Abstract
Free full text

Asymmetric Self-Renewal and Commitment of Satellite Stem Cells in Muscle
Associated Data
SUMMARY
Satellite cells play a central role in mediating the growth and regeneration of skeletal muscle. However, whether satellite cells are stem cells, committed progenitors, or dedifferentiated myoblasts has remained unclear. Using Myf5-Cre and ROSA26-YFP Cre-reporter alleles, we observed that in vivo 10% of sublaminar Pax7-expressing satellite cells have never expressed Myf5. Moreover, we found that Pax7+/Myf5− satellite cells gave rise to Pax7+/Myf5+ satellite cells through apical-basal oriented divisions that asymmetrically generated a basal Pax7+/Myf5− and an apical Pax7+/Myf5+ cells. Prospective isolation and transplantation into muscle revealed that whereas Pax7+/Myf5+ cells exhibited precocious differentiation, Pax7+/Myf5− cells extensively contributed to the satellite cell reservoir throughout the injected muscle. Therefore, we conclude that satellite cells are a heterogeneous population composed of stem cells and committed progenitors. These results provide critical insights into satellite cell biology and open new avenues for therapeutic treatment of neuromuscular diseases.
INTRODUCTION
Satellite cells reside in a niche beneath the basal lamina closely juxtaposed to the muscle fiber, and are responsible for the growth, maintenance, and repair of postnatal skeletal muscle. Satellite cells are normally mitotically quiescent but are activated (i.e., enter the cell cycle) in response to stress induced by weight bearing or by trauma such as injury. The descendants of activated satellite cells, called myogenic precursor cells, undergo multiple rounds of division prior to terminal differentiation and fusion to form multinucleated myofibers. Activated satellite cells also generate progeny that restore the pool of quiescent satellite cells. However, the molecular mechanisms regulating satellite cell self-renewal and differentiation remain poorly understood (McKinnell et al., 2005).
Early experiments using quail-chick chimeras suggested that satellite cells were derived from the somite (Armand et al., 1983). Recent experiments support this work and indicate that a significant proportion of the progenitors of satellite cells originate in embryonic somites as Pax3/Pax7-expressing cells (Ben-Yair and Kalcheim, 2005; Gros et al., 2005; Kassar-Duchossoy et al., 2005; Relaix et al., 2005; Schienda et al., 2006). In addition, satellite cells may also be derived from cells associated with the embryonic vasculature including the dorsal aorta (De Angelis et al., 1999) and from other adult stem cells during regeneration (Asakura et al., 2002; LaBarge and Blau, 2002; Polesskaya et al., 2003). However, whether satellite cells are stem cells, committed progenitors, or dedifferentiated myoblasts remains unresolved.
Satellite cells are widely considered to be a homogeneous population of committed muscle progenitors (Bischoff, 1994). However, several studies have raised the possibility that the satellite cell compartment may be a heterogeneous population. Radioisotope labeling of growing rat muscle revealed that satellite cells are a mixture of 80% fast-cycling cells and 20% slow-cycling “reserve cells” (Schultz, 1996). Examination of the expression of satellite cell markers CD34, M-cadherin, and Myf5-nLacZ in freshly prepared myofibers suggested that a subpopulation of satellite cells exhibits heterogeneous expression of these markers (Beauchamp et al., 2000). However, the molecular identity of any potential subpopulations has not been defined, and the prospective isolation and characterization of these cells has not been achieved.
Transplantation of the cultured primary myoblasts into regenerating muscle typically results in extensive loss of the transplanted cells, terminal differentiation of the surviving cells, and virtually no contribution to the satellite cell compartment (Beauchamp et al., 1999; El Fahime et al., 2003; Fan et al., 1996; Hodgetts et al., 2000; Qu et al., 1998; Rando and Blau, 1994). By contrast, experiments involving transplant of intact fibers carrying satellite cells (Collins et al., 2005) or fresh isolated satellite cells (Montarras et al., 2005) have suggested that at least some portion of satellite cells have the capacity to repopulate the satellite cell compartment as well as extensively contribute to regenerating muscle.
In the present study, we report the existence of hierarchical subpopulations of satellite cells that are distinct in phenotype and function. We document that sublaminar satellite cells expressing Pax7 are heterogeneous based on Myf5 expression. Satellite cells that express Pax7 but not Myf5 give rise to Myf5-expressing cells through sublaminar cell divisions in a basal-apical orientation. Finally, we observe that Pax7+/Myf5− satellite cells are capable of efficiently contributing to the satellite cell reservoir following prospective isolation and transplantation into Pax7−/− muscle. Together, these experiments demonstrate that satellite cells are a heterogeneous population composed of stem cells and committed myogenic progenitors.
RESULTS
Satellite Cells Are Heterogeneous by Myf5 Expression
Satellite cells uniformly express Pax7 (Seale et al., 2000) and have been suggested to express the Myf5-nLacZ knockin allele in the quiescent sublaminar state (Beauchamp et al., 2000). We hypothesized that Myf5 transcription occurs in satellite cells that had undergone commitment to the myogenic lineage. We reasoned that if we could detect satellite cells that had not expressed Myf5, these would represent a candidate stem cell of the satellite cell compartment.
We first examined whether all satellite cells expressing Pax7 also express Myf5 in single myofiber preparations fixed immediately following isolation from extensor digitorum longus (EDL) muscles of Myf5-nLacZ mice. Immunohistological analysis revealed that the majority of satellite cells contained readily detectable levels of Pax7 and β-Gal proteins. Notably, 13 ± 4% (n = 3 mice, > 100 cells/mouse) of Pax7+ satellite cells did not contain detectable levels of β-Gal, indicating that Myf5 was not uniformly expressed (Figure 1A).
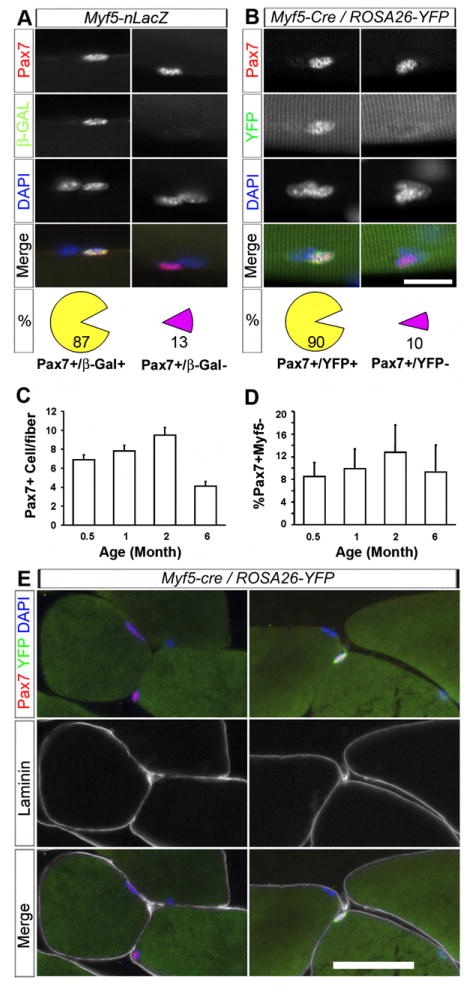
(A) 87% of Pax7+ satellite cells expressed β-Gal (left column), and 13% of Pax7+ cells were β-Gal− (right column, n = 3 mice) on single fibers isolated from Myf5-nLacZ mice.
(B) 90% of Pax7+ cells expressed YFP (left column), and 10% of Pax7+ cells were YFP− (right column, n = 18 mice) on single fibers isolated from Myf5-Cre/ROSA-YFP mice.
(C and D) Total number of Pax7+ satellite cells per EDL myofiber and percentage of Pax7+/YFP− cells at different ages (n = 3, 4, 6, and 4 mice for 0.5-, 1-, 2-, and 6-month-old mice, respectively). Error bars indicate standard error of mean (SEM).
(E) Sublaminar localization of Pax7+/YFP− (left) and Pax7+/YFP+ (right) cells.
Scale bars are 10 μm in (A) and (B) and 25 μm in (E).
