Abstract
Free full text

Uropathogenic Escherichia coli CFT073 Is Adapted to Acetatogenic Growth but Does Not Require Acetate during Murine Urinary Tract Infection
§
Abstract
In vivo accumulation of d-serine by Escherichia coli CFT073 leads to elevated expression of PAP fimbriae and hemolysin by an unknown mechanism. Loss of d-serine catabolism by CFT073 leads to a competitive advantage during murine urinary tract infection (UTI), but loss of both d- and l-serine catabolism results in attenuation. Serine is the first amino acid to be consumed in closed tryptone broth cultures and precedes the production of acetyl phosphate, a high-energy molecule involved in intracellular signaling, and the eventual secretion of acetate. We propose that the colonization defect associated with the loss of serine catabolism is due to perturbations of acetate metabolism. CFT073 grows more rapidly on acetogenic substrates than does E. coli K-12 isolate MG1655. As shown by transcription microarray results, d-serine is catabolized into acetate via the phosphotransacetylase (pta) and acetate kinase (ackA) genes while downregulating expression of acetyl coenzyme A synthase (acs). CFT073 acs, which is unable to reclaim secreted acetate, colonized mouse bladders and kidneys in the murine model of UTI indistinguishably from the wild type. Both pta and ackA are involved in the maintenance of intracellular acetyl phosphate. CFT073 pta and ackA mutants were screened to investigate the role of acetyl phosphate in UTI pathogenesis. Both single mutants are at a competitive disadvantage relative to the wild type in the kidneys but normally colonize the bladder. CFT073 ackA pta was attenuated in both the bladder and the kidneys. Thus, we demonstrate that CFT073 is adapted to acetate metabolism as a result of requiring a proper cycling of the acetyl phosphate pathway for colonization of the upper urinary tract.
Urinary tract infections (UTIs) place a significant burden on the United States healthcare system, costing upwards of $2.4 billion per year (28). The majority of women will experience a UTI in their lifetime, and every year there are approximately 6.8 million physician visits, 1.2 million emergency room visits, a quarter million hospitalizations, and thousands of deaths due to complications of UTIs, most often sepsis (10, 18, 28, 47). Escherichia coli is the most commonly isolated causative agent of community-acquired UTIs (10).
The transition from residence in the gastrointestinal tract, where uropathogenic Escherichia coli (UPEC) transiently resides, to the urinary tract represents a significant change in environment. While the gastrointestinal tract is densely populated with many different species of bacteria, the bladder is normally a sterile environment yet one that presents significant challenges to bacterial growth. In addition to the cleansing flow of urine, numerous innate and acquired immune factors challenge the growth of UPEC in the urinary tract. The host defense involves phagocytic attack, antimicrobial peptides, complement lytic and opsonizing factors, and reactive oxygen and nitrogen species. In addition, the urinary tract as reflected in urine is limited in nutrients common to the intestinal tract, especially neutral sugars and iron (8). We hypothesize that UPEC adapted to take advantage of nutrients present in urine to more effectively colonize the urinary tract than the typical fecal E. coli strains, which rarely cause symptomatic UTIs.
From the standpoint of E. coli nutrition, urine is a dilute mixture of amino acids and small peptides, quite similar to tryptone broth with the notable exception of the abundance of urea in urine, a molecule that E. coli cannot utilize (8). The growth of E. coli in tryptone broth cultures is well characterized. Growing cells preferentially and sequentially utilize serine and then aspartate while secreting acetate. Once these two amino acids are depleted, cells then import and catabolize acetate and tryptophan, followed by alanine, glutamine, and threonine (1, 9, 40, 53, 54). This order of nutrient preference holds true for E. coli grown in broth cultures (9, 40, 53) as well as on semisolid motility agar (1, 54). UPEC growing in the urinary tract may encounter an environment more closely resembling that of a chemostat than that of a closed laboratory system. The production of urine from the kidneys provides a continuous supply of a preferred nutrient, such as serine, and hypothetically leads to production of the excreted product, acetate. One potential nutrient encountered by UPEC is d-serine, which is one of the most abundant amino acids found in human urine at concentrations between 3 and 115 μg/ml. The ratio of d- to l-serine enantomers in urine varies from human to human, but d-serine can range from as little as 18% to as much as 74% of the total serine (20). Serine can be catabolized into ammonia and pyruvate through enantomer-specific deaminases. SdaA and SdaB catabolize l-serine (48, 49), while DsdA will degrade d-serine (36).
