Abstract
Free full text

A molecular switch that controls cell spreading and retraction
Abstract
Integrin-dependent cell spreading and retraction are required for cell adhesion, migration, and proliferation, and thus are important in thrombosis, wound repair, immunity, and cancer development. It remains unknown how integrin outside-in signaling induces and controls these two opposite processes. This study reveals that calpain cleavage of integrin β3 at Tyr759 switches the functional outcome of integrin signaling from cell spreading to retraction. Expression of a calpain cleavage–resistant β3 mutant in Chinese hamster ovary cells causes defective clot retraction and RhoA-mediated retraction signaling but enhances cell spreading. Conversely, a calpain-cleaved form of β3 fails to mediate cell spreading, but inhibition of the RhoA signaling pathway corrects this defect. Importantly, the calpain-cleaved β3 fails to bind c-Src, which is required for integrin-induced cell spreading, and this requirement of β3-associated c-Src results from its inhibition of RhoA-dependent contractile signals. Thus, calpain cleavage of β3 at Tyr759 relieves c-Src–mediated RhoA inhibition, activating the RhoA pathway that confines cell spreading and causes cell retraction.
Introduction
Integrins are a family of heterodimeric cell adhesion receptors, which not only mediate cell adhesion to extracellular matrix proteins but also transmit signals that are vital for anchorage-dependent cell survival, proliferation, and motility (Hynes, 2002). Thus, integrins play pivotal roles in physiological processes such as wound healing, immune responses, and hemostasis. Aberrant integrin signaling is also centrally involved in the development of human diseases such as thrombosis, cancer, and autoimmune diseases and is the target of many therapeutic agents (Hynes, 2002; Shattil and Newman, 2004). Therefore, understanding the molecular events in the transduction of integrin signals is important in our understanding of many biological processes and has the potential to reveal novel intervention for diseases.
Integrin signaling is bidirectional in that ligand binding function of integrins requires conformational change in the extracellular ligand binding domain induced by intracellular signals (inside-out signaling), and ligand binding transduces outside-in signals that mediate cellular responses (Hynes, 2002; Vinogradova et al., 2002; Ginsberg et al., 2005; Wegener et al., 2007). An early functional outcome of integrin outside-in signaling is cell spreading, which is characterized by the formation of filopodia and lamellipodia and represents the outward movement of cell membrane, polymerizing actin, and cytoskeletal complexes at the leading edge (Hall, 2005). Integrin signaling later induces cell retraction, which is the inward movement of the cell membrane and cytoskeletal complexes, often at the rear end of cells. Coordinated spreading and retraction allows cell migration and in blood platelets facilitates stable platelet adhesion, thrombus formation, and consolidation. It has been shown that small GTP binding proteins Rac and cdc42 are involved in signaling mechanisms of cell spreading and that RhoA-mediated signaling is important in cell retraction (Hall, 2005). However, it remains unclear how integrin signaling initiates and temporally regulates these two seemingly opposing cellular responses; this is a fundamental question in cell biology. In this study, we have discovered that calpain cleavage of β3 at Tyr759 serves as a molecular switch that changes the outcome of the integrin outside-in signals from mediating cell spreading to promoting cell retraction. Furthermore, the switch from cell spreading to retraction is mediated by calpain cleavage of the c-Src binding site at the integrin C terminus, which relieves the c-Src–dependent inhibition of RhoA, and thus facilitates integrin-mediated, RhoA-dependent contractile signaling.
Results
A calpain cleavage–resistant β3 mutant
We have previously reported that the calcium-dependent protease calpain cleaves the cytoplasmic domain of the integrin β3 subunit mainly at Y759, thus removing the C-terminal RGT762 sequence (Du et al., 1995; Xi et al., 2003). Calpain cleavage of β3 is inhibited by tyrosine phosphorylation at Y759 of the β3 cytoplasmic domain (Xi et al., 2006). To understand the physiological role of calpain cleavage of β3, we developed a calpain-resistant mutant of β3 by replacing R760 with a negatively charged glutamic acid (R760E; Fig. 1 A). This mutation is intended to mimic the effect of phosphorylation at the adjacent Y759 by inhibiting calpain cleavage without perturbing the functionally critical NITY motif. The R760E mutant was stably expressed in a CHO cell line (Gu et al., 1999) together with the wild-type (WT) integrin αIIb subunit. To determine whether the R760E mutant confers resistance to calpain-mediated proteolysis, lysates from cells expressing WT integrin αIIbβ3 and the R760E mutant were incubated with or without purified μ-calpain and immunoblotted with Ab762, an antibody recognizing the intact β3 C-terminal TYRGT762 sequence, or Ab759, an antibody that recognizes the β3 TNITY759 sequence only when β3 is cleaved by calpain at Y759 (Du et al., 1995; Xi et al., 2003). The specificities of these antibodies have been previously characterized in Du et al. (1995) and Xi et al. (2003). In particular, we have shown that the calpain cleavage–specific antibody Ab759 only reacts with the β3 that is truncated at the C-terminal side of Y759 and fails to recognize β3 that is not cleaved (WT) or truncated at different sites, and that Ab762 reacts only with WT β3 that is not cleaved and fails to react with β3 cleaved at Y759 or other sites (Xi et al., 2003). MAb 15, recognizing the β3 extracellular domain (which is not disturbed by calpain; Du et al., 1995), serves as loading control (Fig. 1 B). WT β3 shows a large increase in reactivity with Ab759 after treatment with μ-calpain. This increase is mirrored by the loss of reactivity with Ab762, indicating loss of intact C terminus. In contrast, calpain- treated R760E mutant β3 showed no decrease in Ab762 reaction and dramatically diminished reactivity with Ab759. The relatively low reactivity of Ab762 with the R760E mutant compared with WT β3 is caused by R760 to E mutation but is not caused by reduced R760E expression, as indicated by the reaction with mAb 15. These results demonstrate that R760E mutation is calpain cleavage resistant (Fig. 1 B). Levels of stable surface expression of the R760E mutant and WT αIIbβ3 were also analyzed by flow cytometry to ensure comparable expression after repeated cell sorting with an anti-αIIbβ3 monoclonal antibody, D57 (Fig. 1 C). We showed previously that the β3 cytoplasmic domain is cleaved by endogenous calpain in platelets and integrin-expressing CHO cells adherent to fibrinogen (Xi et al., 2003). To test whether R760E is resistant to endogenous calpain during cell adhesion, CHO cells containing either WT cells or R760E mutant β3 were allowed to adhere and spread on immobilized fibrinogen and were subsequently immunolabeled with affinity-purified cleavage-specific antibody Ab759 to detect integrin cleavage and with mAb 15 to mark β3. WT β3 was cleaved by calpain (Fig. 1 D). In contrast, the R760E mutant showed marked reduction in calpain cleavage (Fig. 1 D). Thus, the R760E mutation renders β3 resistant to cleavage by calpain in cells adherent to fibrinogen.
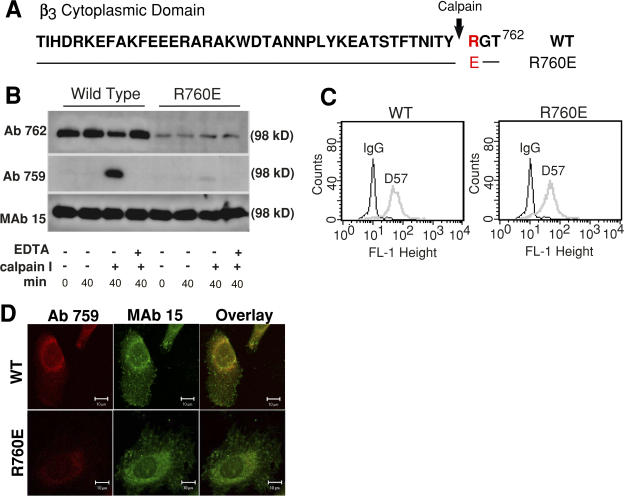
A calpain cleavage–resistant mutation of β3: R760E. (A) WT β3 cytoplasmic domain sequence and the R760E mutation. (B) Cells expressing WT and R760E mutant αIIbβ3 were solubilized, treated with or without purified μ-calpain, and immunoblotted with anti-β3 C-terminal antibody Ab762, calpain cleavage–indicator antibody Ab759, and the anti-β3 extracellular domain antibody mAb 15. Note that although R760E showed lower reactivity with Ab762 because of mutation in the epitope (TYR760GT), calpain caused minimal loss of Ab762 reactivity or gain of Ab759 reactivity, which indicates calpain resistance. (C) Analysis of stable αIIbβ3 expression in cells expressing WT and R760E mutant integrins, using the monoclonal anti-αIIbβ3 antibody D57. (D) WT and R760E cells spreading on fibrinogen were permeabilized and stained with mAb 15 (green) and purified Ab759 (red). Ab759 staining of R760E cells was reduced compared with WT β3. Bar, 10 μm.
