, 50 mM Tris-HCl pH 8.0, 150 mM NaCl, 20 mM EDTA, 1 mM Na3VO4, 1 mM NaF, 0.1% SDS, 1 mM EDTA, 1 mM PMSF, 0.7 μg/ml pepstatin and complete protease inhibitor cocktail). Total proteins (10-25 μg) were denatured by boiling for 3 min in SDS-containing sample buffer, separated by 10% SDS/PAGE, and blotted onto nitrocellulose membranes. Blots were incubated with 5% BSA-TBST (20 mM Tris-HCl pH7.6, 137 mM NaCl, 0.1% Tween 20) for 1 h at room temperature, and then overnight at 4°C with antibodies specific for IκB, Erk1/2, or phosphorylated forms of Erk1/2, Akt (clone 193H12), and Jnk (Cell Signaling, Beverly, MA) at 1:1000 dilution per manufacturer's recommendation. After three washes, membranes were incubated with horseradish peroxidase-conjugated goat anti-rabbit Igs (Pierce, Rockford, IL) at 1:2000 dilution in 5% BSA in TBST for 1 h at room temperature. The Western Lighting Chemiluminescence Reagent Plus (PerkinElmer, Boston, MA) was used for signal detection with X-ray films (Kodak, Rochester, NY). Anti-TLR3 Abs were from Santa Cruz Biotechnology (Santa Cruz, California).
Results
Generation of B cell-specific FADD-deficient mice
Inactivation of the mouse FADD gene upon deletion of the promoter region and the first coding exon (middle, Fig. 1A) results in homozygous FADD knockout (FADD-/-) mice that die during midgestation (22). We recently showed that a FADD:GFP fusion gene reconstituted normal embryonic and lymphocyte development in FADD-/- mice lacking the endogenous FADD (26). FADD:GFPflox contains two loxP sites flanking the coding region (bottom, Fig. 1A) and can be deleted specifically in T cells using the Lck-Cre transgene (26). In order to induce deletion of FADD:GFP specifically in the B lineage, we used CD19-Cre mice in which the Cre recombinase gene is expressed in B cells by using the CD19 promoter (middle, Fig. 1A) (48). The CD19 and FADD genes are closely linked, being approximately 10 centimorgan apart on the mouse chromosome 7 (top, Fig. 1A). Therefore, it was necessary to cross the CD19-Cre allele onto the mouse chromatid containing the FADD knockout allele (middle, Fig. 1A), in order to delete FADD:GFP in FADD-/- mice. For this purpose, CD19-Cre mice were first crossed with viable heterozygous FADD+/- mice, and the resulting FADD+/- CD19-Cre mice were backcrossed with C57BL/6 (B6) mice. Co-segregation of the CD19-Cre and FADD knockout alleles in the offspring would indicate that these two genes are linked on the same chromatid in the parental FADD+/- CD19-Cre mice due to homologous recombnation. The expected 10% of the mice typed were of such a genotype and subsequently crossed with FADD-/- FADD:GFPflox mice to generate FADD-/- FADD:GFPflox CD19-Cre mice.
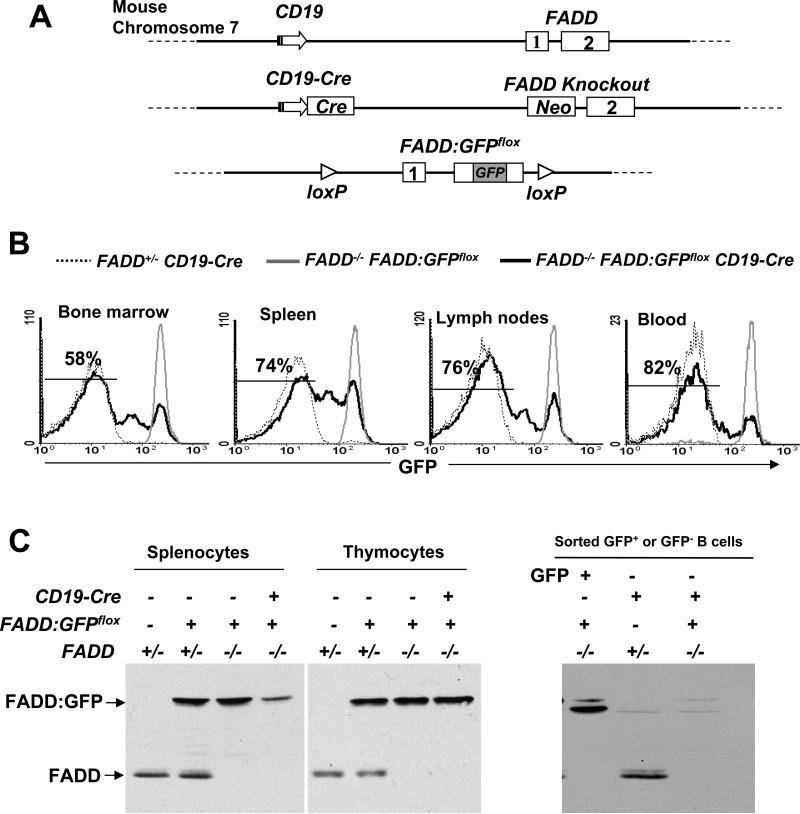
(A) Diagrammatic scheme in generating B cell-specific FADD-/- mice. The CD19 locus is closely linked to FADD on mouse chromosome 7 with a ~10 centimorgan distance (top). The CD19-Cre allele (48) was crossed to the FADD knockout allele (22) (middle) by mouse mating in order to delete FADD:GFPflox specifically in B cells in FADD-/- mice. The promoter of CD19 (arrows), the neomycin-resistant gene (neo), exons of FADD (boxes) and GFP are indicated. Drawings are not to scale. (B) Flow cytometric analysis of FADD:GFP deletion in B cells. Single cell suspensions were prepared from the bone marrow, spleen, lymph nodes, and peripheral blood, and stained for CD19. The indicated percentages of GFP- cells in the CD19+ population of FADD-/- FADD:GFPflox CD19-Cre mice were determined by flow cytometry. Cells from FADD+/- CD19-Cre and FADD-/- FADD:GFPflox mice were used as GFP- and GFP+ controls, respectively. Histograms are from one experiment using one mouse of each genotype, and are representative of 14 independent experiments. (C) Total thymocytes and splenocytes (left) or sorted GFP- B cells (right) from FADD-/- FADD:GFPflox CD19-Cre mice were analyzed by western blotting using anti-FADD antibodies. FADD+/-, FADD+/- FADD:GFPflox, FADD-/- FADD:GFPflox, FADD+/- FADD:GFPflox CD19-Cre mice were used as controls. The absence of FADD:GFP in GFP- cells were confirmed in three independent experiments.