To investigate whether the Pax7+/β-Gal− satellite cells reflect a transient downregulation of Myf5 or represent a distinct population that never expressed Myf5, we employed the Cre-LoxP system to genetically mark cells that have expressed Myf5. We generated heterozygous mice carrying a Myf5-Cre allele (Tallquist et al., 2000) and a Cre-dependent YFP reporter knocked into the ubiquitously expressed ROSA26 locus (Srinivas et al., 2001; Figure S1A). In the Myf5-Cre/ROSA-YFP mice, any satellite cells that have once expressed Myf5-Cre should express YFP irreversibly. Conversely, any cells that are YFP− should have never expressed Myf5-Cre in the past.
Analysis of myofibers isolated from Myf5-Cre/ROSA-YFP EDL muscle demonstrated that 90 ± 2% of satellite cells expressing Pax7 also expressed YFP. Importantly, 10 ± 2% (n = 18 mice, >100 cells/mouse) of Pax7+ satellite cells did not contain detectable levels of YFP, confirming that these cells never expressed Myf5 (Figure 1B). The relative proportion of Pax7+/YFP− satellite cells persisted in muscle in mice up to 6 months old (Figures 1C and 1D).
Similar proportions of β-Gal-positive and -negative satellite cells were observed when Myf5-Cre/ROSA26R3 reporter mice that express β-Gal only in the presence of Myf5-Cre were examined (Figure S1B). By contrast, all Pax7+ satellite cells were also YFP+ in Pax3-Cre/ROSA-YFP mice (n = 4 mice, data not shown), confirming the efficacy of the Cre-LoxP system and the notion that satellite cells are derived from embryonic Pax3+/Pax7+ progenitors.
To ask whether the Pax7+/Myf5− represent newborn satellite cells that have never undergone activation, we treated muscles with cardiotoxin (CTX) to induce the activation of satellite cells and muscle regeneration. We observed substantial numbers of both Pax7+/YFP− and Pax7+/β-Gal− cells in regenerating Myf5-Cre/ROSA-YFP and Myf5-nLacZ muscles, respectively (Figure S2). Many of the Pax7+/β-Gal− cells had incorporated BrdU, indicating they were undergoing DNA synthesis and hence were progressing through the cell cycle (Figure S2B). Therefore, the lack of Myf5 expression in satellite cells does not equate to quiescence.
To confirm the satellite cell identity of the Pax7+/YFP− cells, we triple labeled Myf5-Cre/ROSA-YFP muscle sections with antibodies to Pax7, YFP, and laminin. All Pax7+/YFP− cells were located beneath the basal lamina in a satellite cell position (Figure 1E). Furthermore, Pax7+/YFP− and Pax7+/β-Gal− cells were found to express typical satellite cell markers including CD34, M-Cad, Syn4, and NCAM (Figure S3). These data confirm that sublaminar satellite cells are a heterogeneous population on the basis of Myf5 expression.
Prospective Isolation of Pax7+/YFP− and Pax7+/YFP+ Satellite Cells
To further characterize Pax7+/YFP− and Pax7+/YFP+ satellite cells, we developed a method to prospectively isolate the different satellite cell subpopulations based on the previously reported approach using fluorescence-activated cell sorting (FACS) with antibody reactive with the cell-surface protein α7-integrin (int; Blanco-Bose et al., 2001). Immunostaining of isolated myofibers confirmed that α7- and its dimerization partner β1-int were expressed on both YFP− and YFP+ satellite cells (Figures S4A and S4B).
Initially, we employed positive selection for α7-int, together with negative selection (Lin−, for Sca1, CD31, CD45). FACS analysis of mononuclear cells from Myf5-Cre/ROSA-YFP muscle revealed that the α7-int+ fraction contained both YFP+ and YFP− cells (Figure S4B, right column). RT-PCR analysis indicated that mRNA for Pax7, Myf5, and Cre were only present in the α7-int+ fraction (Figure S4C), suggesting that the α7-int+ fraction contained all the Pax7+ satellite cells. However, only 20 ± 5% (n = 4) FACS-isolated α7-int+Lin− YFP− cells were Pax7+; therefore, we introduced β1-int as a second positive-selection marker and further narrowed the gating based on the side-scatter (SSC) range of satellite cells.
Next, we isolated the YFP+/α7β1-int+/Lin− and the YFP−/α7β1-int+/Lin− (termed YFP+ and YFP− hereafter) cells by FACS (Figure 2A). Immunostaining of freshly sorted cells revealed that 90 ± 1% (n = 3) of the YFP+ cells expressed detectable levels of Pax7, whereas 67 ± 9% (n = 3) of YFP− cells expressed Pax7 (Figure 2B). Therefore, we established an approach for the prospective isolation of the different satellite cell subpopulations with a high degree of purity.

(A) Top panels: FACS profile of cells from control mice with no staining. Bottom panels: FACS profile of Myf5-Cre/ROSA-YFP cells stained with PE conjugated to Sca-1, CD31, CD45 (PE-Lin), α7-integrin, and β1-integrin. The gates used to prospectively isolate α7-int+/β1-int+/Lin−/YFP− and α7-int+/β1-int+/Lin−/YFP+ cells are shown by boxed or circled areas. The bottom right panel shows the α7-int and YFP profile after gating.
(B) Pax7 expression in FACS-isolated YFP+ (upper panels) and YFP− (lower panels) cells.
(C) RT-PCR analysis of sorted cells showing the absence of Myf5, Cre, and YFP expression in the YFP− cells. Myoblasts indicates cultured primary myoblasts isolated from Myf5-Cre/ROSA-YFP mice. NTC indicates no template control.
(D) Absence of Myf5 protein in FACS-isolated YFP− cells.
Scale bars are 25 μm.
RT-PCR analysis of YFP+ cells confirmed expression of Pax7, Myf5, Cre, and YFP mRNAs. By contrast, YFP− cells expressed similar levels of Pax7 but did not express Myf5, Cre, or YFP (Figure 2C). Importantly, Myf5 protein was readily detectable in the FACS-purified YFP+ but not in the YFP− cells (Figure 2D). Together, these data directly confirmed that the YFP− cells did not express Myf5 at either mRNA or protein level.
Pax7+/Myf5− Cells Give Rise to Pax7+/Myf5+ Cells
To investigate the developmental origin and relationship between the Pax7+/Myf5− and Pax7+/Myf5+ cells, we examined the satellite cell progenitors in the somites and limb buds of E11.5 embryos. Interestingly, about 10% of Pax7-expressing cells in the dermamyotome and migrating progenitors near the limb bud were YFP− among the large majority of YFP+ progenitors (Figure S5A and S5B).
To analyze the clonal growth of satellite cells on isolated myofibers in vitro, individual myofibers were isolated from Myf5-Cre/ROSA-YFP EDL muscle and cultured in suspension under growth conditions for 1–3 days. Typically, satellite cells undergo their first cell division at around 24 hr and form 4–8 cell aggregates within 3 days. Live-imaging analysis confirmed that these aggregates were of clonal origin (not shown). After 3 days of culture almost all clones uniformly expressed YFP, but the levels of Pax7 within each clone were variable (Figure 3A). Importantly, nearly 10% of the clones contained both Pax7+/YFP− and Pax7+/YFP+ cells at day 3 (Figure 3B) and day 2 (Figure 3C; 6 out of 70 clones from 4 experiments). Since a colony of mixed YFP+ and YFP− cells can only be derived from a common YFP− progenitor, these results indicate that Pax7+/Myf5− cells can form additional Pax7+/Myf5− satellite cells as well as give rise to Pax7+/Myf5+ satellite cells.
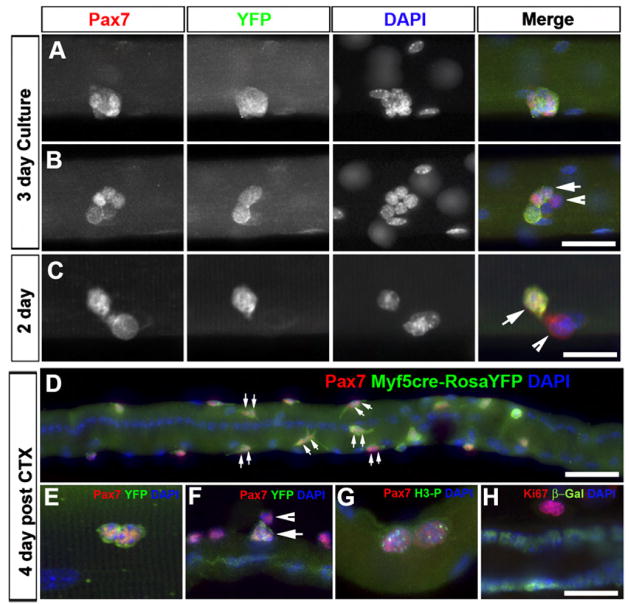
(A) Satellite-cell-derived clonal clusters from Myf5-Cre/ROSA-YFP reporter mice after 3 days in suspended culture. Shown is a clonal cluster of cells that uniformly express YFP.