Previous work in our laboratory focused on serine metabolism of extraintestinal pathogenic E. coli, using the urosepsis UPEC isolate, CFT073 (3, 19, 33, 44). We determined that approximately 85% of UPEC pyelonephritis and urosepsis isolates carry an intact d-serine deaminase operon (dsdCXA) (33). We have also observed that nearly all isolates of the common extraintestinal E. coli O18:K1:H7 pathotype possess two copies of the d-serine deaminase locus (33). This is in stark contrast to the roughly 5% of diarrheal isolates that are able to utilize d-serine (33). CFT073 with a dsdA mutation is at a competitive advantage relative to wild-type CFT073 in the murine model of UTI (44). Further studies demonstrate that multiple CFT073 mutants able to accumulate d-serine intracellularly are at a competitive advantage in the urinary tract (4). A CFT073 dsdA sdaA sdaB strain unable to utilize either enantiomer of serine is at a competitive disadvantage in the murine model (4). This suggests there is a role for serine as a key nutrient in vivo. We propose that this role is tied to acetate metabolism because serine is preferentially consumed by E. coli in a mixture of amino acids and can be metabolized to acetyl phosphate and then secreted as acetate (53).
Acetate metabolism is associated with expression of genes involved in pathogenesis (12, 15, 24, 25, 30, 38, 46, 53). Much interest involves the acetyl phosphate intermediary generated by the conversion of acetyl coenzyme A (acetyl-CoA) to acetyl phosphate by Pta (25, 30, 41, 46, 51, 53). Acetyl phosphate is degraded to acetate by AckA and secreted (25, 51, 53). Acs is used to consume the secreted acetate when nutrients become depleted (26, 53). Acetyl phosphate can serve as a phosphate donor to numerous two-component regulatory systems (15, 25, 41, 46, 51). In E. coli K-12, acetyl phosphate influences cell division (38, 39, 53), motility (15), type I fimbriae, biofilm formation (41), and some forms of the periplasmic stress response (15, 25, 38). We hypothesize that acetyl phosphate plays a role in wild-type colonization of the urinary tract and that ackA and pta mutants will lead to mouse urinary tract colonization defects.
In this study, we set out to determine if acetate metabolism has a fitness role for UPEC colonization and pathogenesis. l-Serine is preferentially consumed in tryptone broth and leads to acetogenic metabolism, but the ability to secrete acetate following d-serine catabolism has not been previously shown (1, 38, 53, 54). We demonstrate that CFT073 will upregulate the genes associated with acetate metabolism, including ackA, pta, and acs, following exposure to d-serine. Next we demonstrate that CFT073 grows more rapidly than MG1655 when utilizing acetogenic substrates d-serine, d-alanine, or pyruvate as a carbon source as well as when growing on acetate as a sole carbon source. A CFT073 acs strain colonizes the urinary tract indistinguishably from wild-type CFT073. Both CFT073 pta and CFT073 akcA colonize the bladder normally but are at a competitive disadvantage in the murine kidney. CFT073 ackA pta is attenuated in both the bladder and the kidney. Our results support a role for acetogenic products, acetyl phosphate and acetyl-CoA, but not acetate in colonization of the urinary tract by CFT073.
MATERIALS AND METHODS
Bacterial strains and growth conditions.
Urosepsis E. coli strain CFT073 was originally isolated from the blood and urine of a woman (32). WAM2880 is a derivative of CFT073 that is deleted for lacZYA (42). E. coli AAEC185 was used as a host for construction of recombinant plasmids (6). Other strains constructed for this study are described below, and a summary of all strains used can be seen in Table Table1.1. L broth and LB agar (Fisher) were used for the propagation of all strains except where noted below. For growth under defined conditions, morpholinepropanesulfonic acid (MOPS) minimal medium (35) was modified with the substitution of the indicated carbon source for glucose and the omission of thiamine. Carbenicillin, kanamycin, and chloramphenicol were used at concentrations of 250 μg/ml, 50 μg/ml, and 20 μg/ml, respectively.
TABLE 1.
Strains used in the study
Strain | Description | Reference or source |
---|---|---|
2267 | CFT073 | 32 |
2603 | AAEC185 | 6 |
2625 | K-12 MG1655 | Gift of Ian C. Blomfield |
2684 | Contains plasmid pKD3 | Gift of Barry L. Wanner (11) |
2685 | Contains plasmid pKD4 | Gift of Barry L. Wanner (11) |
2687 | Contains plasmid pKD46 | Gift of Barry L. Wanner (11) |
2688 | Contains plasmid pCP20 | Gift of Barry L. Wanner (11) |
2850 | CFT073 + pKD46 | 42 |
2880 | CFT073 lacZYA | 42 |
3829 | CFT073 ackA | This study |
3832 | CFT073 pta | This study |
3833 | CFT073 ackA pta | This study |
3886 | AAEC185 + packA | This study |
3887 | AAEC185 + ppta | This study |
3888 | CFT073 ackA + packA | This study |
3889 | CFT073 pta + ppta | This study |
3981 | CFT073 acs | This study |
3992 | CFT073 ackA pta + packA | This study |
3993 | CFT073 ackA pta + ppta | This study |
4008 | CFT073 + packA | This study |
4009 | CFT073 + ppta | This study |
RNA isolation, cDNA synthesis, and cDNA labeling.