To detect the deletion of FADD:GFP in B cells, FADD-/- FADD:GFPflox CD19-Cre mice were analyzed by flow cytometry for the presence of GFP-negative (GFP-) cells in the CD19+ population from the bone marrow, spleen, lymph nodes, and peripheral blood. FADD+/- CD19-Cre mice were used as GFP- controls. In GFP-positive (GFP+) control FADD-/- FADD:GFPflox mice which did not contain CD19-Cre, CD19+ B cells uniformly expressed the FADD:GFP fusion protein, as indicated by a distinct GFP+ peak in flow cytometric histograms (Fig. 1B). In FADD-/- FADD:GFPflox CD19-Cre mice, GFP-CD19+ B cells were detectable in the bone marrow (58%), spleen (74%), lymph nodes (76 %), and blood (82%; Fig. 1B). The gradually decreasing GFP+ and GFPlo (GFP-low) B cell populations indicate continuing FADD:GFP gene deletion and FADD:GFP protein turnover during B cell development and maturation. Western blot analyses of total cells from the spleen revealed reduced levels of the FADD:GFP fusion protein in FADD-/- FADD:GFPflox CD19-Cre mice, in comparison to control mice lacking CD19-Cre (lanes 3 and 4 on the left, Fig. 1C). As expected, the FADD:GFP protein was not reduced in thymocytes of FADD-/- FADD:GFPfloxCD19-Cre mice (lane 8 on the left, Fig. 1C). Western blot analyses of GFP- B cells purified by high-speed sorting indicated undetectable levels of FADD:GFP (lane 3 on the right, Fig. 1C), in comparison to control GFP- and GFP+ B cells isolated from FADD+/- CD19-Cre and FADD-/- FADD:GFPflox mice, respectively. Therefore, FADD-/- FADD:GFPflox CD19-Cre mice contain FADD-/- B cells and are referred to as B cell-specific FADD-deficient (or FADD-/-) mice hereafter. FADD+/- FADD:GFPflox CD19-Cre (FADD+/-) mice containing one allele of the endogenous FADD and CD19 genes were used as controls in the described analyses to follow.
B cell development in the bone marrow and periphery in B cell-specific FADD-/- mice
Cre gene expression is presumably initiated as early as in the pro-B cell stage, since it is integrated into a site immediately downstream of the CD19 gene promoter (48). To determine the deletion of FADD:GFP in various B cell subsets, total bone marrow cells were isolated from B cell-specific FADD-/- mice, stained for the B lineage-specific marker CD19 and stage-specific markers (c-Kit, CD25, IgM, and IgD), and analyzed by flow cytometry. Few GFP- cells (5.4%) were detected in the pro-B population (CD19+c-Kit+) (Fig. 2A). The percentages of GFP- were increasingly higher in the CD19+CD25+ pre-B (56.1%), IgM+IgD- immature (59%), and IgM+IgD+ mature (78.5%) populations, accompanied by a gradual decrease of GFP+ and GFPlo cell numbers in each (Fig. 2A). To analyze the effect of FADD deficiency on B cell development, the bone marrow B cell profile was determined by flow cytometry. When analyzed using stage-specific markers, B cell-specific FADD-/- mice were found to contain pro-B (CD19+c-Kit+), pre-B (CD19+CD25+), immature (IgM+IgD-), and transitional (CD19+AA4.1+) B cells at levels similar to those in the bone marrow in FADD+/- mice ( Fig. 2B). There appeared to be lower percentages of re-circulating IgD+IgM+ mature B cells in the bone marrow of B cell-specific FADD-/- mice than that in FADD+/- control mice (Fig. 2B). Therefore, B cell development in the bone marrow was not significantly affected in B cell-specific FADD-/- mice.
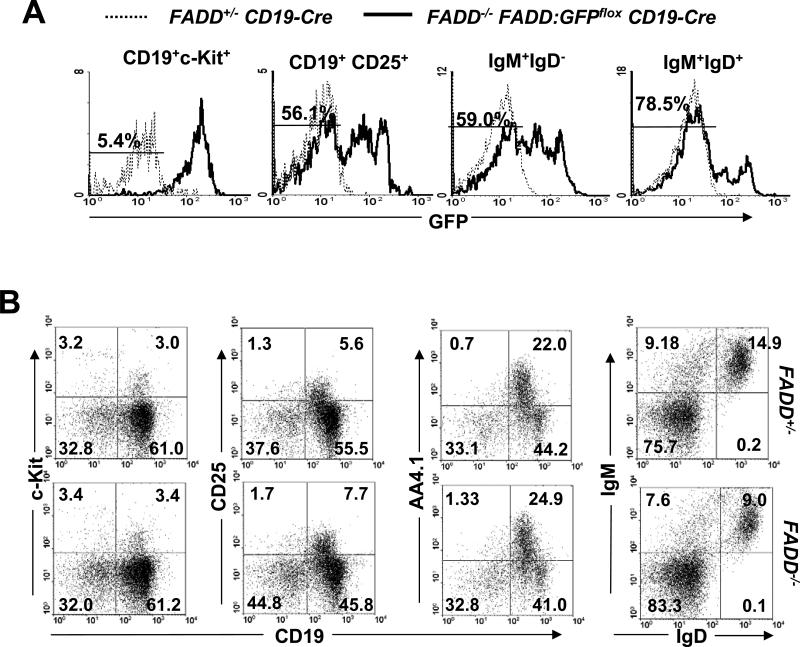
Flow cytometric analyses of the B cell development in the bone marrow in the absence of FADD. (A) Deletion of FADD:GFP in the B lineage in the bone marrow of FADD-/- FADD:GFPflox CD19-Cre mice was detected by the presence of the indicated percentages of GFP- B cells at various developmental stages. FADD+/- CD19-Cre mice were used as GFP- controls (dotted histograms). Histograms are from one pair of mice, representative of 14 independent experiments. (B) Pro-B (CD19+c-Kit+), pre-B (CD19+CD25+), immature (IgD-IgM+), transitional (CD19+AA4.1+), and re-circulating follicular (IgD+IgM+) B cells in the bone marrow of FADD-/- FADD:GFPflox CD19-Cre (FADD-/-) mice were detected by flow cytometry upon staining with the indicated stage-specific markers. Cells from FADD+/- FADD:GFPflox CD19-Cre mice (FADD+/-) were used as controls. Data shown are representative of 10 pairs of mice (age 2 to 6 month) analyzed.