The effects of calpain resistance on β3-mediated clot retraction and cell spreading
Fibrin clot retraction, mediated by platelets and β3 integrin– expressing cells, requires β3-dependent outside-in signaling and cell retraction (Chen et al., 1994; Law et al., 1996). To determine whether and how the calpain-resistant mutation of β3 affects integrin signaling, we compared the ability of the WT β3- and R760E-expressing cells to mediate clot retraction (Fig. 2, A and B). Clot retraction mediated by R760E cells was significantly decreased (P < 0.001). Consistent with this result, calpain cleaved WT β3 at Y759 during clot retraction, and this cleavage was impeded in the R760E mutant cells (Fig. 2 C). It was previously reported that calpain I knockout platelets showed reduced ability to mediate clot retraction (Azam et al., 2001). Similarly, a calpain inhibitor, MDL28170, also reduced clot retraction mediated by β3 integrin–expressing CHO cells (Fig. 2, D and E). However, the calpain substrate responsible for this effect of calpain was unclear. Thus, our data represent a new finding that calpain cleavage of β3 stimulates clot retraction. This finding is consistent with the data obtained in platelets. Although massive integrin cleavage induced by calcium ionophore or high concentrations of thrombin was not significantly affected in calpain I−/− platelets in a previous study (possibly because of the involvement of Calpain II; Azam et al., 2001; Pfaff et al., 1999), we show in Fig. 2 F that calpain cleavage of β3 at Y759 was considerably reduced in calpain I−/− platelets when the cleavage was induced by a lower concentration of thrombin (0.1 U/ml), which is consistent with the finding that the calpain I−/− mice were defective in clot retraction when clot retraction was stimulated with a low concentration of thrombin (Azam et al., 2001).
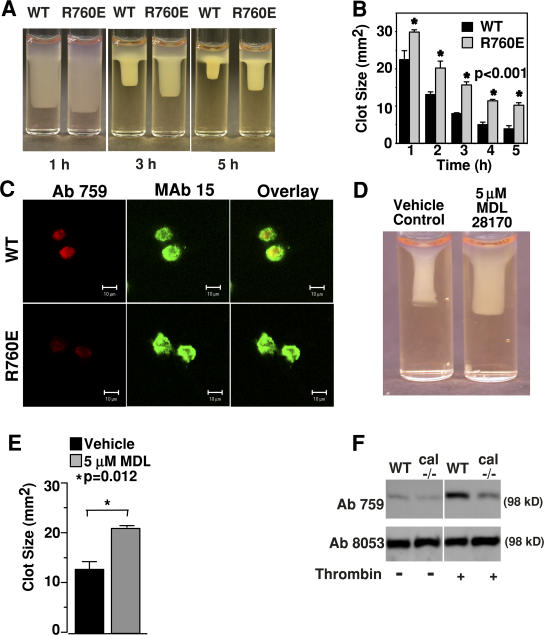
Effects of cleavage-resistant β3 mutation, R760E, and calpain inhibition on clot retraction and β3 cleavage. (A) Fibronectin-depleted plasma mixed with WT or R760E cells was induced to coagulate with 2 U/ml thrombin at 37°C for the indicated times, and then photographed. (B) Quantification of clot size at various time points in A. Error bars are the SD from three separate experiments. (C) Clots containing WT or R760E cells were fixed at 1 h, cryosectioned, stained with purified Ab759 (red) or mAb 15 (green), and observed by confocal microscopy. Note the reduced Ab759 staining of R760E cells. Bars, 10 μm. (D) Clot retraction by WT cells preincubated with either 5 μM of calpain inhibitor MDL 28170 or vehicle control. (E) Quantification of clot sizes in D (mean ± SD). (F) Washed platelets from WT and μ-calpain knockout mice were incubated in an aggregometer with or without 0.1 U/ml thrombin for 7 min. β3 cleavage at Y759, which was detected using cleavage-specific antibody Ab759, was enhanced in the WT compared with the calpain knockout. Ab8053 recognizes β3 extracellular domain and serves as a loading control.
To determine whether the calpain-resistant β3 mutant affects integrin-dependent cell spreading, cells expressing WT or R760E mutant αIIbβ3 were allowed to adhere to fibrinogen, and spreading kinetics of these cells were observed (Fig. 3, A and B). R760E mutation significantly enhanced and accelerated cell spreading compared with WT αIIbβ3 (P < 0.0001), indicating that calpain cleavage of β3 negatively regulates cell spreading. This result is consistent with the data that cells expressing a deletion mutant of β3 mimicking calpain cleavage at Y759 (Δ759 cells) are defective in integrin-dependent cell spreading (Fig. 3 E). Thus, calpain cleavage of β3 negatively regulates the integrin outside-in signals that promote cell spreading while it stimulates the integrin outside-in signaling that activates cellular retractile machinery.
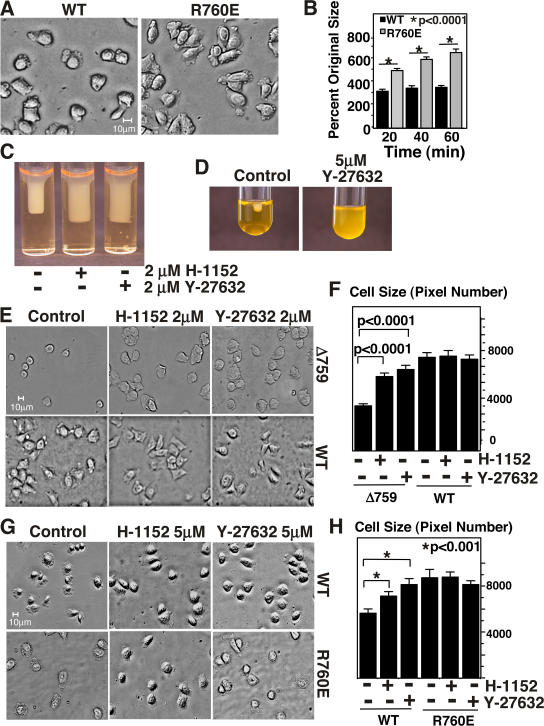
Effects of cleavage-resistant and -mimicking β3 mutations and ROCK inhibitors on cell spreading and clot retraction. (A) Indicated cell lines were incubated on fibrinogen-coated chamber slides at 37°C and photographed at various times. Images at 20 min are shown. (B) Sizes of spread cells were quantified as percentage of original sizes using Image J software. Histogram shows the mean ± SEM. (C and D) 5 μM each of ROCK inhibitors Y-27632 and H-1152 inhibited clot retraction in platelets (D) and in CHO cells expressing WT β3 (C). (E) Spreading of WT cells and cells expressing a calpain cleavage–mimicking β3 mutant (Δ759) on fibrinogen for 1 h. Defective spreading of Δ759 cells was corrected by 2 μM each of ROCK inhibitors H-1152 and Y-27632. (F) Quantification of cell spreading from cells in E, using image J software. Histogram shows the mean ± SEM. (G) 5 μM each of ROCK inhibitors H-1152 and Y-27632 enhance spreading in WT cells but not in cleavage-resistant R760E mutants at 20 min. (H) Quantification of cell spreading from cells in G, using image J software. Histogram shows the mean ± SEM.
The inhibitory effect of calpain cleavage of β3 on cell spreading is mediated by activation of RhoA-dependent cell retraction
The increase in clot retraction and inhibition of cell spreading by calpain cleavage of β3 can result either from cleavage-induced activation of cell retractile function or cleavage-induced inhibition of cell spreading signaling. To differentiate these two possibilities, we sought to inhibit the cell retractile signaling mechanisms. Previous studies demonstrate that stress fiber formation in cells adherent to integrin ligands requires activation of RhoA (Jaffe and Hall, 2003) and Rho-dependent kinases (ROCK), which stimulate myosin light chain phosphorylation (Amano et al., 1996; Kimura et al., 1996) and thus retractile function of the actomyosin complex (Giuliano et al., 1992). Indeed, the ROCK inhibitors Y-27632 or H-1152, when preincubated with WT β3-expressing CHO cells (Fig. 3 C) and platelets (Fig. 3 D), impeded clot retraction, indicating that the activation of the RhoA-ROCK signaling pathway is important in integrin-dependent cell retraction. To determine whether this pathway is responsible for calpain cleavage–dependent reversal of the direction of cell membrane movement, we studied the effects of ROCK inhibitors on cell spreading of WT β3-expressing or calpain cleavage– mimicking β3-expressing cells. The CHO cells expressing the calpain-cleaved form of β3 (Δ759) were defective in spreading on fibrinogen, and this defect was completely corrected by the ROCK inhibitors (Fig. 3 E). Spreading of CHO cells expressing WT αIIbβ3 was not significantly enhanced (P > 0.05) by low concentrations of ROCK inhibitors (Fig. 3, E and F). However, higher concentrations of either H-1152 or Y-27632 accelerate cell spreading in WT cells (Fig. 3, G and H). Interestingly, enhancement of spreading by cells expressing cleavage-resistant integrin mutant R760E was not observed even with higher-dose ROCK inhibition (Fig. 3, G and H), suggesting that the inhibitory effect of Rho-kinase signaling on cell spreading is already diminished when β3 is resistant to calpain cleavage. These results suggest that cleavage of β3 at Y759 caused activation of the RhoA-ROCK signaling pathway and cell retraction, thus preventing cell spreading.