Wild-type CFT073 was grown overnight in MOPS minimal medium with 4% glycerol as the carbon source. Six replicate cultures were then grown aerobically at 37°C to mid-logarithmic phase and treated with either d-serine (Sigma) to a final concentration of 500 μg/ml or an equal volume of water. Bacterial samples were taken from each culture immediately and 30 min after the treatment, stabilized with RNAprotect bacterial reagent (Qiagen), pelleted, and frozen at −80°C as recommended by the manufacturer. RNA was isolated from these pellets, and DNase was treated using the Epicentre MasterPure RNA kit (Epicentre). The RNA samples were then reverse transcribed to cDNA as previously described (19). Briefly, 10 μg of RNA was mixed with 750 ng of random hexamers (Invitrogen) and cDNA was generated by reverse transcription with SuperScript II reverse transcriptase (1,500 U; Invitrogen). First Strand buffer, dithiothreitol, and deoxyribonucleotide were used at concentrations recommended by the manufacturer (Invitrogen). The reactions were incubated at 25°C for 10 min, 37°C for 60 min, 42°C for 60 min, and 70° for 10 min. Following RNase H (Invitrogen) and RNase A (Ambion) digestion for 1 h at 37°C, cDNA was purified with a QIAquick PCR purification kit (Qiagen) according to the QIAquick Spin handbook. Samples (3 μg) of cDNA were fragmented using RQ1 DNase I (Promega) and then 3′ labeled with biotin-N6-ddATP (Perkin-Elmer Life Sciences) using terminal transferase (Roche) as described previously (19).
Microarray hybridization.
The E. coli CFT073-specific DNA microarray (NimbleGen Systems, Inc.) includes 5,611 open reading frames (ORFs) and stable RNAs from prerelease version 17 of the compiled CFT073 genome sequence (from this lab; available upon request). Each ORF is represented on the glass slide by 17 unique probe pairs of 24-mer, in situ-synthesized oligonucleotides. Each pair consists of a sequence perfectly matched to the ORF and another adjacent sequence that harbors two mismatched bases for determination of background and cross-hybridization. Labeled cDNA samples were individually hybridized to the CFT073-specific microarray according to the NimbleGen standard operating procedure. Following washes and labeling with a streptavidin-Cy3 complex according to the NimbleGen procedure, microarrays were scanned at a 5-μm resolution using a GenePix 4000B scanner.
Microarray data analysis and statistical methods.
Data were extracted using NimbleScan (NimbleGen), and an algorithm (courtesy of Yu Qiu, University of Wisconsin School of Medicine) was applied to obtain a measurement of signal intensity for each ORF as well as a call for a transcript's presence or absence. Data were normalized and converted to estimates of transcript abundance, using the total signal intensity, to allow comparison of individual microarrays (2). A P value for each ORF was calculated by a two-tailed Welch's unpaired t test comparison of the three microarray replicates for each strain. Fold changes of an ORF between strains were calculated using the following ratio: average CFT073 dsdA signal intensity/average wild-type CFT073 signal intensity. Only ORFs that had at least twofold changes, a P value of less than or equal to 0.05, and were called present on at least two microarrays were considered significant.
Growth comparisons.
Strains were grown on different carbon sources in a similar manner as that previously described (29). The following carbon source concentrations for MOPS minimal medium were used to monitor growth on a defined carbon source and normalized to 215 μM glycerol: 210 μM d-serine, 210 μM d-alanine, and 315 μM sodium acetate. Sodium pyruvate was used at a concentration of 315 μM. Overnight broth cultures were used to inoculate cultures to the growth curve of 1:50 or to an optical density at 600 nm (OD600) of ~0.02. OD600 was measured hourly with a GENESYS 20 spectrophotometer (Thermo). Time points were graphed and subjected to a two-way analysis of variance with a Bonferroni correction using Prism 4.0c (GraphPad) to determine statistically significant differences in growth of the cultures. Doubling time was estimated with the nonlinear curve fit calculation in Prism 4.0c (GraphPad).
Construction of CFT073 mutants by λ-Red recombination.
The construction of CFT073 ackA, CFT073 pta, CFT073 ackA pta, and CFT073 acs was accomplished by using the λ-Red recombination system designed by Datsenko and Wanner (11). Oligonucleotides were designed for creation of the desired mutants as described previously (11) and were purchased from IDT and shown in Table Table2.2. After each gene was replaced with an antibiotic resistance cassette, the cassettes were excised by Flp recombinase, encoded by pCP20, leaving a single FLP recombination target site as described previously (11). Excision of the antibiotic resistance cassettes was confirmed by PCR and loss of antibiotic resistance on the appropriate selective medium.
TABLE 2.