As discussed above (Fig. 1B), B cell-specific FADD-/- mice contained a large proportion (70-80%) of FADD-/- (GFP-) B cells in the peripheral lymphoid organs. The spleen and lymph nodes of B cell-specific FADD-/- mice were generally larger in size (data not shown), and the total cellularity in these organs was significantly higher in mutant mice when compared to control FADD+/- mice (left, Fig. 3A). When analyzed by flow cytometry after staining for CD19 and the T cell marker CD3, B cell-specific FADD-/- mice were found to contain slightly higher percentages of splenic and lymph node B cells than that in FADD+/- mice (Fig. 3B). When total B cell numbers were compared, there was a significant increase of B cells in the spleen and lymph nodes of FADD-/- mice than in these peripheral lymphoid organs of FADD+/- mice (right, Fig. 3A). Peripheral T cell numbers in B cell-specific FADD-/- mice appeared to be higher in comparison to FADD+/- mice (data not shown). However, this phenotype does not appear to be statistically significant. Because of these phenotypes, B220 and CD4 immunohistochemistry was performed on sections of the spleen and lymph nodes isolated from mutant and control mice in order to examine the microarchitecture of these peripheral lymphoid organs. The B cell follicles in mutant mice were generally larger, particularly in lymph nodes, than in control mice (brown-B220, Fig. 3C), which is consistent with the data showing the increased number of B cells in these mutant peripheral lymphoid organs (Fig. 3A and B). However, the T cell compartment appears to be unaffected by a lack of FADD in B cells (blue-CD4, Fig. 3C).
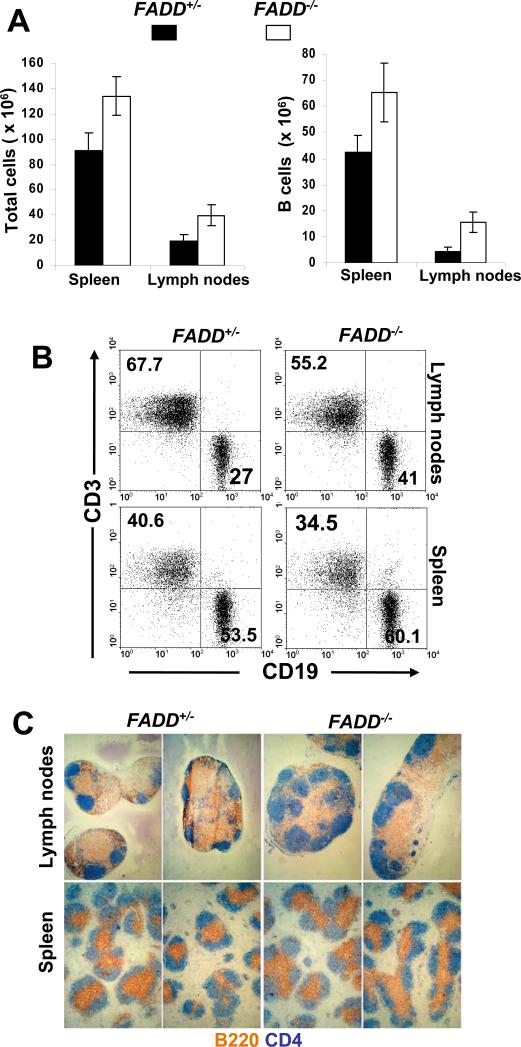
Analysis of the peripheral lymphoid system in B cell-specific FADD-/- mice. (A) Total (left) and CD19+ B (right) cell numbers in the spleen and lymph nodes from FADD-/- FADD:GFPflox CD19-Cre mice (FADD-/-) were compared to that of control FADD+/- FADD:GFPflox CD19-Cre mice (FADD+/-). Error bars indicate standard deviation from analysis of 7 mice of each indicated genotype. (B) Representative dot plots from flow cytometric analyses of CD3+ and CD19+ B cells in 15 mice of each genotype indicated. (C) Immunohistological analysis of cryosections of the spleen and lymph nodes. B cells were stained with anti-B220 antibodies (blue) and T cells were stained with anti-CD4 antibodies (brown). Data shown is representative of analysis of 5 mice of each indicated genotype.
To analyze B cell development in the periphery, splenocytes were isolated and analyzed by flow cytometry. The T1 (IgDloIgMhi) and T2 (IgDhiIgMhi) transitional B cells were present in B cell-specific FADD-/- mice in percentages similar to that in FADD+/- mice (Fig. 4A). The percentages of marginal zone and follicular B cells in the mutant spleen were also similar to that in control mice, as determined by staining for IgD and IgM, or CD21 and CD23 (Fig. 4A and B). These results indicate that the FADD deficiency did not affect B cell development in the periphery. Since the percentages of transitional, marginal zone, and follicular B cells in the periphery of B cell-specific FADD-/- mice are similar to those of FADD+/- mice (Fig. 4A and B), the higher total B cell numbers as seen in the spleen in FADD-/- mice (Fig. 3A) is likely due to increases in every B cell subpopulation, and not because of selective expansion of a particular B cell population. To analyze the effect of FADD deficiency on the B1 cell population, peritoneal cells were isolated from mutant and control mice and analyzed by flow cytometry. As shown in Fig. 4C, the percentage of IgM+Mac1+ B1 cells in B cell-specific FADD-/- mice (9.55% ± 3.84, n=10) was about 60% of that in control FADD+/- mice (16.46% ± 3.61, n=10).