Calpain cleavage of β3 at Y759 induces RhoA activation
RhoA is activated by GTP binding and inactivated when bound GTP is hydrolyzed to GDP (Jaffe and Hall, 2003). The Rho binding domain (RBD) of the RhoA effector protein rhotekin specifically binds GTP-bound RhoA and can therefore be used to indicate RhoA activation (Ren and Schwartz, 2000). To determine whether calpain cleavage of β3 stimulates RhoA activation, cells were allowed to spread on fibrinogen-coated surfaces, and then were fixed, permeabilized, and incubated with fluorescent GST-RBD fusion protein (GST-RBD-555). GST-RBD-555 strongly reacted with the WT integrin-expressing cells spreading on fibrinogen (Fig. 4). GST-RBD-555 binding was specific because it was competitively inhibited by unlabeled GST-RBD (Fig. 4 A) and by preincubation of cells with cell-permeable C3 transferase (Fig. 5) but was unaffected in the presence of 20 mM glutathione (Fig. 4 A). The specificity of GST-RBD-555 is further supported by its colocalization with the stain of a monoclonal antibody that recognizes RhoA (Fig. 4 B). Thus, by using this novel in situ RhoA activation assay, we show that RhoA is activated after WT integrin-mediated cell adhesion and spreading on fibrinogen. In contrast to WT β3, cells expressing the calpain-resistant β3 mutant R760E display reduced reactivity with GST-RBD-555, indicating that the resistance to calpain cleavage by R760E mutation and increased cell spreading in R760E cells are associated with inhibition of integrin-induced RhoA activation. Conversely, Δ759 cells, which are defective in spreading on fibrinogen, showed robust reactivity with GST-RBD-555, indicating that the calpain-cleaved form of β3 induces RhoA activation without requiring cell spreading. Collectively, these data demonstrate that cleavage of β3 at Y759 switches on the RhoA-ROCK signaling pathway, leading to cell retraction and inhibition of cell spreading.
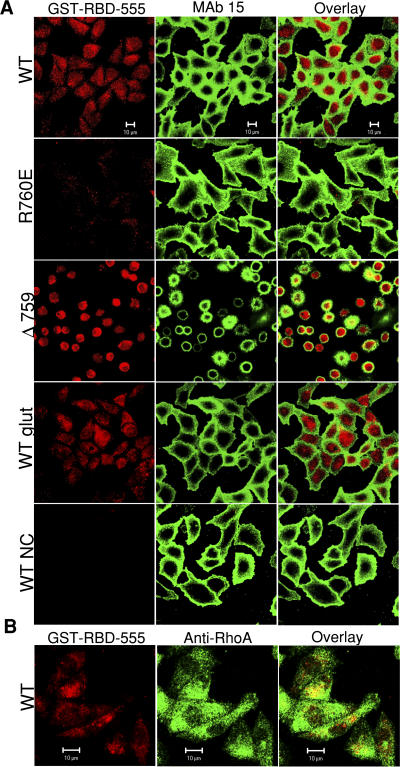
Calpain cleavage of β3 stimulates RhoA activation. (A) WT, R760E, and Δ759 cells spreading on fibrinogen for 1 h were fixed and allowed to react with Alexa Fluor 555–labeled rhotekin (GST-RBD-555 [red]) to indicate RhoA activation. To estimate nonspecific binding, fluorescent rhotekin binding was also performed in the presence of unlabeled rhotekin (NC) or 20 mM glutathione (glut). MAb 15 (green) serves as a marker for cell margins and integrin β3 expression. (B) Activated RhoA, indicated by GST-RBD-555 (red), colocalizes with anti-RhoA (green). Bars, 10 μm.
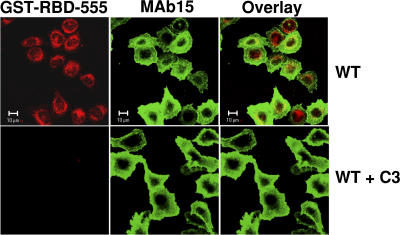
Inhibition of GST-RBD binding to active RhoA by cell-permeable C3 transferase. Cells expressing WT αIIbβ3 were preincubated with or without 5 μg/ml of cell-permeable C3 transferase and were allowed to spread on fibrinogen-coated surfaces for 1 h. Cells were stained with GST-RBD-555 (red) to detect active RhoA and with mAb 15 (green) to mark integrin surface expression. Bars, 10 μM.
Effects of calpain cleavage–resistant or –mimicking mutations on c-Src binding to β3
It has been reported that a member of the Src family of protein kinases (SFK), c-Src, interacts with the β3 cytoplasmic domain and that this interaction requires the YRGT762 sequence in the C terminus of β3 (Arias-Salgado et al., 2005a,b). To determine whether c-Src interaction with β3 is affected by the R760E mutation or calpain cleavage–mimicking truncation of β3, WT and mutant β3, stably expressed in CHO cells, were immunoprecipitated with Ab8053, an anti-β3 antibody, and precipitates were immunoblotted for β3-associated c-Src (Fig. 6). We found that c-Src coimmunoprecipitated with both WT and R760E mutant forms of β3 but failed to interact with the cleavage-mimicking mutant Δ759 (Fig. 6). These data are consistent with previous studies (Arias-Salgado et al., 2005a,b), indicating that cleavage of the β3 C-terminal RGT sequence disrupts c-Src binding to β3.
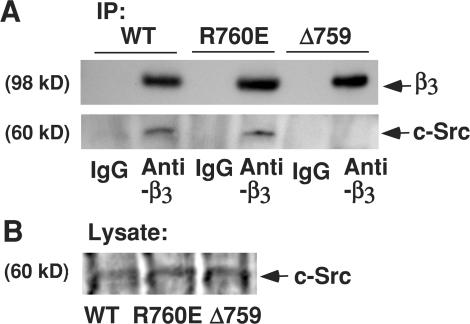
Coimmunoprecipitation of c-Src with WT β3 and β3 mutants. (A) β3 immunoprecipitates from CHO cells expressing WT, R760E mutant, and Δ759 mutant integrins were probed on Western blots with antibodies against β3 (mAb 15) or c-Src. c-Src binding to the integrin cytoplasmic domain is abolished in the calpain cleavage–mimicking mutant Δ759 but not in the calpain cleavage–resistant mutant R760E. (B) Lysates were also immunoblotted with antibody to c-Src.
Calpain cleavage of β3 at Y759 relieves the inhibitory effect of c-Src on RhoA activation and thus promotes cell retraction
It has been shown that c-Src plays an important role in cell spreading (Kaplan et al., 1995) and that c-Src interaction with β3 is important for this function (Arias-Salgado et al., 2003; Shattil, 2005). Therefore, we examined whether the loss of β3-associated c-Src function is responsible for the activation of cell retraction and inhibition of cell spreading induced by calpain cleavage at Y759. PP2, an SFK inhibitor, abolished WT β3-mediated cell spreading on fibrinogen (Fig. 7, A and D). The inhibitory effect of PP2 bears striking resemblance to the deficiency in cell spreading in Δ759 cells. More importantly, the inhibitory effect of PP2 was reversed by ROCK inhibitors H-1152 and Y-27632, indicating that the RhoA-ROCK–mediated retractile signaling is required for the inhibitory effect of this SFK inhibitor on cell spreading (Fig. 7, A and D), which is similar to the requirement for ROCK in β3 cleavage–mediated inhibition of cell spreading. To exclude the possible nonspecific effect of pharmacological inhibitors, WT β3-expressing cells were transfected with cDNA, encoding a dominant-negative mutant of c-Src (K295R/Y527F; Mukhopadhyay et al., 1995), and cotransfected with 1/6 quantity of GFP cDNA to indicate successful transfection. The control cells expressing transfected GFP exhibited normal cell spreading on fibrinogen (Fig. 7, B and E). In contrast, the cells transfected with the dominant-negative c-Src showed a defect in cell spreading similar to that caused by PP2 (Fig. 7, B and D). This inhibitory effect of the dominant-negative c-Src was reversed when cells were cotransfected with an equal concentration of dominant-negative RhoA (N19-RhoA; Fig. 7, B and D; Qiu et al., 1995). The data obtained with cDNA transfection is thus consistent with the data using inhibitors of SFK and ROCK, indicating that integrin-dependent cell spreading occurs independent of c-Src when RhoA-mediated retractile signaling is disabled. Because integrin-mediated c-Src activation inhibits RhoA but does not affect cdc42 and Rac functions (Arthur et al., 2000), our data indicate that c-Src promotes integrin-dependent cell spreading by its inhibition of RhoA-mediated retractile signals. Furthermore, the SFK inhibitor PP2 corrected the defective RhoA activation in calpain cleavage–resistant R760E cells, restoring RhoA activation and inhibiting the spreading of R760E cells (Fig. 7, C and F), which is similar to the phenotype observed in Δ759 cells expressing the calpain-cleaved form of β3. These results indicate that without calpain cleavage of β3, β3-dependent c-Src activity inhibits RhoA activation and thus promotes cell spreading. Conversely, inhibition of SFK with PP2 dramatically enhanced clot retraction (Fig. 7 G), further demonstrating that SFK inhibits cell retractile machinery. Therefore, cleavage of β3 at Y759 relieves the inhibitory effect of c-Src on RhoA activation, switches on RhoA-ROCK–dependent cell retraction, and thus changes the functional outcome of integrin signaling from cell spreading to cell retraction.