Oligonucleotides used in this study
Name | No. | Nucleotide sequencea | Use |
---|---|---|---|
ackA P0 | 1766 | CCCTGACGTTTTTTTAGCCACGTATCAATTATAGGTACTTCCtgtgtaggctggagctgcttc | ackA and ackA pta strain |
ackA P2 | 1767 | GTTACAAAACAGCACCGCCAGCTGAGCTGGCGGTGTGAAAgcatatgaatatcctcctaag | ackA strain |
ackA 3′ ApaLI | 1806 | gtcagtgcacTCAGGCAGTCAGGCGGCTCGCG | Addition of 3′ ApaLI site to ackA for cloning |
AatII ackA 5′ | 1807 | gacctcgacgtcATGTCGAGTAAGTTAGTACTGG | Addition of 5′ AattII site to ackA for cloning |
pta P0 | 1764 | GTAACCCGCCAAATCGGCGGTAACGAAAGAGGATAAACCtgtgaggctggagctgcttc | pta strain |
pta P2 | 1768 | GGTTCAGATATCCGCAGCGCAAAGCTGCGGATGATGACGAGAgcatatgaatatcctcctaag | ackA and ackA pta strain |
pta 3′ ApaLI | 1808 | ctatgcgtgcacTACTGCTGCTGTGCAGACTGAA | Addition of 3′ ApaLI site to pta for cloning |
AattII pta 5′ | 1809 | cggccggacgtcGTGTCCCGTATTATTATGCTG | Addition of 5′ AattII site to pta for cloning |
acs P0 | 1889 | CTTATGCCACATATTATTAACATCTTACAAGGAGAACAAAAGC tgtgaggctggagctgcttc | acs strain |
acs P2 | 1890 | CGTTTCCGACTTGTTGCCCGGAGCGCATCCGGCAATTGTGGGatatgaatatcctcctaag | acs strain |
Murine model of UTI.
Urinary tract colonization was assayed using the competitive murine model of UTI as previously described (42). CFT073 lacZYA (WAM2880) was used as the wild type to allow discrimination of (lacZ+) mutants as pink colonies and WAM2880 as white colonies on MacConkey lactose agar medium. This strain colonizes mouse bladders and kidneys indistinguishably from the wild type (42). Strains were grown independently in L broth without shaking at 37°C for 7 days, with passage to fresh medium on the second, fourth, and sixth days. Cultures of wild-type or mutant bacteria were concentrated by centrifugation at 7,500 rpm in an Eppendorf 5215D centrifuge for 2 min, resuspended in phosphate-buffered saline, and then mixed in a 1:1 ratio. Inocula of 50 μl, carrying between 1 × 108 and 5 × 108 CFU, were delivered via a catheter to the bladders of isoflurane-anesthetized Swiss-Webster mice (Harlan). After 2 days, mice were euthanized by CO2 asphyxiation, and the bladders and kidneys were excised, homogenized, serially diluted in phosphate-buffered saline and plated to MacConkey lactose agar medium. When tested strains carried plasmids for complementation studies, the medium contained antibiotics at the proper concentration to select for bacteria that maintained the plasmids. After enumeration, the competitive index (ratio of recovered CFU of mutant/recovered CFU of the wild type) colonization levels were graphed using Prism 4.0c (GraphPad) and the numbers of wild-type and mutant CFU were compared using a paired Wilcoxon signed rank test in Prism 4.0c (GraphPad). Strains that showed statistically significant levels of colonization compared to wild-type CFT073 were tested for type I and P fimbriae and hemolysin expression by standard methods (42).
RESULTS
The response of CFT073 to d-serine in minimal media.
Our laboratory previously demonstrated a role for both d- and l-serine (19) catabolism in urinary tract colonization. We postulate that serine catabolism required for wild-type colonization of the urinary tract is important because degradation of l- or d-serine generates acetyl phosphate, which acts as a major phosphate donor to two-component regulatory systems involved in colonization of the urinary tract (25, 53). E. coli generates acetyl phosphate and subsequently secretes acetate following consumption of l-serine (1, 40, 53, 54), but a similar response following consumption of d-serine has not been previously demonstrated. We examined the response of CFT073 grown in MOPS glycerol following the addition of d-serine in order to determine if d-serine induces acetate metabolism in a similar fashion to the manner in which E. coli K-12 will respond to l-serine.
Thirty seconds after the addition of d-serine to CFT073 cells in exponential growth phase in a MOPS glycerol culture, the most highly upregulated genes were the d-serine-responsive gene dsdX and glycerate metabolism gene garP (data not shown). Thirty minutes postaddition, the genes coding for pyruvate dehydrogenase (aceEF), NADH dehydrogenase II (ndh), phosphotransacetylase (pta), and acetate kinase (ackA) were among the most highly upregulated genes, as shown in Table Table3.3. Several genes were downregulated, notably the gene encoding acetyl-coA synthase (acs) (see Table S1 in the supplemental material), suggesting dissimilatory acetate metabolism in the presence of d-serine, consistent with acetyl phosphate accumulation (53). Additionally, genes coding for glycerol metabolism (glpQTA and glpKF) were downregulated, suggesting d-serine is a preferred carbon source to glycerol. The catabolic breakdown of d-serine by DsdA generates pyruvate, which in turn leads to the production of acetyl phosphate, acetyl-CoA, and acetate.
TABLE 3.