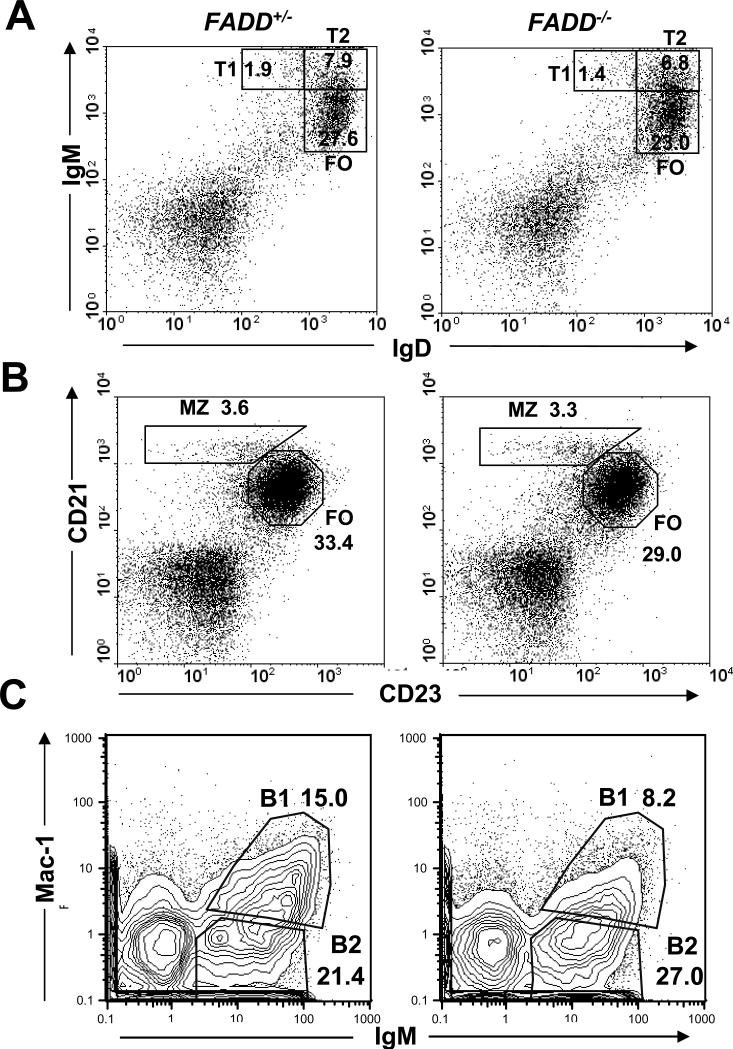
Flow cytometric analysis of B cell development in the spleen and peritoneal cavity in the B cell-specific FADD-/- mice. FADD+/- FADD:GFPflox CD19-Cre mice (FADD+/-) were used as controls. Spleen cells were stained for IgD and IgM (A), or for CD21 and CD23 (B), and peritoneal cells were stained for IgM and Mac-1 (C). Percentages of splenic transitional (T1 and T2), follicular (FO), marginal zone (MZ), and peritoneal B cells are indicated. Flow cytometric plots are representative of analysis of 5 mice of each indicated genotype.
Apoptosis and serum Ig analysis
FADD is critical for Fas-induced apoptosis in fibroblasts and T cells (16, 26). To determine Fas-induced cell death responses, B cells were sorted from the spleen and lymph nodes and cultured in the presence of FLAG-tagged soluble Fas ligand (sFasL) which was then cross-linked by using anti-FLAG antibodies. Both FADD+/- B cells and those lacking the endogenous FADD but expressing FADD:GFP isolated from FADD-/- FADD:GFPflox mice were killed in a dose-dependent manner by this treatment (Fig. 5A), indicating that FADD:GFP functions similarly to the endogenous FADD. In contrast, FADD-/- B cells were resistant to cell death induced by sFasL at various concentrations (Fig. 5A). Defective Fas signaling may lead to autoimmune diseases such as arthritis, higher levels of serum Igs, and increased mortality in aged mice. Although B cell-specific FADD-/- mice contained increased numbers of B cells defective in Fas-induced apoptosis, they showed no obvious joint swelling, a symptom of arthritis, and had survival rates similar to that of control littermates even when aged. We also collected sera of mice aged 2 to10 months and assayed for Ig levels. The data in Fig. 5B indicates that B cell-specific FADD-/- mice aged from 2 to 10 months contained lower average serum Ig levels in comparison with those in FADD+/- control mice. Therefore, FADD is essential for B cell apoptosis induced by Fas-induced signaling, yet a lack of FADD in B cells is not sufficient for induction of autoimmune diseases in mice.
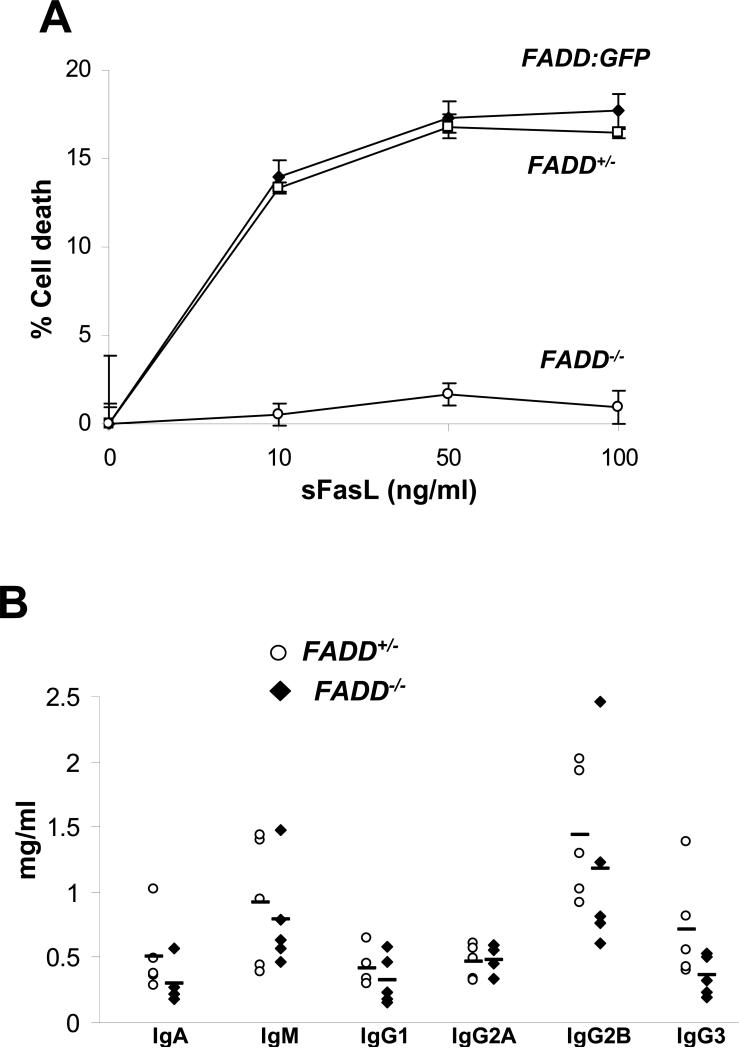
Apoptosis and serum Ig analyses. (A) FADD:GFP, FADD+/-, and FADD-/- B cells were sorted from FADD-/- FADD:GFPflox, FADD+/- FADD:GFPflox CD19-Cre and FADD-/- FADD:GFPflox CD19-Cre mice, respectively. Apoptosis in these cells was induced by stimulation with sFasL for 16 h, and measured by PI staining and flow cytometry. Error bars indicate standard deviation of triplicates from one experiment, and is representative of seven independent experiments using mice of different ages. (B) Sera were collected from 5 mice of the FADD+/- FADD:GFPflox CD19-Cre (open circle) and FADD-/- FADD:GFPflox CD19-Cre (filled diamond) genotypes, aged from 2 to 10 months. Ig levels were determined by ELISA. Bars indicate mean values for each Ig assay.
