Abstract
Objective
The A1C assay, expressed as the percent of hemoglobin that is glycated, measures chronic glycemia and is widely used to judge the adequacy of diabetes treatment and adjust therapy. Day-to-day management is guided by self-monitoring of capillary glucose concentrations (milligrams per deciliter or millimoles per liter). We sought to define the mathematical relationship between A1C and average glucose (AG) levels and determine whether A1C could be expressed and reported as AG in the same units as used in self-monitoring.Research design and methods
A total of 507 subjects, including 268 patients with type 1 diabetes, 159 with type 2 diabetes, and 80 nondiabetic subjects from 10 international centers, was included in the analyses. A1C levels obtained at the end of 3 months and measured in a central laboratory were compared with the AG levels during the previous 3 months. AG was calculated by combining weighted results from at least 2 days of continuous glucose monitoring performed four times, with seven-point daily self-monitoring of capillary (fingerstick) glucose performed at least 3 days per week.Results
Approximately 2,700 glucose values were obtained by each subject during 3 months. Linear regression analysis between the A1C and AG values provided the tightest correlations (AG(mg/dl) = 28.7 x A1C - 46.7, R(2) = 0.84, P < 0.0001), allowing calculation of an estimated average glucose (eAG) for A1C values. The linear regression equations did not differ significantly across subgroups based on age, sex, diabetes type, race/ethnicity, or smoking status.Conclusions
A1C levels can be expressed as eAG for most patients with type 1 and type 2 diabetes.Free full text

Translating the A1C Assay Into Estimated Average Glucose Values
Associated Data
Abstract
OBJECTIVE
The A1C assay, expressed as the percent of hemoglobin that is glycated, measures chronic glycemia and is widely used to judge the adequacy of diabetes treatment and adjust therapy. Day-to-day management is guided by self-monitoring of capillary glucose concentrations (milligrams per deciliter or millimoles per liter). We sought to define the mathematical relationship between A1C and average glucose (AG) levels and determine whether A1C could be expressed and reported as AG in the same units as used in self-monitoring.
RESEARCH DESIGN AND METHODS
A total of 507 subjects, including 268 patients with type 1 diabetes, 159 with type 2 diabetes, and 80 nondiabetic subjects from 10 international centers, was included in the analyses. A1C levels obtained at the end of 3 months and measured in a central laboratory were compared with the AG levels during the previous 3 months. AG was calculated by combining weighted results from at least 2 days of continuous glucose monitoring performed four times, with seven-point daily self-monitoring of capillary (fingerstick) glucose performed at least 3 days per week.
RESULTS
Approximately 2,700 glucose values were obtained by each subject during 3 months. Linear regression analysis between the A1C and AG values provided the tightest correlations (AGmg/dl = 28.7 × A1C − 46.7, R2 = 0.84, P < 0.0001), allowing calculation of an estimated average glucose (eAG) for A1C values. The linear regression equations did not differ significantly across subgroups based on age, sex, diabetes type, race/ethnicity, or smoking status.
CONCLUSIONS
A1C levels can be expressed as eAG for most patients with type 1 and type 2 diabetes.
The A1C assay is widely accepted and used as the most reliable means of assessing chronic glycemia (1–3). Its close association with risk for long-term complications, established in epidemiologic studies and clinical trials (4–6), has lead to the establishment of specific A1C targets for diabetes care with the goal of preventing or delaying the development of long-term complications (2,7–9). Diabetes treatment is adjusted based on the A1C results, expressed as the percentage of hemoglobin that is glycated. The vast majority of assays have been standardized worldwide, through the National Glycohemoglobin Standardization Program (10), to the assay used in the Diabetes Control and Complications Trial (DCCT), which established the relationship between A1C levels and risk for long-term diabetes complications (4,5).