Genes upregulated in the d-serine-treated sample at 30 mina
Function | Gene name | Product | Fold change at 30 min (P value) |
---|---|---|---|
Carbon metabolism and energy generation | aceE | Pyruvate dehydrogenase | 4.2 (P < 0.001) |
aceF | Pyruvate dehydrogenase | 3.4 (P < 0.001) | |
dsdX | d-Serine transporter | 15 (P < 0.0005) | |
dsdA | d-Serine deaminase | 15 (P < 0.0005) | |
ndh | NADH dehydrogenase II | 3.9 (P < 0.005) | |
mqo (yojH) | Malate quinone oxidoreductase | 3.8 (P < 0.05) | |
ackA | Acetate kinase | 3.9 (P < 0.005) | |
pta | Phosphotransacetylase | 2.3 (P < 0.05) | |
garL | Glucarate, galactarate, glycerate metabolism | 3.6 (P < 0.001) | |
garR | Glucarate, galactarate, glycerate metabolism | 2.7 (P < 0.001 | |
yfiD | Pyruvate formate lyase accessory protein | 3 (P < 0.005) | |
Methionine synthesis | metE | Tetrahydropteroyltriglutamate methyltransferase | 2.8 (P < 0.001) |
metB | Cystathionine gamma-synthase | 3.6 (P < 0.001) | |
metL | Aspartokinase II and homoserine dehydrogenase II | 2.1 (P < 0.001) | |
metF | 5,10-Methylenetetrahydrofolate reductase | 3.4 (P < 0.001) | |
sbp | Periplasmic sulfate-binding protein | 3.0 (P < 0.001) | |
Unknown | c3104 | Adjacent to yfiD | 2.5 (P < 0.05) |
c0143 | Intergenic to aceE and aceF | 3.6 (P < 0.005) | |
c4752 | Adjacent to metE | 3.2 (P < 0.001) |
E. coli CFT073 grows more rapidly on acetogenic substrates than E. coli MG1655.
Urine is analogous to tryptone broth insofar as both are poor sources of free carbohydrate but contain high levels of peptides and amino acids (8). When growing in tryptone broth, E. coli bacteria consume amino acids in a specific order, starting with serine, and favor production of acetate during early-log-phase growth (1). The variable nature of urine contents makes generalized statements difficult to test practically, but we assume that serine is the first amino acid consumed. Catabolism of either enantiomer of serine leads to acetogenic growth. The acetate metabolism would follow trends similar to those in tryptone broth, including an initial intracellular accumulation of acetyl phosphate, followed by secretion of acetate. If acetate metabolism is important for urinary tract colonization and disease pathogenesis, then it is logical to hypothesize that a uropathogen such as CFT073 will be better adapted for growth on acetogenic substrates than MG1655, a model K-12 fecal isolate. Growth experiments were conducted in MOPS broth with the indicated carbon source shown in Fig. Fig.1.1. Interestingly, CFT073 and MG1655 show similar growth kinetics when growing on d-serine (Fig. (Fig.1a),1a), though CFT073 does reach stationary phase slightly sooner than MG1655. The similarity of growth between the strains is not surprising as the nucleotide sequence of the dsdCXA locus is highly conserved between the two strains. CFT073 and MG1655 show markedly different growth rates when grown on sodium pyruvate as a sole carbon source, as shown in Fig. Fig.1b.1b. These data suggest that DsdA enzymatic activity is the rate-limiting step for growth on d-serine and that the subsequent utilization of pyruvate in acetogenic metabolism or in the tricarboxylic acid cycle occurs relatively rapidly. The reason for the difference of growth rate between the two strains on pyruvate is not entirely clear. In the published genome sequence of CFT073, aceF is not counted as present due to ambiguous sequence reads across this region and an apparent nucleotide insertion, but based on our resequencing of the CFT073 genome from base pairs 136525 through 136625, aceF in CFT073 appears identical to the MG1655 allele (data not shown). The difference in growth rates cannot be readily explained by functional differences between the pyruvate dehydrogenase complex of CFT073 and that of MG1655 because the amino acid sequences of AceE, AceF, and LpdA that make up the complex are nearly identical with only conservative substitutions present. Additionally, key acetate metabolism genes acs, pta, and ackA and proximal genes appear to be nearly identical in sequence in CFT073 and MG1655.

Growth of CFT073 and MG1655 in MOPS medium cultures supplemented with different carbon sources: d-serine (a), pyruvate (b), d-alanine (c), and acetate (d). The times indicated below the strain names on the legends are the estimated doubling times based on the observed growth rates. The solid arrow in each graph indicates the time point at which the OD600 was at a statistically significant difference (P < 0.05) as determined by the Bonferroni posttest. In panel a, the dashed line indicates where the OD600s of the two cultures became statistically indistinguishable. All experiments were conducted a minimum of three times.
When grown on d-alanine as a sole carbon source, CFT073 grows significantly more rapidly than MG1655 as shown in Fig. Fig.1c.1c. CFT073 also grows more rapidly on sodium acetate as a sole carbon source than does MG1655 as shown in Fig. Fig.1d.1d. These data show that in general, CFT073 is better suited for rapid growth on certain acetogenic substrates as well as acetate than MG1655.