Proliferation responses in FADD-/- B cells
T cell-specific deficiency of FADD resulted in not only defective Fas-induced apoptosis, but also abnormal TCR-induced proliferation responses (26). We therefore examined FADD-/- B cell proliferation in response to various stimuli. Signaling through the BCR can be induced by treatment with F(ab’)2 anti-IgM antibodies. FADD+/- and FADD-/- B cells were isolated by sorting for the GFP- population from the spleen and lymph nodes, and treated with increasing concentrations of anti-IgM antibodies. As shown in Fig. 6A, no significant difference between control and mutant B cells was detected in [3H] thymidine labeling assays. Stimulation of CD40 using anti-CD40 Abs can also induce proliferation responses in B cells, which was unaffected by the absence of FADD (Fig. 6B). B cells have certain functions related to innate immunity, such as TLR expression and proliferative responses induced by various pathogen-associated macromolecules, which are natural ligands for TLRs. For example, LPS produced by Gram-negative bacteria, dsRNA, and CpG-containing DNA of the viral origin can stimulate TLR4, 3, and 9, respectively. To analyze TLR-mediated responses, FADD+/- and FADD-/- B cells were sorted from the spleen and lymph nodes of mutant and control mice and stimulated with increasing concentrations of poly (I:C), LPS, or CpG-containing DNA. As shown in Fig. 6C and D, the absence of FADD dramatically reduced B cell proliferation in response to poly (I:C), or LPS. In contrast, FADD-/- B cells proliferated as efficiently as FADD+/- B cells when stimulated with CpG-containing oligo DNA (Fig. 6E). Control oligo DNA only induced background responses in both mutant and control B cells (Fig. 6F). A FADD deficiency did not result in any obvious defects in the expression of TLR3 and TLR4 in B cells as determined by western blot and flow cytometric analyses (Fig. 6G and H). These results indicate that FADD plays a negligible role in proliferative responses induced by BCR, CD40, and TLR9, but is essential for those induced by TLR3 and TLR4.
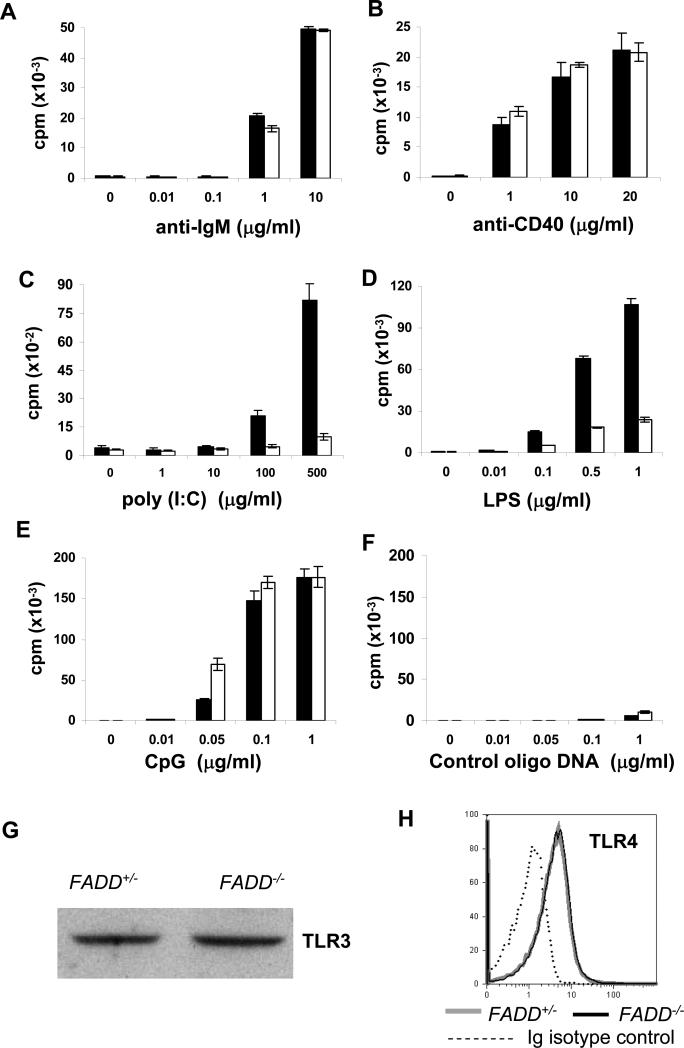
Analysis of B cell proliferation. FADD+/- (filled bars) and FADD-/- B cells (open bars) were sorted for the GFP- population from FADD+/- FADD:GFPflox CD19-Cre and FADD-/- FADD:GFPflox CD19-Cre mice, and stimulated in triplicates with anti-IgM Abs (A), anti-CD40 Abs (B), poly (I:C) (C), LPS (D), CpG-containing DNA (E), or nonspecific, control oligo DNA (F) at the concentrations indicated. Proliferation was indicated by the amount of [3H] thymidine incorporated (shown as counts per min on Y axis). Error bars indicate standard deviation of triplicates. Data shown are from one experiment and represent three to five independent experiments. (G) Expression of TLR3 expression was determined by western blotting. (H) Expression of TLR4 was determined by flow cytometry.