A new, more stable and specific method of standardization of the A1C assay, which is not intended for use in routine assays, has been developed and proposed to be used for global standardization by the International Federation of Clinical Chemists (11,12). However, the new method results in values that are 1.5–2.0 percentage points lower than current National Glycohemoglobin Standardization Program values (13), potentially causing confusion for patients and health care providers. Moreover, the International Federation of Clinical Chemists results would be expressed in new units (millimoles per mole), which would add to the confusion. Chronic glycemia (A1C) is usually expressed as a percentage of hemoglobin that is glycated, whereas the day-to-day monitoring and therapy of diabetes are based on acute glucose levels expressed as milligrams per deciliter or millimoles per liter. This discrepancy has always been problematic. If we could reliably report chronic metabolic control and long-term management goals as average glucose (AG), i.e., in the same units of measurement as acute glycemia, it would eliminate these potential sources of confusion.
The relationship between A1C and chronic glycemia has been explored in several studies that have supported the association of A1C with AG levels over the preceding 5–12 weeks (14–21). However, the older studies have been limited, including relatively small homogeneous cohorts of patients, usually with type 1 diabetes (14–19). Moreover, almost all of the prior studies have relied on infrequent measures of capillary glucose levels, calling into question the validity of their assessment of chronic glycemia. We performed an international multicenter study to examine the relationship between average glucose, assessed as completely as possible with a combination of continuous glucose monitoring and frequent fingerstick capillary glucose testing, and A1C levels over time to estimate the relationship between the two.
RESEARCH DESIGN AND METHODS
Study subjects were recruited at 11 centers in the U.S., Europe, Africa, and Asia according to the consensus protocol. Type 1 and type 2 diabetic and nondiabetic volunteers were between the ages of 18 and 70 years and were judged as likely to be able to complete the protocol, including performance of the self-monitoring by fingerstick and continuous glucose monitoring. To be eligible, nondiabetic subjects had to have no history of diabetes, a plasma glucose level <97 mg/dl (5.4 mmol/l) after an overnight fast, and an A1C level <6.5%. The diabetic subjects had to have stable glycemic control as evidenced by two A1C values within 1 percentage point of each other in the 6 months before recruitment. Any conditions that might result in a major change in glycemia, such as diseases that might require steroid therapy or plans for pregnancy during the study period, were exclusionary. Similarly, any conditions or treatments that might interfere with the measurement of A1C by any of the study methods, such as hemoglobinopathies (22), or that might interfere with the putative relationship between A1C and AG values, including anemia (hematocrit <39% in men and <36% in women), high erythrocyte turnover as evidenced by reticulocytosis, blood loss and/or transfusions, chronic renal or liver disease, or high-dose vitamin C or erythropoetin treatment, were grounds for exclusion. The study was approved by the human studies committees at the participating institutions, and informed consent was obtained from all participants.
Measures of glycemia
Measures of glycemia included continuous interstitial glucose monitoring (CGM) (CGMS; Medtronic Minimed, Northridge, CA), which measures glucose levels every 5 min and was performed for at least 2 days at baseline and then every 4 weeks during the next 12 weeks. For calibration purposes and as an independent measure of glycemia, subjects performed eight-point (premeal, 90 min postmeal, prebed, and at 3:00 a.m.) self-monitoring of capillary glucose with the HemoCue blood glucose meter (Hemocue Glucose 201 Plus; Hemocue, Ángelholm, Sweden) during the 2 days of CGM. As a third and independent measure of glycemia, subjects were asked to perform seven-point (same as the eight-point profile above without the 3:00 a.m. measurement) fingerstick capillary glucose monitoring (OneTouch Ultra; Lifescan, Milipitas, CA) for at least 3 days per week, at times when CGM was not being performed, for the duration of the study. The results from the CGM and fingerstick monitoring were downloaded from their respective meters and exported to the data coordinating center. To be acceptable for analysis, the CGM data had to include at least one successful 24-h profile out of the 2–3 days of monitoring with no gaps >120 min and a mean absolute difference compared with the Hemocue calibration results <18%, as recommended by the manufacturer.