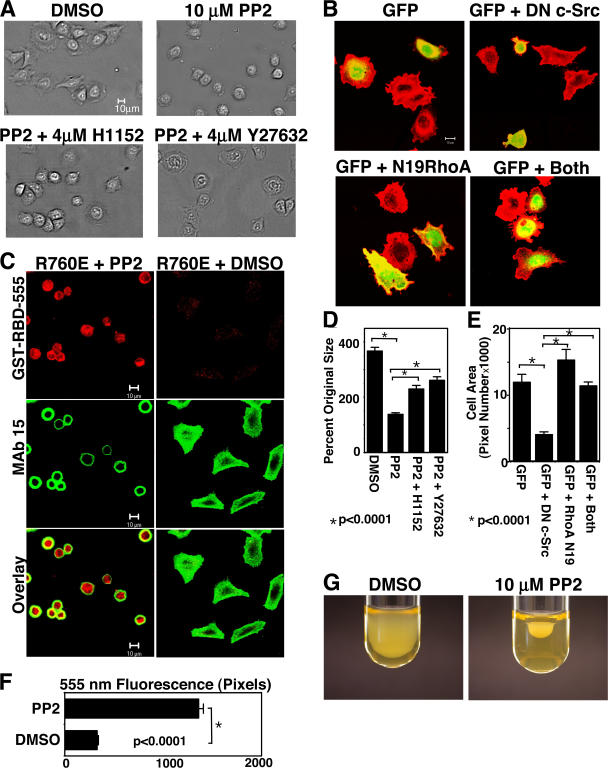
The effect of calpain cleavage of β3 on c-Src–dependent inhibition of RhoA-mediated cell retraction. (A) WT cells incubated with or without 10 μM PP2 or PP2 plus 4 μM of ROCK inhibitors H-1152 or Y-27632 were allowed to spread on fibrinogen for 1 h. Inhibition of cell spreading by PP2 is reversed by ROCK inhibitors. Bar, 10 μm. (B) Spreading of WT cells transfected with GFP alone, a dominant-negative c-Src (DN c-Src) plus GFP, N19-RhoA plus GFP, or N19-RhoA and dominant-negative c-Src plus GFP. Note that N19-RhoA reversed the inhibitory effect of dominant-negative c-Src. (C) R760E cells are allowed to adhere to fibrinogen in the presence of PP2 or vehicle control DMSO and are stained with GST-RBD-555 (red) and mAb 15 (green). Defective RhoA activation in R760E cells is corrected by PP2. Bars, 10 μM. (D) Quantitation of cell sizes in A using Image J (mean ± SEM from four random fields). (E) Quantitation of cell sizes in B using Image J (mean ± SEM from three experiments). (F) Stain of GST-RBD-555 in cells from C was quantified using LSM 5 software (mean ± SEM from three experiments). (G) 10 μM of SFK inhibitor PP2 accelerates clot retraction in platelets.
Cleavage of β3 at Y759 diminishes c-Src– dependent cell spreading by dissociating c-Src from β3 but not by inhibiting overall c-Src activation
Previous studies suggested that activity of c-Src associated with β3 is increased compared with that of c-Src that is not associated with β3 (Arias-Salgado et al., 2005a). To investigate the possibility that cleavage of β3 at Y759 regulates integrin-dependent overall c-Src activation in adherent cells, we measured the amount of active c-Src in CHO cells expressing WT and calpain cleavage–resistant (R760E) and –mimicking (Δ759) mutants of β3. All three cell lines were similarly capable of inducing considerable c-Src phosphorylation at Y416 (an indicator of c-Src activation) when allowed to adhere and spread onto fibrinogen-coated surfaces (Fig. 8 A), suggesting that deletion of the c-Src binding site in β3 did not affect the overall integrin-mediated c-Src activation. Furthermore, if c-Src activity alone was sufficient to mediate the inhibition of RhoA, constitutively active c-Src would be able to induce integrin-mediated cell spreading. Thus, Δ759 cells were transfected with cDNA encoding a constitutively active mutant of c-Src (E378G) and cotransfected with 1/6 quantity of GFP cDNA to indicate successful transfection. Increased levels of total c-Src and expression of constitutively active c-Src (Y416 phosphorylated) in E378G c-Src–transfected Δ759 cells in suspension were verified by Western blot (Fig. 8 B). The E378G c-Src–transfected Δ759 cells are not different from the GFP-expressing control Δ759 cells in that both spread poorly on fibrinogen (Fig. 8, C and D). These data suggest that the inhibitory effect of β3 cleavage on cell spreading is not because of its effect on overall c-Src activation but is associated with dissociation of c-Src (and therefore c-Src activity) from the β3 cytoplasmic domain. Thus, only the β3-associated c-Src activity is responsible for mediating RhoA inhibition and promoting cell spreading. Collectively, our results strongly suggest that cleavage of β3 at Y759 dissociates c-Src from the β3 C-terminal domain and thus relieves the inhibitory effect of β3-associated c-Src on RhoA activation, stimulating the RhoA-ROCK retractile signals, which switches the functional outcome of integrin signaling from mediating cell spreading to promoting cell retraction.
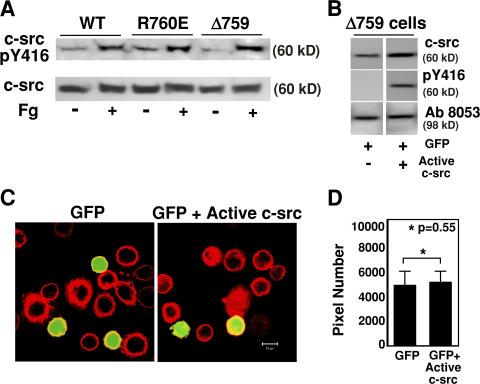
The effect of c-Src activation on the integrin-cleavage–dependent inhibition of cell spreading. (A) WT, R760E, and Δ759 cells were either in suspension or allowed to spread on fibrinogen for 30 min, and then solubilized and analyzed by Western blot with antibodies specific for c-Src or for Src phosphorylated at Y416 as an indicator of Src activation. Note that c-Src activation is similar in all three cell lines. (B) Δ759 cells were transfected with either GFP or GFP plus a constitutively active mutant of c-Src, E378G. Nonadherent transfected cells were solubilized and immunoblotted with antibodies against total c-Src, Y416-phosphorylated c-Src, and β3 (Ab8053, to verify equal loading). (C) Δ759 cells were transfected with GFP alone or GFP plus a constitutively active mutant of c-Src, E378G. The transfected cells were allowed to spread on fibrinogen-coated surfaces. Note that active c-Src cannot reverse the spreading defect in Δ759 cells. (D) Quantitation of cell sizes in C using Image J software (mean ± SEM from three experiments).
Discussion
In this study, we have discovered that β3 cleavage by calpain serves as a molecular switch that changes the outcome of integrin outside-in signaling from mediating cell spreading to promoting cell retraction. The switch in the outcome of integrin signaling from cell spreading to retraction is mediated by β3 cleavage–dependent activation of the RhoA retractile signaling pathway, and β3 cleavage–dependent activation of the RhoA signaling pathway is mediated by cleavage-dependent relief of the inhibitory effects of β3-associated c-Src on the RhoA signaling pathway. Thus, our study also reveals that the requirement of SFK in integrin-dependent cell spreading is caused by its inhibition of the RhoA-dependent retractile function of the cell. These new findings represent major conceptual advances in our understanding of how integrin signaling controls and regulates the direction of cell membrane movement.
The data supporting our conclusions in this study are mainly obtained using the reconstituted integrin signaling model in CHO cells expressing WT and calpain cleavage–resistant or –mimicking mutants of β3. Integrins play critical roles in all mammalian cells that adhere to extracellular matrix. Although different cell types express different members of the integrin family, integrin signaling, particularly outside-in signaling, is generally conserved in adherent cells, including CHO cells. In the past 20 yr, many important findings in β3 integrin function and signaling have been discovered using the CHO cell model expressing the WT and mutant β3 integrins, including the finding of the important role of talin in integrin activation (O'Toole et al., 1990; Calderwood et al., 1999; Tadokoro et al., 2003; Xi et al., 2003). Furthermore, the results obtained in CHO cells have been consistently verified in native β3-expressing cells, such as platelets (Li et al., 2001, 2003a, 2006; Tadokoro et al., 2003; Zou et al., 2007), indicating the validity of the CHO cell model. Importantly, the data obtained in CHO cell models in this study are consistent with the role of calpain I in clot retraction and in β3 cleavage as indicated in experiments using human and mouse platelets. Nevertheless, it is recognized that CHO cells are not totally identical to native β3-expressing cells such as platelets, and thus the results obtained with the CHO cell model will need to be further verified in platelets and other β3-expressing cells in the future.