(B) Shown is a clonal cluster containing both Pax7+/YFP+ (arrow) and Pax7+/YFP− (arrowhead) cells.
(C) A couplet of sister cells with one Pax7+/YFP− cell (arrow head) and one Pax7+/YFP+ cell (arrow) after 2 days in culture.
(D) Extensive in vivo proliferation of satellite cells on a regenerating EDL myofiber 4 days post CTX injection. The majority of satellite cells have undergone mitosis as indicated by the presence of two adjacent Pax7+ nuclei (double arrows) within a single satellite cell niche.
(E) Couplet of identical sister cells that were both Pax7+/YFP+.
(F) Couplet of sister cells with one Pax7+/YFP− cell (arrowhead) and one Pax7+/YFP+ cell (arrow).
(G) Satellite cell couplets expressed the meta-phase mitosis marker phosphorylated his-tone-H3 (H3-P).
(H) Satellite cell couplets expressed the proliferation marker Ki67. β-Gal labeling denotes Myf5-nLacZ expression.
Scale bars are 20 μm in (A), (B), and (D) and 10 μm in (C), (E), (F), (G), and (H).
To confirm that Pax7+/Myf5− cells give rise to Pax7+/Myf5+ cells, we conducted time-lapse imaging analysis of satellite cell division on cultured Myf5-Cre/ROSA-YFP myofibers. We observed that single YFP− satellite cells directly gave rise to one YFP− and one YFP+ cell (Figure 4; Movie S1). Furthermore, we examined expression of MyoD, an established marker for myogenic commitment and differentiation. Notably, Pax7+/YFP− cells did not express MyoD (Figure S5C), consistent with the hypothesis that Pax7+/YFP− cells have yet to undergo myogenic commitment.
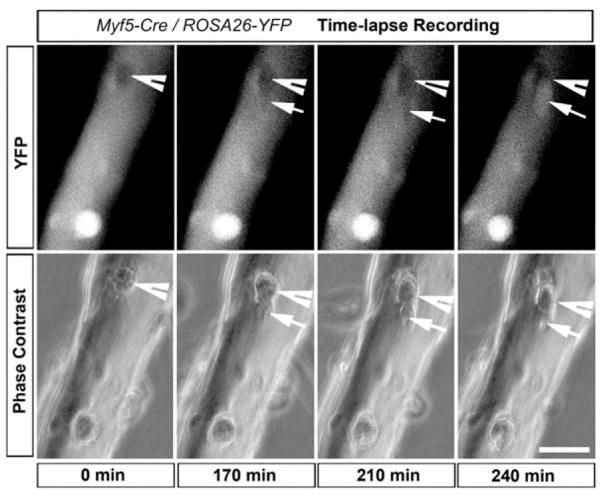
Time-lapse image analysis showing a YFP− satellite cell (arrowhead at T = 0) give rise to YFP+ (arrows) and YFP− (arrowheads) daughter cells. EDL myofibers isolated from Myf5-Cre/ROSA-YFP reporter mouse were plated on Matrigel, and satellite cell growth was monitored with a live imaging system. Cell division occurred at approximately 0 min (around 36 hr in real time), and YFP expression became apparent in the lower daughter cell at 240 min. Scale bar is 25 μm.
To examine satellite cell divisions within their natural niche, we developed an approach to analyze satellite cell proliferation in vivo. Typically, induction of muscle regeneration with CTX results in death of myofibers and massive immunoinfiltration, making it technically difficult to isolate viable myofibers. Therefore, we instead isolated fibers from the adjacent EDL muscle 4–5 days following CTX injection into the tibialis anterior (TA) muscle. We found that this approach allowed us to isolate viable myofibers containing numerous satellite cells that were undergoing cell division in vivo (Figure 3D).
Numerous doublets of sister cells (double arrows in Figure 3D) were observed beneath the basal lamina on viable regenerating myofibers, which contained long arrays of centrally located nuclei (Figure 3D). The close physical proximity of the cells within each doublet, the expression of proliferation markers in both cells (Ki67 and phospho-H3; Figures 3G and 3H), and their sublaminar localization within the same satellite cell niche (Refer to Figures 5C and 5D) evidence the clonal origin of these doublet sister cells. The vast majority of the doublets in Myf5-Cre/ROSA-YFP mice displayed identical expression of Pax7 and YFP (Figure 3E). Nonetheless, we identified doublets of proliferating cells with one Pax7+/YFP− cell and one Pax7+/YFP+ cell (Figure 3F), presumably derived from a common Pax7+/YFP− progenitor.
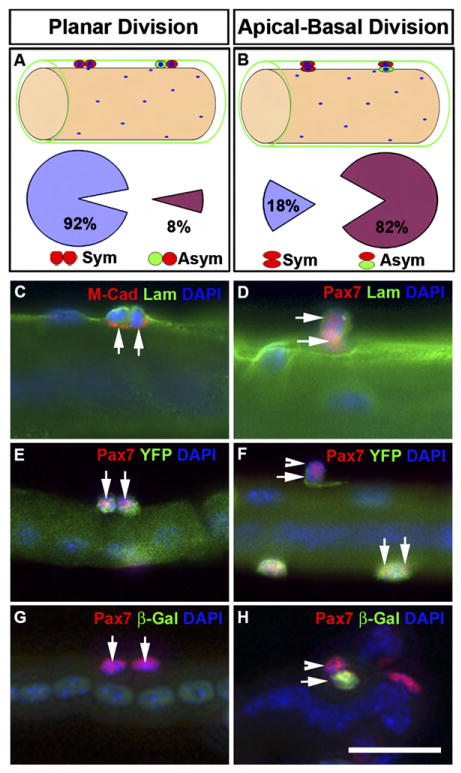
Regenerating EDL myofibers were isolated from Myf5-Cre/ROSA-YFP (C–F) or Myf5-nLacZ (G–H) reporter mice and fixed immediately for labeling of Pax7 and YFP or β-Gal. Myofibers were horizontally oriented in all panels.
(A and B) Schematics of planar (A) and apical-basal (B) oriented sister cells and the corresponding frequency of symmetric (Sym) versus asymmetric (Asym) expression of Myf5 (based on YFP or β-Gal). Satellite cells (red and green cells) are located beneath the basal lamina (green) and are adjacent to the myofiber (brown).
(C and D) Newly formed sister cells (double arrows) were both located under the basal lamina (Lam) but were separated from myofiber by M-cadherin (M-Cad) regardless of their relative orientation (C, planar; D, apical-basal).
(E and F) Examples of planar (double arrows) and apical-basal (arrow and arrowhead)-oriented Pax7+ sister cells that displayed symmetric and asymmetric YFP expression, respectively.
(G and H) Examples of planar (double arrows in G) and apical-basal (arrow and arrowhead in H)-oriented Pax7+ sister cells that displayed symmetric and asymmetric β-Gal expression, respectively. Centrally located myonuclei indicated the regenerating status of the myofibers.
Scale bar is 12.5 μm.
Altogether, our in vivo and in vitro analyses support the notion that Pax7+/Myf5− cells give rise to both Pax7+/Myf5− and Pax7+/Myf5+ cells and therefore represent a reservoir that maintains a hierarchical composition of the satellite cell compartment.
Apical-Basal Location Determines Self-Renewal versus Differentiation
We next investigated whether the fate of daughter cells is determined by their relative orientation within the satellite cell compartment. Theoretically, sister cells can either be in a planar orientation where both cells remain in direct contact with the basal lamina and host myofiber (Figure 5A) or in an apical-basal orientation where one daughter cell is pushed toward the basal lamina and the other cell apically toward the host myofiber (Figure 5B).
