Abstract
Free full text

Loss of Angiotensin-Converting Enzyme-2 Leads to the Late Development of Angiotensin II-Dependent Glomerulosclerosis
Abstract
Angiotensin-converting enzyme-2 (ACE2), a membrane-bound carboxymonopeptidase highly expressed in the kidney, functions as a negative regulator of the renin-angiotensin system. Here we report early accumulation of fibrillar collagen in the glomerular mesangium of male ACE2 mutant (ACE2−/y) mice followed by development of glomerulosclerosis by 12 months of age whereas female ACE2 mutant (ACE2−/−) mice were relatively protected. Progressive kidney injury was associated with increased deposition of collagen I, collagen III and fibronectin in the glomeruli and increased urinary albumin excretion compared to age-matched control mice. These structural and functional changes in the glomeruli of male ACE2 mutant mice were prevented by treatment with the angiotensin II type-1 receptor antagonist irbesartan. Loss of ACE2 was associated with a marked increase in renal lipid peroxidation product formation and activation of mitogen-activated protein kinase and extracellular signal-regulated kinases 1 and 2 in glomeruli, events that are also prevented by angiotensin II type-1 receptor blockade. We conclude that deletion of the ACE2 gene leads to the development of angiotensin II-dependent glomerular injury in male mice. These findings have important implications for our understanding of ACE2, the renin-angiotensin system, and gender in renal injury, with ACE2 likely to be an important therapeutic target in kidney disease.
The renin-angiotensin system (RAS) plays a key role in renal development and injury and in cardiovascular diseases.1–3 Angiotensin 1–8 (ANG II) acting via the angiotensin type 1 receptor (AT1) mediates both hemodynamic and direct cellular effects that contribute to the progression of chronic kidney disease, including diabetic nephropathy.1,4–9 Classically, activation of RAS leads to the generation of ANG II in two stages: first, the protease renin generates angiotensin 1–10 (ANG I) from angiotensinogen, and then the dicarboxypeptidase angiotensin-converting enzyme (ACE) cleaves two amino acids from the C terminus of ANG I to yield ANG II. This pathway for the production of ANG II peptide from its precursor, angiotensinogen, has been challenged by the discovery and characterization of other enzymes that process angiotensin peptides.3,10,11 The recent discovery of a homologue of the ACE gene, ACE2, is one such example. ACE2 is a mono-carboxypeptidase that generates angiotensin 1–9 (ANG 1–9) from ANG I and angiotensin 1–7 (ANG 1–7) from ANG II with a catalytic efficiency that is 400-fold higher with ANG II as a substrate than with ANG I.10,12
The predicted sum effect of ACE2 on angiotensin peptide processing is to shift the balance of production away from ANG II toward ANG 1–7, which is a physiological antagonist of ANG II, at least in part by interacting with the G-protein-coupled receptor mas.13,14 ACE2 acts as an endogenous ACE inhibitor by altering the relative generation of ANG II and ANG 1–7 and an important determinant of injury mediated by activation of RAS.3 In addition to its peptidase action, ACE2 is also a functional receptor for SARS coronavirus.10,15 ACE2 is highly expressed in the heart and kidney. Deletion of the ACE2 gene leads to a cardiac phenotype that is characterized by impaired cardiac contractility and ventricular dilatation.3,16 Interestingly, this cardiac phenotype is associated with increased tissue levels of ANG I and ANG II and is rescued by deletion of the ACE gene. Renal ACE2 expression is decreased in hypertensive16 and diabetic17 rodent models. Deletion of the ACE2 gene is also associated with an increase in plasma and kidney ANG I and ANG II levels,16 but the critical role of ACE2 in renal disease remains unexplored. To assess the role of ACE2 in kidney disease, we investigated the impact of ACE2 deletion on kidney structure and function in male and female ACE2 mutant (ACE2−/−) mice, and we treated a third group of male ACE2 mutant (ACE2−/y) mice with an ANG II receptor antagonist to test the hypothesis that deletion of the ACE2 gene would be associated with the development of ANG II-dependent glomerular injury. Our data establish that loss of ACE2 is associated with the development of gender-specific and age-dependent glomerular injury in male ACE2 mutant mice and that the glomerular injury is mediated by ANG II.
Materials and Methods
Experimental Animals and Protocols
Male and female mice were generated as previously described16 and used in accordance with the standards of the Canadian Council on Animal Care. ACE2 mutant mice were treated with the specific AT1 blocker irbesartan (kindly provided by Dr. J. Froehlich from Bristol-Myers Squibb) in the drinking water to obtain a dose of 50 mg/kg/day. Treatment was initiated at 9 to 10 weeks of age and maintained until 1 year of age. Water intake was monitored twice weekly to ensure adequate dosing.
Histopathology and Electron Microscopy
Kidneys from wild-type littermate (ACE2+/y) and male (ACE2−/y) and female (ACE2−/−) mutant mice were dissected under anesthesia and immediately either snap-frozen or placed into 4% neutral buffered formalin for 24 hours. All formalin-fixed, paraffin-embedded kidneys were stained with periodic acid Schiff (PAS) reaction and Picric Sirius Red and examined by light microscopy. In addition, 10-μm-thick sections stained with Picric Sirius Red were examined by immunofluorescence microscopy. Glomerulosclerosis scores were assessed by A.M.H. and J.W.S. by assessing approximately 100 glomeruli in a PAS-stained renal cross-section. Each glomerulus was scored from 0 to 4 assessing a score of 0 or 1 depending on whether sclerosis was absent or present, respectively, in each glomerular quadrant. For electron microscopy, kidneys were fixed in buffered 1% glutaraldehyde-4% formaldehyde, postfixed in 1% osmium tetroxide, and embedded in Epon-araldite. Ultrathin sections were stained with uranyl acetate and lead citrate and examined with a transmission electron microscope (1200 EX-II; Jeol, Peabody, MA).
Biochemical and Blood Pressure Measurements
Blood samples were collected from anesthetized mice after administration of 0.1 ml of 1000 IU/ml heparin (1:1 with normal saline) intraperitoneally, and 200 μl of plasma was used for measurement of pH, lactate, Na+, K+, Cl−, ionized calcium (Ca2+), and glucose using a Stat Profile M7 Analyzer (Nova Biomedical Corp., Waltham, MA). Plasma osmolality was measured using a microosmometer (Model 3MO; Advanced Instruments, Norwood, MA) based on the freezing point depression method. Mice were fasted overnight for 15 hours while allowing free access to water. Blood glucose levels were measured using an Elite glucose meter (Lifescan, Toronto, ON, Canada) using blood samples obtained from the tail vein in conscious mice. Plasma and kidney ANG 1–7 levels were determined as previously described.13,16 For the measurement of tissue aldehydes, kidney samples (100 mg) were ground into a powder in liquid nitrogen and suspended in 0.5 ml of deionized filtered water with [ethylenebis (oxyethylenenitrilo)]tetraacetic acid (EGTA, 400 μmol/L; a Ca2+ and iron chelator), butylated hydroxytoluene (20 μmol/L; a lipid peroxidation blocker), and deferoxamine (20 μmol/L; an iron chelator). Samples were analyzed by capillary-column gas chromatography-negative ion chemical ionization mass spectrometry as previously described.18 Blood pressure measurements were made in mice anesthetized with isoflurane/oxygen (1%/99%). The right common carotid was cannulated using a 1.4 French catheter (Millar Inc., Houston, TX), which was advanced into the proximal aorta for blood pressure measurements. Pressure recordings were filtered at 200 Hz and sampled at 2 kHz (Acq 3.73; BioPac Systems Inc., Goleta, CA).