We have previously shown that the cytoplasmic domain of β3 is a calpain substrate and that the cleavage occurs mainly at Y759, removing the C-terminal RGT sequence (Du et al., 1995; Xi et al., 2003). However, although calpain is activated after calcium elevation is induced by ligand binding to the integrin, very little cleavage of β3 occurs early during cell spreading because of the protection of integrin from calpain cleavage by tyrosine phosphorylation of the β3 cytoplasmic domain, especially at the periphery of a cell (Xi et al., 2006). In contrast, at a later time during cell spreading when Y759 of β3 becomes dephosphorylated, substantial β3 cleavage occurs (Xi et al., 2006). Also, calpain cleavage of β3 is readily detectable both during clot retraction in platelets and in the β3-expressing CHO cells. The kinetics of integrin cleavage are consistent with our data, indicating a role for calpain in the transition between cell spreading and cell retraction in both CHO cells and in platelets. In fact, the data obtained with cells expressing the calpain-resistant and calpain cleavage–mimicking mutants clearly reveal that cleavage of β3 at Y759 generates the change in integrin signaling from mediating cell spreading to promoting cell retraction. Thus, we have discovered a calpain-dependent molecular switch that is regulated temporally and topographically by tyrosine phosphorylation of β3 and that controls the direction of membrane and cytoskeleton movement induced by integrin outside-in signaling. It is important to note that the cleavage-mimicking mutant of β3 creates an experimental condition where 100% of β3 cytoplasmic domain is cleaved at Y759, which serves to clearly reveal the cleavage-induced changes in functional outcome. In platelets, >22% of β3 is cleaved at Y759 within an hour after platelet activation (Xi et al., 2003), which coincides with the occurrence of marked clot retraction. However, in more delicate situations, such as during cell spreading and migration, local and dynamic calpain activation signals are likely to cause localized cleavage of a small percentage of β3 molecules and thus dynamically control the local movement of membranes and cytoskeleton.
The β3 cleavage–mediated inhibition of cell spreading and promotion of cell retraction is not caused by its inhibition of cell spreading mechanisms but is mediated by the integrin cleavage-dependent activation of the RhoA retractile signaling pathway. Previous studies suggest that Rac and cdc42 play important roles in mediating cell spreading (filopodia and lamellipodia formation) and that RhoA is increasingly activated in spread cells (Hall, 2005). RhoA activates ROCK, which phosphorylates and inactivates myosin light chain phosphatase, and enhances myosin light chain phosphorylation and retractile function of the actomyosin complex (Giuliano et al., 1992; Amano et al., 1996; Kimura et al., 1996), inducing cell retraction. Cleavage of β3 at Y759 inhibits integrin-mediated cell spreading (Fig. 3; Xi et al., 2003). If the inhibitory effects of β3 cleavage on cell spreading should result from its inhibition of Rac- and cdc42-mediated cell spreading signaling, this inhibitory effect should not be corrected by inhibition of RhoA pathway. Therefore, our data that β3 cleavage–dependent inhibition of cell spreading is corrected by ROCK inhibitors indicate that calpain cleavage of β3 activates RhoA-dependent cell retraction, which subsequently inhibits the opposite movement of cell membrane resulting in inhibition of cell spreading.
Previous studies indicate that members of SFK are required for integrin-mediated signaling that leads to cell spreading (Shattil, 2005). The mechanism responsible for this role of SFK is not clear but is believed to be an early step in cell spreading signaling. However, we show that the inhibitory effect of an SFK inhibitor and a dominant-negative c-Src mutant on β3-mediated cell spreading is reversed by inhibition of the RhoA signaling pathway, indicating that cell spreading is independent of c-Src when RhoA signaling is abrogated. These data therefore provide the first direct evidence that the role of SFK in promoting integrin-dependent cell spreading is not that it is required for cdc42- and Rac-dependent cell spreading signaling, but rather is its inhibition of RhoA-dependent retractile signaling and thus cell retraction, which confines the outward movement of the cell membrane and cytoskeleton. Our data are consistent with the previous studies, which show that SFK inhibits RhoA activity but has no effect on the activity of cdc42 and Rac (Arthur et al., 2000). Our data are also consistent with the finding that RhoA inactivation by p190RhoGAP promotes membrane protrusion and polarity (Arthur and Burridge, 2001). More importantly, we show that cleavage of β3 at Y759 abolishes c-Src binding to β3 but does not significantly affect overall c-Src activation in cells spread on fibrinogen, and that the RhoA-dependent inhibition of cell spreading by β3 cleavage at Y759 is not reversed by expression of a constitutively active Src. These data suggest that whereas overall intracellular c-Src activation during cell adhesion does not require c-Src association with β3, active c-Src molecules dissociated from β3 are not capable of inhibiting the RhoA signaling pathway and promoting cell spreading on β3 ligands, possibly because of the lack of β3 scaffold. However, the calpain cleavage–resistant mutation in β3 inhibits RhoA activation in a Src-dependent manner. Thus, our results suggest that only the β3-associated c-Src is responsible for the inhibition of the RhoA pathway and facilitation of cell spreading. Conversely, our results suggest that calpain cleavage of β3, which abolishes Src binding to β3 (Fig. 6; Arias-Salgado et al., 2005b), relieves the inhibitory effects of β3-associated c-Src on the RhoA pathway, activates RhoA, and thus switches the outcome of integrin signaling from cell spreading to retraction.
Interestingly, β3 cleavage–dependent relief of c-Src– mediated RhoA inhibition and activation of RhoA-dependent cell retraction is associated with the cleavage of only a fraction of β3 molecules (Fig. 2; Xi et al., 2003) and cannot be prevented by the presence of uncleaved β3 molecules in the same cell. This apparently dominant effect of β3 cleavage suggests that c-Src activity at one location in a cell does not cross-inhibit RhoA activation induced by calpain cleavage of β3 at a different location and is consistent with the knowledge that spreading (such as filopodia and lamellipodia) and retraction can occur simultaneously at different locations within a cell (such as during cell migration). The requirement of c-Src association with β3 for the c-Src–mediated RhoA inhibition provides a mechanism for the localized role of c-Src in promoting cell spreading. Further studies are required to understand how β3-associated c-Src activity locally regulates RhoA and whether additional mechanisms are needed for RhoA activation when β3 cleavage by calpain relieves the inhibitory effect of β3-associated c-Src.
Based on data from this and other studies, we propose a new model of integrin signaling that incorporates a phosphorylation- and protease-mediated mechanism regulating integrin-mediated cell membrane movements. In this model (Fig. 9), initial integrin signaling activates protein tyrosine kinases, which induce phosphorylation of the cytoplasmic domain of β3 (Law et al., 1996, 1999), and elevates intracellular calcium levels, which activate calpain (Fox et al., 1993). Tyrosine phosphorylation at Y759 of β3 in the early phase of cell spreading protects the c-Src binding site in the C-terminal domain of β3 from calpain cleavage (Xi et al., 2006). The interaction of c-Src with β3 promotes activation of β3-associated c-Src by a Csk- (Obergfell et al., 2002) and protein tyrosine phosphatase IB–dependent mechanism (Arias-Salgado et al., 2005a) and/or allows c-Src–dependent signaling in the vicinity of the β3 C terminus, which facilitates cell spreading by inhibiting RhoA-dependent cell retraction. In spread cells, Y759 of β3 becomes dephosphorylated (Xi et al., 2006), allowing β3 cleavage by activated calpain, which removes the C-terminal RGT sequence of β3 important in c-Src binding. Integrin cleavage by calpain thus relieves the local inhibitory effect of β3-associated c-Src on the RhoA-ROCK signaling pathway and locally activates RhoA-dependent cell retractile signals (Xi et al., 2003; Arias-Salgado et al., 2005b). Thus, calpain cleavage of β3 switches the direction of integrin outside-in signaling from mediating spreading to promoting cell retraction.