Examination of myofibers isolated from regenerating EDL muscle revealed doublets of sister satellite cells beneath the basal lamina in both planar and apical-basal orientations (Figures 5C and 5D). Doublets of cells within the satellite cell niche were predominantly planar in orientation (91%, n = 248 pairs). Planar divisions were observed to give rise almost exclusively (92%, n = 89 pairs) to identical daughter cells that were either both Pax7+/Myf5− or both Pax7+/Myf5+, as reported by Myf5-nLacZ and ROSA-YFP reporter mice (Figures 5A, 5E, and 5G).
Strikingly, we observed apical-basal oriented doublets where the sister cells assumed asymmetric cell fates (82%, n = 38 pairs; Figure 5B). The majority of apical-basal oriented doublets contained a Pax7+/Myf5− cell against the basal surface and a Pax7+/Myf5+ cell located on the apical side against the muscle fiber (Figures 5F, 5H, and S6–S7). In addition, we observed rare events where the basal cell expressed Pax7 and Myf5 and the apical cell had downregulated Pax7 and was presumably undergoing terminal differentiation (Figure S7).
Taken together, these results demonstrate that satellite cell niche plays an important role in the maintenance of stem cell identity of newly divided daughter cells. The daughter cell attached to the basal lamina remains Myf5−, whereas the daughter that loses contact with the basal lamina upregulates Myf5 and becomes a committed myogenic cell.
Notch Regulation of Asymmetric Self-Renewal
To further explore the signaling mechanism underlying the apical-basal asymmetric self-renewal of satellite stem cells, we conducted gene-expression analysis of FACS-purified YFP+ and YFP− satellite cells. Importantly, YFP+ cells expressed the Notch ligand Delta-1 whereas YFP− cells did not (Figure 6A). Moreover, YFP− cells but not YFP+ cells expressed high levels of Notch-3 (Figure 6A). By contrast, Notch-1 and Notch-2 receptors and the Notch signaling inhibitor Numb were equally expressed in both cell types.
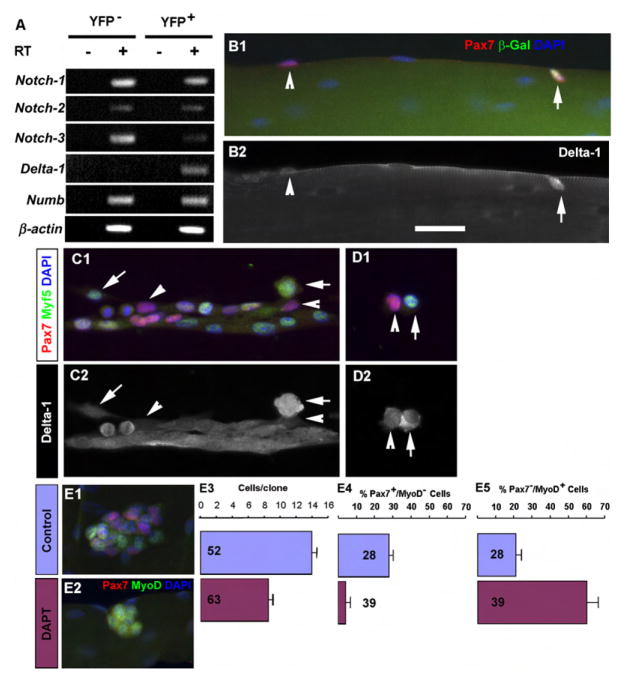
(A) RT-PCR analysis of FACS-purified YFP− and YFP+ satellite cells from Myf5-Cre/ROSA-YFP muscles.
(B) Fresh isolated Myf5-nLacZ myofiber showing Pax7+/β-Gal− (arrowhead) and Pax7+/β-Gal+ (arrow) satellite cells (B1) and their corresponding expressions of Delta-1 (B2).
(C) Satellite-cell-derived cultures showing differential expression of Delta-1 in Pax7+/Myf5− (arrowhead) and Pax7+/Myf5+ (arrows) cells.
(D) High levels of Delta-1 were observed in Pax7+/Myf5+ cells and low levels of Delta-1 in Pax7+/Myf5− cells in newly formed sister doublets.
(E) Abrogation of satellite cell self-renewal by the γ-secretase inhibitor DAPT. (E1–E2) Expression of the self-renewing marker Pax7 and myogenic differentiation marker MyoD in colonies of satellite cell progenies in control (E1) and DAPT (E2)-treated (3 day) myofiber cultures. Notice the lack of Pax7 expression in DAPT-treated cells. (E3–E5) Quantification of cell number (E3) and the relative proportion of Pax7+ (E4) and MyoD+ (E5) cells. Numbers in the graphs indicate n. Error bars indicate SEM.
Scale bar is 15 μm.
Immunostaining of isolated myofibers revealed that Delta-1 was expressed at high levels in Pax7+/Myf5+ satellite cells but low levels in Pax7+/Myf5− cells (Figure 6B). In cultured satellite cells, Delta-1 expression was upregulated in Myf5+ but not in Myf5− cells (Figure 6C). In newly divided cells, high-level Delta-1 expression was observed specifically in Myf5-expressing cells (Figures 6C–6D).
To directly test the role of Notch signaling in satellite cell self-renewal, we treated proliferating satellite cells on freshly isolated myofibers with DAPT, an inhibitor of γ-secretase that is necessary for Notch signaling. DAPT treatment significantly reduced the total number of cells after 3 days of culture. Importantly, Pax7+/MyoD− satellite cells were markedly reduced in number following DAPT treatment. By contrast, the percentage of Pax7−/MyoD+ cells was significantly increased (Figure 6E). Together, these results strongly suggest that Notch signaling plays a critical role in the maintenance of Pax7+/YFP− satellite cells in an undifferentiated stem cell state.
Transplanted Pax7+/Myf5− Cells Extensively Contribute to the Satellite Cell Compartment
Our experiments provided compelling evidence that satellite cells are a hierarchal population composed of Pax7+/Myf5− and Pax7+/Myf5+ satellite cells. To examine the biological function of these two subpopulations of satellite cells, we transplanted FACS-purified YFP+ and YFP− satellite cells from Myf5-Cre/ROSA-YFP mice into Pax7−/− muscle and assessed: (1) their ability to terminally differentiate and (2) their contribution to the satellite cell compartment. Notably, Pax7−/− mice lack functional satellite cells (Kuang et al., 2006; Seale et al., 2000). Therefore, the transplanted cells can readily colonize the unoccupied satellite cell niche.
Three weeks after transplantation (900–10,000 cells/TA muscle), we observed donor-derived cells as indicated by the expression of YFP or Pax7 (n = 6 animals in each group). Both YFP+ and YFP− donor cells were capable of undergoing terminal differentiation as revealed by the presence YFP+ myofibers (Figures 7A and 7B). Transplantation of YFP+ cells typically gave rise to clusters of 20–60 YFP+ myofibers at the injection site, whereas transplanted YFP− cells formed 0–22 YFP+ fibers. Furthermore, we observed in half of the transplanted muscles (n = 6) a massive accumulation of cells (DAPI nuclei in Figures 7A and 7E) surrounding the newly formed YFP+ myofibers, suggesting a host immune reaction against donor cells. Little or no immune reaction was observed in muscle transplanted with YFP− cells (Figures 7B, 7D, and 7F). These data indicate that YFP+ donor cells readily differentiated following transplantation.

TA muscles of Pax7−/− mice were engrafted with freshly sorted YFP+ and YFP− cells from Myf5-Cre/ROSA-YFP mice. Three weeks later, transplanted muscles were examined for the expression of YFP and Pax7.
(A and B) Transplanted YFP+ (A) and YFP− (B) satellite cells both differentiated into YFP+ myofibers; however, YFP− satellite cells also gave rise to several Pax7+/YFP− cells associated with host (YFP−) myofibers.
(C) Transplanted YFP+ cells gave rise to a satellite cell (arrowhead) that was associated with a YFP+ fiber.
(D) Transplanted YFP− satellite cells gave rise to several Pax7+/YFP− (arrows) satellite cells that migrated onto host fibers (YFP−) and located beneath the basal lamina (white staining). Notice that one Pax7+/YFP+ (arrowhead) cell closely associated with a YFP+ myofiber.
(E) TA muscle transplanted with YFP+ satellite cells showing numerous nuclei surrounding a YFP+ myofiber and two interstitial Pax7+/YFP+ cells (arrowheads) indicative of host immune rejection.