To further analyze the proliferation defects in FADD-/- B cells, cell division kinetics were determined using carboxyfluorescein diacetate succinimidyl ester (CFSE) to label intracellular molecules, as CFSE fluorescence intensity halves upon each cell division. FADD+/- and FADD-/- B cells were sorted from the spleen and lymph nodes, labeled with CFSE, and stimulated with LPS to induce proliferation. As shown in Fig. 7A (top), cell division potentials in FADD+/- and FADD-/- B cells were equivalent in response to BCR stimulation with anti-IgM antibodies, a result in agreement with [3H] thymidine labeling assays (Fig. 6A). However, FADD-/- B cells had a profound defect in cell division in comparison with control FADD+/- B cells in cultures stimulated with LPS stimulation for 72, 96, and 120 h (bottom, Fig. 7A). To analyze any intrinsic defects in survival due to FADD deletion, B cells were cultured in complete medium without any stimulation and cell survival was determined by propidium iodide (PI) exclusion and flow cytometry. No consistent difference between FADD-/- and FADD+/- B cells in survival capability was detected over a period of two days in culture in several independent experiments (Fig. 7B). We further analyzed B cell death during stimulation with LPS by PI uptake and flow cytometry. Similar cell death rates were detected in both mutant and control B cells during an initial 12 h stimulation (Fig. 7B). In the FADD+/- B cell culture stimulated with LPS, cell death peaked at 24 h and gradually decreased thereafter, whereas higher percentages of cell death were detected in FADD-/- B cells at these time points during the two day stimulation (Fig. 7B). In a different assay, we performed two-color flow cytomtetric analyses by staining the CFSE-labeled B cell with 7-AAD. Cell death in the B cell cultures were indidcated by 7-AAD positive populations. As shown in Fig. 7C, there was more cell death in FADD-/- than in FADD+/- B cell cultures stimulated by LPS. These results indicate that FADD-/- B cells do not appear to have intrinsic defects in ex vivo survival, but are not capable of complete division induced by LPS stimulation, thus resulting in increased cell death.

Cell division, survival and death analyses. (A) Purified FADD+/- and FADD-/- splenic and lymph node B cells were labeled with CFSE, and stimulated with anti-IgM Abs (10 μg/ml; top) or LPS (10 μg/ml, bottom), and division of B cells was determined by flow cytometry at 72, 96, and 120 h post stimulation. Percentages of divided cells were indicated. (B) To determine survival capability, sorted FADD-/- and FADD+/- control B cells were cultured in complete medium for two days, and at indicated times cells were analyzed by flow cytometry for viablility as indicated by PI exclusion. Cell death in mutant and control B cells stimulated with LPS (1 μg/ml) were determined similarly by flow cytometry. (C) Cell death and division were analyzed by two-color flow cytometry by 7-AAD staining of CFSE-labeled B cells stimulated with LPS for 72 h. Data shown are from one experiment using one pair of mice, and are representative of 3 independent experiments.
Analysis of activation markers and signaling processes induced by LPS stimulation
In normal B cells, LPS induces expression of CD54 (ICAM-1), the co-stimulatory receptor ligand B7.2 (CD86), and MHC class II molecules. The expression of these activation markers was analyzed by flow cytometry. Freshly isolated FADD-/- B cells from the spleen and lymph nodes express basal levels of CD54 and CD86 on their surface, similar to that on FADD+/- B cells (Fig. 8A). Within 16 h after stimulation by LPS, these two proteins were up-regulated equivalently in both mutant and control B cells (Fig. 8A). Resting B cells acting as professional antigen presenting cells expressed high levels of MHC class II, which was further up-regulated during stimulation by LPS for 16 h in both FADD+/- and FADD-/- B cells (Fig. 8A). Therefore, a FADD deficiency did not appear to affect expression of activation markers induced by LPS stimulation.
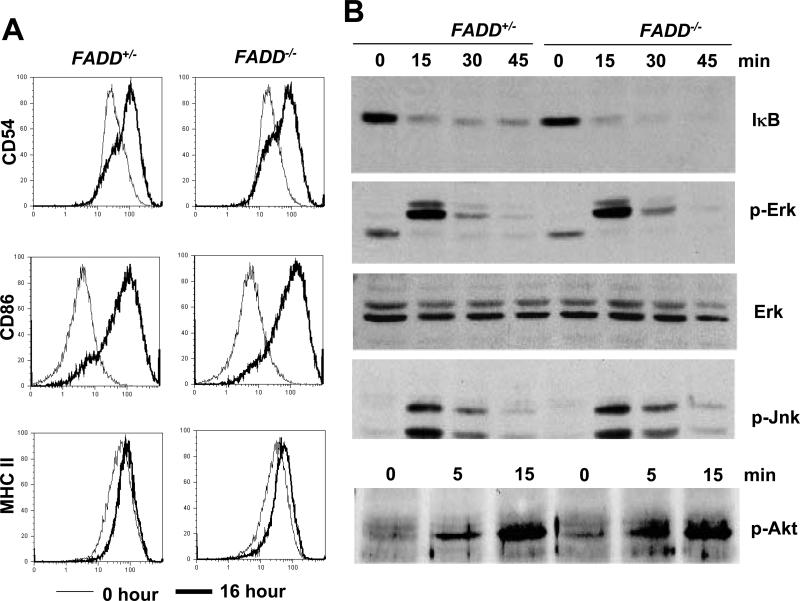
Analysis of activation marker upregulation and intracellular signaling in B cells. (A) Induction of CD54, CD86 and MHC class II was analyzed by staining with indicated antibodies at 0 (thin lines) and 16 h (thick lines) after LPS stimulation of sorted FADD-/- mutant and FADD+/- control B cells. (B) Total proteins from FADD+/- and FADD-/- B cells stimulated with LPS (10 μg/ml) for the indicated times were analyzed for NF-κB activation by detecting degradation of IκB. To analyze Erk activation, the same nitrocellulose membrane was re-probed with antibodies specific for phosphorylated (p)-Erk1/2, and probed with anti-Erk1/2 antibodies after stripping. The membrane was probed for the fourth time with anti-p-Jnk antibodies after the second stripping. A separate membrane with samples collected at the indicated times was probed with anti-p-Akt antibodies to detect Akt phopshorylation induced by LPS stimulation (10 μg/ml). FADD+/- B cells were used as control. Data shown are representative of at least three independent experiments.