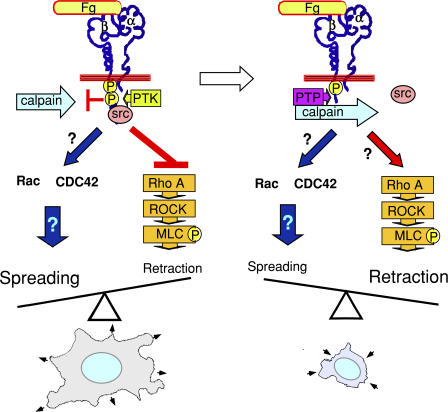
A new calpain-dependent switch that controls cell spreading and retraction. This schematic illustrates the calpain-dependent switch that controls the direction of integrin signaling. In this model, ligand binding to integrins induces not only Rac- and cdc42-dependent cell spreading signals, but also phosphorylation of the cytoplasmic domain of β3 and elevation of intracellular calcium levels that activate calpain. Tyrosine phosphorylation at Y759 of β3 protects the c-Src binding site in the C-terminal domain of β3 from calpain cleavage. The β3-associated c-Src facilitates cell spreading by inhibiting RhoA-dependent cell retraction signals. In spread cells, Y759 of β3 becomes dephosphorylated, allowing β3 cleavage by calpain. Removal of the c-Src binding site in β3 by calpain relieves the local inhibitory effect of c-Src on the RhoA-ROCK signaling pathway, activates RhoA-dependent cell retraction, and thus switches the outcome of local integrin signaling from mediating cell spreading to retraction. However, the possible involvement of additional pathways is not totally excluded.
Materials and methods
Reagents
Plasma containing 0.38% sodium citrate and 40 μg/ml aprotinin (Sigma-Aldrich) was depleted of fibronectin as previously reported in Chen et al. (1994). Ab762 and Ab759 were previously described in Du et al. (1995) and Xi et al. (2003). mAb 15, D57, and Ab8053 were provided by M. Ginsberg (University of California, San Diego, La Jolla, CA; O'Toole et al., 1990). Phosphospecific anti-Src Y416 antibody was obtained from Cell Signaling; anti-RhoA and cell permeable C3 transferase were obtained from Cytoskeleton, Inc.; Sudan black was obtained from Sigma-Aldrich; Alexa Fluor 594–conjugated goat anti–rabbit IgG and Alexa Fluor 488– conjugated goat anti–mouse IgG were obtained from Invitrogen; anti–v-Src monoclonal antibody, SFK inhibitor PP2, ROCK inhibitors H-1152 and Y-27632, and calpain inhibitor MDL 28170 were obtained from Calbiochem; and an anti–c-Src polyclonal antibody was obtained from Santa Cruz Biotechnology, Inc. GST-RBD cDNA were a gift from T. Kozasa (University of Illinois, Chicago, IL). GST-RBD fusion protein was purified as previously described in Ren and Schwartz (2000). Purified protein was fluorescently labeled with an Alexa Fluor 555 microscale protein labeling kit (Invitrogen).
Construction of R760E mutant
The β3 R760E mutation was performed using PCR. The forward primer has the sequence of AGAGCTTAAGGACAC. The reverse primers (CTCATTAAGTCCCCTCGTAGGTGATATTGG) contain an XhoI digestion site, stop codon, and the 18-nucleotide C-terminal β3 sequence coding the intended R760E mutation. PCR products were digested with AspI and XhoI and ligated into a β3 cDNA construct in a modified cDM8 vector containing only one XhoI site at the 3′ end of the multiple cloning site. The construct was verified by DNA sequencing.
Cell lines and transfection
CHO cells stably expressing WT human αIIbβ3 (2b3a), human glycoprotein Ib-IX (GPIb-IX; 1b9), or both αIIbβ3 and GPIb-IX (123) were previously described in Gu et al. (1999) and Xi et al. (2003). CHO cells expressing GPIb-IX and a truncation mutant β3, mimicking calpain cleavage at Y759, and complexed with WT αIIb (Δ759) were also described previously in Gu et al. (1999) and Xi et al. (2003). DNA transfection was performed using Lipofectamine 2000 (Invitrogen). R760E β3 mutant cDNA was cotransfected with WT αIIb and pcDNA 3.1/Hyg plasmid at a ratio of 5:5:1 into 1b9 cells. Complex formation between αIIb and β3 subunits was verified by flow cytometry using antibody D57 against αIIbβ3 complex. Stable R760E mutant cell lines were obtained by antibiotic selection and repeated cell sorting using D57 as previously described (Gu et al., 1999). To ensure comparable expression, R760E and Δ759 cells were sorted using the expression level of the stable WT αIIbβ3-expressing (123) cells as a gate (Gu et al., 1999; Xi et al., 2003). For transient transfections, cells stably coexpressing WT αIIbβ3 were transfected with either 2 μg GFP, 2 μg GFP plus 12 μg of dominant-negative c-Src (Mukhopadhyay et al., 1995), 2 μg GFP plus 12 μg of dominant-negative N19-RhoA (Qiu et al., 1995), or 2 μg GFP plus 12 μg of both dominant-negative c-Src and N19-RhoA, using Lipofectamine 2000. In some experiments, cells were transiently transfected with either 2 μg GFP or 2 μg GFP plus 12 μg of constitutively active c-Src (E378G). Cells were analyzed 24 h after transfection.
Calpain cleavage of β3 in CHO cells and mouse platelets
107 CHO cells were directly solubilized in buffer containing 2% Triton X-100, 0.15 M NaCl, 1 mM CaCl2, and 0.02 M Tris, pH 7.4, in the presence or absence of 10 mM EDTA. Cell lysates were incubated for 40 min at 37°C with or without 1 μg of purified μ-calpain. SDS-PAGE sample buffer containing 10 mM EDTA, 1 mM PMSF, and 0.2 mM E64 was added to stop reactions. For experiments using mice, 6–8-wk-old mice of either sex were anesthetized by intraperitoneal injection of pentobarbital and blood was drawn from the inferior vena cava. Blood from 5–6 (calpain I+/+ and calpain I−/−; Azam et al., 2001) mice was pooled or platelets were isolated by differential centrifugation as described in Li et al. (2003b). 3 × 108/ml washed platelets were resuspended in modified Tyrode's buffer (Du et al., 1991) and incubated at 22°C for 2 h before use. Platelets were stimulated with 0.1 U/ml thrombin in a platelet aggregometer at 37° for 7 min, and then solubilized in SDS-PAGE sample buffer. All samples were analyzed by SDS-PAGE using 4–15% gradient gels and were immunoblotted using various antibodies. Enhanced chemiluminescence (GE Healthcare) was used for visualization of antibody reactions.
Immunofluorescence and confocal microscopy
Chamber slides (Lab-Tek; Nunc) were precoated with 10 μg/ml fibrinogen and blocked with 5% BSA in PBS. 50 μl CHO cells (expressing WT or mutant integrins; 5 × 105/ml) in Tyrode's buffer was added to the wells and incubated at 37°C for 1 h. After washing, cells were fixed with 4% paraformaldehyde and permeabilized with 0.1% Triton X-100, 0.1 M Tris, 10 mM EGTA, 0.15 M NaCl, 5 mM MgCl2, 1 mM PMSF, 0.1 mM E64, and 1% BSA (permeabilization buffer, pH 7.5). Samples were blocked with 5% BSA, incubated with 10 μg/ml mAb 15 and 0.5 μg/ml of purified Ab759, and, after washing, stained with fluorescently labeled secondary antibodies. To detect RhoA activation, cells were fixed in 4% paraformaldehyde in PBS with 10 mM MgCl2 and incubated with 0.7 μg/ml mAb 15 and Alexa Fluor 555–labeled GST-RBD in permeabilization buffer containing 10 mM MgCl2. After further staining with Alexa Fluor 488–conjugated goat anti–mouse IgG and washes, the antibody and GST-RBD staining were detected using a confocal microscope (Carl Zeiss MicroImaging, Inc.). In some experiments, cells were also preincubated with or without 5 μg/ml of cell-permeable C3 transferase to inhibit RhoA, according to the manufacturer's instructions, and then stained with GST-RBD-555. Surface area in spreading cells was estimated by measuring total pixel number per cell in digitally recorded images using Image J software (National Institutes of Health). Quantitation of 555-nm fluorescence in R760E cells was determined by using the area tool in LSM 5 software (Carl Zeiss MicroImaging, Inc.) and expressed as total pixel number. Statistical significance was determined using a t test.
Clot retraction
400 μl of indicated CHO cell lines (4 × 106/ml), in complete DME in the presence or absence of 5 μM ROCK inhibitors H-1152 or Y-27632 or calpain inhibitor MDL 28170, was mixed with 100 μl of plasma, 2 U/ml thrombin, and 5 mM CaCl2 in a partially Sigmacote-treated glass cuvette (Chrono-Log). For platelet samples, citrated platelet-rich plasma was mixed with 0.2 U/ml thrombin. The clots were allowed to retract at 37°C and were photographed at various times. The two-dimensional sizes of retracted clots on photographs were quantified using Image J software and were expressed as clot size. Statistical significance was determined using a t test.
Confocal microscopy analysis of clot sections
Clots were fixed with 4% paraformaldehyde, embedded in 50% Tissue-Tek OCT compound (Sakura Finetek), placed in 25 × 20 × 5-mm Tissue-Tek Cryomolds (Sakura Finetek), and snap frozen in liquid nitrogen. Frozen sections were made using a cryostat microtome (Microm HM 505 E; Carl Zeiss MicroImaging, Inc.). Slides were then washed and quenched with 5% Sudan black (wt/vol) in 70% ethanol. Sections were permeabilized with 0.1% Triton X-100, 0.1 M Tris, 10 mM EGTA, 0.15 M NaCl, 5 mM MgCl2, 1 mM PMSF, 0.1 mM E64, and 1% BSA, pH 7.5 (permeabilization buffer), blocked with 5% BSA, and immunolabeled with 10 μg/ml mAb 15 and 0.5 μg/ml of purified Ab 759. After washing, cells were stained with Alexa Fluor 594–conjugated anti–rabbit IgG and 488–conjugated anti–mouse IgG. Data was collected using a confocal microscope.