Recycling of acetate via Acs is not necessary for colonization of the urinary tract.
The enhanced ability of CFT073 to utilize acetate and acetogenic compounds in vitro may reflect a role for acetate catabolism in vivo during the course of a UTI. To investigate the role of acetate scavenging in vivo, a CFT073 acs strain was screened in coinfection with the wild type in the murine model of UTI. CFT073 acs was not attenuated in the murine urinary tract as depicted in Fig. Fig.2.2. Conversion of high concentrations of acetate to acetyl-CoA mediated by Acs is not required for normal colonization of the urinary tract.

Bladder and kidney colonization of mice with wild-type (wt) and CFT073 acs. Mice were transurethrally inoculated with a 1:1 ratio of CFT073 lacZYA and CFT073 acs. Each data point represents the competitive index that is the ratio of recovered mutant CFU/g tissue to recovered wild-type CFU/g tissue for the designated organ tissue from one mouse 48 h postinoculation. Horizontal bars represent the median value of ratios for the organ type, which are 0.681 for the bladder (P < 0.008) and 0.483 for the kidney (P < 0.305). The dashed line indicates a competitive index of 1.0.
E. coli CFT073 requires pta and ackA for wild-type colonization of the murine urinary tract.
We hypothesize that acetyl phosphate is needed for colonization of the urinary tract. Acetyl phosphate acts as a phosphate donor to many two-component regulatory systems that regulate type 1 fimbria expression, swimming motility, cell division, and the stress response in E. coli K-12 (25, 53). Many, if not all, of these responses facilitated by acetyl phosphate would influence the outcome of E. coli UTIs. CFT073 lacks some of the genes present in K-12 that respond to acetyl phosphate, such as fimZ (52, 53), so it is not obvious if CFT073 will show altered colonization if acetyl phosphate metabolism is perturbed.
To determine if acetyl phosphate plays a role in UTI pathogenesis, pta, ackA, and ackA pta mutants were screened in mice with coinfections with wild-type CFT073. When grown in tryptone broth, the mutants shown in Fig. Fig.33 have a slightly reduced doubling rate of an hour per doubling (31) compared to CFT073, which doubles approximately every 50 min. The pta mutant was attenuated in the kidneys in a coinfection with wild-type CFT073 as shown in Fig. Fig.4a.4a. Similarly, the ackA mutant was attenuated in the kidneys in a coinfection with the wild type as shown in Fig. Fig.4b.4b. Both single-deletion mutants colonized the bladder to a similar degree, as did the wild-type strain. The ackA pta double mutant was attenuated in both the bladder and the kidneys in a coinfection with the wild type as shown in Fig. Fig.4c.4c. CFT073 pta, ackA, and pta ackA were indistinguishable from wild-type CFT073 in terms of expression of type I fimbriae, P fimbriae, and hemolysin (data not shown). Thus, we demonstrate that CFT073 requires acetyl phosphate metabolism in order to colonize the urinary tract to wild-type levels.

Growth of indicated strains in tryptone broth. Plasmid-complemented strains show growth similar to that of the wild-type CFT073. No antibiotic was used for the maintenance of the complementing plasmids. Complemented strains were checked for retention of plasmids by plasmid extraction with a QIAprep spin miniprep kit (Qiagen) at the end of the experiment. All complemented strains retained plasmids for the duration of the experiment without the use of antibiotic selective pressure.
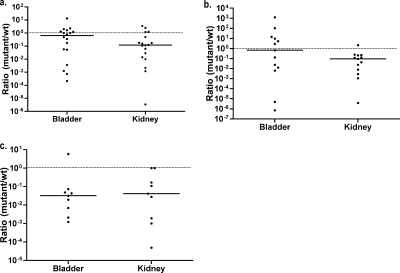
Mice were transurethrally inoculated with a 1:1 ratio of CFT073 lacZYA and CFT073 pta (a), CFT073 lacZYA and CFT073 ackA (b), or CFT073 lacZYA and CFT073 ackA pta (c). Each data point represents the competitive index that is the ratio of recovered mutant CFU/g tissue to recovered wild-type (wt) CFU/g tissue for the designated organ tissue from one mouse 48 h postinoculation. Horizontal bars represent the median value of ratios for the organ type, which were equal to 0.636 (P = 0.232) and 0.120 (P = 0.021) (a), 0.670 (P = 0.787) and 0.092 (P = 0.007) (b), and 0.032 (P = 0.039) and 0.042 (P = 0.016) (c) for the bladder and kidney, respectively. The dashed line indicates a competitive index of 1.0.
DISCUSSION
We have previously shown that d-serine is able to act as an intracellular signal, enhancing urinary tract colonization, but it also can serve as a key nutrient in the absence of l-serine catabolic potential (4). As shown in this work and in that of other laboratories, the catabolism of serine leads to the generation of acetyl phosphate (40, 53), among other compounds, which aids signal transduction via several two-component regulatory systems (25, 46, 51, 53). To examine how acetate metabolism may be tied to both d-serine metabolism and UTI pathogenesis, we first examined the effects that d-serine addition has on gene expression levels in UPEC CFT073. In addition to a significant upregulation of the d-serine-responsive genes dsdX and dsdA, genes responsible for pyruvate metabolism as well as key genes in acetate metabolism, including ackA and pta, were upregulated 30 min following the addition of d-serine to minimal medium while acs was downregulated.