Immunohistochemistry
Formalin-fixed, paraffin-embedded sections were used for all immunohistochemical analysis from mouse kidneys. Heat-induced antigen retrieval was used for all antibodies. Endogenous peroxidase activity was prevented by pretreating all sections with 3% hydrogen peroxide. The primary antibodies and dilutions used in this study are as follows: anti-collagen I (1:20; Cedarlane, Hornby, ON), anti-collagen III (1:50; Cedarlane), anti-fibronectin (1:1000; BD Transduction, San Diego, CA), anti-α-smooth muscle actin (1:200, Dako, Carpinteria, CA), anti-phospho ERK1/2 (1:800, Cell Signaling Technology, Beverly, MA), and anti-AT1 receptor (1:1000, Cedarlane). With the exception of phospho-ERK1/2 staining, all immunohistochemistry was analyzed by light microscopy using Image Pro Plus (Media Cybernetics, Crofton, MA) computer image analysis software to analyze brown staining pixel density. Glomerular cells staining positively with anti-p-ERK1/2 antibody were manually counted in ~100 glomeruli per animal. Negative controls with no primary antibody added were performed for all immunohistochemical staining.
Glomerular Morphometry
Glomerular basement membrane thickness was measured by calculating the harmonic mean of 50 orthogonal intercepts of the lamina densa in four glomeruli per mouse in ultrathin tissue sections stained with uranyl acetate and lead citrate and examined with a Jeol 1200 EX-II transmission electron microscope (n = 4 in each group). The glomerular tuft area was measured using Image Pro Plus 5.0 (Media Cybernetics) image analysis software. Seventy-five consecutive glomeruli in a single 2-μm PAS mouse kidney section from each mouse were measured to derive a mean glomerular tuft area (n = 6 in each group). Each glomerulus was digitally photographed, and the glomerular tuft circumference was traced. The software subsequently calculated the area (square microns) of the outlined glomerular tuft.
Urine Protein Electrophoresis
Urine protein detection was performed using the sodium dodecyl sulfate-polyacrylamide gel electrophoresis technique. Urine samples (25 μl) were run on an 8% sodium dodecyl sulfate-polyacrylamide gel electrophoresis at 110 V for 80 minutes. The gel was then stained with low toxicity staining solution (0.1% Coomassie Blue, 10% acetic acid, 40% ethanol) for 2 hours and destained (4% ethanol and 1% acetic acid) for 3 hours at room temperature.
Real-Time RT-PCR
Kidney samples from mice were snap frozen in liquid nitrogen and later dissected into the cortex, medulla, and papillae using an RNA-stabilizing solution (RNAlater; Ambion Inc., Austin TX) at −10°C under microscopic visualization. Renal cortical RNA expression levels for the genes reported in this study were quantified by real-time TaqMan PCR using a sequence detection system (Prism 7700; Applied Biosystems Inc., Foster City, CA) as described previously.19 Briefly, 1 μg of total RNA was reverse-transcribed using random hexamers. TaqMan reactions were performed in 96-well plates using 0.5% cDNA, 12.5 μl of 2× TaqMan Universal PCR Mastermix, 100 μmol/L probe, and 200 μmol/L of each primer and water to a final volume of 25 μl. 18S rRNA was used as an endogenous control, and primers and probe are indicated in Table 1.
Table 1
TaqMan Primer and Probe Sequences for TaqMan Real-Time RT-PCR Analysis
AT1 | Forward | 5′-CCATTGTCCACCCGATGAAG-3′ |
Reverse | 5′-TGCAGGTGACTTTGGCCAC-3′ | |
Probe | 5′-FAM-CTCGCCTCCGCCGCACGA-TAMRA-3′ | |
AT2 | Forward | 5′-CAGCAGCCGTCCTTTTGATAA-3′ |
Reverse | 5′-TTATCTGATGGTTTGTGTGAGCAA-3′ | |
Probe | 5′-FAM-CAACTGGCACCAATGAGTCCGCCT-TAMRA-3′ | |
ACE | Forward | 5′-TGAGAAAAGCACGGAGGTATCC-3′ |
Reverse | 5′-FAM-ACCCTGAAATATGGCACCCGGGC-TAMRA-3′ | |
Probe | 5′-AGAGTTTTGAAAGTTGCTCACATCA-3′ |
Results
Development of Glomerulosclerosis in ACE2 Mutant Mice
The kidneys of young (3-month-old) ACE2 mutant (ACE2−/y) mice showed no gross abnormalities and normal architecture of the cortex and medulla, comparable to littermate and age-matched wild-type (ACE2+/y) mice (Figure 1a). Morphologically, renal development appeared normal with normal renal arteries, veins, ureters, and normal kidney/body weights (8.14 ± 0.21 vs. 8.21 ± 0.17 mg/g; n = 8; P = 0.56). Glomeruli appeared developmentally normal and showed no glomerulosclerosis (Figure 1b). Consistent with the paucity of renal morphological abnormalities, littermate ACE2−/y and ACE2+/y mice had normal renal function, electrolytes, and plasma glucose values (Table 2). However, electron microscopy of 3-month-old ACE2−/y mice showed evidence of mesangial injury with small foci of fibrillar collagen deposition suggestive of an early disease process (Figure 1c). Because the progression of heart disease in ACE2 mutant mice is age-dependent,16 we decided to study older animals.
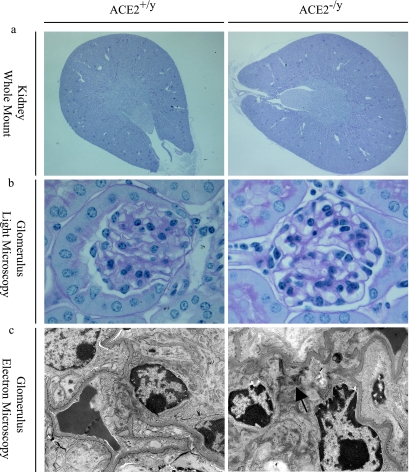
Normal architecture in young (3-month-old) ACE2 mutant mice with early ultrastructural changes. a: Whole mount of ACE2−/y mouse kidneys showing normal architecture (PAS, original magnification, ×16). b: ACE2−/y glomeruli are normal by light microscopy, comparable to ACE2+/y controls (PAS, original magnification, ×600). c: Electron microscopy shows early fibrillar collagen deposition (arrow) in the ACE2−/y mice but not in wild-type controls (original magnification, ×4800).
Table 2
Plasma Biochemistry in 3-Month and 12-Month-Old Mice
Age (months) | Na+ (mmol/L) | K+ (mmol/L) | Cl− (mmol/L) | Ca2+ (mmol/L) | |
---|---|---|---|---|---|
ACE2+/y (n = 7) | 3 | 140.8 ± 1.8 | 5.04 ± 0.14 | 110 ± 1.2 | 1.11 ± 0.04 |
ACE2−/y (n = 7) | 3 | 143 ± 1.7 | 5.48 ± 0.26 | 112.8 ± 1.2 | 1.14 ± 0.06 |
ACE2+/y (n = 7) | 12 | 140.7 ± 2.1 | 5.13 ± 0.21 | 108 ± 1.4 | 1.15 ± 0.06 |
ACE2−/y (n = 7) | 12 | 142.1 ± 2.3 | 5.3 ± 0.16 | 111 ± 2.2 | 1.18 ± 0.07 |
(table continues)
Table 2A
pH | Lactate (mmol/L) | Urea (mmol/L) | Cr (mmol/L) | Glucose (mmol/L) |
---|---|---|---|---|
7.49 ± 0.02 | 5.26 ± 0.62 | 8.15 ± 1.2 | 85 ± 9.2 | 8.54 ± 1.41 |
7.46 ± 0.024 | 5.67 ± 0.65 | 8.41 ± 1.31 | 81 ± 10.4 | 8.73 ± 1.35 |
7.47 ± 0.021 | 5.11 ± 0.68 | 8.87 ± 1.28 | 89 ± 9.3 | 8.15 ± 1.32 |
7.51 ± 0.025 | 5.35 ± 0.71 | 9.03 ± 1.49 | 93 ± 8.7 | 8.23 ± 1.46 |
Values are mean ± SE; Cr = creatinine.