Spreading assays
CHO cells expressing WT, R760E mutant, and Δ759 mutant integrins (100 μl/well, 1.5 × 105/ml) in complete DME were incubated in fibrinogen- coated chamber slides at 37°C for 20, 40, and 60 min. At each time, cells were immediately rinsed and fixed with 4% paraformaldehyde. For assays using PP2 and ROCK inhibitors, cells were preincubated at 22°C for 30 min with or without 10 μM PP2 and/or 4 μM H-1152 or Y-27632. Cells were allowed to spread for 1 h at 37°C, rinsed, and fixed with 4% paraformaldehyde. Images were captured using a microscope (DM IRB; Leica). The size of the cells from four random fields for each time and cell line was determined using Image J software. Relative increase in cell size was calculated by dividing the mean size of spread cells by the mean size of suspended cells from each cell line. Statistical significance was determined using a t test.
Immunoprecipitation
Cells were lysed in lysis buffer containing 1% NP-40, 150 mM NaCl, 50 mM Tris, pH 7.4, 1 mM sodium orthovanadate, 1 mM NaF, and Complete protease inhibitor mixture (Roche Molecular Biochemicals). Lysates were incubated with either rabbit anti-β3 or an equal amount of rabbit IgG and subsequently with protein A–Sepharose (GE Healthcare). Beads were washed four times with lysis buffer, analyzed by SDS-PAGE using 4–15% gradient gels, and immunoblotted with mAb 15 for β3 and monoclonal anti-Src (Calbiochem).
Immunoblot analysis of c-Src activation in cells adherent on fibrinogen-coated surfaces
10-cm polystyrene dishes (Fisher Scientific) were coated with 10 μg/ml fibrinogen and blocked with 5% BSA in PBS. Equal suspensions containing 5 × 106 CHO cells stably expressing WT, R760E mutant, or Δ759 mutant integrins in modified Tyrode's buffer were either kept in suspension or added to the dishes and incubated at 37°C for 30 min. Plates were rinsed three times with PBS and cells were subsequently lysed with the buffer containing 1% NP-40, 150 mM NaCl, 50 mM Tris, pH 7.4, 1 mM sodium orthovanadate, 1 mM NaF, and Complete protease inhibitor mixture. Lysates were analyzed by SDS-PAGE using 4–15% gradient gels, and then immunoblotted with anti–phospho-Src Y416 and anti–c-Src.
Acknowledgments
We thank Dr. Sanford Shattil for critical reading of the manuscript, Dr. Tohru Kozasa and Nicole Hajieck for helping with the Rho activation experiments, and Dr. Michael Burleigh for helping with histochemistry.
This work is supported by grants from the National Institutes of Health/National Heart, Lung, and Blood Institute (HL080264, HL062350, and HL068819; to X. Du).
Notes
Abbreviations used in this paper: RBD, Rho binding domain; ROCK, Rho-dependent kinase; SFK, Src family kinase; WT, wild type.
References
- Amano, M., M. Ito, K. Kimura, Y. Fukata, K. Chihara, T. Nakano, Y. Matsuura, and K. Kaibuchi. 1996. Phosphorylation and activation of myosin by Rho-associated kinase (Rho-kinase). J. Biol. Chem. 271:20246–20249. [Abstract] [Google Scholar]
- Arias-Salgado, E.G., S. Lizano, S. Sarkar, J.S. Brugge, M.H. Ginsberg, and S.J. Shattil. 2003. Src kinase activation by direct interaction with the integrin beta cytoplasmic domain. Proc. Natl. Acad. Sci. USA. 100:13298–13302. [Europe PMC free article] [Abstract] [Google Scholar]
- Arias-Salgado, E.G., F. Haj, C. Dubois, B. Moran, A. Kasirer-Friede, B.C. Furie, B. Furie, B.G. Neel, and S.J. Shattil. 2005. a. PTP-1B is an essential positive regulator of platelet integrin signaling. J. Cell Biol. 170:837–845. [Europe PMC free article] [Abstract] [Google Scholar]
- Arias-Salgado, E.G., S. Lizano, S.J. Shattil, and M.H. Ginsberg. 2005. b. Specification of the direction of adhesive signaling by the integrin beta cytoplasmic domain. J. Biol. Chem. 280:29699–29707. [Abstract] [Google Scholar]
- Arthur, W.T., and K. Burridge. 2001. RhoA inactivation by p190RhoGAP regulates cell spreading and migration by promoting membrane protrusion and polarity. Mol. Biol. Cell. 12:2711–2720. [Europe PMC free article] [Abstract] [Google Scholar]
- Arthur, W.T., L.A. Petch, and K. Burridge. 2000. Integrin engagement suppresses RhoA activity via a c-Src-dependent mechanism. Curr. Biol. 10:719–722. [Abstract] [Google Scholar]
- Azam, M., S.S. Andrabi, K.E. Sahr, L. Kamath, A. Kuliopulos, and A.H. Chishti. 2001. Disruption of the mouse mu-calpain gene reveals an essential role in platelet function. Mol. Cell. Biol. 21:2213–2220. [Europe PMC free article] [Abstract] [Google Scholar]
- Calderwood, D.A., R. Zent, R. Grant, D.J. Rees, R.O. Hynes, and M.H. Ginsberg. 1999. The Talin head domain binds to integrin beta subunit cytoplasmic tails and regulates integrin activation. J. Biol. Chem. 274:28071–28074. [Abstract] [Google Scholar]
- Chen, Y.P., T.E. O'Toole, J. Ylanne, J.P. Rosa, and M.H. Ginsberg. 1994. A point mutation in the integrin beta 3 cytoplasmic domain (S752→P) impairs bidirectional signaling through alpha IIb beta 3 (platelet glycoprotein IIb-IIIa). Blood. 84:1857–1865. [Abstract] [Google Scholar]
- Du, X.P., E.F. Plow, A.L.I. Frelinger, T.E. O'Toole, J.C. Loftus, and M.H. Ginsberg. 1991. Ligands “activate” integrin αIIbβ3 (platelet GPIIb-IIIa). Cell. 65:409–416. [Abstract] [Google Scholar]
- Du, X., T.C. Saido, S. Tsubuki, F.E. Indig, M.J. Williams, and M.H. Ginsberg. 1995. Calpain cleavage of the cytoplasmic domain of the integrin β3 subunit. J. Biol. Chem. 270:26146–26151. [Abstract] [Google Scholar]
- Fox, J.E., R.G. Taylor, M. Taffarel, J.K. Boyles, and D.E. Goll. 1993. Evidence that activation of platelet calpain is induced as a consequence of binding of adhesive ligand to the integrin, glycoprotein IIb-IIIa. J. Cell Biol. 120:1501–1507. [Europe PMC free article] [Abstract] [Google Scholar]
- Ginsberg, M.H., A. Partridge, and S.J. Shattil. 2005. Integrin regulation. Curr. Opin. Cell Biol. 17:509–516. [Abstract] [Google Scholar]
- Giuliano, K.A., J. Kolega, R.L. DeBiasio, and D.L. Taylor. 1992. Myosin II phosphorylation and the dynamics of stress fibers in serum-deprived and stimulated fibroblasts. Mol. Biol. Cell. 3:1037–1048. [Europe PMC free article] [Abstract] [Google Scholar]
- Gu, M., X. Xi, G.D. Englund, M.C. Berndt, and X. Du. 1999. Analysis of the roles of 14-3-3 in the platelet glycoprotein Ib-IX–mediated activation of integrin αIIbβ3 using a reconstituted mammalian cell expression model. J. Cell Biol. 147:1085–1096. [Europe PMC free article] [Abstract] [Google Scholar]
- Hall, A. 2005. Rho GTPases and the control of cell behaviour. Biochem. Soc. Trans. 33:891–895. [Abstract] [Google Scholar]
- Hynes, R.O. 2002. Integrins: bidirectional, allosteric signaling machines. Cell. 110:673–687. [Abstract] [Google Scholar]
- Jaffe, A.B., and A. Hall. 2003. Cell biology. Smurfing at the leading edge. Science. 302:1690–1691. [Abstract] [Google Scholar]
- Kaplan, K.B., J.R. Swedlow, D.O. Morgan, and H.E. Varmus. 1995. c-Src enhances the spreading of src−/− fibroblasts on fibronectin by a kinase-independent mechanism. Genes Dev. 9:1505–1517. [Abstract] [Google Scholar]
- Kimura, K., M. Ito, M. Amano, K. Chihara, Y. Fukata, M. Nakafuku, B. Yamamori, J. Feng, T. Nakano, K. Okawa, et al. 1996. Regulation of myosin phosphatase by Rho and Rho-associated kinase (Rho-kinase). Science. 273:245–248. [Abstract] [Google Scholar]