Stimulation of TLRs induces activation of NF-κB and mitogen-activated protein kinases (MAPKs) such as extracellular signal-regulated kinases (Erks) and c-Jun N-terminal kinase (Jnk) (42). Upon signaling, the inhibitory subunit of NF-κB, IκB, is targeted for ubiquitination and degradation by the proteosome, thus releasing NF-κB for translocation to the nucleus (49). To analyze NF-κB activation, western blotting was used to detect IκB. As demonstrated in Fig. 8B, the IκB protein was present in unstimulated B cells (0 min) and was reduced at later time points (15, 30, 45 min), indicating degradation of IκB was initiated in both FADD+/- and FADD-/- B cells upon stimulation with LPS. Phosphorylation of IκB, as determined by western blot analyses using phospho-IκB-specific antibodies, was not affected in FADD-/- B cells stimulated with LPS (data not shown). To analyze activation of MAPKs, western blotting was used to detect phosphorylation of ERK1/2. In FADD+/- B cells, activation of Erk1/2 was evident, as indicated by the presence of the phosphorylated Erk1/2 within 15 min following LPS stimulation (Fig. 8B). Similar Erk1/2 activation kinetics were observed in FADD-/- B cells. Activation of Jnk was also analyzed by western blotting using phospho-specific antibodies, and no apparent difference between FADD+/- and FADD-/- B cells stimulated by LPS was detected (Fig. 8B). Phosphatidylinositol 3 kinase (PI-3K) can be activated by LPS, leading to the activation of the oncogenic serine/threonine kinase Akt, also known as protein kinase B (50, 51). As shown in Fig. 8B, Akt activation was readily achieved by LPS stimulation in both FADD+/- and FADD-/- B cells, as indicated by phosphorylation of Akt.
Discussion
The FADD protein was initially identified as an adaptor molecule required for cell death signaling induced by DD-containing receptors such as Fas, TNF-R1, and TRAIL-R. These death receptors play an important role in regulating the function of the immune system and possibly in tumor surveillance, but are dispensable during embryonic development and hematopoiesis. FADD has additional functions since its absence in germ cells results in early embryonic lethality. In this study, we analyzed the function of FADD in the B cell lineage using a conditional FADD mutant mouse model, and demonstrated that the deletion of FADD specifically in B cells at the pre-B stage had no major effect on B cell development (Fig. 2 and and3).3). A FADD deficiency inhibited Fas-induced apoptosis (Fig. 5), resulting in an increased number of splenic and lymph node B cells (Fig. 3). Interestingly, peritoneal B1 cells were reduced in these B cell-specific FADD-deficient mice (Fig. 4). Unlike FADD-/- T cells which are defective in antigen receptor-induced proliferation responses (26), FADD-/- B cells appear to proliferate normally in response to stimulation of the BCR (Fig. 6). In addition, proliferation of B cells induced by CD40 or TLR9 stimulation was not affected in the absence of FADD. Surprisingly however, FADD-/- B cells have a profound defect in proliferation induced by stimulation of TLR3 and TLR4. These results have thus revealed a novel function of FADD in the innate immune responses mediated by TLRs.
During the early stages of B lymphocyte development in the bone marrow, lymphoid progenitor cells give rise to fully committed B lymphocytes after a process of sequential recombination and assembly of Ig gene segments. In a previous study using viable mutant chimeras generated using FADD-/- ES cells and Rag-1-/- blastocytes, FADD was absent through embryonic and lymphoid development (22). These chimeras contained few T cells and undetectable levels of B cells in the periphery. In this study, an absence of FADD at the pre-B and subsequent stages still allowed the generation of immature and mature B cells in the bone marrow and periphery at levels similar to FADD-expressing control mice (Fig. 2, ,3,3, and and4).4). Therefore, it is likely that FADD plays a more important role in earlier progenitor cells before development reaches the pre-B and later stages. B1 cells represent a unique population of B lymphocytes and can be distinguished from other B cells by their surface phenotype (39, 40). They constitute a substantial fraction of B cells in the peritoneal cavity and express Mac-1 (CD11b/CD18 or complement receptor type 3). The B1 lineage is presumably derived from precursors in the fetal liver and would persist for the life of the animal by self-renewal in adults. Mutations in several genes in mice such as CD19, B cell linker protein, Bruton's tyrosine kinase, Vav, phospholipase Cγ2, the PI3K p85 subunit, and protein kinase C result in reduced B-1 cells (40). In this study, B cell-specific FADD-/- mice also contained reduced numbers of B1 cells, indicating that FADD may play a role in the development of B1 B cells.
It is interesting that similar to FADD, caspase8 and the caspase 8-like regulatory protein cFLIP are also essential for embryonic development (24, 25). T cell-specific caspase 8-/- mice have no major defect in thyomcyte development, but contain reduced numbers of peripheral T cells (30). B cell-specific caspase 8-/- mice have no major defect in bone marrow B cell development, but contain increased number of peripheral conventional B cells and reduced B1 B cells (52). These phenotypes are strikingly similar to those of lymphocyte-specific FADD-/- mice as described in previous and this studies (26). In the analysis of cFLIP function, deletion of the cFLIP gene in ES cells or lymphocytes resulted in phenotypes similar to those of FADD gene deletion in ES cells or lymphocytes. Whereas cFLIP-/-→ Rag-1-/- chimeras generated from cFLIP-/- ES cells have severe defects in T and B cell development (33), T cell-specific deletion of cFLIP has no obvious effect on thymocyte development, but inhibits peripheral T cell production (32). Additional Cre systems could be used in future studies in order to help determine the temporal requirement of FADD, caspase 8, and cFLIP during development of lymphoid progenitors.
The phenotype of increased B cells in the spleen and lymph nodes of B cell-specific FADD-/- mice is distinct from that of T cell-specific FADD-/- mice, which contain a reduced peripheral T cell pool (26). This phenotypic difference may be due to the differential roles of FADD in these two distinct cell lineages. Whereas FADD is essential for antigen receptor signaling in T cells, it is dispensable in BCR signaling (Fig. 6). Defective TCR signaling resulted in a reduced capacity for homeostatic expansion, a possible cause for T lymphopenia in T cell-specific FADD-/- mice (26). The increased peripheral B cell numbers in B cell-specific FADD-/- mice may be due in part to defective Fas-induced apoptosis (Fig. 5), which also regulates B cell homeostasis. Unexpectedly, a FADD deficiency in B cells did not appear to result in an elevated serum Ig concentration or ALPS, even in aged mice (Fig. 5), unlike that caused by the Fas deficiency in mice. This phenotype may not be surprising, given the results from a recent study showing that a B cell-specific Fas deficiency did not result in autoimmune diseases in mice (53). However, an lpr-like disease becomes obvious when Fas is deleted simultaneously in both lymphoid and non-lymphoid cells. Therefore, a lack of FADD in B cells along with other cell types may result in autoimmune pathology. It would be of interest to determine whether this is the case when additional Cre-systems are used to delete FADD in multiple cell types in adult mice.
