Abstract
Free full text

The Nalp3 inflammasome is essential for the development of silicosis
Abstract
Inhalation of crystalline silica and asbestos is known to cause the progressive pulmonary fibrotic disorders silicosis and asbestosis, respectively. Although alveolar macrophages are believed to initiate these inflammatory responses, the mechanism by which this occurs has been unclear. Here we show that the inflammatory response and subsequent development of pulmonary fibrosis after inhalation of silica is dependent on the Nalp3 inflammasome. Stimulation of macrophages with silica results in the activation of caspase-1 in a Nalp3-dependent manner. Macrophages deficient in components of the Nalp3 inflammasome were incapable of secreting the proinflammatory cytokines interleukin (IL)-1β and IL-18 in response to silica. Similarly, asbestos was capable of activating caspase-1 in a Nalp3-dependent manner. Activation of the Nalp3 inflammasome by silica required both an efflux of intracellular potassium and the generation of reactive oxygen species. This study demonstrates a key role for the Nalp3 inflammasome in the pathogenesis of pneumoconiosis.
Silica crystals are abundant and naturally occurring. Airborne silica particles are commonly encountered in numerous occupations, including mining, construction, manufacturing, and farming. The inhalation of these particles causes the development of silicosis, which is a chronic interstitial pulmonary disease characterized by the delayed development of fibrosis with progressive pulmonary dysfunction (1–3). Similarly, inhalation of asbestos particles causes the development of the associated fibrotic disease asbestosis (1–3). There is no effective therapy for silicosis or asbestosis, nor are the underlying immunologic mechanisms leading to disease clearly understood. Alveolar macrophages, and the IL-1β produced by these cells, have been suggested to play a crucial role during the early inflammatory response after exposure to silica or asbestos. Alveolar macrophages from patients with asbestosis spontaneously secrete elevated amounts of IL-1β compared with healthy controls (4, 5). Macrophages treated with silica have been shown to secrete IL-1β, and IL-1β-deficient mice are more resistant to developing pulmonary pathology in response to inhaled silica (6, 7). Additionally polymorphisms in the IL-1Ra allele have been linked to human susceptibility to silicosis (8).
The mammalian NLR family is composed of over 20 members that contain a C-terminal leucine-rich repeat (LRR) domain, a central nucleotide-binding NACHT domain, and an N-terminal protein–protein-interaction domain composed of a CARD (caspase activation and recruitment domain) or pyrin domain (9, 10). A number of NLR family members have been shown to activate the cysteine protease caspase-1 within a multiprotein complex called the inflammasome (11). Caspase-1 is capable of initiating the cell death pathway known as pyroptosis as well as cleaving the precursor cytokines pro-IL-1β, pro-IL-18, and pro-IL-33 into their mature forms, IL-1β, IL-18, and IL-33, respectively. The NLR family member Nalp3 (also known as cryopyrin, CIAS1, and NLRP3) can activate caspase-1 in response to a number of diverse stimuli, including ATP, monosodium urate crystals (MSU), and bacterial pore-forming toxins (12–14). Upon activation Nalp3 recruits the adaptor molecule ASC by a pyrin–pyrin-domain interaction; ASC then recruits caspase-1 by CARD–CARD interactions, thus forming the NALP3 inflammasome.
In this study we identify Nalp3 as being an important innate immune receptor involved in the recognition of silica and asbestos by macrophages. Stimulation of macrophages with either silica or asbestos resulted in the robust secretion of IL-1β in a manner dependent on the Nalp3 inflammasome. We also demonstrate that silica-induced production of reactive oxygen species (ROS) is required for the activation of the Nalp3 inflammasome. Finally, we show that the chronic fibrosis seen in a murine model of silicosis in vivo is dependent upon the presence of ASC and Nalp3. These findings suggest that silica-induced production of IL-1β through the Nalp3 inflammasome plays an important role in the initial inflammatory responses that lead to silicosis.
Results and Discussion
Silica Induces IL-1β but Not TNFα or IL-6 Secretion in Vitro.
