Abstract
Purpose
Massive macronodular adrenocortical disease (MMAD) may be caused by aberrant microRNA expression. To determine the microRNA profile in MMAD and identify putative microRNA-gene target pairs involved in adrenal tumourigenesis.Experimental design
We performed microRNA microarray analysis in 10 patients with ACTH-independent Cushing syndrome caused by MMAD (ages 39-60 years) and four normal adrenal cortex samples were used as controls. Microarray data were validated by real-time polymerase chain reaction (qRT-PCR). Identification of potential microRNA-gene target pairs implicated in MMAD pathogenesis has been performed by integrating our microRNA data with previously obtained cDNA microarray data. Experimental validation of specific microRNA gene targets was performed by transfection experiments and luciferase assay.Results
A total of 37 microRNAs were differentially expressed between MMAD and normal tissues; 16 microRNAs were down-regulated, including miR-200b and miR-203, whereas 21 microRNAs were up-regulated, miR-210 and miR-484 among them. Comparison of microRNA data with different clinicopathological parameters revealed miR-130a and miR-382 as putative diagnostic MMAD markers. Interestingly, we detected miR-200b targeting directly Matrin 3 (MATR3) expression in an adrenocortical cancer cell line (H295R).Conclusions
MicroRNAs appear to have distinct regulatory effects in MMAD, including an association with clinical presentation and severity of the disease, expressed by the degree of hypercortisolism. This is the first investigation of microRNAs in MMAD, a disease with complex pathogenesis; the data indicate that specific microRNAs such as miR-200b may play a significant role in MMAD formation and/or progression.Free full text

MicroRNA signature in massive macronodular adrenocortical disease and implications for adrenocortical tumorigenesis
Abstract
Purpose
Massive macronodular adrenocortical disease (MMAD) may be caused by aberrant microRNA expression. To determine the microRNA profile in MMAD and identify putative microRNA-gene target pairs involved in adrenal tumorigenesis.
Experimental design
We performed microRNA microarray analysis in 10 patients with ACTH-independent Cushing syndrome caused by MMAD (ages 39 - 60 years) and 4 normal adrenal cortex samples were used as controls. Microarray data were validated by real-time polymerase chain reaction (qRT-PCR). Identification of potential microRNA-gene target pairs implicated in MMAD pathogenesis has been done by integrating our microRNA data with previously obtained cDNA microarray data. Experimental validation of specific microRNA gene targets was performed by transfection experiments and luciferase assay.
Results
A total of 37 microRNAs were differentially expressed between MMAD and normal tissues; 16 microRNAs were down-regulated, including miR-200b and miR-203, while 21 microRNAs were up-regulated, miR-210 and miR-484 among them. Comparison of microRNA data with different clinicopathological parameters revealed miR-130a and miR-382 as putative diagnostic MMAD markers. Interestingly, we detected miR-200b targeting directly Matrin 3 (MATR3) expression in an adrenocortical cancer cell line (H295R).
Conclusions
MicroRNAs appear to have distinct regulatory effects in MMAD, including an association with clinical presentation and severity of the disease, expressed by the degree of hypercortisolism. This is the first investigation of microRNAs in MMAD, a disease with complex pathogenesis; the data indicate that specific microRNAs such as miR-200b may play a significant role in MMAD formation and/or progression.
Introduction
ACTH-independent Cushing syndrome (CS) may be caused by several types of bilateral adrenal hyperplasias (BAHs), including primary pigmented nodular adrenocortical disease (PPNAD) and massive macronodular adrenocortical disease (MMAD) or ACTH-independent macronodular adrenal hyperplasia (AIMAH) (1). Unlike the case with PPNAD, most patients with MMAD do not have family history of this disease and present with CS in middle age or even late adulthood (2). Up to date only few studies have molecular data regarding MMAD pathogenesis. Aberrant expression of G protein-coupled receptors (GPCRs) has been identified as the main molecular mechanism that underlies the cause of MMAD in the majority of the cases (3).