(F) TA muscle transplanted with YFP− satellite cells showing several Pax7+/YFP− cells (arrows) and two YFP+ cells (arrowheads) associated with host (YFP−) myofibers and minimal immune rejection.
(G and H) Number of donor-derived satellite cells/TA cross-section after transplantation of YFP+ (G) and YFP− (H) satellite cells (n = 6 mice). Error bars indicate SEM.
Scale bars are 25 μm in (A) and (B) and 12.5 μm in (C)–(F).
Transplanted YFP− cells efficiently gave rise to both Pax7+/YFP− and Pax7+/YFP+ satellite cells (94 ± 32 cells/section/10000 Pax7+ cells injected or 13 ± 3 cells/cross-section, n = 6 mice). Many YFP− satellite cells were observed to have migrated large distances into the host muscle tissue, and were located in sublaminar positions (Figures 7D and 7F). By contrast, transplanted YFP+ cells only occasionally gave rise to Pax7+/YFP+ satellite cells (12 ± 7 cells/section/10000 Pax7+ cells injected or 4 ± 2 cells/cross-section, n = 6 mice), and these were virtually all associated with the new YFP+ myofibers formed at the injection site (Figure 7C). Rare mononuclear YFP+ cells were also observed in interstitial spaces between fibers (Figure 7E).
Finally, we assessed the relative repopulating efficiency of YFP− and YFP+ cells after engrafting myofibers isolated from Myf5-Cre/ROSA-YFP mice into Pax7−/− recipients. We hypothesized that the relative ratio of reconstituted YFP− and YFP+ satellite cells should remain unchanged or decline if both populations repopulate equally well (given that some YFP− can turn into YFP+ cells). Ten freshly isolated EDL myofibers containing 7% YFP− and 93% YFP+ satellite cells were grafted into the TA muscle of Pax7−/− mice. Four weeks later, the satellite cells derived from the grafted fibers differentiated into bundles of 20–100 YFP+ myofibers and also gave rise to numerous Pax7+ satellite cells (14.7 ± 2.5 cells/cross-section, three mice; Figure S8). Importantly, 24 ± 8% of engrafted Pax7+ satellite cells remained YFP−. Most of these YFP− satellite cells had migrated away from the injection site and infiltrated the surrounding muscle tissue to repopulate the host muscle. By contrast, almost all of the YFP+ satellite cells remained at the injection site (Figure S8). Consistent with the notion that Pax7+/Myf5− satellite cells represent a stem cell population within the satellite cell compartment, YFP− satellite cells rapidly expanded in number as they repopulated the vacant satellite cell niche in Pax7−/− muscle.
Together, these data indicate that transplanted Pax7+/Myf5+ satellite cells preferentially undergo terminal differentiation, whereas transplanted Pax7+/Myf5− satellite cells extensively contribute to the satellite compartment and give rise to both classes of satellite cells. Therefore, we conclude that the Pax7+/Myf5− satellite cells represent a novel population of stem cells that actively maintain the homeostatic composition of stem cells and committed progenitors within the satellite cell niche.
DISCUSSION
Previously, our laboratory found that satellite cells uniformly express the transcription factor Pax7 (Seale et al., 2000). Using a Myf5-Cre knockin allele and a ROSA-YFP Cre reporter, we observed that in vivo about 10% of satellite cells express Pax7 but have never expressed Myf5. Moreover, we found that Pax7+/Myf5− satellite cells give rise to Pax7+/Myf5+ satellite cells through basal-apical oriented divisions within the satellite cell niche. Prospective isolation followed by transplantation confirmed that Pax7+/Myf5+ satellite cells preferentially differentiate, whereas Pax7+/Myf5− satellite cells extensively contribute to the satellite cell compartment. Therefore, anatomical and functional experiments provide compelling evidence that the satellite cell population is composed of hierarchal subpopulations of stem cells (Pax7+/Myf5−) and committed myogenic progenitors (Pax7+/Myf5+). This discovery provides a new paradigm that redefines satellite cell biology and opens new doors for therapeutic intervention for the treatment of devastating neuromuscular diseases such as Duchenne muscular dystrophy (DMD).
Transplantation experiments have raised the possibility that only a portion of satellite cells exhibit repopulating activity. Unlike cultured primary myoblasts (Collins et al., 2005; Cousins et al., 2004; Heslop et al., 2001), some portion of freshly isolated satellite cells or single myofibers carrying satellite cells give rise to satellite cells after transplantation (Collins et al., 2005; Montarras et al., 2005). These results suggest that satellite cells in vivo contain a subpopulation of repopulating cells that are lost in culture. Our findings are consistent with this body of work and demonstrate that repopulating cells represent a pool of premyogenic cell as evidenced by lack of Myf5 expression.
Recent advances have provided important insights into the role played by the microenvironment in regulating stem cell identity and the asymmetric generation of committed daughter cells (Fuchs et al., 2004; Knoblich, 2001; Moore and Lemischka, 2006). In several other systems, stem cell polarity and spindle orientation relative to the basal lamina determines the fate of daughter cells. Stem cell divisions in a planar orientation are symmetric and generate identical daughter stem cells. By contrast, stem cell divisions in an apical-basal orientation are asymmetric and give rise to a stem cell at the basal surface and a committed daughter cell destined for differentiation on the apical surface (Fuchs et al., 2004; Knoblich, 2001; Lechler and Fuchs, 2005). Therefore, the discovery of the stem cell niche provides an anatomical means to establish the identity of a candidate stem cell population within a particular tissue.
Muscle satellite cells occupy a niche featuring a structural foundation for asymmetric self-renewal. The basal side of satellite cells is covered by the basal lamina ensheathing the muscle fiber, while the apical side of satellite cells is adjacent to the myofiber. The laminin receptor α7β1 integrin is specifically expressed in satellite cells on the basal surface, whereas the cell-adhesion molecule M-cadherin is specifically expressed on the apical surface toward the muscle fiber (Bornemann and Schmalbruch, 1994; Burkin and Kaufman, 1999; Collo et al., 1993; Irintchev et al., 1994). Our data document the asymmetric generation of Pax7+/Myf5− stem cells and Pax7+/Myf5+ committed cells upon apical-basal oriented cell divisions as well as symmetric generation of daughters upon planar cell divisions. Notably, we observed that Pax7+/Myf5− cells remained at the basal surface, whereas Pax7+/Myf5+ cells were localized to the apical surface against the muscle fiber. Presumably the apical cell became committed due to loss of contact with the basal lamina and the extracellular matrix. These results suggest that the satellite cell niche plays an important role in regulating both the maintenance of stem cell identity and the generation of committed daughter cells through asymmetric cell divisions.
Within the stem cell niche, signaling pathways such as Wnt, Notch, BMP/TGFβ, and STAT and proteins such as Numb, PAR, PKCζ, LGL, and NUMA have been implicated in the regulation of asymmetric cell division in model systems (Betschinger and Knoblich, 2004; Betschinger et al., 2003; Fuchs et al., 2004; Knoblich, 2001; Knoblich et al., 1995; Moore and Lemischka, 2006; Rhyu et al., 1994). We observed that sublaminar satellite stem cells displayed elevated expression of Notch-3, whereas committed progenitors exhibited upregulation of Delta-1. We further demonstrated that in newly divided primary myoblasts the level of Delta-1 is high in Myf5+ and low in Myf5− sister cells. Moreover, we observed that inhibition of Notch signaling resulted in the loss of the Pax7+/Myf5− population. Therefore, activation of the Notch signaling pathway, probably stimulated by Delta-1 ligand and Notch-3 receptor interactions, plays a positive role in the self-renewal of satellite stem cells.
Several lines of evidence implicate Notch signaling in the regulation of satellite cell function. Delta-1 inhibits myogenic differentiation in cell culture and during embryonic myogenesis (Delfini et al., 2000; Kuroda et al., 1999). Cosegregation of template DNA strand and asymmetric distribution of Numb to one daughter cell were observed during satellite cell division (Conboy et al., 2005; Shinin et al., 2006). Furthermore, asymmetric distribution of Numb to the basal side of dividing cells occurs in the dermamyotome during embryonic myogenesis (Holowacz et al., 2006; Venters and Ordahl, 2005). Finally, Inscuteable and Numb are segregated to the apical and basal sibling cells, respectively, during muscle differentiation in Drosophila, and perturbation of this segregation pattern leads to the failure of muscle development (Carmena et al., 1998). One of the challenges for future study is to pinpoint the intrinsic and extrinsic molecules and signaling pathways that control the asymmetric distribution of Delta and Numb in satellite cells and how this asymmetry leads to cell fate segregation.