In contrast to 3-month-old mice, 1-year-old male ACE2 mutant mice showed significant acquired glomerular abnormalities (Figure 2). The kidneys appeared grossly normal and comparable to those of age-matched wild-type mice with intact cortical, medullary, and hilar architecture (Figure 2a) and normal kidney/body weight ratio (6.56 ± 0.15 vs. 6.44 ± 0.22 mg/g; n = 8; P = 0.39). Light microscopic examination revealed diffuse glomerulosclerosis (Figure 2b). In 1-year-old female ACE2−/− mice (n = 8), glomeruli were unremarkable with no evidence of glomerulosclerosis (Figure 2b, far right panel). Semiquantitative assessment of four quadrant glomerulosclerosis by two of the authors (A.M.H. and J.W.S.) blinded to the mouse genotype, showed a significantly increased glomerulosclerosis score in 1-year-old male ACE2 mutant mice (P < 0.01; Figure 2c). Values for glomerular tuft areas were similar in male ACE2−/y mice and male ACE2+/y mice (5290 ± 170 vs. 4931 ± 153 μm2, P > 0.05). Measures of glomerular basement membrane thickness were also similar in male ACE2−/y mice and male ACE2+/y mice (171 ± 23 vs. 163 ± 19 nm, P > 0.05).

Glomerulosclerosis in old (1-year) ACE2 mutant mice. a: Whole mount of ACE2+/y, ACE2−/y, and ACE2−/− (female mutant mice) mouse kidneys show normal architecture (PAS, original magnification, ×16). b: ACE2−/y, but not ACE2+/yor ACE2−/−, glomeruli show glomerulosclerosis, segmental scarring, and hyalinosis (PAS, original magnification, ×600). c: Glomerulosclerosis scores of ACE2+/y, ACE2−/y, and ACE2−/− mice (*P < 0.01 vs. ACE2+/y and ACE2−/−).
There was a range of glomerular abnormalities by light microscopy involving the mesangium, capillary loops, and glomerular hila (Figure 3a). There was widespread segmental capillary loop hyalinosis and foci of mesangial expansion (Figure 3a, first panel) with capillary loop microaneurysms (Figure 3a, second panel), suggestive of a loss of glomerular basement membrane anchoring points to the mesangium due to mesangiolysis. Focal glomerulosclerosis was also present in the form of segmental obliteration of capillary loops by extracellular matrix (Figure 3a, third panel). Several glomerular hila showed hyaline arteriolosclerosis not present in extraglomerular arterioles (Figure 3a, fourth panel).
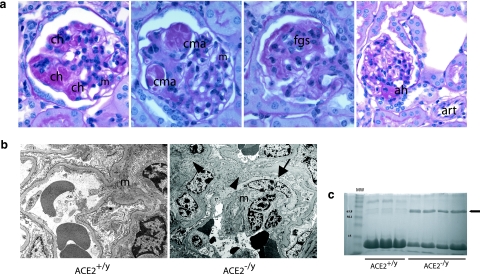
a: Survey of glomerular pathological changes in 1-year-old male ACE2 mutant mice. The mesangium (m) shows expansion and foci of mesangial hypercellularity. There are numerous foci of glomerular capillary hyalinosis (ch) and segmental capillary microaneurysm (cma) formation. Focal segmental glomerulosclerosis (fgs) is evident in the form of segmental scarring with adhesions. Evidence of arteriolar hyalinosis (ah) is present only at glomerular hila, with sparing of extraglomerular arterioles (art) (PAS, original magnifications, ×600 and ×400 last panel). b: Electron microscopy shows mesangial cell transition to a smooth muscle cell phenotype (arrow) and increased fibrillar collagen deposition (arrowheads) in an expanded mesangium (m) (original magnification, ×4800). c: Urine protein electrophoresis showing that glomerulosclerosis was associated with the development of albuminuria (arrow) in 1-year ACE2−/y but not wild-type ACE2+/y mice. Lane 1 shows molecular weight (MW) markers.
Electron microscopy showed widespread mesangial expansion due to an increase in extracellular matrix and mesangial hypercellularity (Figure 3b). Ultrastructural evidence of mesangial injury included the development of a fusiform appearance of mesangial cells, which contained striated microfilaments and resembled a smooth-muscle cell phenotype.20,21 There was also evidence of widespread mesangial fibrillar collagen deposition. Glomerular basement membranes had normal thickness, and there were no immune complex deposits. To determine whether there were alterations in renal function accompanying the histological abnormalities in 12-month-old ACE2 −/y mice, urine protein electrophoresis and serum biochemical analyses were performed. Albumin was detected in urine samples from ACE2−/y mice (n = 4) but not in urine from ACE2+/y mice (n = 3, Figure 3c). Serum biochemistry in 12-month-old ACE2+/y (n = 7) and ACE2−/y (n = 7) mice revealed normal electrolytes, urea, creatinine, and random plasma glucose, indicating the absence of renal insufficiency (Table 2).
To further characterize the mesangial injury, immunohistochemical studies were performed on 1-year-old ACE2−/y (n = 4) and ACE2+/y (n = 4) mouse kidneys (Figure 4). Immunohistochemical staining was quantified by computerized image analysis. There was a fivefold increase in collagen I staining (Figure 4a) and a sixfold increase in collagen III staining (Figure 4b) in the glomeruli of ACE2 mutant mice. In addition, there was a threefold increase in glomerular staining for fibronectin (Figure 4c) and a threefold increase for α-smooth muscle actin (Figure 4d) in ACE2−/y mice compared with ACE2+/y mice. To exclude secondary causes of glomerular changes, we investigated whether these mice were diabetic or hypertensive. Fasting blood glucose was similar in age-matched littermate ACE2−/y (n = 5) and ACE2+/y-mice (n = 5) (5.53 ± 0.44 vs. 6.11 ± 0.28 mmol/L; P = 0.34). Consistent with our previous findings,16 mean systemic blood pressure was lower in 1-year-old ACE2−/y mice (n = 6) compared with ACE2+/y mice (n = 6) (78.9 ± 2.9 vs. 102.6 ± 2.5 mmHg, P < 0.01), whereas heart rate was similar in both groups of mice (508 ± 8 vs. 520 ± 11 beats per minute, P = 0.41). These observations established that the primary glomerular changes in male ACE2 mutant mice are not related to systemic elevation in blood pressure or blood glucose.
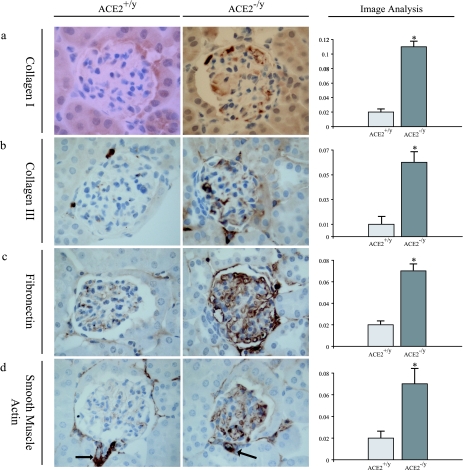
Glomerular immunohistochemical profile of 1-year-old ACE2+/y and ACE2−/y mice. Far right panel shows computer image analysis scores of glomerular immunostaining. a: Collagen I. b: Collagen III. c: Fibronectin. d: α-Smooth muscle actin. As expected arterioles exhibit positive α-smooth muscle actin staining (arrows) in both ACE2+/yand ACE2−/y mice. Immunoperoxidase, original magnification, ×600 (a–d). *P < 0.05 versus ACE2+/y.
AT1 Receptor Blockade Prevents Glomerulosclerosis
These glomerular changes in the setting of chronic elevation of plasma and renal ANG II levels in the ACE2 mutant mice suggest that ACE-generated ANG II plays a key pathophysiological role.16 Therefore, we examined the kidneys of the ACE−/−/ACE2−/y double mutant mice. In contrast to the cardiac phenotype,16 the kidneys showed markedly abnormal features (Figure 5). The cortices and medulla of these double mutant mice were thinned, and the renal calyces were cystically dilated with widespread interstitial fibrosis, accompanied by interstitial lymphocytic infiltrates and abnormally appearing glomeruli with small and incomplete capillary loops within a loose extracellular matrix. These profound abnormalities precluded the use of this model to test whether the renal phenotype in ACE2 mutant mice was ANG II-dependent. Instead, we treated ACE2−/y mice with the AT1 blocker (ARB) irbesartan for 10 months. The mean arterial blood pressure was 101.4 ± 4.6 mmHg in the ACE2+/y mice, whereas mean arterial blood pressure values were lower in both the ACE2−/y mice and ACE2−/y mice treated with irbesartan (83.8 ± 6.2 and 77.2 ± 5.6 mmHg, respectively, P < 0.05 vs. the ACE2+/y mice). The 1-year-old ARB-treated ACE2−/y mice showed no gross renal abnormalities and showed normal cortical, medullary, and vascular architecture (Figure 6, a–c). Glomeruli in the treated mice appeared normal, comparable to 1-year-old placebo-treated ACE2+/y wild-type mice (Figure 6a) and markedly different from placebo-treated ACE2−/y mice which showed diffuse glomerulosclerosis (Figure 6b). AT1 blockade prevented glomerulosclerosis, hyalinosis, capillary microaneurysms, mesangial expansion, or glomerular arteriolar hyalinosis (Figure 6c). The semiquantitative glomerulosclerosis score was normalized in the ARB-treated ACE2 mutant mice (Figure 6d), and there was complete resolution of the albuminuria (Figure 6e).