Blood samples for A1C were obtained at baseline and monthly for 3 months. The blood samples were frozen at −80° C and were sent on dry ice by overnight shipment to the central laboratory. Samples were analyzed with four different DCCT-aligned assays, including a high-performance liquid chromatography assay (Tosoh G7; Tosoh Bioscience, Tokyo, Japan), two immunoassays (Roche A1C and Roche Tina-quant; Roche Diagnostics), and an affinity assay (Primus Ultra-2; Primus Diagnostics, Kansas City, MO). The mean A1C value was used. The laboratory assays were approved by the National Glycohemoglobin Study Program (10) and have intra- and interassay coefficients of variation <2.5% for low and high values. The assays were highly intercorrelated with R2 values of 0.99 and slopes of ~1.0 and intercepts between 0.01 and 0.18. Any samples that demonstrated “aging peaks” on high-performance liquid chromatography, evidence of degradation during storage and/or shipment, were considered unacceptable for analysis. One center in Asia was unable to store samples acceptably, resulting in samples that could not be assayed for A1C. The center was eliminated from the study.
Diabetes management
The study was observational in design. Diabetes management was left to the patients and their usual health care providers and was adjusted based on their fingerstick self-monitoring results. CGM results were reviewed by the study staff at the time they were downloaded. Participants were usually masked to the CGM results during the study; unmasking was required if otherwise undetected frequent or prolonged periods of hypoglycemia were observed, in which case, the health care provider was alerted so that treatment could be adjusted.
Statistical analysis
We calculated an arithmetic mean glucose (AG) for each subject by combining the CGM measurement of interstitial glucose levels, corrected by a factor of 1.05 to be equivalent to capillary glucose levels in our study, and the Lifescan fingerstick measurements of capillary glucose. Because glucose levels were measured much more frequently on the CGM days (n ~ 288 per day) than during the Lifescan days (n ~ 7), the results were weighted so that each measurement was proportional to the inverse of the total number of measurements taken in the same day. Therefore, equal weight was attached to each day during which glucose levels were measured. Subjects with fewer than 7 days of CGM during the study were excluded from analysis. We applied linear and quadratic regression models to estimate the relationship between A1C and AG. The quadratic model did not provide a significant improvement over the linear regression model (P=0.82). An exponential model was considered but not used, since the paucity of data in the higher A1C range led to highly variable estimates. Prediction intervals were calculated to represent the range of predicted AG at given A1C levels (23). To correct for heteroschedasticity, we fit a model where the variance of AG is an increasing function of A1C. As a result, the 90% prediction intervals for AG given A1C is given by
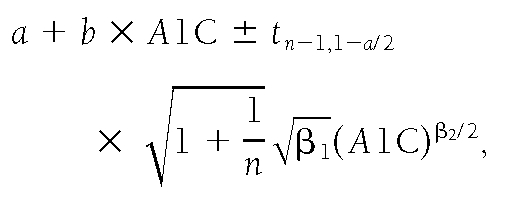
where n=507 and α=0.1, which leads to

.
The mathematical details of the Bayesian method are given in online appendix 1, available at http://dx.doi.org/10.2337/dc08-0545.
For the overall study results to be considered acceptable, it was decided a priori that ≥90% of the individual patients’ calculated AG would have to fall within ±15% of the study-wide calculated AG.
We examined the influence of factors such as age, sex, race (Caucasian, African or African American, or Hispanic), and smoking history on the relationship between A1C and AG through a multivariate regression model. We compared the slopes and intercepts of the regression equations for the individual subgroups and calculated the SDs of the prediction error for each. Age was divided by tertiles separately for type 1 (<40, 40–50, >50 years) and type 2 diabetes (<50, 50–60, >60 years).
RESULTS
Between April 2006 and August 2007, 661 patients were recruited from 10 clinical centers: 6 in the U.S., 3 in Europe, and 1 in Cameroon. A total of 335 participants had type 1 diabetes, 236 had type 2 diabetes, and 90 were nondiabetic (Table 1). The participants were distributed by baseline A1C in three groups, with 18% with A1C >8.5%, 44% between 6.6 and 8.5%, and 38% between 4 and 6.5%. The lowest A1C group consisted of 63% diabetic patients and 37% nondiabetic participants.