Somatic mutations of the GNAS gene are present in patients with McCune-Albright syndrome (MAS) and CS due to BAH (4); in addition, a number of patients with MMAD were found to harbor GNAS mutations in their adrenocortical tissue (5). In these cases, aberrant GPCR expression may be due to activation of the protein kinase A (PKA) signaling pathway by increased cAMP levels (6); in most patients with MMAD the underlying cause of this phenomenon remains a mystery, as sated above. Our laboratory reported several genes that may play a role, following an expression microarray analysis of tissues affected by MMAD (7).
MicroRNAs are non coding RNA sequences that have recently been identified as important regulators of target gene expression at the mRNA or protein level: if there is partial complementarity between the sequence of a given microRNA and that of its target gene, then the microRNA binds in the 3’UTR of the gene and regulates translation; if, on the other hand, the sequences of a microRNA and the target gene are perfectly complementary, then regulation takes place at the target gene's mRNA level resulting usually in cleavage and inhibition of transcription (8-10). The precursor molecules of microRNAs (pri-miRNAs) are transcribed by nuclear polymerase II and are then processed into pre-miRNAs, by the enzymatic complex Drosha-DGCR8. Pre-miRNAs are 60 to 70 nucleotide sequences that form a hairpine structure and are transported from the nucleus into the cytoplasm through exportin 5 (9). Mature miRNAs are then generated after the cleavage of pre-miRNAs by Dicer, another RNase III (10). MicroRNAs exhibit their effects into the RISC complexes (RNA-induced silencing complex) where the mRNA target is suppressed (9). MicroRNAs exhibit a critical role in multiple cellular functions, such as growth and proliferation, cell cycle control, and apoptosis (10). Consequently, microRNAs have been implicated in the pathogenesis or progression of several diseases, including many types of cancer (11-16), where they can act as tumor suppressors or oncogenes. Although widespread aberrations are indicated in different cancer types, it is well documented that several microRNAs play very specific roles in tumorigenesis regulating certain pathways (10-16).
The present study is the first one that attempts to investigate the role of microRNAs in MMAD; we selected to study MMAD for the role of microRNAs because of its largely unknown primary molecular etiology, the availability of a complete and recent set of cDNA microarray data, and our access to several samples from patients with this disease. In this study we have identified an MMAD microRNA gene signature and have associated miR-130a and miR-382 expression with the severity of the disease. We found a highly inverse correlation between miR-200b and matrin 3 (MATR3) expression in MMAD tissues and in addition we detected that miR-200b regulates directly MATR3 expression in an adrenocortical cancer cell line.
Materials and Methods
Subjects
The investigation of patients with CS caused by MMAD was completed under the National Institute of Child Health and Human Development protocols 95-CH-0059 and 00-CH-160. Both protocols were approved by the Institutional Review Board and all subjects signed informed consents. A total of 10 patients, aged 39-60 years, were diagnosed with ACTH-independent CS that was caused by non-familial MMAD the diagnosis was confirmed by histology post-operatively. Adrenal samples were collected during surgery and immediately dissected by the pathologist; periadrenal fat was carefully removed and all tissues were stored at -80° C until further use. Four normal whole adrenal RNA samples were used as controls: two from two different healthy individuals (Ambion, Biochain), another that was actually a pool of adrenal RNA from 5 normal subjects (Biochain) and a normal adrenal cortex sample from a patient. All the RNA samples from control adrenals were extracted from tissues collected at the time of autopsy.
MicroRNA Array Analysis
MirVana miRNA isolation Kit (Ambion, Inc, TX, USA) was used according to manufacturer's instructions in order to isolate RNA enriched for small RNAs from MMAD and control adrenal tissues. The quality of total RNA was tested using the Bioanalyzer 2100 and it was quantified using Nanodrop. Expression levels of 365 microRNAs were evaluated with TaqMan microRNA array assays as previously described (16). Statistical analysis was performed by using the SPSS statistics software package (SPSS). MicroRNA expression data were normalized to U6 expression levels (loading control). The cut-off for differentially expressed microRNAs was 1.5-fold (up- or down-regulated).