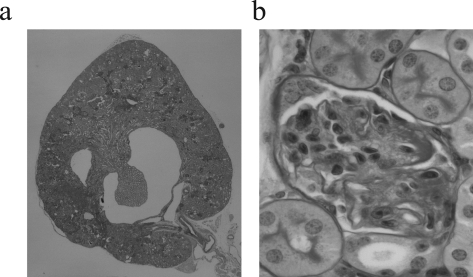
Kidneys of ACE−/−/ACE2−/y double mutant mice (n = 4). a: Whole mount of ACE−/−/ACE2−/y mouse kidneys show abnormal renal architecture. There is cortical and medullary thinning, cystic dilatation of the renal calyx, and an abnormal papilla, possibly due to hydronephrosis. b: Glomeruli of ACE−/−/ACE2−/y mouse are abnormal. Capillary loops are incomplete, small, and hyalinotic. The mesangium shows abnormal architecture characterized by a loose-appearing matrix. PAS stain, original magnifications, ×16 (a) and ×600 (b).

Treatment with the ARB irbesartan prevents development of glomerulosclerosis in ACE2 mutant mice. a: Whole mount of ACE2+/y mouse kidney and glomerulus. b: ACE2−/y mouse kidney, treated with placebo, showing normal gross architecture. The glomerulus shows segmental sclerosis, capillary hyalinosis, and capillary microaneurysm formation. c: ARB-treated ACE2−/y mice (ACE2−/y ARB) show normal gross architectural features and glomeruli, comparable to ACE2+/y mice. d: Glomerulosclerosis scores: *P < 0.05 vs. ACE2−/y ARB. PAS stain, original magnifications, ×16 (whole mounts), ×600 (glomeruli) (a–c). e: Urine protein electrophoresis of ACE2−/y ARB mice. There is no detectable albumin in the urine of the ARB-treated ACE2−/y mice (lanes 1 to 5). Lane 6 shows molecular weight (MW) markers.
ANG II-dependent glomerular injury is mediated by oxidative stress and involves activation of several downstream signaling domains, including the mitogen-activated protein kinase pathway.22,23 To investigate whether reactive oxygen species (ROS) could be effectors of glomerular injury, analyses of lipid peroxidation products, hexanal (Figure 7a), malondialdehyde (Figure 7b), and hydroxynonenal (Figure 7c), were performed. Male ACE2 mutant mice showed significantly higher levels of aldehydes in their kidneys compared to age-matched wild-type controls (n = 6). These levels were normalized in the ARB-treated ACE2−/y mice (n = 8) to levels comparable to wild-type control mice. The measures of lipid peroxidation products in the kidneys of female ACE2+/+ and ACE2−/− mice were also comparable to wild-type control male mice (Figure 7, a–c). Immunohistochemical staining for the mitogen-activated protein kinase, phospho-extracellular signal-regulated kinase (ERK)1/2, showed a normal distribution of phospho-ERK1/2-positive nuclei in the epithelial cells of distal tubules and collecting ducts (data not shown). However, glomeruli of ACE2−/y mice showed a significantly higher number of phospho-ERK1/2-positive nuclei compared to wild-type mice, which was prevented by treatment with ARB (Figure 8a). The number of phospho-ERK1/2-positive nuclei in the glomeruli of female ACE2−/− mice was similar to the wild-type male mice (Figure 8, a and b). Quantitative manual scoring of phospho-ERK1/2-positive nuclei per glomerulus confirmed these findings in all three groups (Figure 8b). Thus, loss of ACE2 in male mice leads to an ANG II-dependent increase in oxidative stress and activation of mitogen-activated protein kinase in the kidneys of male ACE2 mutant mice.
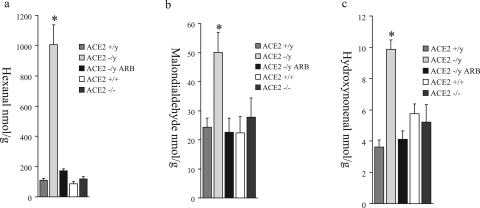
Kidney levels of the lipid peroxidation products, hexanal (a), malondialdehyde (b), and hydroxynonenal (c) in male ACE2+/y, male ACE2−/y, ARB-treated male ACE2−/y, female ACE2+/+, and female ACE2−/− mice. *P < 0.05 versus male ACE2+/y, ARB-treated male ACE2−/y, female ACE2+/+, and female ACE2−/− mice.
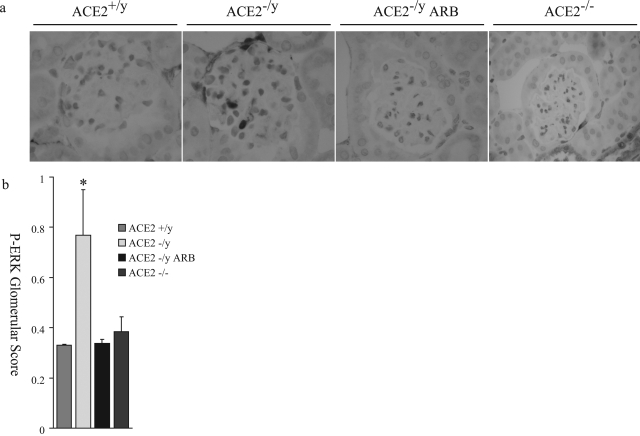
Phospho-ERK1/2 immunohistochemistry of mouse glomeruli. a: Increased nuclear staining of phospho-ERK1/2 is observed in the male ACE2−/y mouse glomerulus, compared to male ACE2+/y, ARB-treated male ACE2−/y, and female ACE2−/− mouse glomeruli (magnification, ×600). b: Quantitative score of phospho-ERK1/2-positive nuclei per glomerular cross-section in the four groups of mice. *P < 0.01 versus ACE2+/y, ARB-treated ACE2−/y, and female ACE2−/− mice.
We hypothesized that, in response to elevated renal and systemic ANG II levels in ACE2 mutant mice, there was compensatory down-regulation of ANG II receptors and ACE expression. We performed TaqMan real-time RT-PCR of 1-year-old ACE2−/y (n = 8), ACE2+/y (n = 8), and ARB-treated ACE2−/y (n = 8) mouse renal cortex mRNA for AT1, the ANG II type-2 receptor (AT2), and ACE. There was decreased expression of kidney AT1 (Figure 9a) in the ACE2−/y mice compared to the ACE2+/y mice but no change in kidney AT2 expression (Figure 9b). AT1 expression was normalized by ARB treatment (Figure 9a). Kidney ACE mRNA (Figure 9c) was also decreased in ACE2−/y mice compared to ACE2+/y wild-type controls and normalized by ARB treatment. AT1 receptor protein expression by immunohistochemical analysis was also reduced in ACE2−/y compared to ACE2+/y mice (Figure 9, d and e).