Our laboratory demonstrated that UPEC CFT073 upregulates d-serine-responsive genes in the murine urinary tract (19), and Roos and Klemm (45) showed that asymptomatic-bacteriuria strain 83972 significantly upregulates the d-serine deaminase gene, dsdA, in the human urinary tract. These observations when paired with our current observations that d-serine is degraded to acetate by CFT073 suggest UPEC can readily generate acetyl phosphate in vivo while in the urinary tract. The consumption of the relatively plentiful nutrient d-serine within the urinary tract may lead CFT073 to be better adapted to acetate metabolism than E. coli strains lacking the d-serine deaminase locus. To further investigate the hypothesis that UPEC and specifically CFT073 are adapted for growth on acetogenic substrates, we examined the growth of CFT073 and MG1655 on different relevant carbon sources. Both strains have similar doubling rates with d-serine as a sole carbon source, but interestingly, CFT073 grew much more readily and rapidly than MG1655 when utilizing d-alanine, pyruvate, or acetate as a sole carbon source. The concentration of either acetate or pyruvate in urine is unknown; however, d-alanine is present at concentrations that approach the levels of d-serine (20, 37). The more-rapid growth rate of CFT073 on these carbon sources suggests, when combined with previous observations, that efficient acetogenic carbon metabolism is an adaptive response by CFT073 which permits it to readily exploit the nutritional niche of the urinary tract.
When grown in tryptone broth, E. coli first consumes the serine present in the medium (39, 46, 53). Concurrent with the utilization of serine, there is an increase in the intracellular concentration of acetyl phosphate. Acetyl phosphate levels decrease, following depletion of serine, as the acetyl phosphate is secreted as acetate (53). Similar events occur when E. coli is grown on other acetogenic carbon sources (53). The intracellular concentration of acetyl phosphate is approximately 3 mM, similar in magnitude to that of ATP (25). Acetyl phosphate can act as a global signal by serving as a phosphate donor to two-component regulatory systems due to the high-energy phosphate bond (25, 30, 38, 46, 53). The events influenced by acetyl phosphate are of relevance to the study of pathogenesis as acetyl phosphate directly phosphorylates OmpR (38, 40, 46), RssB (7), RcsB (15), NtrC (13), and other (53) response regulators involved in the periplasmic stress response and in the regulation of virulence factors (25, 53).
We demonstrate that assimilatory acetate metabolism mediated by Acs is not required for wild-type colonization of the urinary tract by CFT073. The CFT073 acs mutant colonizes the murine urinary tract indistinguishably from wild-type CFT073. Therefore, Acs-mediated reclamation of the acetate secreted following active serine catabolism is not an important metabolic event during murine urinary tract colonization. The CFT073 acs mutant results also suggest that UPEC bacteria are growing rapidly on acetogenic carbon sources, like serine and alanine, and probably do not come close to reaching stationary phase while colonizing the urinary tract.
Unlike assimilatory acetate metabolism, dissimilatory acetate metabolism does play a role in colonization of the murine urinary tract. We demonstrate that mutations that affect the normal balance of acetyl-CoA and acetyl phosphate negatively affect the ability of CFT073 to colonize the murine urinary tract. CFT073 ackA and CFT073 pta showed an inability to properly colonize the kidney while CFT073 ackA pta was impaired in its ability to colonize both the bladder and the kidney. These data clearly show that the components of dissimilatory acetate metabolism are necessary for wild-type colonization of the murine urinary tract and suggest that maintenance of a proper intracellular acetyl phosphate concentration is also important.
Intracellular acetyl phosphate concentrations peak early in log phase, concurrent with depletion of serine (39, 53). Our interest in intracellular acetyl phosphate extends beyond its ties to growth phase. We are interested in intracellular acetyl phosphate because acetyl phosphate is able to directly phosphorylate response regulators involved in the periplasmic stress response (25, 50, 53), which is vital for wild-type colonization of the urinary tract (42, 43). We have observed a loss of ability to colonize the murine urinary tract associated with an inability to deaminate l- and d-serine (4). When these observations are paired with our previous observations of the importance of the periplasmic stress response in colonization of the mouse model (42, 43), the alterations in colonization associated with the dissimilatory acetate metabolism mutants seem likely due to an inability to accumulate acetyl phosphate, an inappropriate acetyl-CoA level, or an altered stress response.