Table 1
Baseline characteristics
Screened cohort | Analyzed subjects | |||||||
---|---|---|---|---|---|---|---|---|
Type 1 | Type 2 | Nondiabetic | All | Type 1 | Type 2 | Nondiabetic | All | |
n | 335 | 236 | 90 | 661 | 268 | 159 | 80 | 507 |
Age | 42 ± 13.1 | 54 ± 9.4 | 38 ± 13.1 | 46.1 ± 13.5 | 43 ± 13 | 56 ± 9 | 40 ± 14 | 46 ± 14 |
Sex (% female) | 171 (51) | 119 (50) | 60 (67) | 350 (53) | 140 (52) | 81 (51) | 55 (69) | 276 (54) |
Race/ethnicity | ||||||||
![]() ![]() ![]() ![]() | 282 (84) | 145 (61) | 60 (67) | 487 (74) | 248 (93) | 118 (73) | 56 (71) | 422 (83) |
![]() ![]() ![]() ![]() | 23 (7) | 59 (25) | 15 (17) | 97 (15) | 5 (2) | 21 (13) | 12 (15) | 38 (8) |
![]() ![]() ![]() ![]() | 18 (5) | 23 (10) | 15 (17) | 56 (8) | 15 (6) | 12 (8) | 12 (15) | 39 (8) |
![]() ![]() ![]() ![]() | 12 (4) | 9 (4) | 0 | 21 (3) | 0 | 8 (5) | 0 | 8 (2) |
Smoking status | 40 (12) | 29 (12) | 7 (8) | 76 (12) | 32 (12) | 14 (9) | 7 (9) | 53 (11) |
A1C (%) | 7.3 ± 1.1 | 6.7 ± 0.9 | 5.3 ± 0.3 | 6.8 ± 1.2 | 7.3 ± 1.1 | 6.8 ± 1.1 | 5.2 ± 0.3 | 6.8 ± 1.3 |
Treatment | ||||||||
![]() ![]() ![]() ![]() | 42% | 47% | ||||||
![]() ![]() ![]() ![]() | 58% | 53% | ||||||
![]() ![]() ![]() ![]() | 12% | 10% | ||||||
![]() ![]() ![]() ![]() | 54% | 52% | ||||||
![]() ![]() ![]() ![]() | 17% | 19% | ||||||
![]() ![]() ![]() ![]() | 17% | 19% |
Data are means ± SD or n (%) unless otherwise indicated.
Of 661 subjects who completed screening visits, 154 (23%) were not included in the final analyses for the following reasons: 91 (15%) did not complete the study or were excluded before study end because of conditions that were predefined (such as sickle cell trait [n=5] or anemia [n=5]), were identified during screening, or developed during the study; 11 (2%) did not have adequate CGM; and 52 (8%) did not have samples that could be evaluated for A1C for technical reasons, including sample degradation because of storage or shipment problems.
A total of 507 subjects completed the study and had adequate glucose-monitoring and A1C samples to be included in the analyses (Table 1). The CGM and the Lifescan fingerstick capillary-monitoring data included ~2,500 and 230 measurements per subject, respectively, for a total of ~2,700 glucose tests during the 3-month period. The median number of days of CGM was 13 and of fingerstick capillary monitoring was 39; 36% of the seven-point profiles were complete, with the mean number of tests being 5.1 per day. The correlation of the CGM and simultaneous Hemocue measurements not used for calibrating CGM was excellent, with the 95% limit of the overall average CGMS minus average Hemocue equaling −30.6 to 30.6 mg/dl (−1.7 to 1.7 mmol/l).
For measuring the steady-state correlation between AG and A1C, the study was designed to include subjects with relatively stable glycemia. A1C values were generally stable, with 96% of the subjects maintaining A1C within 1 percentage point of their baseline value over the course of the study.