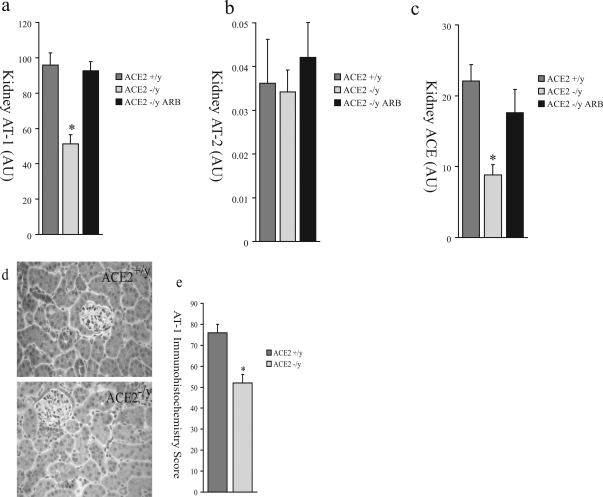
Renal expression of components of the RAS in ACE2+/y, ACE2−/y, and ARB-treated ACE2−/y mice by real-time RT-PCR for AT1 (a), AT2 (b), and ACE (c). d: Immunohistochemical study of AT1 receptor protein expression in the renal cortex of male ACE2+/y and male ACE2−/y mice. e: Quantitative scoring of AT1 receptor expression (P < 0.01 vs. ACE2+/y mice).
Discussion
The RAS and the generation of angiotensin peptides are critically important for the maintenance of renal and cardiovascular structure and function and play a key role in the pathophysiology of kidney and cardiovascular diseases.1–3,9,24 In clinical studies, blockade of RAS by ACE inhibition or angiotensin receptor blockade limits the progression of chronic kidney disease, especially when the kidney disease is associated with proteinuria.1,4–9 In addition to the glomerular injury, interstitial fibrosis is a main determinant of the progression of renal damage in patients with nephropathies.25–27 ANG II interacts with a cognate receptor on cell surfaces to influence functions as diverse as vascular contractility, hemodynamic forces, cellular transport, hypertrophy, growth factor synthesis, generation of ROS, and gene expression. ACE2 is a membrane-bound monocarboxypeptidase that converts ANG I to ANG 1–9 and ANG II to ANG 1–7, thereby functioning effectively as an endogenous ACE inhibitor,10,12 as shown in the setting of cardiovascular disease.3,16 We have reported that plasma and kidney ANG I and ANG II levels are increased in ACE2−/y compared to ACE2+/y mice, and this observation provided the rationale for the current studies.16
Our first major observation was that loss of ACE2 in male mice leads to the age-dependent development of glomerular mesangial expansion with increased deposition of fibrillar collagens I/III and the extracellular matrix protein fibronectin. The emergence of a contractile-like, smooth muscle cell phenotype in the glomerular mesangial cells was associated with increased expression of α-smooth muscle actin. Glomerular vascular injury was limited to the microvasculature of the glomerular capillary tuft and the preglomerular afferent arteriole with arteriolar hyalinosis and microaneurysm formation. The development of glomerulosclerosis was associated with impairment in the glomerular filtration barrier such that urinary albumin was detected in the male ACE2 mutant mice, but there was no biochemical evidence of azotemia, and creatinine values were similar in the ACE+/y mice and ACE−/y mice. These structural and functional glomerular abnormalities were not accompanied by diabetes mellitus or hypertension.
Our finding of late development of glomerulosclerosis in ACE2-null male mice differs from other studies of mice with gene deletions in RAS. For example, kidneys of 3-week-old angiotensinogen knockout mice (Agt−/−) had substantially smaller glomerular tufts, tubular dilatation, arterial wall thickening, atrophic papillae, hyperplasia of the interlobular arteries and afferent arterioles, interstitial fibrosis, renal lesions, and mesangial expansion.28 When human renin (R) and angiotensinogen (A) transgenes were inserted into Agt−/− mice, R−A−Agt−/− mice presented with the same kidney injury as Agt−/− mice by 3 to 5 months, whereas R+A+Agt−/− mice possessed a rescued phenotype, except for rare mild focal regions of fibrosis.29 Krege and coworkers30 reported that the kidneys were abnormal in ACE−/− mice. Intrarenal arteries exhibited a marked hypercellularity and increased wall thickness, and these vascular abnormalities were associated with cortical atrophy and interstitial inflammation, changes similar to the findings in Agt−/− mice.30 Mice lacking the AT1A receptor (Agtr1a−/−) and AT1B receptor (Agtr1b−/−) (ie, double nullizygotes (Agtr1a−/− and Agtr1b−/−)) had multiple structural and functional renal abnormalities: atrophied papillae, severely thickened arterial walls, areas of cortical interstitial inflammation, fibrosis, tubular atrophy, and medial hyperplasia of the interlobular arteries and afferent arterioles.31,32 Increasing copies of the ACE gene in 6-month-old mice were positively correlated with urinary albumin excretion but not with any structural injury.33 To determine the mechanism responsible for the development of glomerulosclerosis, we first studied double mutant (ACE−/−/ACE2−/y) mice. We have previously reported that the postnatal cardiac phenotype is rescued in the double mutant (ACE−/−/ACE2−/y) mice and appears similar to the ACE mutant mice.16 In the current study, we found that the kidneys of the ACE−/−/ACE2−/y mice were markedly abnormal with findings that were similar to those described in ACE−/− mice30,34 and angiotensinogen28 mutant mice. These findings clearly support a critical role for ANG II in the maintenance of normal kidney structure.16,28,30,31
In contrast to our observations in male mice, female ACE2 mutant mice did not show glomerular injury. Gender is an important determinant of kidney disease progression, but the one or more mechanisms responsible for the protective effect of female gender on kidney disease have not been fully elucidated.35 Our finding is consistent with the potent ability of estrogens to antagonize the pathological effects of ANG II in animal models36,37 and patients.35,38,39 The biological effect of estrogen on kidney has also been the focus of a number of investigations.37,40–42 The natural history of a number of experimental animal models of kidney disease is also influenced by gender or treatment with exogenous estrogen.37,42 Cellular mechanisms that may play a role in gender protection include inhibition of transforming growth factor-β1-induced collagen gene expression40 and mechanical strain-induced mesangial cell signaling.41 Although we did not define the mechanism responsible for the protective effect of female gender in the current study, we did find that there was no increase in lipid peroxidation products in the kidneys of ACE2−/− female compared to ACE2+/+ female mice. In this regard, Baumer and coworkers43 reported that treatment with a selective estrogen receptor modulator can reduce ANG II-induced oxidative stress in vascular smooth muscle cells. Estrogen treatment also reduces oxidative stress in hepatic stellate cells due to suppression of NADPH oxidase activity.44 Accordingly, an estrogen-mediated effect on oxidative stress may be responsible for the gender effect that we observed, but further studies will be necessary to determine whether this is the case.
In addition, we found that there was no increase in the number of phospho-ERK-positive cells in the glomeruli of ACE2−/− female compared to ACE2+/y male mice. This may have been due to the attenuation in oxidative stress in the kidneys of the ACE2−/− female mice. However, estrogen can also attenuate ANG II-induced activation of ERK in vascular smooth muscle cells by activating intracellular phosphatases like mitogen-activated protein kinase phosphatase-1.45 Moreover, we have reported that estrogen can attenuate mechanical strain-induced ERK activation in glomerular mesangial cells.41 Taken together, these studies suggest that both the direct (cellular) and indirect (hemodynamic) effects of ANG II on activation of ERK in the glomerulus can be attenuated by estrogen perhaps accounting, at least in part, for the gender protection that we observed in the ACE2−/− female mice. However, the discrepancy in renal injury between male and female ACE2 mutant mice may not be due to an interaction between angiotensin II and estrogen. Further studies will be necessary to more fully define the mechanism(s) responsible for the gender protection that we observed.