The role of inflammation in the development of silicosis is well accepted. Surprisingly, crystalline silica (SiO2) was found to be a poor inducer of macrophage TNFα and IL-6 (Fig. 1 A and B) when compared with the potent inflammatory stimulus LPS. The expression of IL-1β and IL-12p40 mRNA in macrophages after stimulation with either silica or LPS was examined by RT-PCR. LPS stimulation resulted in the expression of both IL-1β and IL-12p40 mRNA, which was not detected in silica-stimulated macrophages (Fig. 1C). However, treatment of LPS-primed macrophages with silica resulted in the rapid secretion of IL-1β in a dose-dependent manner (Fig. 1D). Treatment of LPS-primed macrophages with asbestos also resulted in secretion of IL-1β in a dose-dependent manner (Fig. 1E). Silica, asbestos, or LPS-priming alone was not capable of inducing IL-1β secretion (Fig. 1 D and E), which is consistent with the actions of other stimuli such as MSU and ATP that require macrophage priming to activate caspase-1 (12–14). In contrast, titanium dioxide (TiO2), which does not cause fibrotic disease upon inhalational exposure (15), did not induce the secretion of IL-1β from LPS-primed macrophages (Fig. 1F). LPS-primed human alveolar macrophages were also capable of secreting IL-1β in response to silica or asbestos (Fig. 1G), demonstrating that this effect was not restricted to murine macrophages. These data suggest that silica induced IL-1β production is an early step in the inflammatory response to silica and likely drives the subsequent production of other inflammatory mediators. This makes IL-1β an attractive target for potential therapeutic intervention. The specificity for silica to induce IL-1β secretion and not TNFα and IL-6 is consistent with the clinical syndrome of silicosis, which is a chronic low-level inflammatory process that is manifest years after the initial exposure to silica.
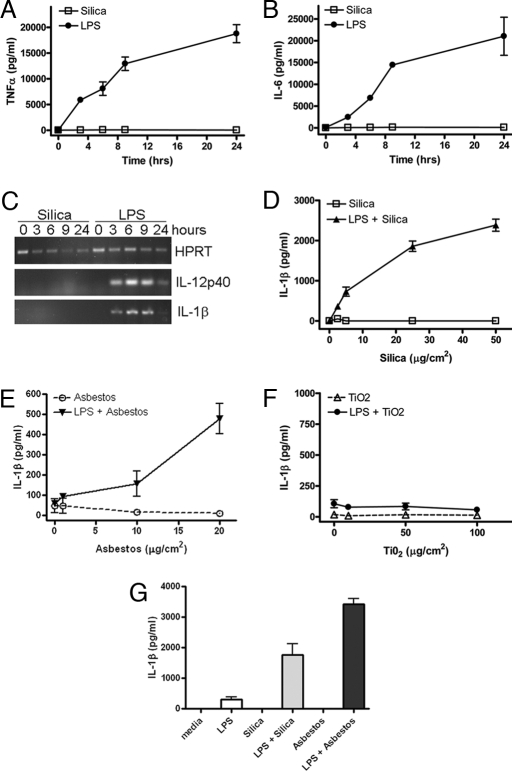
Silica induces IL-1β secretion from LPS-primed macrophages. (A–C) Macrophages from WT mice were stimulated with either 50 μg/cm2 silica or 50 ng/ml LPS. Culture supernatants were collected at the indicated time after stimulation; TNFα (A) and IL-6 (B) release into culture supernatants was measured by ELISA. (C) IL-1β and IL-12p40 expression in silica- or LPS-stimulated macrophages was determined by RT-PCR. (D–F) Macrophages from WT mice were either primed with 50 ng/ml LPS or left untreated. Macrophages were stimulated with the indicated dose of silica (D), asbestos (E), or titanium dioxide (F), and culture supernatants were collected 4 h later. IL-1β release into culture supernatants was measured by ELISA. (G) Human alveolar macrophages were either primed with 50 ng/ml LPS or left untreated. Macrophages were stimulated with 50 μg/cm2 silica or 40 μg/cm2 asbestos for 4 h, and IL-1β release into culture supernatants was measured by ELISA. Determinations were performed in triplicate and expressed as the mean ± SEM. Results are representative of two separate experiments.
Silica and Asbestos Induce IL-1β Secretion in a Nalp3-Dependent Manner.
Nalp3 along with the adaptor molecule ASC can activate caspase-1 in response to a variety of stimuli, including ATP, nigericin, maitotoxin, MSU, and the pathogens Listeria monocytogenes and Staphylococcus aureus (12–14). We used macrophages deficient in specific components of the Nalp3 inflammasome to test whether they were required for silica-induced IL-1β secretion. LPS-primed Nalp3-, ASC- and caspase-1-deficient macrophages all displayed a marked defect in their ability to secrete IL-1β in response to silica compared with macrophages from WT mice (Fig. 2A). In contrast, macrophages deficient in the NLR molecule IPAF, which is important for macrophage caspase-1 activation in response to infection with type III and type IV secretion system carrying Gram-negative bacteria (16–18), had an intact response to silica and were capable of secreting IL-1β (Fig. 2A). In addition, LPS-primed Nalp3-, ASC-, and caspase-1-deficient macrophages were also unable to secrete the other caspase-1-processed cytokine IL-18 in response to silica (Fig. 2B).