The “pattern recognition” receptors, TLRs, play important roles in innate immune responses against various microbial pathogens (42, 54). TLRs recruit the shared cytoplasmic adaptor protein MyD88 via TIR-TIR interaction, leading to the activation of IRAKs. Later studies revealed a MyD88-independent pathway mediated by another TIR-containing adaptor, TRIF, particularly in TLR3 and TLR4 signaling (46). In this study, we showed that FADD deficiency abrogated proliferation of B cells stimulated by either dsRNA or LPS, but not by CpG-containing DNA, thus introducing FADD as a new component of TLR3 and TLR4-induced signaling pathways. Interestingly, the recently reported B cell-specific caspase 8-deficient mice also have a defect in B cell proliferative responses induced by TLR3 and TLR4 (52). TLRs are capable of inducing signal transduction pathways involving NF-κB and MAPKs such as Erk and Jnk. Inactivation of MyD88 still allows for the activation of these pathways in response to LPS, albeit with delayed kinetics (55). Disruption of TRIF has no obvious effect on the activation of MAPKs and NF-κB induced by LPS, but inhibits upregulation of CD54, CD86 and MHC class II molecules (46, 56). However, deletion of both MyD88 and TRIF completely inhibited LPS-induced activation of NF-kB and MAPKs (46). Previous reports suggested that FADD was able to activate the NF-κB and MAPK pathways (57, 58). Another early signal required for survival during B cell proliferation is the activation of PI-3K (50, 51). Stimulation of TLR4 by LPS can activate PI-3K in B cells, leading to the activation of the serine/threonine kinase Akt. A recent study has shown that the receptor interacting kinase protein RIP plays a role in TLR-4 signal-induced activation of Akt in B cells (59). In this study, LPS- and dsRNA-induced proliferative responses were defective in FADD-/- B cells in [3H] thymidine incorporation and cell division kinetics assays (Fig. 6 and and7).7). However, LPS-induced activation of NF-κB and MAPKs appeared to be unaffected in FADD-/- B cells (Fig. 8B). Furthermore, Akt activation in FADD-/- B cells appeared to be normal as following LPS stimulation (Fig 8B). LPS-induced upregulation of MHC class II proteins as well as of the activation markers CD54 and CD86, which are dependent on the TRIF pathway (46, 56), were not affected by a lack of FADD in B cells (Fig. 8B). In the recently reported B cell-specific caspase 8-deficient mice, B cells did not appear to have defects in activation marker upregulation or the activation of NF-κB and MAPKs (52). Therefore, it is likely that FADD and caspase 8 mediate additional pathways induced by TLR3 or TLR4 signaling.
Acknowledgement
The authors would like to thank Dr. Bice Perussia for critical reading of the manuscript; Dr. Arthur Buchberg, Dr. Fritz Melchers, and Dr. Kishore Alugupalli for discussion, Dr. Klaus Rajewsky for providing CD19-Cre mice, Dr. R. C. Rickert for providing the protocol for PCR typing of CD19-Cre mice, and Xiaohe Liu for technical help.
Footnotes
1This study is supported in part by a NIH grant (CA95454), a W. W. Smith Charitable Trust grant, and a CONCERN Foundation grant to J.Z. S.R. is supported by a NRSA training grant (T32-AI07492).
References
Full text links
Read article at publisher's site: https://doi.org/10.4049/jimmunol.176.11.6852
Read article for free, from open access legal sources, via Unpaywall:
http://www.jimmunol.org/content/176/11/6852.full.pdf
Citations & impact
Impact metrics
Citations of article over time
Alternative metrics
Smart citations by scite.ai
Explore citation contexts and check if this article has been
supported or disputed.
https://scite.ai/reports/10.4049/jimmunol.176.11.6852
Article citations
Caspase 8 deletion causes infection/inflammation-induced bone marrow failure and MDS-like disease in mice.
Cell Death Dis, 15(4):278, 18 Apr 2024
Cited by: 1 article | PMID: 38637559 | PMCID: PMC11026525
Apoptotic cell death in disease-Current understanding of the NCCD 2023.
Cell Death Differ, 30(5):1097-1154, 26 Apr 2023
Cited by: 93 articles | PMID: 37100955 | PMCID: PMC10130819
Review Free full text in Europe PMC
RIPK3 Suppresses the Progression of Spontaneous Intestinal Tumorigenesis.
Front Oncol, 11:664927, 30 Apr 2021
Cited by: 10 articles | PMID: 33996591 | PMCID: PMC8120274
Soluble TNF-like weak inducer of apoptosis (TWEAK) enhances poly(I:C)-induced RIPK1-mediated necroptosis.
Cell Death Dis, 9(11):1084, 22 Oct 2018
Cited by: 7 articles | PMID: 30349023 | PMCID: PMC6197222
Redundant and receptor-specific activities of TRADD, RIPK1 and FADD in death receptor signaling.
Cell Death Dis, 10(2):122, 11 Feb 2019
Cited by: 40 articles | PMID: 30741924 | PMCID: PMC6370826
Go to all (54) article citations
Other citations
Wikipedia
Data
Similar Articles
To arrive at the top five similar articles we use a word-weighted algorithm to compare words from the Title and Abstract of each citation.
Conditional Fas-associated death domain protein (FADD): GFP knockout mice reveal FADD is dispensable in thymic development but essential in peripheral T cell homeostasis.
J Immunol, 175(5):3033-3044, 01 Sep 2005
Cited by: 49 articles | PMID: 16116191 | PMCID: PMC3110086
Fas-mediated apoptosis and activation-induced T-cell proliferation are defective in mice lacking FADD/Mort1.
Nature, 392(6673):296-300, 01 Mar 1998
Cited by: 458 articles | PMID: 9521326
Rapid B cell apoptosis induced by antigen receptor ligation does not require Fas (CD95/APO-1), the adaptor protein FADD/MORT1 or CrmA-sensitive caspases but is defective in both MRL-+/+ and MRL-lpr/lpr mice.
Int Immunol, 12(4):517-526, 01 Apr 2000
Cited by: 27 articles | PMID: 10744653
The roles of FADD in extrinsic apoptosis and necroptosis.
BMB Rep, 45(9):496-508, 01 Sep 2012
Cited by: 74 articles | PMID: 23010170
Review
Funding
Funders who supported this work.
NCI NIH HHS (3)
Grant ID: R01 CA095454
Grant ID: R01 CA095454-05
Grant ID: CA95454
NIAID NIH HHS (2)
Grant ID: T32 AI007492
Grant ID: T32-AI07492