We hypothesize that the link between deletion of ACE2 gene and renal injury is the chronic exposure to increased circulating and tissue ANG II levels.16 In accordance with this hypothesis, Lautrette and co-workers46 have recently reported that ANG II infusion leads to glomerular injury (and tubulointerstitial injury) in mice. Rats are much more sensitive to the effects of ANG II infusion than mice, and kidney injury is evident within 14 days.21 Moreover, ANG II type 1 receptor blocker prevented glomerular injury and development of glomerulosclerosis and albuminuria in ACE2 male mutant mice. ANG II activates NADPH oxidase in vascular smooth muscle cells and mesangial cells and the subsequent generation of ROS, such as superoxide (O2).23,47,48 ANG II and ROS play an important role in cellular signaling and the activation of gene expression, including genes of extracellular matrix proteins like collagens and fibronectin via transforming growth factor-β- and NFκB-mediated gene expression.49–51 ANG II-induced activation of the mitogen-activated protein kinase-signaling molecule ERK1/2 is mediated, at least in part, by the trans-activation of the epidermal growth factor receptor in a ROS-dependent manner.22 Accordingly, aldehydes, which are lipid peroxidation products and sensitive indicators of oxidative stress,18,52 were increased in the kidneys from ACE2 mutant mice consistent with an increase in the generation of ROS. Increased oxidative injury was associated with activation of glomerular ERK1/2 leading to accumulation of extracellular matrix in the glomerular capillary tuft. Increase in glomerular α-smooth muscle actin occurs in response to cellular injury to the glomeruli20 and can be recapitulated by infusion of ANG II.21 The critical role of ANG II in mediating these changes was confirmed by complete blockade of ROS formation, phospho-ERK1/2 nuclear staining and α-smooth muscle actin formation by treatment with the AT1 blocker irbesartan. Taken together, this data support the hypothesis that ANG II-induced generation of ROS is responsible for the glomerular injury in male ACE2 mutant mice.
Although the glomerular injury that developed in the male ACE2 mutant mice was ANG II-dependent, the mechanisms responsible for the increase in ANG II activity may be multifactorial. Similarly, we cannot discount the role of other ACE2 reaction products in the pathogenesis of renal injury. Increased circulating and kidney ANG II levels are evident by 3 months of age in ACE2 mutant mice,16 but by 3 months of age, the mice also exhibit contractile dysfunction. It is possible that the cardiac dysfunction was associated with systemic neurohumoral activation, including activation of the RAS. However, circulating ANG I levels in ACE2 mutant are not increased, suggesting a lack of secondary activation of the RAS.16 Interestingly, we did not observe glomerulosclerosis in female ACE2 mutant mice despite the fact that female mice developed contractile dysfunction,16 suggesting that the gender protection of the kidney occurred despite cardiac dysfunction and supporting, at least in part, a role for altered ANG peptide processing in the development of glomerulosclerosis in the male mice. In addition, normal plasma creatinine in the ACE2 mutant mice (see Table 2) indicates the absence of prerenal azotemia despite the development of glomerular injury.
The role of ACE2 in experimental and clinical kidney disease has not been fully elucidated, and the current data are conflicting. Renal ACE2 expression is decreased in hypertensive rat models.16 In experimental diabetes, Tikellis and coworkers17 have reported that ACE2 protein expression is localized mainly in kidney tubules and that the level of expression is decreased in the renal tubules of rats with streptozotocin-induced diabetes mellitus (measured at 24 weeks). In contrast, Ye and co-workers53 found that ACE2 expression was increased in the renal tubules of 8-week-old db/db mice compared to control db/m mice, and the authors speculated that the increase was an early protective response. Lely and coworkers54 studied ACE2 immunostaining in renal biopsies from 58 patients with primary and secondary renal diseases (including IgA glomerulopathy, minimal change nephropathy, diabetic nephropathy, focal glomerulosclerosis, and membranous glomerulopathy) and 18 normal renal biopsy samples. In normal kidneys, ACE2 was present in tubular cells, glomerular epithelial cells, vascular smooth muscle cells, and the endothelial cells of interlobular arteries. All of the primary and secondary renal diseases were associated with the de novo expression of ACE2 in glomerular and peritubular capillary endothelial cells, but further studies will be necessary to better define the possible protective role of ACE2 in human renal disease.
In summary, we report that deletion of the ACE2 gene in male mice is associated with a renal phenotype characterized by early fibrillar collagen deposition in the glomeruli and the eventual development of glomerulosclerosis. The glomerular injury is ANG II-dependent, whereas the renal vasculature and tubulointerstitium are relatively protected. These observations may have important implications for our understanding of the role of ACE2 in human kidney disease.
Footnotes
Address reprint requests to Gavin Y. Oudit, M.D., Ph.D., Rm. 68, 150 College St., Heart and Stroke/Richard Lewar Centre of Excellence, Fitzgerald Bldg., University of Toronto, Toronto M5S 3E2, Canada. [email protected] :liam-E
Supported by the Canadian Diabetes Association (grant 456770 to J.M.S., A.M.H., and G.Y.O.) and the Canadian Institute for Health Research New Emerging Team (grant 45723 to J.W.S. and A.M.H.). G.Y.O. is a clinician-scientist supported by the Heart and Stroke Foundation of Canada, Canadian Institute for Health Research and the TACTICS program. Z.K. is supported by the Heart and Stroke Foundation of Canada and a TACTICS post-doctoral fellowship. H.R. is supported by the Kidney Foundation of Canada (Biomedical Research Fellowship). J.M.P. is supported by the Institute for Molecular Biotechnology, Austrian Academy of Sciences, and the Austrian National Bank.
G.Y.O. and A.M.H. contributed equally to this study.
References
- Taal MW, Brenner BM. Renoprotective benefits of RAS inhibition: from ACEI to angiotensin II antagonists. Kidney Int. 2000;57:1803–1817. [Abstract] [Google Scholar]
- Zaman MA, Oparil S, Calhoun DA. Drugs targeting the renin-angiotensin-aldosterone system. Nat Rev Drug Discov. 2002;1:621–636. [Abstract] [Google Scholar]
- Oudit GY, Crackower MA, Backx PH, Penninger JM. The role of ACE2 in cardiovascular physiology. Trends Cardiovasc Med. 2003;13:93–101. [Abstract] [Google Scholar]
- Lewis EJ, Hunsicker LG, Bain RP, Rohde RD, The Collaborative Study Group The effect of angiotensin-converting-enzyme inhibition on diabetic nephropathy. N Engl J Med. 1993;329:1456–1462. [Abstract] [Google Scholar]
- Maschio G, Alberti D, Janin G, Locatelli F, Mann JF, Motolese M, Ponticelli C, Ritz E, Zucchelli P, The Angiotensin-Converting-Enzyme Inhibition in Progressive Renal Insufficiency Study Group Effect of the angiotensin-converting-enzyme inhibitor benazepril on the progression of chronic renal insufficiency. N Engl J Med. 1996;334:939–945. [Abstract] [Google Scholar]