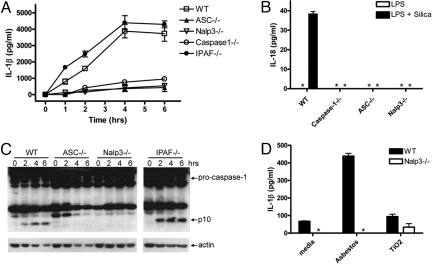
Silica- and asbestos-induced IL-1β secretion is dependent on Nalp3. LPS-primed macrophages from WT, caspase-1-, ASC-, Nalp3-, or IPAF-deficient mice were stimulated with 50 μg/cm2 silica. Culture supernatants were collected at 4 h or at the indicated time after stimulation (A–C) and IL-1β and IL-18 release into culture supernatants was measured by ELISA (A and B). Determinations were performed in triplicate and expressed as the mean ± SEM. Results are representative of three (A) and two (B) separate experiments. (C) Lysates from LPS-primed WT, ASC-, NALP3-, or IPAF-deficient macrophages stimulated with 50 μg/cm2 silica for the indicated times were immunoblotted with antibodies against the p10 subunit of caspase-1. (D) LPS-primed macrophages from WT and NALP3-deficient mice were stimulated with either 40 μg/cm2 asbestos or 50 μg/cm2 titanium dioxide. Culture supernatants were collected 6 h later and IL-1β release into culture supernatants was measured by ELISA. Determinations were performed in triplicate and expressed as the mean ± SEM. Results are representative of three separate experiments. *, Nondetectable.
Caspase-1 activation involves autocatalytic processing of the 45-kDa pro-caspase-1 to generate two subunits, p20 and p10. Caspase-1 activation in silica-stimulated LPS-primed WT macrophages was detected by Western blotting by the appearance of the p10 cleavage product 2 h after stimulation with silica (Fig. 2C). We did not observe caspase-1 activation in response to silica in either Nalp3- or ASC-deficient LPS-primed macrophages (Fig. 2C). As expected, IPAF-deficient LPS-primed macrophages did not display any defect in caspase-1 activation in response to silica (Fig. 2C). These data identify the Nalp3 inflammasome to be required for caspase-1-mediated IL-1β and IL-18 secretion in response to stimulation with silica.
The inhalation of asbestos leads to a similar fibrotic syndrome, asbestosis, characterized by chronic inflammation and insidious deterioration of lung function, which usually first manifests more than 15 years after the initial exposure to asbestos. Asbestos, but not titanium dioxide, stimulation of LPS-primed WT macrophages resulted in the secretion of IL-1β in a Nalp3-dependent manner (Fig. 2D). This suggests a common mechanism for inflammation in response to the pro-fibrotic agents silica and asbestos requiring Nalp3, which is not shared by other, nonfibrotic, inhalant particles.
Silica-Induced Cytotoxicity Is Independent of Nalp3.
It has been shown that treatment of macrophages with silica is cytotoxic (19). To determine whether silica-induced cytotoxicity was associated with Nalp3, we assessed the degree of cell death in WT and Nalp3-deficient macrophages after silica treatment. We confirmed the cytotoxic effect of silica and found it to be equivalent in macrophages from WT and Nalp3-deficient mice (Fig. 3A). These data demonstrate that silica-induced cytotoxicity is not dependent on Nalp3, and that IL-1β release was not a consequence of cell lysis, because WT and Nalp3-deficient macrophages had similar amounts of cell death but only the former released IL-1β.