BrdU-labeling experiments in freshly isolated satellite cells have suggested that template DNA cosegregates with Numb in label-retaining cells that express the self-renewal marker Pax7 (Shinin et al., 2006). The relationship between Pax7+/Myf5− satellite cells and label-retaining cells thus represents an interesting question. In preliminary short-term label-retention experiments, we applied a short pulse of BrdU to label the newly synthesized DNA strand in proliferating satellite cells, which was followed by a 3 day chase. From cross-sections, we observed that 14% (n = 71) of Pax7+/Myf5− cells were BrdU+, whereas 41% (n = 309) of Pax7+/Myf5+ cells were BrdU+. From single myofibers, we observed cosegregation of template DNA to Pax7+/Myf5− cells in 38% (5 out of 13) of newly divided sister cells. By contrast, only 9% (2 out of 23) of newly divided Pax7+/Myf5+ cells displayed cosegregation of template DNA to one sister cell. These results are consistent with the observations of Shinin and coworkers (Shinin et al., 2006).
In studies of mdx mice, a mouse model of human DMD, it has been noted that a radiation-resistant subpopulation of satellite cells is depleted relative to wild-type muscle (Heslop et al., 2000). Moreover, satellite-cell-derived myoblasts display accelerated differentiation kinetics when isolated from mdx mice (Yablonka-Reuveni and Anderson, 2006). These data suggest that under pathological conditions, the equilibrium between stem cells and committed progenitors within the satellite cell compartment is perturbed. It is interesting to speculate that this phenomenon contributes to the progression and severity of DMD.
It is worth mentioning that symmetric expansion of satellite stem cells and myogenic cells may represent a primary means of cell division during CTX-induced muscle regeneration, as manifested by the focal enrichment of Pax7+/YFP− cells (Figure S2). We suspect that symmetric expansion of stem cells represents the primary mechanism of self-renewal under certain devastating pathological conditions, whereas asymmetric self-renewal occurs as the primary mechanism for the maintenance of the homeostasis of satellite cells under normal physiological conditions.
The identification of a satellite stem cell represents an important advance in our understanding of satellite cell biology and opens new avenues to explore for the treatment of degenerative neuromuscular diseases. Satellite stem cells could be used for direct transplantation into diseased muscle. Molecular characterization of satellite stem cells should lead to identification of markers for their prospective isolation from human muscle tissue. Alternatively, understanding the molecular regulation of satellite stem cell symmetric versus asymmetric cell division will lead to identification of biologics or small drugs that specifically target the relevant pathway leading to satellite stem cell expansion. Future experiments will investigate both the utility of satellite stem cells for cell transplantation and the molecular control of cell fate determination.
EXPERIMENTAL PROCEDURES
Mice and Animal Care
ROSA26-LacZ (ROSA26R3) reporter mice, mdx mice carrying homologous mutated dystrophin gene, and Pax3-Cre mice strains were purchased from JaxMice (Jackson Laboratories, MI). Heterozygous Myf5-nLacZ (Tajbakhsh et al., 1996) mice were directly used, whereas Myf5-Cre (Tallquist et al., 2000) heterozygous mice were bred with ROSA26-YFP (Srinivas et al., 2001), also referred to as “ROSA-YFP” in the text, or ROSA26R3 reporter mice. Pax7 mutant mice carrying the lacZ knockin mutation were maintained in an inbred 129SV/J background (Kuang et al., 2006). All mice are maintained inside a barrier facility, and experiments were performed in accordance with the University of Ottawa regulations for animal care and handling.
Myofiber Isolation and Immunohistochemistry
Single myofibers were isolated from the EDL muscles as previously described (Rosenblatt et al., 1995). To induce activation of satellite cells, 6- to 8-week-old Myf5-nLacz or Myf5-Cre/ROSA-YFP mice were injected with 25 μl CTX (10 μM, Latoxan, France) into the TA muscles and examined 4 days later. For analysis of satellite cell proliferation in vitro, single myofibers were either plated on Matrigel-coated chamber slides or cultured in suspension in 60 mm Petri dishes coated with horse serum to prevent fiber attachment (Kuang et al., 2006). Primary myoblasts were isolated from hindlimb muscles and cultured as previously reported (Megeney et al., 1996).
Histological processing and immunochemical labeling of cryosections, single myofibers, and cells were performed as previously reported (Kuang et al., 2006). M.O.M. blocking kit (BMK-2202, Vector Lab) was used in staining of muscle sections. Primary antibodies are listed in Table S1. Secondary antibodies used were Alexa488, Alexa568, and Alexa647 conjugated to specific IgG types (Invitrogen Molecular Probes) that matched the primary antibodies (Invitrogen Molecular Probes). Zenon tricolor anti-Rabbit IgG labeling kit (Invitrogen Molecular Probes) and Avidin-conjugated fluorophores were also used where applicable. Nuclei were counterstained with DAPI.
Cell Sorting, PCR Analysis, and Transplantation
Cells were isolated from hindlimb muscle of 6- to 8-week-old mice as previously described (Megeney et al., 1996). Erythrocytes were removed with the Red Blood Cell Lysing Buffer Hybri-Max (Sigma). Mononuclear cells were blocked with goat serum for 10 min and incubated with primary antibodies in DMEM with 2% FBS at 1–3 × 107 cell/ml for 15 min at 4°C. Cells were briefly washed and incubated with appropriate secondary antibodies (1: 1000) at 4°C for 15 min. After staining, cells were washed, passed through 30 μm filters (Miltenyi Biotec) and suspended at a concentration of 1 × 107 cells/ml. Cells were separated on a MoFlo cytometer (DakoCytomation) equipped with three lasers. Sorting gates were strictly defined based on single antibody-stained control cells as well as the forward and SSC patterns of satellite cells based on preliminary tests. Total RNA was prepared from 2 × 104 of sorted cell populations by TRIzol (GIBCO) or MicroRNA kit (Qiagen). cDNA synthesis and PCR were performed as previously described (Minoguchi et al., 1997). Primers are listed in Table S2.
Equal numbers of FACS-purified YFP− and YFP+ cells were injected with 0.5 cc insulin syringes into the left and right TA muscles of the same Pax7−/− mouse. The number of cells used for each injection varied from 900–10,000 based on availability of FACS-purified cells. Injected TA muscles were harvested 3 weeks later for analysis. For myofiber graft, 10 EDL myofibers were grafted together with 10 μl DMEM into the TA muscles of Pax7−/− mice with custom-made glass needles controlled by a microsyringe. Grafted muscles were collected 4 weeks later for analysis.
Microscopy and Live Imaging Analysis
Samples were visualized with a Zeiss Axioplan 2 microscope, and images were acquired using a Zeiss AxioCam camera and the Axioview 3.1 software as previously described (Kuang et al., 2006). For clonal tracing of satellite cells, single myofiber cultures were monitored using a Zeiss Axiovert 200 microscope equipped with an incubator (XL-3, Zeiss), a CO2 controller, and a heating unit that cooperate to maintain 37°C and 5% CO2 within the incubator. Fluorescent and phase-contrast images were recorded at one frame every 5 min using a 20× objective (LD Plan-Neofluar N.A. 0.4) with a Zeiss AxioCam and the AxioView 4.5 software.
Supplementary Material
Supplementary Figures
Supplementary information
Acknowledgments
We thank Caroline Vergette and Vanessa Seale for expert technical assistance and Iain McKinnell and Mark Gillespie for careful reading of the manuscript. We thank Drs. P. Soriano for the Myf5-Cre mice, F. Costantini for the ROSA-YFP mice, and S. Tajbakhsh for the Myf5-nLacZ mice. M.A.R. holds the Canada Research Chair in Molecular Genetics and is an International Research Scholar of the Howard Hughes Medical Institute. This work was supported by grants to M.A.R. from the Canadian Institutes of Health Research, the National Institutes of Health, Muscular Dystrophy Association, the Howard Hughes Medical Institute, and the Canada Research Chair Program. S.K. was supported by an NSERC Postdoctoral Fellowship.