- Investigators HOPES. Effects of ramipril on cardiovascular and microvascular outcomes in people with diabetes mellitus: results of the HOPE study and MICRO-HOPE substudy. Lancet. 2000;355:253–259. [Abstract] [Google Scholar]
- Parving HH, Lehnert H, Brochner-Mortensen J, Gomis R, Andersen S, Arner P. The effect of irbesartan on the development of diabetic nephropathy in patients with type 2 diabetes. N Engl J Med. 2001;345:870–878. [Abstract] [Google Scholar]
- Lewis EJ, Hunsicker LG, Clarke WR, Berl T, Pohl MA, Lewis JB, Ritz E, Atkins RC, Rohde R, Raz I. Renoprotective effect of the angiotensin-receptor antagonist irbesartan in patients with nephropathy due to type 2 diabetes. N Engl J Med. 2001;345:851–860. [Abstract] [Google Scholar]
- Brenner BM, Cooper ME, de Zeeuw D, Keane WF, Mitch WE, Parving HH, Remuzzi G, Snapinn SM, Zhang Z, Shahinfar S. Effects of losartan on renal and cardiovascular outcomes in patients with type 2 diabetes and nephropathy. N Engl J Med. 2001;345:861–869. [Abstract] [Google Scholar]
- Turner AJ, Hiscox JA, Hooper NM. ACE2: from vasopeptidase to SARS virus receptor. Trends Pharmacol Sci. 2004;25:291–294. [Europe PMC free article] [Abstract] [Google Scholar]
- Ferrario CM, Chappell MC. Novel angiotensin peptides. Cell Mol Life Sci. 2004;61:2720–2727. [Abstract] [Google Scholar]
- Vickers C, Hales P, Kaushik V, Dick L, Gavin J, Tang J, Godbout K, Parsons T, Baronas E, Hsieh F, Acton S, Patane M, Nichols A, Tummino P. Hydrolysis of Biological Peptides by Human Angiotensin-converting Enzyme-related Carboxypeptidase. J Biol Chem. 2002;277:14838–14843. [Abstract] [Google Scholar]
- Ferrario CM, Averill DB, Brosnihan KB, Chappell MC, Iskandar SS, Dean RH, Diz DI. Vasopeptidase inhibition and Ang-(1–7) in the spontaneously hypertensive rat. Kidney Int. 2002;62:1349–1357. [Abstract] [Google Scholar]
- Santos RA, Simoes e Silva AC, Maric C, Silva DM, Machado RP, de Buhr I, Heringer-Walther S, Pinheiro SV, Lopes MT, Bader M, Mendes EP, Lemos VS, Campagnole-Santos MJ, Schultheiss HP, Speth R, Walther T. Angiotensin-(1–7) is an endogenous ligand for the G protein-coupled receptor Mas. Proc Natl Acad Sci USA. 2003;100:8258–8263. [Europe PMC free article] [Abstract] [Google Scholar]
- Li W, Moore MJ, Vasilieva N, Sui J, Wong SK, Berne MA, Somasundaran M, Sullivan JL, Luzuriaga K, Greenough TC, Choe H, Farzan M. Angiotensin-converting enzyme 2 is a functional receptor for the SARS coronavirus. Nature. 2003;426:450–454. [Europe PMC free article] [Abstract] [Google Scholar]
- Crackower MA, Sarao R, Oudit GY, Yagil C, Kozieradzki I, Scanga SE, Oliveira-dos-Santos AJ, da Costa J, Zhang L, Pei Y, Scholey J, Ferrario CM, Manoukian AS, Chappell MC, Backx PH, Yagil Y, Penninger JM. Angiotensin-converting enzyme 2 is an essential regulator of heart function. Nature. 2002;417:822–828. [Abstract] [Google Scholar]
- Tikellis C, Johnston CI, Forbes JM, Burns WC, Burrell LM, Risvanis J, Cooper ME. Characterization of renal angiotensin-converting enzyme 2 in diabetic nephropathy. Hypertension. 2003;41:392–397. [Abstract] [Google Scholar]
- Oudit GY, Sun H, Trivieri MG, Koch SE, Dawood F, Ackerley C, Yazdanpanah M, Wilson GJ, Schwartz A, Liu PP, Backx PH. L-type Ca(2+) channels provide a major pathway for iron entry into cardiomyocytes in iron-overload cardiomyopathy. Nat Med. 2003;9:1187–1194. [Abstract] [Google Scholar]
- Kassiri Z, Oudit GY, Sanchez O, Dawood F, Mohammed FF, Nuttall RK, Edwards DR, Liu PP, Backx PH, Khokha R. Combination of tumor necrosis factor-alpha ablation and matrix metalloproteinase inhibition prevents heart failure after pressure overload in tissue inhibitor of metalloproteinase-3 knock-out mice. Circ Res. 2005;97:380–390. [Abstract] [Google Scholar]
- Johnson RJ, Iida H, Alpers CE, Majesky MW, Schwartz SM, Pritzi P, Gordon K, Gown AM. Expression of smooth muscle cell phenotype by rat mesangial cells in immune complex nephritis. Alpha-smooth muscle actin is a marker of mesangial cell proliferation. J Clin Invest. 1991;87:847–858. [Europe PMC free article] [Abstract] [Google Scholar]
- Johnson RJ, Alpers CE, Yoshimura A, Lombardi D, Pritzl P, Floege J, Schwartz SM. Renal injury from angiotensin II-mediated hypertension. Hypertension. 1992;19:464–474. [Abstract] [Google Scholar]
- Gorin Y, Ricono JM, Wagner B, Kim NH, Bhandari B, Choudhury GG, Abboud HE. Angiotensin II-induced ERK1/ERK2 activation and protein synthesis are redox-dependent in glomerular mesangial cells. Biochem J. 2004;381:231–239. [Europe PMC free article] [Abstract] [Google Scholar]
- Agarwal R, Campbell RC, Warnock DG. Oxidative stress in hypertension and chronic kidney disease: role of angiotensin II. Semin Nephrol. 2004;24:101–114. [Abstract] [Google Scholar]
- Kakoki M, Takahashi N, Jennette JC, Smithies O. Diabetic nephropathy is markedly enhanced in mice lacking the bradykinin B2 receptor. Proc Natl Acad Sci USA. 2004;101:13302–13305. [Europe PMC free article] [Abstract] [Google Scholar]
- Remuzzi G, Bertani T. Pathophysiology of progressive nephropathies. N Engl J Med. 1998;339:1448–1456. [Abstract] [Google Scholar]
- Mezzano S, Droguett A, Burgos ME, Ardiles LG, Flores CA, Aros CA, Caorsi I, Vio CP, Ruiz-Ortega M, Egido J. Renin-angiotensin system activation and interstitial inflammation in human diabetic nephropathy. Kidney Int Suppl. 2003:S64–S70. [Abstract] [Google Scholar]
- Inazaki K, Kanamaru Y, Kojima Y, Sueyoshi N, Okumura K, Kaneko K, Yamashiro Y, Ogawa H, Nakao A. Smad3 deficiency attenuates renal fibrosis, inflammation, and apoptosis after unilateral ureteral obstruction. Kidney Int. 2004;66:597–604. [Abstract] [Google Scholar]
- Niimura F, Labosky PA, Kakuchi J, Okubo S, Yoshida H, Oikawa T, Ichiki T, Naftilan AJ, Fogo A, Inagami T, Hogan BLM, Ichikawa I. Gene targeting in mice reveals a requirement for angiotensin in the development and maintenance of kidney morphology and growth factor regulation. J Clin Invest. 1995;96:2947–2954. [Europe PMC free article] [Abstract] [Google Scholar]
- Davisson RL, Kim HS, Krege JH, Lager DJ, Smithies O, Sigmund CD. Complementation of reduced survival, hypotension, and renal abnormalities in angiotensinogen-deficient mice by the human renin and human angiotensinogen genes. J Clin Invest. 1997;99:1258–1264. [Europe PMC free article] [Abstract] [Google Scholar]
- Krege JH, John SW, Langenbach LL, Hodgin JB, Hagaman JR, Bachman ES, Jennette JC, O’Brien DA, Smithies O. Male-female differences in fertility and blood pressure in ACE-deficient mice. Nature. 1995;375:146–148. [Abstract] [Google Scholar]
- Oliverio MI, Kim HS, Ito M, Le T, Audoly L, Best CF, Hiller S, Kluckman K, Maeda N, Smithies O, Coffman TM. Reduced growth, abnormal kidney structure, and type 2 (AT2) angiotensin receptor-mediated blood pressure regulation in mice lacking both AT1A and AT1B receptors for angiotensin II. Proc Natl Acad Sci USA. 1998;95:15496–15501. [Europe PMC free article] [Abstract] [Google Scholar]
- Tsuchida S, Matsusaka T, Chen X, Okubo S, Niimura F, Nishimura H, Fogo A, Utsunomiya H, Inagami T, Ichikawa I. Murine double nullizygotes of the angiotensin type 1A and 1B receptor genes duplicate severe abnormal phenotypes of angiotensinogen nullizygotes. J Clin Invest. 1998;101:755–760. [Europe PMC free article] [Abstract] [Google Scholar]