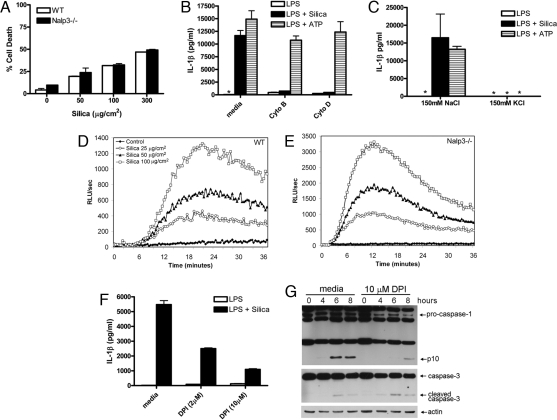
Silica-induced IL-1β secretion requires internalization of silica, a potassium efflux, and generation of reactive oxygen species. (A) LPS-primed WT macrophages were stimulated with the indicated amount of silica. Culture supernatants were collected 4 h later and cytotoxicity was measured by lactate dehydrogenase (LDH) release and expressed as a percentage of LDH release by Triton X-100 detergent. (B) LPS-primed WT macrophages were incubated with 10 μM cytochalasin B or 20 μM cytochalasin D for 10 min before the addition of 50 μg/cm2 silica or 5 mM ATP. (C) LPS-primed macrophages were incubated in either high Na+- or high K+-containing medium and then stimulated with either 50 μg/cm2 silica or 5 mM ATP. ATP-treated cells had their medium replaced with fresh medium after 20 min. Four hours after initial stimulation, culture supernatants were collected and IL-1β release was measured by ELISA. (A–C) Determinations were performed in triplicate and expressed as the mean ± SEM. Results are representative of two separate experiments. (D and E) WT and Nalp3−/− macrophages were stimulated with the indicated amount of silica, and ROS production was measured by luminol-amplified chemiluminescence. RLU, relative light units. (F and G) LPS-primed WT macrophages were incubated with 10 μM or the indicated amount of diphenyleneiodonium chloride (DPI) for 10 min before the addition of 50 μg/cm2 silica. Four hours after initial stimulation, culture supernatants were collected and IL-1β release was measured by ELISA (F). Determinations were performed in triplicate and expressed as the mean ± SEM and are representative of three separate experiments. At the indicated time after stimulation lysates were collected and immunoblotted with antibodies against the p10 subunit of caspase-1, caspase-3, and actin (G). *, Nondetectable.
Silica-Induced IL-1β Secretion Requires Crystal Internalization and Potassium Efflux.
To understand how silica might activate the Nalp3 inflammasome, we tested whether the endocytic ability of macrophages was required for silica-stimulated IL-1β production. Inhibiting actin polymerization with either cytochalasin B or cytochalasin D inhibited IL-1β production by silica in LPS-primed macrophages (Fig. 3B). Neither cytochalasin B nor cytochalasin D reduced IL-1β production in response to stimulation with ATP, which uses the P2X7 receptor to activate the Nalp3 inflammasome, confirming that macrophages were still viable and capable of secreting Nalp3-dependent IL-1β (Fig. 3B). These data suggest that, unlike ATP, endocytosis of silica by macrophages is needed to activate the Nalp3 inflammasome in response to silica for the resultant processing and secretion of proinflammatory cytokines. Endocytosis of particulates may be occurring through the scavenger receptors SR-A and MARCO, which have been implicated in playing an important role in the inflammatory response to silica (20). Further studies will be required to determine whether these receptors are also required for silica-induced Nalp3 inflammasome activation.
A number of diverse stimuli can activate the Nalp3 inflammasome. However, a common step shared by these stimuli to induce Nalp3-dependent caspase-1 activation is the efflux of cellular potassium (21). Preventing the potassium efflux by increasing extracellular potassium inhibits caspase-1 activation in response to stimuli that activate Nalp3 but not stimuli that activate caspase-1 through IPAF (21). Increased extracellular potassium significantly inhibited silica-induced IL-1β production from macrophages (Fig. 3C). As expected, increased extracellular potassium also inhibited ATP-mediated IL-1β production, which is also dependent on Nalp3. Silica therefore is a previously unrecognized Nalp3 stimulus, which like other Nalp3 stimuli requires a cellular potassium efflux to activate caspase-1.
Silica-Induced ROS Are Required for Nalp3 Inflammasome Activation.
There are two main sources of cellular ROS. The first is the phagocyte NADPH oxidase, which generates superoxide anions as part of the antimicrobial response to ingested microbes (22). In the resting phagocyte, components of the NADPH oxidase are spatially segregated, with flavocytochrome b558 in the membrane and p47phox, p67phox, and Rac in the cytoplasm. Upon stimulation, the cytosolic components translocate to the membrane and assemble into a functioning enzyme complex, shuttling electrons from cytoplasmic NADPH to molecular oxygen to generate extracellular or phagosomal superoxide anion. Mitochondria have also long been known to generate reactive oxidants and are a major source of cellular ROS (22). The mitochondrial respiratory chain is made up of four electron-transporting complexes and a proton-translocating complex, with the majority of intracellular ROS produced during mitochondrial respiration by complex I. ROS have also been shown to play important signaling functions within the cell either for homeostatic functions or to signal stress. This is particularly the case for the mitochondria, which can either confine its production of ROS locally or flood the cell with oxidants, causing damage to itself and the cell in the process.