Acetate metabolism is an important factor for many other microbial pathogens and is proving to be a conserved and useful signaling molecule. In Salmonella enterica serovar Typhimurium, a pta mutant is attenuated in the mouse model of peritonitis (24). Both ackA and pta are upregulated in vivo in Pasteurella multocida (22). A probable role for acetyl phosphate metabolism in extraintestinal, gram-negative pathogenesis is in the indirect effect acetyl phosphate has on the histone-like protein H-NS (21). H-NS is capable of repressing numerous processes, often associated with horizontally acquired DNA that plays a role in pathogenesis (5, 14, 16, 17, 23, 27, 34). UPEC 536 hns upregulated numerous virulence factors (34) similarly to a CFT073 mutant that colonizes the murine urinary tract in a robust fashion (19). Interestingly, OmpR-P is able to overcome H-NS repression of ompR expression (5). OmpR can be phosphorylated independently of EnvZ (25, 39, 40, 46, 51, 53), its cognate sensor kinase, in the presence of acetyl phosphate. Acetyl phosphate is therefore able to relieve some of the effects of H-NS repression (5). We propose that mutants in the ackA-pta pathway are attenuated in the urinary tract due to an inability to maintain proper intracellular acetyl phosphate concentrations, which leads to reduced derepression of H-NS. We are currently investigating the role of EnvZ-independent phosphorylation of OmpR in UTI pathogenesis.
Acknowledgments
We thank Laura Walters, Holly Hamilton, Shahaireen Pellett, Rose Szabady, and Gary Baisa for help with experiments and manuscript preparation.
This research was supported by NIH grant R01DK063250. B.J.H. was also supported by NIH National Research Service Award T32 GM07215.
Footnotes
Published ahead of print on 6 October 2008.
§Supplemental material for this article may be found at http://iai.asm.org/.
REFERENCES
Articles from Infection and Immunity are provided here courtesy of American Society for Microbiology (ASM)
Full text links
Read article at publisher's site: https://doi.org/10.1128/iai.00618-08
Read article for free, from open access legal sources, via Unpaywall:
https://iai.asm.org/content/76/12/5760.full.pdf
Free after 4 months at iai.asm.org
http://iai.asm.org/cgi/content/full/76/12/5760
Free after 4 months at iai.asm.org
http://iai.asm.org/cgi/reprint/76/12/5760
Free to read at iai.asm.org
http://iai.asm.org/cgi/content/abstract/76/12/5760
Citations & impact
Impact metrics
Citations of article over time
Article citations
The Role of Metabolomics and Microbiology in Urinary Tract Infection.
Int J Mol Sci, 25(6):3134, 08 Mar 2024
Cited by: 0 articles | PMID: 38542107 | PMCID: PMC10969911
Review Free full text in Europe PMC
The distinct transcriptome of virulence-associated phylogenetic group B2 Escherichia coli.
Microbiol Spectr, e0208523, 19 Sep 2023
Cited by: 2 articles | PMID: 37724859 | PMCID: PMC10580932
Bacteria-Infected Artificial Urine Characterization Based on a Combined Approach Using an Electronic Tongue Complemented with 1H-NMR and Flow Cytometry.
Biosensors (Basel), 13(10):916, 05 Oct 2023
Cited by: 1 article | PMID: 37887109 | PMCID: PMC10605348
Evaluation of Antimicrobial Activities against Various E. coli Strains of a Novel Hybrid Peptide-LENART01.
Molecules, 28(13):4955, 23 Jun 2023
Cited by: 3 articles | PMID: 37446618
Inactivation of ackA and pta Genes Reduces GlpT Expression and Susceptibility to Fosfomycin in Escherichia coli.
Microbiol Spectr, 11(3):e0506922, 18 May 2023
Cited by: 2 articles | PMID: 37199605 | PMCID: PMC10269713
Go to all (30) article citations
Data
Data behind the article
This data has been text mined from the article, or deposited into data resources.
BioStudies: supplemental material and supporting data
Similar Articles
To arrive at the top five similar articles we use a word-weighted algorithm to compare words from the Title and Abstract of each citation.
In vivo gene expression analysis identifies genes required for enhanced colonization of the mouse urinary tract by uropathogenic Escherichia coli strain CFT073 dsdA.
Infect Immun, 75(1):278-289, 30 Oct 2006
Cited by: 57 articles | PMID: 17074858 | PMCID: PMC1828413
Roles of serine accumulation and catabolism in the colonization of the murine urinary tract by Escherichia coli CFT073.
Infect Immun, 75(11):5298-5304, 04 Sep 2007
Cited by: 52 articles | PMID: 17785472 | PMCID: PMC2168303
dsdA Does Not Affect Colonization of the Murine Urinary Tract by Escherichia coli CFT073.
PLoS One, 10(9):e0138121, 14 Sep 2015
Cited by: 13 articles | PMID: 26366567 | PMCID: PMC4569052
Bacterial characteristics of importance for recurrent urinary tract infections caused by Escherichia coli.
Dan Med Bull, 58(4):B4187, 01 Apr 2011
Cited by: 85 articles | PMID: 21466767
Review
Funding
Funders who supported this work.
NIDDK NIH HHS (2)
Grant ID: R01 DK063250
Grant ID: R01DK063250
NIGMS NIH HHS (2)
Grant ID: T32 GM07215
Grant ID: T32 GM007215