The relationship between the A1C level at the end of the 3-month study period and the calculated AG during the preceding 3 months, expressed as the simple linear regression AGmg/dl=28.7 × A1C – 46.7 (AGmmol/l=1.59 × A1C − 2.59), R2=0.84, P < 0.0001, is shown in Fig. 1. The correlation has an SD of prediction error of 15.7 mg/dl (0.87 mmol/l). Based on the model described in the statistical analysis section, the estimated values are as follows: α=−41.4, 95% CI −48.8 to −33.5; β=27.9, 26.7–29.0; β1=4.81, 2.18–15.33; β2=2.03, 1.42–2.59. This leads to an estimated error SD of 13.4, 15.7, and 18.0 mg/dl when A1C is 6, 7, and 8%, respectively. The Bayesian model–suggested regression line differs <2 mg/dl from a simple linear regression line in the A1C range of 4–10%, which includes 98.5% of our samples; the prediction intervals widen (P < 0.05) as A1C values increase to 12%, but the difference between the Bayesian and simple linear regression is still <5 mg/dl. A Bland-Altman type of analysis examining the difference between the estimated glucose and observed glucose over the range of glucose values is shown in online appendix 2. The 90% prediction limits for the AG, based on the varying SD model, were very close to the preset limits of ±15% of the predicted mean over the full range of A1C; 89.95% of the samples fell within 15% of the calculated AG.
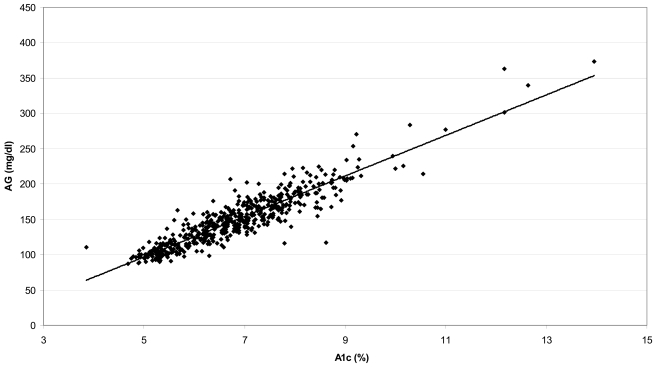
Linear regression of A1C at the end of month 3 and calculated AG during the preceding 3 months. Calculated AGmg/dl=28.7 × A1C − 46.7 (AGmmol=1.59 × A1C − 2.59) (R2=0.84, P < 0.0001).
The translation of A1C to estimated AG (eAG) based on the linear regression is shown in Table 2, for conventional and SI units, and with the 95% prediction limits. Of note, the regression equation for A1C and AG using only the CGM results to calculate AG was AGCGM=28.0 × A1C − 36.9 (R2=0.82, P < 0.0001); the regression using only the seven-point fingerstick profiles to calculate AG was AG7-POINT=29.1 × A1C − 50.7 (R2=0.82, P < 0.0001). The difference in the regressions was not statistically significant for slope and intercept combined (P=0.11).
Table 2
Estimated average glucose
mg/dl* | mmol/l† | |
---|---|---|
A1C (%) | ||
![]() ![]() ![]() ![]() | 97 (76–120) | 5.4 (4.2–6.7) |
![]() ![]() ![]() ![]() | 126 (100–152) | 7.0 (5.5–8.5) |
![]() ![]() ![]() ![]() | 154 (123–185) | 8.6 (6.8–10.3) |
![]() ![]() ![]() ![]() | 183 (147–217) | 10.2 (8.1–12.1) |
![]() ![]() ![]() ![]() | 212 (170–249) | 11.8 (9.4–13.9) |
![]() ![]() ![]() ![]() | 240 (193–282) | 13.4 (10.7–15.7) |
![]() ![]() ![]() ![]() | 269 (217–314) | 14.9 (12.0–17.5) |
![]() ![]() ![]() ![]() | 298 (240–347) | 16.5 (13.3–19.3) |
Data in parentheses are 95% CIs.
*Linear regression eAG (mg/dl)=28.7 × A1C − 46.7.
†Linear regression eAG (mmol/l)=1.5944 × A1C − 2.594.
The relationship between A1C and AG was the same when only the diabetic subjects were included (linear regression eAG=28.3 × A1C − 43.9 [R2=0.79, P < 0.0001]) as that for the whole cohort. A comparison of the regression equations within the specified subgroups is shown in Table 3. There were no significant differences in the slope or intercept for the regression equations for any of the subgroup comparisons, and the SDs of the prediction error were all close to the 15.7 mg/dl (0.87 mmol/l) value for the entire study cohort.