The Nalp3 inflammasome activator ATP has been shown to be capable of inducing the production of ROS, which are important for caspase-1 activation (23). As silica can also induce ROS production from macrophages (19) we examined whether this was required for caspase-1 mediated IL-1β secretion. Using HRP-dependent luminol-enhanced chemiluminescence to detect both intra- and extracellular ROS, we confirmed that silica induced ROS production from macrophages (Fig. 3D) and that the response was blocked by preincubation of macrophages with the inhibitor of NADPH oxidase diphenyleneiodonium chloride (DPI) (data not shown). DPI also markedly inhibited silica-induced IL-1β secretion and caspase-1 activation (Fig. 3 F and G), thereby suggesting that ROS signaling was required for silica-induced Nalp3 inflammasome activation. DPI did not inhibit silica-induced caspase-3 activation in LPS-primed macrophages (Fig. 3G). Nalp3-deficient macrophages were capable of producing ROS in response to silica (Fig. 3E), indicating that ROS signaling is likely occurring upstream of Nalp3. Although these data demonstrate a role for ROS in silica-induced IL-1β secretion, they do not distinguish between the two most likely sources for ROS in stimulated cells, because DPI can also exert inhibitory effects on mitochondrial ROS production in addition to NADPH oxidase. However, taken together these studies clearly define a novel signaling pathway used to activate the Nalp3 inflammasome.
ASC- and Nalp3-Deficient Mice Have Decreased Pulmonary Pathology in Response to Inhaled Silica.
To confirm the physiologic relevance of our in vitro data, we assessed the role of the Nalp3 inflammasome in a murine model of silicosis. We treated WT, ASC−/−, and Nalp3−/− mice with intranasal instillation of silica and assessed pulmonary inflammation and fibrosis after 3 months. WT mice had profound cellular inflammatory changes and granulomas as seen on H&E staining; in contrast there was a reduction in inflammation and granuloma formation in both ASC- and Nalp3-deficient mice (Fig. 4A). The reduction in inflammation in ASC- and Nalp3-deficient mice was verified by using a blinded scoring of the sections (Fig. 4A). Much of the pathology of clinical silicosis is caused by the development of pulmonary fibrosis. This could be seen by the increased deposition of collagen on trichrome staining in lung sections from WT mice 3 months after silica challenge (Fig. 4B). Both ASC- and Nalp3-deficient mice had less collagen deposition compared with WT mice (Fig. 4B). Thus, the activation of the Nalp3 inflammasome by silica is instrumental in the development of the silicosis.
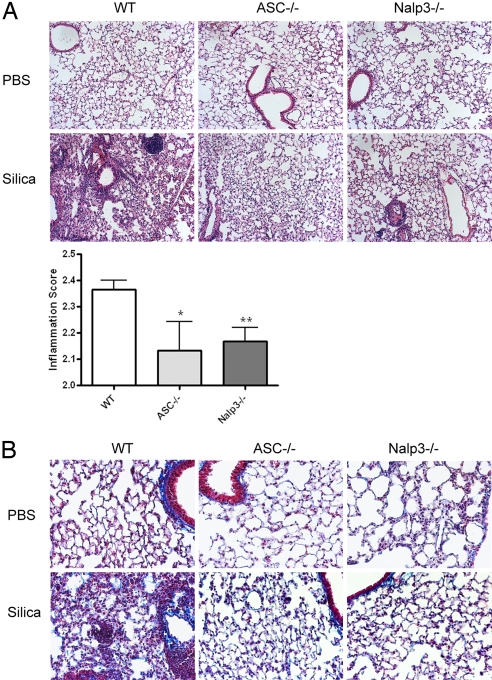
Decreased inflammation and collagen deposition in ASC- and Nalp3-deficient mice after intranasal instillation of silica. WT, ASC−/−, and Nalp3−/− mice received either intranasal PBS or silica. Three months after the initial challenge, lungs were stained with H&E to examine inflammation (A) or trichrome stain to examine collagen deposition (B). H&E-stained sections were scored in a blinded fashion for cellular tissue infiltration (*, P = 0.039; **, P = 0.0062 compared with WT by one-tailed unpaired Student's t test). Images were obtained at ×10 (A) and ×20 (B) magnification and are representative of two mice per group for PBS and five mice per group for silica.