MicroRNA Real-Time PCR Analysis
Validation of these results was performed using the mirvana quantitative real time (qRT)-polymerase chain reaction (PCR) miRNA detection kit and qRT-PCR primer sets, according to the manufacturer's instructions (Ambion, Inc, TX, USA). MicroRNA expression data were normalized using the U6 small nuclear RNA expression levels as a loading control, as suggested by others (16, 17). Specifically, the ΔCt was calculated by subtracting the Ct of U6 RNA from each individual microRNA. Then ΔΔCt values were calculated by subtracting the ΔCt of the average of the normal sample from the ΔCt of the MMAD sample.
Real-Time PCR Analysis
Transcription of 0.1 ug RNA to cDNA was performed using the AMV kit (Roche, Indianapolis, USA). Lightcycler FastStart DNA master SYBR Green which contains Taq DNA polymerase, DNTPs mix, SYBR Green I dye and MgCl2 was used as a reaction mix for PCR. GAPDH expression levels were used as a control. RT-PCR analysis was completed in the Light Cycler Instrument (Roche Molecular Systems, Alameda, CA). The oligonucleotide primers used for MATR3, PUM2, PSMC6, CCNG1, KRT1, TXNIP and CPE have been described previously (17). All samples were analyzed in triplicate and the average value of the triplicates was used for quantification; data were expressed as the ratio of target gene mRNA levels versus those of the GAPDH housekeeping gene (16-20).
MicroRNA target prediction methods
The microRNA database Target scan version 4.2 (see http://www.targetscan.org/index.html) database was used to identify potential miRNA targets. To increase the accuracy of the prediction, we chose only target genes that were identified in this database and were conserved in other species. We then integrated the microRNA and our previous cDNA array data (7) to further increase the stringency of selected micro-RNA-gene interactions and detect “functional” microRNA-gene target pairs. We focused only on microRNA-gene target pairs with inverse correlations under the assumption that the vast majority of known microRNAs are negative regulators of gene expression. For example, a microRNA that was down-regulated in MMAD would target a gene that was identified as “up-regulated” in the cDNA array data, if this gene was the direct target.
Transfection experiments
H295R adrenocortical carcinoma cell line was purchased by ATCC and was maintained in DMEM/F12 medium. H295R cells were transfected with negative control miR (100 nM) or miR-200b (50-100 nM) for 48h (Negative control miR and miR-200b were purchased from Ambion); RNA was extracted and MATR3 mRNA expression was tested by real-time PCR analysis. The experiment was performed in triplicate and data are shown as mean ± SD.
Luciferase reporter assay
HEK293 cells in 6-well plates were transfected using Fugene6 (Roche, Penzberg, Germany). Firefly luciferase reporter gene construct (MATR3 3’UTR in pEZX-MT01 vector) (200 ng) and 1 ng of the pRL-SV40 Renilla luciferase construct (for normalization) were cotransfected per well. Cell extracts were prepared 24h after transfection, and the luciferase activity was measured using the Dual Luciferase Reporter Assay System (Promega, WI, USA). Luciferase reporter assays were performed in triplicate and the data are presented as mean ± SD.
Statistical analysis
A two-sample t test was done for experiments described above. Experiments were done at least in triplicate, and a mean was calculated. A P value of <0.05 was considered significant.
Results
miRNA gene signature in MMAD
We tested the expression of a total of 365 microRNAs and identified 37 differentially expressed microRNAs between MMAD and normal adrenal RNA. Specifically, we detected 16 down-regulated microRNAs and 21 up-regulated microRNAs (Figure 1). Down-regulated microRNAs in MMAD included miR-200b, miR-203, miR-146b and miR-133b, whereas up-regulated microRNAs included miR-210, miR-484, miR-196a, miR-424, miR-491 and miR-324-5p.