- Huang W, Gallois Y, Bouby N, Bruneval P, Heudes D, Belair MF, Krege JH, Meneton P, Marre M, Smithies O, Alhenc-Gelas F. Genetically increased angiotensin I-converting enzyme level and renal complications in the diabetic mouse. Proc Natl Acad Sci USA. 2001;98:13330–13334. [Europe PMC free article] [Abstract] [Google Scholar]
- Esther CR, Jr, Howard TE, Marino EM, Goddard JM, Capecchi MR, Bernstein KE. Mice lacking angiotensin-converting enzyme have low blood pressure, renal pathology, and reduced male fertility. Lab Invest. 1996;74:953–965. [Abstract] [Google Scholar]
- Neugarten J, Acharya A, Silbiger SR. Effect of gender on the progression of nondiabetic renal disease: a meta-analysis. J Am Soc Nephrol. 2000;11:319–329. [Abstract] [Google Scholar]
- Gross ML, Adamczak M, Rabe T, Harbi NA, Krtil J, Koch A, Hamar P, Amann K, Ritz E. Beneficial effects of estrogens on indices of renal damage in uninephrectomized SHRsp rats. J Am Soc Nephrol. 2004;15:348–358. [Abstract] [Google Scholar]
- Elliot SJ, Karl M, Berho M, Potier M, Zheng F, Leclercq B, Striker GE, Striker LJ. Estrogen deficiency accelerates progression of glomerulosclerosis in susceptible mice. Am J Pathol. 2003;162:1441–1448. [Europe PMC free article] [Abstract] [Google Scholar]
- Miller JA, Anacta LA, Cattran DC. Impact of gender on the renal response to angiotensin II. Kidney Int. 1999;55:278–285. [Abstract] [Google Scholar]
- Reyes D, Lew SQ, Kimmel PL. Gender differences in hypertension and kidney disease. Med Clin North Am. 2005;89:613–630. [Abstract] [Google Scholar]
- Silbiger S, Lei J, Ziyadeh FN, Neugarten J. Estradiol reverses TGF-beta1-stimulated type IV collagen gene transcription in murine mesangial cells. Am J Physiol. 1998;274:F1113–F1118. [Abstract] [Google Scholar]
- Krepinsky J, Ingram AJ, James L, Ly H, Thai K, Cattran DC, Miller JA, Scholey JW. 17β-Estradiol modulates mechanical strain-induced MAPK activation in mesangial cells. J Biol Chem. 2002;277:9387–9394. [Abstract] [Google Scholar]
- Mankhey RW, Bhatti F, Maric C. 17β-Estradiol replacement improves renal function and pathology associated with diabetic nephropathy. Am J Physiol. 2005;288:F399–F405. [Abstract] [Google Scholar]
- Baumer AT, Wassmann S, Ahlbory K, Strehlow K, Muller C, Sauer H, Bohm M, Nickenig G. Reduction of oxidative stress and AT1 receptor expression by the selective oestrogen receptor modulator idoxifene. Br J Pharmacol. 2001;134:579–584. [Europe PMC free article] [Abstract] [Google Scholar]
- Itagaki T, Shimizu I, Cheng X, Yuan Y, Oshio A, Tamaki K, Fukuno H, Honda H, Okamura Y, Ito S. Opposing effects of oestradiol and progesterone on intracellular pathways and activation processes in the oxidative stress-induced activation of cultured rat hepatic stellate cells. Gut. 2005;54:1782–1789. [Europe PMC free article] [Abstract] [Google Scholar]
- Takeda-Matsubara Y, Nakagami H, Iwai M, Cui TX, Shiuchi T, Akishita M, Nahmias C, Ito M, Horiuchi M. Estrogen activates phosphatases and antagonizes growth-promoting effect of angiotensin II. Hypertension. 2002;39:41–45. [Abstract] [Google Scholar]
- Lautrette A, Li S, Alili R, Sunnarborg SW, Burtin M, Lee DC, Friedlander G, Terzi F. Angiotensin II and EGF receptor cross-talk in chronic kidney diseases: a new therapeutic approach. Nat Med. 2005;11:867–874. [Abstract] [Google Scholar]
- Haugen EN, Croatt AJ, Nath KA. Angiotensin II induces renal oxidant stress in vivo and heme oxygenase-1 in vivo and in vitro. Kidney Int. 2000;58:144–152. [Abstract] [Google Scholar]
- Seshiah PN, Weber DS, Rocic P, Valppu L, Taniyama Y, Griendling KK. Angiotensin II stimulation of NAD(P)H oxidase activity: upstream mediators. Circ Res. 2002;91:406–413. [Abstract] [Google Scholar]
- Kagami S, Border WA, Miller DE, Noble NA. Angiotensin II stimulates extracellular matrix protein synthesis through induction of transforming growth factor-beta expression in rat glomerular mesangial cells. J Clin Invest. 1994;93:2431–2437. [Europe PMC free article] [Abstract] [Google Scholar]
- Border WA, Noble NA. Interactions of transforming growth factor-beta and angiotensin II in renal fibrosis. Hypertension. 1998;31:181–188. [Abstract] [Google Scholar]
- Mezzano SA, Ruiz-Ortega M, Egido J. Angiotensin II and renal fibrosis. Hypertension. 2001;38:635–638. [Abstract] [Google Scholar]
- Lee SH, Oe T, Blair IA. Vitamin C-induced decomposition of lipid hydroperoxides to endogenous genotoxins. Science. 2001;292:2083–2086. [Abstract] [Google Scholar]
- Ye M, Wysocki J, Naaz P, Salabat MR, LaPointe MS, Batlle D. Increased ACE 2 and decreased ACE protein in renal tubules from diabetic mice: a renoprotective combination? Hypertension. 2004;43:1120–1125. [Abstract] [Google Scholar]
- Lely AT, Hamming I, van Goor H, Navis GJ. Renal ACE2 expression in human kidney disease. J Pathol. 2004;204:587–593. [Abstract] [Google Scholar]
Articles from The American Journal of Pathology are provided here courtesy of American Society for Investigative Pathology
Full text links
Read article at publisher's site: https://doi.org/10.2353/ajpath.2006.051091
Read article for free, from open access legal sources, via Unpaywall:
https://europepmc.org/articles/pmc1606622?pdf=render
Citations & impact
Impact metrics
Citations of article over time
Alternative metrics
Article citations
Effects of Two Soluble ACE2-Fc Variants on Blood Pressure and Albuminuria in Hypertensive Mice: Research Letter.
Can J Kidney Health Dis, 10:20543581231207146, 23 Oct 2023
Cited by: 0 articles | PMID: 37881406 | PMCID: PMC10594958
Role of sex hormones in diabetic nephropathy.
Front Endocrinol (Lausanne), 14:1135530, 18 Apr 2023
Cited by: 4 articles | PMID: 37143724 | PMCID: PMC10151816
Review Free full text in Europe PMC
Increased mRNA Levels of ADAM17, IFITM3, and IFNE in Peripheral Blood Cells Are Present in Patients with Obesity and May Predict Severe COVID-19 Evolution.
Biomedicines, 10(8):2007, 18 Aug 2022
Cited by: 0 articles | PMID: 36009555 | PMCID: PMC9406212
Severe acute respiratory syndrome and thyroid: A molecular point of view.
Clin Nutr ESPEN, 48:1-4, 24 Feb 2022
Cited by: 1 article | PMID: 35331481 | PMCID: PMC8866166
Review Free full text in Europe PMC
The mechanism of multiple organ dysfunction syndrome in patients with COVID-19.
J Med Virol, 94(5):1886-1892, 08 Feb 2022
Cited by: 24 articles | PMID: 35088424 | PMCID: PMC9015222
Review Free full text in Europe PMC
Go to all (160) article citations
Data
Similar Articles
To arrive at the top five similar articles we use a word-weighted algorithm to compare words from the Title and Abstract of each citation.
Loss of angiotensin-converting enzyme-2 (Ace2) accelerates diabetic kidney injury.
Am J Pathol, 171(2):438-451, 28 Jun 2007
Cited by: 184 articles | PMID: 17600118 | PMCID: PMC1934545
Angiotensin II-mediated oxidative stress and inflammation mediate the age-dependent cardiomyopathy in ACE2 null mice.
Cardiovasc Res, 75(1):29-39, 21 Apr 2007
Cited by: 162 articles | PMID: 17499227
Deletion of angiotensin-converting enzyme 2 exacerbates renal inflammation and injury in apolipoprotein E-deficient mice through modulation of the nephrin and TNF-alpha-TNFRSF1A signaling.
J Transl Med, 13:255, 06 Aug 2015
Cited by: 22 articles | PMID: 26245758 | PMCID: PMC4527357
Angiotensin-converting enzyme 2 is a key modulator of the renin-angiotensin system in cardiovascular and renal disease.
Curr Opin Nephrol Hypertens, 20(1):62-68, 01 Jan 2011
Cited by: 99 articles | PMID: 21099686
Review