Table 3
Comparison of regression equations between A1C and AG for subgroups
Comparison | Difference in slope | Difference in intercept | P* | |
---|---|---|---|---|
Sex | Male vs. female | 0.17 ± 1.14 | 0.57 ± 7.94 | 0.91 |
Diabetes type | Type 1 vs. type 2 | −1.46 ± 1.61 | 9.35 ± 11.21 | 0.41 |
Age, type 1 diabetes | 1st vs. 2nd tertile | −1.03 ± 2.27 | −5.61 ± 16.56 | 0.71 |
1st vs. 3rd tertile | 1.53 ± 2.37 | −6.99 ± 17.59 | 0.18 | |
2nd vs. 3rd tertile | 0.50 ± 2.47 | −1.38 ± 18.29 | 0.69 | |
Age, type 2 diabetes | 1st vs. 2nd tertile | −7.40 ± 3.67 | 52.00 ± 24.43 | 0.08 |
1st vs. 3rd tertile | −1.57 ± 3.36 | 11.59 ± 22.31 | 0.84 | |
2nd vs. 3rd tertile | 5.83 ± 3.07 | −40.41 ± 21.45 | 0.17 | |
Ethnicity | Caucasian vs. African/African-American | 3.87 ± 1.85 | 23.35 ± 12.48 | 0.07 |
Caucasian vs. Hispanic | −1.80 ± 3.12 | 5.89 ± 20.51 | 0.81 | |
Hispanic vs. African/African-American | −2.06 ± 3.49 | 17.46 ± 22.94 | 0.43 | |
Smoking | Never vs. current | 2.62 ± 1.48 | −16.76 ± 10.93 | 0.14 |
Data are means ± SE.
*χ2 test with 2 d.f. comparing the intercept and slope simultaneously.
CONCLUSIONS
The results of the A1c-Derived Average Glucose (ADAG) study support the notion of a close relationship between A1C levels and AG for both type 1 and type 2 diabetes. The A1C assay plays a central role in the clinical management of diabetes. Treatment goals designed to reduce the development of long-term complications were adopted in the wake of the DCCT (4), and A1C assay methods have been standardized to the DCCT values in most of the world (10). A newly developed method of assay calibration, which is more stable and specific, should further improve the comparability of assays worldwide (11,12). Since this method measures a well-defined analyte of only one molecular species of glycated hemoglobin, the reference values are lower, compared with the previous DCCT-aligned assays. To avoid confusion and potential deterioration of glycemic control as a result of having to report lower A1C values (24), the current study set out to determine the relationship between A1C and AG. The ultimate aim was to determine whether the A1C index of chronic glycemia could be reported in the same units as used for day-to-day monitoring (12,25).
Previous studies of the relationship between A1C and average glycemia have generally been hampered by limited measurements of glucose values, casting doubt on the reliability of the estimates of AG. CGM provides the opportunity to measure all glucose levels. A recent study that included CGM for 3 months arrived at a relationship between A1C and AG very similar to that presented here, providing external validation, but included only 25 subjects, most of whom had type 1 diabetes (21). The current study provides a relatively complete assessment of day-to-day glycemia and establishes a strong enough relationship between A1C and AG levels to justify a direct translation from measured A1C to an easier-to-understand value that is in the same units as fingerstick monitoring. Of note, the regression equation in this study provides lower eAG values, compared with the widely used equation derived from the DCCT, and the scatter around the regression line is less wide (18). The most obvious explanation for the difference between AG calculated from the DCCT and that calculated in the current study is the difference in the frequency of glucose measurements used to calculate AG (a single seven-point profile with no overnight measurements during 3 months in the DCCT compared with numerous CGM and seven-point profile measurements that captured a median of 52 days in ADAG), providing a more complete and representative measure of average glucose in ADAG.
Our results strongly support a simple linear relationship between mean glucose and A1C levels in a clinically relevant range of glycemia. Our data fulfilled the a priori quality criterion; i.e., 90% of the estimates fell within the ±15% range of the regression line. This criterion was considered realistic, allowing for the imprecision of the A1C assay, CGM, and self-monitored blood glucose tests.