Macrophage production of IL-1β has been implicated to play an important role in the pathogenesis of both silicosis and asbestosis. Macrophages treated with silica have been shown to secrete IL-1β, and IL-1β-deficient mice are more resistant to developing silicosis compared with WT mice (6, 7). IL-1R antagonists have also been successfully used to block fibrosis development in murine models of pulmonary fibrosis (24, 25). Our data support these findings and identify Nalp3 as being the pattern recognition receptor that is responsible for responding to silica by activating caspase-1 and resulting in the secretion of IL-1β. It is tempting to speculate that mutations in Nalp3, similar to those that result in the autoinflammatory syndromes Muckle Wells, familial cold autoinflammatory syndrome, and CINCA, may also predispose patients to developing silicosis and asbestosis.
It is known that development of silicosis is multifactorial, with environmental factors and genotype likely to be critical in the pathogenesis of disease. Secretion of IL-1β by macrophages in response to silica and asbestos in vitro is clearly a two-step process. Macrophages initially have to be primed to respond to silica or asbestos. This does not appear to be restricted to murine macrophages, as we have also observed the requirement for priming in human alveolar macrophages stimulated with silica and asbestos in vitro (Fig. 1G). The source for this priming step in vivo remains unclear and may be mediated by locally produced inflammatory cytokines. Disease in humans may also be accelerated by a concomitant respiratory infection, which would augment macrophage priming.
Taking the findings together, this study demonstrates that macrophages directly recognize and react to silica by means of the Nalp3 inflammasome. This initial interaction leads to the release of ROS and the activation of caspase-1 with subsequent driving of an inflammatory response. Inflammatory cytokines such as TNFα have been shown to play a role in silicosis. Our data suggest that these inflammatory cytokines are likely a consequence of silica-induced IL-1β production and not a direct result of silica's action upon the macrophage. Recent studies have implicated caspase-1 in modulating unconventional protein secretion and in particular that of fibroblast growth factor-2. (FGF-2) (26). This has intriguing implications for additional roles of caspase-1 in disease pathogenesis. The central role of Nalp3 in recognition of silica and activation of caspase-1 makes it an attractive target for potential therapeutic intervention.
Materials and Methods
Mice.
The generation of NALP3-, ASC-, caspase-1-, and IPAF-deficient mice has been described previously (12, 27, 28). Caspase-1-, ASC-, and NALP3-deficient mice were backcrossed onto the C57BL/6 genetic background for at least nine generations. IPAF-deficient mice were backcrossed onto the C57BL/6 genetic background for at least six generations. Age- and sex-matched C57BL/6 mice purchased from the National Cancer Institute were used as WT controls. All protocols used in this study were approved by the Institutional Animal Care and Use Committees at the University of Iowa and Yale University.
Macrophages.
Thioglycollate-elicited peritoneal macrophages were generated by injecting 1 ml of 3% thioglycollate solution into the peritoneal cavity of mice. Three to 4 days later macrophages were collected by peritoneal lavage and plated in DMEM supplemented with 10% FCS, 2 mM l-glutamine, 100 units/ml penicillin G, and 100 μg/ml streptomycin. Unless indicated, macrophages were activated by stimulating with 50 ng/ml LPS from Escherichia coli serotype O111:B4 (InvivoGen) for 4–12 h before stimulation with silica or asbestos.
Human alveolar macrophages were obtained from normal volunteers by bronchoalveolar lavage as described in ref. 29, and the procedure was approved by the Committee for Investigations Involving Human Subjects at the University of Iowa.
In Vitro Stimulation of Macrophages.
Macrophages that were either unstimulated or had been activated with 50 ng/ml LPS for 4–12 h were stimulated with either 50 μg/cm2 silica (Min-U-Sil-5; Pennsylvania Glass Sand), 40 μg/cm2 crocidolite asbestos (North American Insulation Manufacturers Association Fiber Repository), or 50 μg/cm2 titanium dioxide (Sigma) unless otherwise indicated. Four hours, or at the indicated time, after stimulation supernatants were collected. Culture supernatants were then assayed for IL-1β and TNFα and IL-6. Antibody pairs for the IL-1β ELISAs were from R&D Systems. Antibody pairs for the TNFα and IL-6 ELISAs were from eBiosciences. Macrophage cell death was determined 4 h after stimulation with silica by using a cytotoxicity (LDH release) detection kit (Promega). The inhibitors cytochalasin B (10 μM), cytochalasin D (20 μM), and diphenyleneiodonium chloride (DPI) (2 μM and 10 μM) were added 10 min before the addition of silica.