- Law, D.A., A.L. Nannizzi, and D.R. Phillips. 1996. Outside-in integrin signal transduction. αIIbβ3-(GPIIb-IIIa) tyrosine phosphorylation induced by platelet aggregation. J. Biol. Chem. 271:10811–10815. [Abstract] [Google Scholar]
- Law, D.A., F.R. DeGuzman, P. Heiser, K. Ministri-Madrid, N. Killeen, and D.R. Phillips. 1999. Integrin cytoplasmic tyrosine motif is required for outside-in alphaIIbbeta3 signalling and platelet function. Nature. 401:808–811. [Abstract] [Google Scholar]
- Li, Z., X. Xi, and X. Du. 2001. A mitogen-activated protein kinase-dependent signaling pathway in the activation of platelet integrin αIIbβ3. J. Biol. Chem. 276:42226–42232. [Abstract] [Google Scholar]
- Li, Z., X. Xi, M. Gu, R. Feil, R.D. Ye, M. Eigenthaler, F. Hofmann, and X. Du. 2003. a. A stimulatory role for cGMP-dependent protein kinase in platelet activation. Cell. 112:77–86. [Abstract] [Google Scholar]
- Li, Z., G. Zhang, G.C. Le Breton, X. Gao, A.B. Malik, and X. Du. 2003. b. Two waves of platelet secretion induced by thromboxane A2 receptor and a critical role for phosphoinositide 3-kinases. J. Biol. Chem. 278:30725–30731. [Abstract] [Google Scholar]
- Li, Z., G. Zhang, R. Feil, J. Han, and X. Du. 2006. Sequential activation of p38 and ERK pathways by cGMP-dependent protein kinase leading to activation of the platelet integrin {alpha}IIb{beta}3. Blood. 107:965–972. [Abstract] [Google Scholar]
- Mukhopadhyay, D., L. Tsiokas, X.M. Zhou, D. Foster, J.S. Brugge, and V.P. Sukhatme. 1995. Hypoxic induction of human vascular endothelial growth factor expression through c-Src activation. Nature. 375:577–581. [Abstract] [Google Scholar]
- O'Toole, T.E., J.C. Loftus, X.P. Du, A.A. Glass, Z.M. Ruggeri, S.J. Shattil, E.F. Plow, and M.H. Ginsberg. 1990. Affinity modulation of the alpha IIb beta 3 integrin (platelet GPIIb-IIIa) is an intrinsic property of the receptor. Cell Regul. 1:883–893. [Europe PMC free article] [Abstract] [Google Scholar]
- Obergfell, A., K. Eto, A. Mocsai, C. Buensuceso, S.L. Moores, J.S. Brugge, C.A. Lowell, and S.J. Shattil. 2002. Coordinate interactions of Csk, Src, and Syk kinases with αIIbβ3 initiate integrin signaling to the cytoskeleton. J. Cell Biol. 157:265–275. [Europe PMC free article] [Abstract] [Google Scholar]
- Pfaff, M., X. Du, and M.H. Ginsberg. 1999. Calpain cleavage of integrin beta cytoplasmic domains. FEBS Lett. 460:17–22. [Abstract] [Google Scholar]
- Qiu, R.G., J. Chen, F. McCormick, and M. Symons. 1995. A role for Rho in Ras transformation. Proc. Natl. Acad. Sci. USA. 92:11781–11785. [Europe PMC free article] [Abstract] [Google Scholar]
- Ren, X.D., and M.A. Schwartz. 2000. Determination of GTP loading on Rho. Methods Enzymol. 325:264–272. [Abstract] [Google Scholar]
- Shattil, S.J. 2005. Integrins and Src: dynamic duo of adhesion signaling. Trends Cell Biol. 15:399–403. [Abstract] [Google Scholar]
- Shattil, S.J., and P.J. Newman. 2004. Integrins: dynamic scaffolds for adhesion and signaling in platelets. Blood. 104:1606–1615. [Abstract] [Google Scholar]
- Tadokoro, S., S.J. Shattil, K. Eto, V. Tai, R.C. Liddington, J.M. de Pereda, M.H. Ginsberg, and D.A. Calderwood. 2003. Talin binding to integrin beta tails: a final common step in integrin activation. Science. 302:103–106. [Abstract] [Google Scholar]
- Vinogradova, O., A. Velyvis, A. Velyviene, B. Hu, T. Haas, E. Plow, and J. Qin. 2002. A structural mechanism of integrin alpha(IIb)beta(3) “inside-out” activation as regulated by its cytoplasmic face. Cell. 110:587–597. [Abstract] [Google Scholar]
- Wegener, K.L., A.W. Partridge, J. Han, A.R. Pickford, R.C. Liddington, M.H. Ginsberg, and I.D. Campbell. 2007. Structural basis of integrin activation by talin. Cell. 128:171–182. [Abstract] [Google Scholar]
- Xi, X., R.J. Bodnar, Z. Li, S.C.-T. Lam, and X. Du. 2003. Critical roles for the COOH-terminal NITY and RGT sequences of the integrin β3 cytoplasmic domain in inside-out and outside-in signaling. J. Cell Biol. 162:329–339. [Europe PMC free article] [Abstract] [Google Scholar]
- Xi, X., P. Flevaris, A. Stojanovic, A. Chishti, D.R. Phillips, S.C. Lam, and X. Du. 2006. Tyrosine phosphorylation of the integrin β3 subunit regulates beta(3) cleavage by calpain. J. Biol. Chem. 281:29426–29430. [Abstract] [Google Scholar]
- Zou, Z., H. Chen, A.A. Schmaier, R.O. Hynes, and M.L. Kahn. 2007. Structure-function analysis reveals discrete beta3 integrin inside-out and outside-in signaling pathways in platelets. Blood. 109:3284–3290. [Abstract] [Google Scholar]
Articles from The Journal of Cell Biology are provided here courtesy of The Rockefeller University Press
Full text links
Read article at publisher's site: https://doi.org/10.1083/jcb.200703185
Read article for free, from open access legal sources, via Unpaywall:
https://rupress.org/jcb/article-pdf/179/3/553/1332916/jcb_200703185.pdf
Citations & impact
Impact metrics
Article citations
How Protein Depletion Balances Thrombosis and Bleeding Risk in the Context of Platelet's Activatory and Negative Signaling.
Int J Mol Sci, 25(18):10000, 17 Sep 2024
Cited by: 0 articles | PMID: 39337488 | PMCID: PMC11432290
Review Free full text in Europe PMC
Integrin-Dependent Transient Density Increase in Detergent-Resistant Membrane Rafts in Platelets Activated by Thrombin.
Biomedicines, 12(1):69, 27 Dec 2023
Cited by: 0 articles | PMID: 38255176 | PMCID: PMC10813660
Intracellular tension sensor reveals mechanical anisotropy of the actin cytoskeleton.
Nat Commun, 14(1):8011, 04 Dec 2023
Cited by: 6 articles | PMID: 38049429 | PMCID: PMC10695988
Inhibition of GPIb-α-mediated apoptosis signaling enables cold storage of platelets.
Haematologica, 108(11):2959-2971, 01 Nov 2023
Cited by: 4 articles | PMID: 37345472
Integrin β3 directly inhibits the Gα13-p115RhoGEF interaction to regulate G protein signaling and platelet exocytosis.
Nat Commun, 14(1):4966, 16 Aug 2023
Cited by: 1 article | PMID: 37587112 | PMCID: PMC10432399
Go to all (111) article citations
Similar Articles
To arrive at the top five similar articles we use a word-weighted algorithm to compare words from the Title and Abstract of each citation.
Calpain cleaves RhoA generating a dominant-negative form that inhibits integrin-induced actin filament assembly and cell spreading.
J Biol Chem, 277(27):24435-24441, 18 Apr 2002
Cited by: 63 articles | PMID: 11964413
Tyrosine phosphorylation of the integrin beta 3 subunit regulates beta 3 cleavage by calpain.
J Biol Chem, 281(40):29426-29430, 25 Aug 2006
Cited by: 41 articles | PMID: 16935858
ADAM12/syndecan-4 signaling promotes beta 1 integrin-dependent cell spreading through protein kinase Calpha and RhoA.
J Biol Chem, 278(11):9576-9584, 31 Dec 2002
Cited by: 68 articles | PMID: 12509413
Calpain regulation of integrin alpha IIb beta 3 signaling in human platelets.
Platelets, 11(4):189-198, 01 Jun 2000
Cited by: 19 articles | PMID: 10938897
Review
Funding
Funders who supported this work.
NHLBI NIH HHS (6)
Grant ID: HL068819
Grant ID: R01 HL062350
Grant ID: HL062350
Grant ID: R01 HL068819
Grant ID: HL080264
Grant ID: R01 HL080264