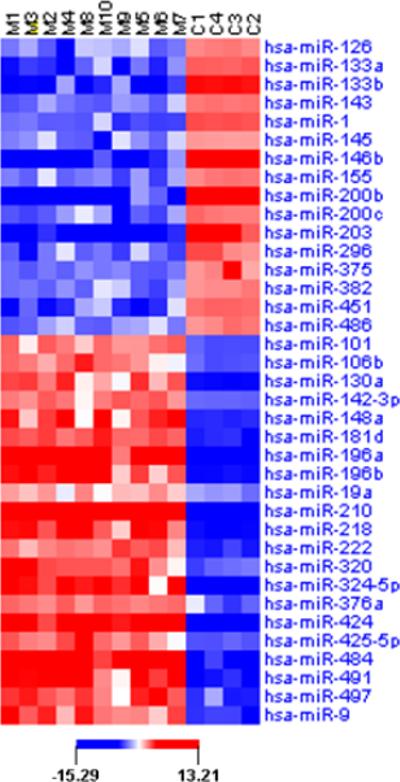
A color code is used for the level of microRNA expression: blue: lower microRNA expression, red: higher microRNA expression. The cut-off for differentially expressed microRNAs was 1.5-fold (up- or down-regulated). MMAD tissues are shown as M1-10; C1 is whole normal adrenal tissue (Ambion); C2 is whole normal adrenal tissue (Biochain); C3 is a pool of adrenal RNA from 5 normal subjects (Biochain) and C4 is normal adrenal cortex from a patient.
Validation of microRNA microarray data
SYBR Green qRT-PCR analysis validated the 37 microRNA gene signature that clearly distinguished MMAD tissues from normal adrenal samples (Figure 2). The microRNAs that were most highly down-regulated included miR-200b (-9.36 fold), miR-203 (-7.68 fold), miR-146b (-6.33fold). Other down-regulated microRNAs were decreased from -4.05 to -1.74 fold. Highly up-regulated microRNAs were miR-210 (10.78 fold), miR-484 (6.69 fold), miR-196a (5.66 fold) and miR-424 (5.23 fold); other up-regulated microRNAs increased from 4.42 to 1.48 fold.
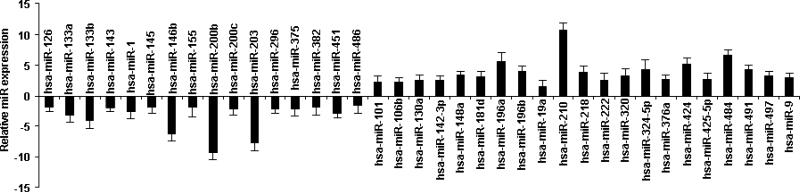
In order to validate microRNA microarray data, we performed microRNA SYBR Green Real-time PCR analysis. The results were consistent with the microarray data, showing that 16 microRNAs were down-regulated while 21 microRNAs were up-regulated in MMAD tissues relative to normal tissues. Statistical analysis was performed by using the SPSS statistics software package (SPSS). All results were expressed as mean ± SD, and P < 0.05 was used for significance. The cut-off for differentially expressed microRNAs was 1.5-fold (up- or down-regulated). MicroRNA expression data were normalized to U6 expression levels (loading control).
MiR-130a and miR-382 expression correlates with increased cortisol levels
As a test of the clinical significance of the above data, we sub-grouped our patients in 3 categories based on the “severity” of their disease (Table 1): group A (patients 4, 1, 5, 3) included patients with midnight cortisol levels less than 276 nmol/L; group B (patients 9, 6, 2) had cortisol levels that ranged between 276-552 nmol/L, and group C (patients 10, 8, 7) over 552 nmol/L. Up-regulation of miR-130a (r =0.9115) and miR-382 (r =0.8585) were associated with higher midnight cortisol levels (Figure 3).
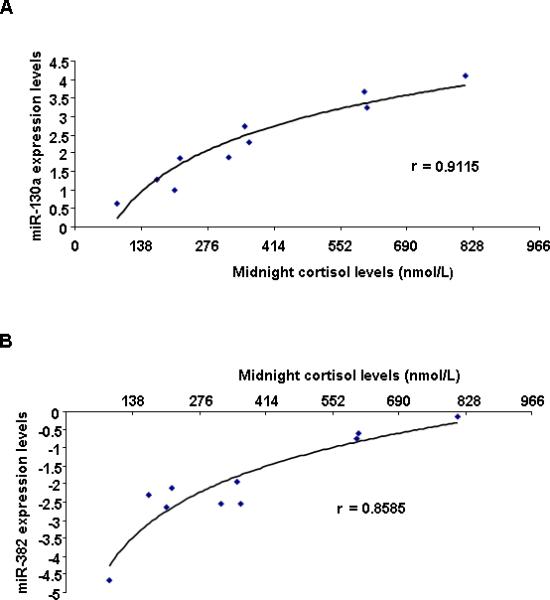
A, miR-382 and B, miR-130a expression have a positive correlation with midnight cortisol levels in MMAD patients. Correlation between microRNAs and midnight cortisol levels (nmol/L) was calculated with correlation coefficient.