RT-PCR.
RNA was isolated from macrophages by using the Qiagen RNeasy Mini kit and following the manufacturer's instructions. cDNA was synthesized by using SuperScript II reverse transcriptase (Invitrogen). The primers used for RT-PCR amplification were as follows: HPRT, 5′-GTTGGATACAGGCCAGACTTTGTT and 3′-GAGGGTAGGCTGGCCTATAGGCT; IL-12p40, 5′-ATGGCCATGTGGGAGCTGGAGAAAG and 3′-GTGGAGCAGCAGATGTGAGTGGCT; IL-1β, 5′-GACAGTGATGAGAATGACCTGTTC and 3′-CCTGACCACTGTTGTTTCCC.
Inhibition of Potassium Efflux.
To inhibit potassium efflux, macrophages were primed with 50 ng/ml LPS for 12 h and then medium was replaced with serum-free buffer with 150 mM KCl (10 mM Hepes, 5 mM NaH2PO4, 150 mM KCl, 1 mM MgCl2, 1 mM CaCl2, 1% BSA, pH 7.4). In parallel, a buffer with 150 mM NaCl was used (10 mM Hepes, 150 mM NaCl, 5 mM KH2PO4, 1 mM MgCl2, 1 mM CaCl2, 1% BSA, pH 7.4).
ROS Measurement.
Production of ROS was measured by the peroxidase-dependent luminol-amplified chemiluminescence technique, as previously described (30). Briefly, chemiluminescence was monitored with a Lumistar Galaxy (BMG Labtech) luminometer. Macrophages were plated on white polystyrene microtiter plates (Optiplate, Perkin–Elmer) in Hanks balanced salt solution containing 1 mM CaCl2, 1.5 mM MgCl2, 10 mM glucose, and 1% human serum albumin. Cells were incubated at 37°C in the presence of 50 μM luminol and 4 units of horseradish peroxidase. Stimuli were added to the respective wells and chemiluminescence was followed continuously for 60 min. Assays were run in duplicate.
Western Blotting.
Electrophoresis of proteins was performed by using the NuPAGE system (Invitrogen) according to the manufacturer's protocol. Briefly, after stimulation with silica, macrophages and supernatants were lysed in lysis buffer [50 mM Tris·HCl, 5 mM EDTA, 150 mM NaCl, 1% Triton X-100, and a protease inhibitor mixture (Roche)] and stored at −80°C until analyzed. Proteins were separated on a NuPAGE gel and transferred to a PVDF membrane by electroblotting. To detect caspase-1 a rabbit polyclonal anti-mouse casapse-1 p10 antibody (Santa Cruz Biotechnology) was used. Caspase-3 and actin were detected by using antibodies from Cell Signaling and Calbiochem, respectively.
In Vivo Silicosis Model.
Six to 8-week-old C57BL/6, ASC−/−, and Nalp3−/− mice were anesthetized with metophane before intranasal instillation of either 50 μl of sterile PBS or 50 μl of a PBS suspension of silica (20 mg/ml). Mice received instillations of silica on days 0 and 14. Three months after the first instillation of silica mice were killed and lungs were harvested. Lungs were fixed and embedded in paraffin. Sections were stained with either trichrome or hematoxylin and eosin (H&E). H&E stains were used for analysis of airway inflammation and pathological changes. Inflammation was scored on a scale of 1–3 arbitrary units by a pathologist blinded to the experimental protocol, taking into account both the degree of inflammation and its distribution. Lymphoid and macrophage infiltration severity were scored separately and the scores were pooled. For example, none to rare lymphoid or macrophages were given a score of 1, small to moderate-sized perivascular lymphoid aggregates or activated/foamy macrophages scattered within alveoli/interstitium were given a score of 2, and large perivascular lymphoid aggregates or activated/foamy macrophages filling alveoli/interstitium were given a score of 3. The percentage of lung affected was given a score of 0–3 with 0% getting a score of 0, 1–5% getting a score of 1, 6–40% getting a score of 2, and >40% getting a score of 3.
Acknowledgments.