Footnotes
Supplemental Data
Supplemental Data include eight figures, two tables, and one movie and can be found with this article online at http://www.cell.com/cgi/content/full/129/5/999/DC1/.
The authors declare no conflict of interests.
References
- Armand O, Boutineau AM, Mauger A, Pautou MP, Kieny M. Origin of satellite cells in avian skeletal muscles. Arch Anat Microsc Morphol Exp. 1983;72:163–181. [Abstract] [Google Scholar]
- Asakura A, Seale P, Girgis-Gabardo A, Rudnicki MA. Myogenic specification of side population cells in skeletal muscle. J Cell Biol. 2002;159:123–134. [Europe PMC free article] [Abstract] [Google Scholar]
- Beauchamp JR, Morgan JE, Pagel CN, Partridge TA. Dynamics of myoblast transplantation reveal a discrete minority of precursors with stem cell-like properties as the myogenic source. J Cell Biol. 1999;144:1113–1122. [Europe PMC free article] [Abstract] [Google Scholar]
- Beauchamp JR, Heslop L, Yu DS, Tajbakhsh S, Kelly RG, Wernig A, Buckingham ME, Partridge TA, Zammit PS. Expression of CD34 and myf5 defines the majority of quiescent adult skeletal muscle satellite cells. J Cell Biol. 2000;151:1221–1234. [Europe PMC free article] [Abstract] [Google Scholar]
- Ben-Yair R, Kalcheim C. Lineage analysis of the avian dermomyotome sheet reveals the existence of single cells with both dermal and muscle progenitor fates. Development. 2005;132:689–701. [Abstract] [Google Scholar]
- Betschinger J, Mechtler K, Knoblich JA. The Par complex directs asymmetric cell division by phosphorylating the cytoskeletal protein Lgl. Nature. 2003;422:326–330. [Abstract] [Google Scholar]
- Betschinger J, Knoblich JA. Dare to be different: asymmetric cell division in Drosophila, C. elegans and vertebrates. Curr Biol. 2004;14:R674–R685. [Abstract] [Google Scholar]
- Bischoff R. The satellite cell and muscle regeneration. In: Engel AG, Franzini-Armstrong C, editors. Myology. New York: McGraw-Hill; 1994. pp. 97–118. [Google Scholar]
- Blanco-Bose WE, Yao CC, Kramer RH, Blau HM. Purification of mouse primary myoblasts based on alpha 7 integrin expression. Exp Cell Res. 2001;265:212–220. [Abstract] [Google Scholar]
- Bornemann A, Schmalbruch H. Immunocytochemistry of M-cadherin in mature and regenerating rat muscle. Anat Rec. 1994;239:119–125. [Abstract] [Google Scholar]
- Burkin DJ, Kaufman SJ. The alpha7beta1 integrin in muscle development and disease. Cell Tissue Res. 1999;296:183–190. [Abstract] [Google Scholar]
- Carmena A, Murugasu-Oei B, Menon D, Jimenez F, Chia W. Inscuteable and numb mediate asymmetric muscle progenitor cell divisions during Drosophila myogenesis. Genes Dev. 1998;12:304–315. [Europe PMC free article] [Abstract] [Google Scholar]
- Collins CA, Olsen I, Zammit PS, Heslop L, Petrie A, Partridge TA, Morgan JE. Stem cell function, self-renewal, and behavioral heterogeneity of cells from the adult muscle satellite cell niche. Cell. 2005;122:289–301. [Abstract] [Google Scholar]
- Collo G, Starr L, Quaranta V. A new isoform of the laminin receptor integrin alpha 7 beta 1 is developmentally regulated in skeletal muscle. J Biol Chem. 1993;268:19019–19024. [Abstract] [Google Scholar]
- Conboy IM, Conboy MJ, Wagers AJ, Girma ER, Weissman IL, Rando TA. Rejuvenation of aged progenitor cells by exposure to a young systemic environment. Nature. 2005;433:760–764. [Abstract] [Google Scholar]
- Cousins JC, Woodward KJ, Gross JG, Partridge TA, Morgan JE. Regeneration of skeletal muscle from transplanted immortalised myoblasts is oligoclonal. J Cell Sci. 2004;117:3259–3269. [Abstract] [Google Scholar]
- De Angelis L, Berghella L, Coletta M, Lattanzi L, Zanchi M, Cusella-De Angelis MG, Ponzetto C, Cossu G. Skeletal myogenic progenitors originating from embryonic dorsal aorta coexpress endothelial and myogenic markers and contribute to postnatal muscle growth and regeneration. J Cell Biol. 1999;147:869–878. [Europe PMC free article] [Abstract] [Google Scholar]
- Delfini M, Hirsinger E, Pourquie O, Duprez D. Delta 1-activated notch inhibits muscle differentiation without affecting Myf5 and Pax3 expression in chick limb myogenesis. Development. 2000;127:5213–5224. [Abstract] [Google Scholar]
- El Fahime E, Bouchentouf M, Benabdallah BF, Skuk D, Lafreniere JF, Chang YT, Tremblay JP. Tubulyzine, a novel tri-substituted triazine, prevents the early cell death of transplanted myogenic cells and improves transplantation success. Biochem Cell Biol. 2003;81:81–90. [Abstract] [Google Scholar]
- Fan Y, Maley M, Beilharz M, Grounds M. Rapid death of injected myoblasts in myoblast transfer therapy. Muscle Nerve. 1996;19:853–860. [Abstract] [Google Scholar]
- Fuchs E, Tumbar T, Guasch G. Socializing with the neighbors: stem cells and their niche. Cell. 2004;116:769–778. [Abstract] [Google Scholar]
- Gros J, Manceau M, Thome V, Marcelle C. A common somitic origin for embryonic muscle progenitors and satellite cells. Nature. 2005;435:954–958. [Abstract] [Google Scholar]
- Heslop L, Morgan JE, Partridge TA. Evidence for a myogenic stem cell that is exhausted in dystrophic muscle. J Cell Sci. 2000;113:2299–2308. [Abstract] [Google Scholar]
- Heslop L, Beauchamp JR, Tajbakhsh S, Buckingham ME, Partridge TA, Zammit PS. Transplanted primary neonatal myoblasts can give rise to functional satellite cells as identified using the Myf5nlacZl+ mouse. Gene Ther. 2001;8:778–783. [Abstract] [Google Scholar]
- Hodgetts SI, Beilharz MW, Scalzo AA, Grounds MD. Why do cultured transplanted myoblasts die in vivo? DNA quantification shows enhanced survival of donor male myoblasts in host mice depleted of CD4+ and CD8+ cells or Nk1.1+ cells. Cell Transplant. 2000;9:489–502. [Abstract] [Google Scholar]
- Holowacz T, Zeng L, Lassar AB. Asymmetric localization of numb in the chick somite and the influence of myogenic signals. Dev Dyn. 2006;235:633–645. [Europe PMC free article] [Abstract] [Google Scholar]
- Irintchev A, Zeschnigk M, Starzinski-Powitz A, Wernig A. Expression pattern of M-cadherin in normal, denervated, and regenerating mouse muscles. Dev Dyn. 1994;199:326–337. [Abstract] [Google Scholar]
- Kassar-Duchossoy L, Giacone E, Gayraud-Morel B, Jory A, Gomes D, Tajbakhsh S. Pax3/Pax7 mark a novel population of primitive myogenic cells during development. Genes Dev. 2005;19:1426–1431. [Europe PMC free article] [Abstract] [Google Scholar]
- Knoblich JA. The Drosophila nervous system as a model for asymmetric cell division. Symp Soc Exp Biol. 2001;53:75–89. [Abstract] [Google Scholar]
- Knoblich JA, Jan LY, Jan YN. Asymmetric segregation of Numb and Prospero during cell division. Nature. 1995;377:624–627. [Abstract] [Google Scholar]
- Kuang S, Charge SB, Seale P, Huh M, Rudnicki MA. Distinct roles for Pax7 and Pax3 in adult regenerative myogenesis. J Cell Biol. 2006;172:103–113. [Europe PMC free article] [Abstract] [Google Scholar]
- Kuroda K, Tani S, Tamura K, Minoguchi S, Kurooka H, Honjo T. Delta-induced Notch signaling mediated by RBP-J inhibits MyoD expression and myogenesis. J Biol Chem. 1999;274:7238–7244. [Abstract] [Google Scholar]