The large population allowed us to demonstrate that the relationship between A1C and AG was consistent across prespecified subgroups. The tight relationship and the consistency of the relationship across different subgroups suggest that for many, if not most, patients with diabetes, there are no important factors that affect the relationship between mean glucose levels and A1C. There was a suggestion (P=0.07) that the regression line was different for African Americans such that for a given value of A1C, African Americans might have a slightly lower mean glucose level. This borderline result requires further study to be confident that there is no relationship between ethnicity and the relationship between mean glucose and A1C. There was also a suggestion that age may affect the relationship between AG and A1C; however, the effect was not monotonic. The regression lines for each age-group crossed at A1C of 7%, with the first and last tertile being similar and the middle tertile being different. We suspect that this is a spurious finding. There are other well-recognized clinical factors, such as anemia and altered erythrocyte turnover, which can affect A1C results measured with all assay methods, and hemoglobinopathies, which interfere with the measurement of A1C with specific methods (22). Potential subjects with these conditions were excluded from the study.
The ADAG study has a few limitations. In contrast to our intention and expectation, some ethnic/racial groups were underrepresented, primarily because of the withdrawal of one of the centers with a large Asian population and a limited number of subjects of African descent. In addition, the average glucose estimation was based predominantly on two methods: CGM and intermittent self-monitoring of capillary glucose. (The Hemocue measurements, recognized as providing values that are equivalent to laboratory measurements, were used primarily to calibrate the CGM [26].) To combine these measurements into a single calculated AG, the CGM and fingerstick capillary measurements had to be weighted to take into account the different number of measurements in a day; however, in separate analyses comparing the relationships between A1C and AG measured with CGM or fingerstick capillary measurements, there was no significant difference in the relationships. Finally, since only diabetic patients in stable control and without any suggestion of erythrocyte disorders were entered into the study, the current results are only directly applicable to this population. Children and pregnant women were also excluded; additional data in these groups are needed to confirm the established relationship. Of note, a recently published study compared the calculated mean glucose of 47 children with type 1 diabetes between the ages of 4 and 18 years who had at least one 24-h period of CGM in 6 of 13 weeks with the A1C at the end of the 13-week period (27). Although the authors also concluded that “A1C directly reflects mean glucose over time,” they found substantially greater inter-individual variation in the relationship between AG and A1C than present in the current study. The potential sources of this variability can be identified by comparing the DirectNet study in children (27) with ADAG and with the recent study in adults (21) who were selected for stable glycemic control and performed CGM for 97% of the 12-week study period. The DirectNet study used a noncentralized A1C method with relatively poor correlation with a high-performance liquid chromatography method. Moreover, the children had highly variable glycemia and only performed CGM for 67% of the study period; this may have failed to accurately capture mean glycemia.
The current results support the reporting of the measured A1C as eAG. The interpretation of the A1C, analogous to reporting serum creatinine as a calculated glomerular filtration rate, should provide health care providers with a more useful index of chronic glycemia. A recently published consensus guideline has endorsed reporting A1C values along with the calculated eAG level, assuming that the results of the ADAG were acceptable (25).
Acknowledgments
This work was supported by research grants from the American Diabetes Association and European Association for the Study of Diabetes. Financial support was provided by Abbott Diabetes Care, Bayer Healthcare, GlaxoSmithKline, sanofi-aventis Netherlands, Merck, Lifescan, and Medtronic Minimed, and supplies and equipment were provided by Medtronic Minimed, Lifescan, and Hemocue.
Appendix
ADAG Study Group
Study centers: J.K. (principle investigator [PI]), G.S.M.A Kerner, and A. van Iperen, Amsterdam, the Netherlands; E. Horton (PI), A. Cohen, S. Herzlinger-Botein, and J. Paradis, Boston, MA; C. Saudek (PI), K. Moore, A. Greene, and M. Islas, Baltimore, MD; J. Nerup (PI), R.B., and C. Glümer, Copenhagen, Denmark; A. Mosca (co-PI), A. Lapolla (co-PI), D. Fedele, and G. Sartore, Padova, Italy; X. Pi-Sunyer (PI), C. Maggio, L. Haselman, and C. Bellino, New York, NY; S. Smith (PI), A. Reynolds, T. Robertson, H. Binner, and K. Hurtis, Rochester, MN; S. Schwartz (PI), A. Ramos, A. Gonzales, A. Childress, and Y. Martinez, San Antonio, TX; I. Hirsch (PI), D. Khakpour, and C. Farricker, Seattle, WA; and J.C. Mbanya (PI), E. Sobngwi, and E. Balti, Yaoundé, Cameroon. Central laboratory: R. Slingerland (PI), E. Lenters, and H.P van Berkel, Zwolle, the Netherlands. Biostatistics center: D.S. (PI), H.Z., K. Pelak, and R. Wilson, Boston, MA. Coordinating center: D.M.N. (PI), N. Kingori, and H. Turgeon, Boston, MA. Study chairs: R.H. and D.M.N.