We thank David Meyerholz for pathology analysis. We thank William Nauseef and Joao Pedra for critical review of the manuscript and Anthony Coyle, Ethan Grant, and John Bertin for providing mutant mice. This work was supported by grants from the Ellison Foundation (R.A.F.), National Institutes of Health Grant K08 AI065517 (F.S.S.), National Institutes of Health Grant K08 AI067736 (S.L.C.), National Institutes of Health Grant ES014871 (A.B.C.), and National Institutes of Health Grant ES015981 (A.B.C.). R.A.F. is an investigator of the Howard Hughes Medical Institute.
References
Articles from Proceedings of the National Academy of Sciences of the United States of America are provided here courtesy of National Academy of Sciences
Full text links
Read article at publisher's site: https://doi.org/10.1073/pnas.0803933105
Read article for free, from open access legal sources, via Unpaywall:
https://europepmc.org/articles/pmc2449360?pdf=render
Free after 6 months at www.pnas.org
http://www.pnas.org/cgi/content/abstract/105/26/9035
Free after 6 months at www.pnas.org
http://www.pnas.org/cgi/content/full/105/26/9035
Free after 6 months at www.pnas.org
http://www.pnas.org/cgi/reprint/105/26/9035.pdf
Citations & impact
Impact metrics
Citations of article over time
Alternative metrics

Discover the attention surrounding your research
https://www.altmetric.com/details/101817300
Smart citations by scite.ai
Explore citation contexts and check if this article has been
supported or disputed.
https://scite.ai/reports/10.1073/pnas.0803933105
Article citations
GLP-1R activation attenuates the progression of pulmonary fibrosis via disrupting NLRP3 inflammasome/PFKFB3-driven glycolysis interaction and histone lactylation.
J Transl Med, 22(1):954, 21 Oct 2024
Cited by: 0 articles | PMID: 39434134 | PMCID: PMC11492558
Gasdermin D and Gasdermin E Are Dispensable for Silica-Mediated IL-1β Secretion from Mouse Macrophages.
Immunohorizons, 8(9):679-687, 01 Sep 2024
Cited by: 0 articles | PMID: 39264735 | PMCID: PMC11447662
Implication of the LRR Domain in the Regulation and Activation of the NLRP3 Inflammasome.
Cells, 13(16):1365, 16 Aug 2024
Cited by: 0 articles | PMID: 39195255 | PMCID: PMC11352923
Review Free full text in Europe PMC
Pulmonary osteoclast-like cells in silica induced pulmonary fibrosis.
Sci Adv, 10(28):eadl4913, 10 Jul 2024
Cited by: 2 articles | PMID: 38985878 | PMCID: PMC11235167
Influence of metallic particles and TNF on the transcriptional regulation of NLRP3 inflammasome-associated genes in human osteoblasts.
Front Immunol, 15:1397432, 01 May 2024
Cited by: 0 articles | PMID: 38751427 | PMCID: PMC11094288
Go to all (542) article citations
Other citations
Wikipedia
Data
Similar Articles
To arrive at the top five similar articles we use a word-weighted algorithm to compare words from the Title and Abstract of each citation.
Silica crystals and aluminum salts activate the NALP3 inflammasome through phagosomal destabilization.
Nat Immunol, 9(8):847-856, 11 Jul 2008
Cited by: 1841 articles | PMID: 18604214 | PMCID: PMC2834784
Activation of the NALP3 inflammasome is triggered by low intracellular potassium concentration.
Cell Death Differ, 14(9):1583-1589, 29 Jun 2007
Cited by: 894 articles | PMID: 17599094
Pure Hemozoin is inflammatory in vivo and activates the NALP3 inflammasome via release of uric acid.
J Immunol, 183(8):5208-5220, 25 Sep 2009
Cited by: 113 articles | PMID: 19783673 | PMCID: PMC3612522
Silica-induced inflammasome activation in macrophages: role of ATP and P2X7 receptor.
Immunobiology, 220(9):1101-1106, 18 May 2015
Cited by: 29 articles | PMID: 26024943
Review
Funding
Funders who supported this work.
Howard Hughes Medical Institute
NHLBI NIH HHS (1)
Grant ID: T32 HL007974
NIAID NIH HHS (2)
Grant ID: K08 AI065517
Grant ID: K08 AI067736
NIAMS NIH HHS (1)
Grant ID: T32 AR007016
NIEHS NIH HHS (4)
Grant ID: R01 ES015981
Grant ID: ES014871
Grant ID: R01 ES014871
Grant ID: ES015981