- LaBarge MA, Blau HM. Biological progression from adult bone marrow to mononucleate muscle stem cell to multinucleate muscle fiber in response to injury. Cell. 2002;111:589–601. [Abstract] [Google Scholar]
- Lechler T, Fuchs E. Asymmetric cell divisions promote stratification and differentiation of mammalian skin. Nature. 2005;437:275–280. [Europe PMC free article] [Abstract] [Google Scholar]
- McKinnell IW, Parise G, Rudnicki MA. Muscle stem cells and regenerative myogenesis. Curr Top Dev Biol. 2005;71:113–130. [Abstract] [Google Scholar]
- Megeney LA, Kablar B, Garrett K, Anderson JE, Rudnicki MA. MyoD is required for myogenic stem cell function in adult skeletal muscle. Genes Dev. 1996;10:1173–1183. [Abstract] [Google Scholar]
- Minoguchi S, Taniguchi Y, Kato H, Okazaki T, Strobl LJ, Zimber-Strobl U, Bornkamm GW, Honjo T. RBP-L, a transcription factor related to RBP-Jkappa. Mol Cell Biol. 1997;17:2679–2687. [Europe PMC free article] [Abstract] [Google Scholar]
- Montarras D, Morgan J, Collins C, Relaix F, Zaffran S, Cumano A, Partridge T, Buckingham M. Direct isolation of satellite cells for skeletal muscle regeneration. Science. 2005;309:2064–2067. [Abstract] [Google Scholar]
- Moore KA, Lemischka IR. Stem cells and their niches. Science. 2006;311:1880–1885. [Abstract] [Google Scholar]
- Polesskaya A, Seale P, Rudnicki MA. Wnt signaling induces the myogenic specification of resident CD45+ adult stem cells during muscle regeneration. Cell. 2003;113:841–852. [Abstract] [Google Scholar]
- Qu Z, Balkir L, van Deutekom JC, Robbins PD, Pruchnic R, Huard J. Development of approaches to improve cell survival in myoblast transfer therapy. J Cell Biol. 1998;142:1257–1267. [Europe PMC free article] [Abstract] [Google Scholar]
- Rando TA, Blau HM. Primary mouse myoblast purification, characterization, and transplantation for cell-mediated gene therapy. J Cell Biol. 1994;125:1275–1287. [Europe PMC free article] [Abstract] [Google Scholar]
- Relaix F, Rocancourt D, Mansouri A, Buckingham M. A Pax3/Pax7-dependent population of skeletal muscle progenitor cells. Nature. 2005;435:948–953. [Abstract] [Google Scholar]
- Rhyu MS, Jan LY, Jan YN. Asymmetric distribution of numb protein during division of the sensory organ precursor cell confers distinct fates to daughter cells. Cell. 1994;76:477–491. [Abstract] [Google Scholar]
- Rosenblatt JD, Lunt AI, Parry DJ, Partridge TA. Culturing satellite cells from living single muscle fiber explants. In Vitro Cell Dev Biol. 1995;31:773–779. [Abstract] [Google Scholar]
- Schienda J, Engleka KA, Jun S, Hansen MS, Epstein JA, Tabin CJ, Kunkel LM, Kardon G. Somitic origin of limb muscle satellite and side population cells. Proc Natl Acad Sci USA. 2006;103:945–950. [Europe PMC free article] [Abstract] [Google Scholar]
- Schultz E. Satellite cell proliferative compartments in growing skeletal muscles. Dev Biol. 1996;175:84–94. [Abstract] [Google Scholar]
- Seale P, Sabourin LA, Girgis-Gabardo A, Mansouri A, Gruss P, Rudnicki MA. Pax7 is required for the specification of myogenic satellite cells. Cell. 2000;102:777–786. [Abstract] [Google Scholar]
- Shinin V, Gayraud-Morel B, Gomes D, Tajbakhsh S. Asymmetric division and cosegregation of template DNA strands in adult muscle satellite cells. Nat Cell Biol. 2006;8:677–687. [Abstract] [Google Scholar]
- Srinivas S, Watanabe T, Lin CS, William CM, Tanabe Y, Jessell TM, Costantini F. Cre reporter strains produced by targeted insertion of EYFP and ECFP into the ROSA26 locus. BMC Dev Biol. 2001;1:4. [Europe PMC free article] [Abstract] [Google Scholar]
- Tajbakhsh S, Rocancourt D, Buckingham M. Muscle progenitor cells failing to respond to positional cues adopt non-myogenic fates in myf-5 null mice. Nature. 1996;384:266–270. [Abstract] [Google Scholar]
- Tallquist MD, Weismann KE, Hellstrom M, Soriano P. Early myotome specification regulates PDGFA expression and axial skeleton development. Development. 2000;127:5059–5070. [Abstract] [Google Scholar]
- Venters SJ, Ordahl CP. Asymmetric cell divisions are concentrated in the dermomyotome dorsomedial lip during epaxial primary myotome morphogenesis. Anat Embryol (Berl) 2005;209:449–460. [Abstract] [Google Scholar]
- Yablonka-Reuveni Z, Anderson JE. Satellite cells from dystrophic (mdx) mice display accelerated differentiation in primary cultures and in isolated myofibers. Dev Dyn. 2006;235:203–212. [Abstract] [Google Scholar]
Full text links
Read article at publisher's site: https://doi.org/10.1016/j.cell.2007.03.044
Read article for free, from open access legal sources, via Unpaywall:
http://www.cell.com/article/S0092867407005132/pdf
Citations & impact
Impact metrics
Citations of article over time
Alternative metrics
Article citations
PTPN1/2 inhibition promotes muscle stem cell differentiation in Duchenne muscular dystrophy.
Life Sci Alliance, 8(1):e202402831, 30 Oct 2024
Cited by: 0 articles | PMID: 39477543 | PMCID: PMC11527974
Macrophages in the Context of Muscle Regeneration and Duchenne Muscular Dystrophy.
Int J Mol Sci, 25(19):10393, 27 Sep 2024
Cited by: 0 articles | PMID: 39408722 | PMCID: PMC11477283
Review Free full text in Europe PMC
Organoid culture promotes dedifferentiation of mouse myoblasts into stem cells capable of complete muscle regeneration.
Nat Biotechnol, 11 Sep 2024
Cited by: 2 articles | PMID: 39261590
Hotspots and trends in satellite cell research in muscle regeneration: A bibliometric visualization and analysis from 2010 to 2023.
Heliyon, 10(18):e37529, 05 Sep 2024
Cited by: 0 articles | PMID: 39309858 | PMCID: PMC11415684
Congenital myopathies: pathophysiological mechanisms and promising therapies.
J Transl Med, 22(1):815, 02 Sep 2024
Cited by: 0 articles | PMID: 39223631 | PMCID: PMC11370226
Review Free full text in Europe PMC
Go to all (825) article citations
Data
Data behind the article
This data has been text mined from the article, or deposited into data resources.
BioStudies: supplemental material and supporting data
Similar Articles
To arrive at the top five similar articles we use a word-weighted algorithm to compare words from the Title and Abstract of each citation.
The molecular regulation of muscle stem cell function.
Cold Spring Harb Symp Quant Biol, 73:323-331, 01 Jan 2008
Cited by: 154 articles | PMID: 19329572
Review
Embryonic founders of adult muscle stem cells are primed by the determination gene Mrf4.
Dev Biol, 381(1):241-255, 25 Apr 2013
Cited by: 29 articles | PMID: 23623977
MLL1 is required for PAX7 expression and satellite cell self-renewal in mice.
Nat Commun, 10(1):4256, 18 Sep 2019
Cited by: 21 articles | PMID: 31534153 | PMCID: PMC6751293
Myf5-positive satellite cells contribute to Pax7-dependent long-term maintenance of adult muscle stem cells.
Cell Stem Cell, 13(5):590-601, 08 Aug 2013
Cited by: 155 articles | PMID: 23933088 | PMCID: PMC4082715
Funding
Funders who supported this work.
NIAMS NIH HHS (2)
Grant ID: R01 AR044031-11
Grant ID: R01 AR044031