Footnotes
See accompanying editorial, p. 1704.
References
Articles from Diabetes Care are provided here courtesy of American Diabetes Association
Full text links
Read article at publisher's site: https://doi.org/10.2337/dc08-0545
Read article for free, from open access legal sources, via Unpaywall:
http://care.diabetesjournals.org/content/31/8/1473.full.pdf
Free to read at intl-care.diabetesjournals.org
http://intl-care.diabetesjournals.org/cgi/content/abstract/31/8/1473
Free after 6 months at intl-care.diabetesjournals.org
http://intl-care.diabetesjournals.org/cgi/content/full/31/8/1473
Free after 6 months at intl-care.diabetesjournals.org
http://intl-care.diabetesjournals.org/cgi/reprint/31/8/1473.pdf
Citations & impact
Impact metrics
Article citations
Identification of gut microbiome features associated with host metabolic health in a large population-based cohort.
Nat Commun, 15(1):9358, 29 Oct 2024
Cited by: 0 articles | PMID: 39472574 | PMCID: PMC11522474
What is the optimal range of fasting stress hyperglycemia ratio for all-cause mortality in American adults: An observational study.
Medicine (Baltimore), 103(43):e40288, 01 Oct 2024
Cited by: 0 articles | PMID: 39470510 | PMCID: PMC11521012
Hemoglobin A1c Genetics and Disparities in Risk of Diabetic Retinopathy in Individuals of Genetically Inferred African American/African British and European Ancestries.
Diabetes Care, 47(10):1731-1739, 01 Oct 2024
Cited by: 0 articles | PMID: 39042486
Evaluating HbA<sub>1c</sub>-to-average glucose conversion with patient-specific kinetic models for diverse populations.
Sci Rep, 14(1):22098, 27 Sep 2024
Cited by: 0 articles | PMID: 39333162 | PMCID: PMC11437029
Techniques for Implementing Continuous Glucose Monitoring in Primary Care: Key CGM Updates and Highlights from the ATTD 2024 Conference [Podcast].
Diabetes Metab Syndr Obes, 17:3577-3583, 23 Sep 2024
Cited by: 0 articles | PMID: 39345824 | PMCID: PMC11436668
Go to all (838) article citations
Other citations
Data
Data behind the article
This data has been text mined from the article, or deposited into data resources.
BioStudies: supplemental material and supporting data
Similar Articles
To arrive at the top five similar articles we use a word-weighted algorithm to compare words from the Title and Abstract of each citation.
Translating the HbA1c assay into estimated average glucose values in children and adolescents with type 1 diabetes mellitus.
Acta Biomed, 89(s5):22-26, 23 May 2018
Cited by: 3 articles | PMID: 30049928 | PMCID: PMC6179094
Associations between features of glucose exposure and A1C: the A1C-Derived Average Glucose (ADAG) study.
Diabetes, 59(7):1585-1590, 27 Apr 2010
Cited by: 60 articles | PMID: 20424232 | PMCID: PMC2889756
[Glycated haemoglobin may in future be reported as estimated mean blood glucose concentration--secondary publication].
Ugeskr Laeger, 171(45):3262-3265, 01 Nov 2009
Cited by: 0 articles | PMID: 19916193
Glycated hemoglobin A1c as a modern biochemical marker of glucose regulation.
Med Pregl, 67(9-10):339-344, 01 Sep 2014
Cited by: 11 articles | PMID: 25546982
Review