Groups | Patient Number | Patient Code | Sex | Age(yr)a | Diagnosis | Surgical Procedure | Dimensions (cm) | Tumor weight (g) | Diurnal Cortisol | Levels (nmol/L) | UFC *nmol/d | 17-OH- Steroid (umol/d) | ||
---|---|---|---|---|---|---|---|---|---|---|---|---|---|---|
![]() | ||||||||||||||
Rt adrenal | Lt adrenal | Rt adrenal | Lt adrenal | midnight cortisol | morning cortisol | |||||||||
![]() | ||||||||||||||
A | 4 | ADT55.02 | F | 42 | MMAD | RA | 5 | - | N/A | - | 88.3 | 226.2 | 22.63 | 49.956 |
1 | ADT03.01 | M | 54 | MMAD | BADX | 9×5×3 | 6×2.5×2 | 190 | 154 | 171.1 | 344.9 | 319.88 | 104.052 | |
5 | ADT36.01 | M | 46 | MMAD | BADX | 10×8×3.5 | 8×4.5×2.5 | 101 | 490 | 206.9 | 270.4 | 309.12 | 46.92 | |
3 | ADT65.02 | F | 51 | MMAD | RA | 6.2×4 | - | 111 | - | 218.0 | 281.4 | 49.68 | 19.32 | |
B | 9 | ADT29.03 | M | 45 | MMAD | BADX | 10.5×6.5×2.5 | 13×5×4 | N/A | 131 | 320.0 | 549.0 | 366.39 | 30.36 |
6 | CAR73.01 | M | 60 | MMAD | BADX | 12×8×4 | 14×7×4 | 155 | 182 | 353.2 | 447.0 | 494.04 | 49.68 | |
2 | M2 | F | 49 | MMAD | BADX | 9×5×2 | 7×3×5 | 31.8 | 34.4 | 361.4 | 433.2 | 390.54 | N/A | |
C | 10 | ADT27.03 | F | 43 | MMAD | BADX | 9×4×2 | 9×5×2 | 52.3 | 94.8 | 601.5 | 841.5 | 1749.84 | 66.792 |
8 | ADT23.01 | F | 57 | MMAD | BADX | 8×8.5×3.5 | 11.5×.5×2.5 | 65 | 54.3 | 607.0 | 858.0 | 528.82 | 51.612 | |
7 | CAR 589.01 | M | 39 | MMAD | BADX | 10×5.5×2.8 | 7×6×2.5 | 36 | 44 | 811.1 | 780.8 | 1239.24 | 49.68 | |
![]() | ||||||||||||||
(137.95-698.75) | (22.2-213.6) | (8.28-27.6) | ||||||||||||
normal range |
Age (yr)a = age at the year of diagnosis
BADX=Bilateral adrenalectomy
RA= Right Adrenalectomy
MicroRNA - Gene Target Pairs in MMAD
Since microRNA exert their biological functions through suppression of target genes, it is important to identify microRNA-gene target pairs. We have used the TargetScan microRNA target prediction algorithm to identify potential gene targets for differentially expressed microRNAs in MMAD (Figure 4). To identify microRNAs modulating important signaling pathways in MMAD, we integrated our microRNA expression data with cDNA microarray data from our previous study in MMAD (7) together with computational analysis. Using this strategy, we identified 10 microRNA-gene target pairs that could be potentially involved in macronodular adrenal hyperplasia pathogenesis (Table 2, Figure 5). Specifically, miR-146b potentially targets Proteasome 26S Subunit ATPase 6 (PSMC6) and microRNAs miR-200b and miR-200c potentially target Matrin 3 (MATR3) and Pumilio homolog 2 (PUM2). In addition, Cyclin G1 (CCNG1) and Keratin 1 (KRT1) are putative gene targets of miR-203. Also, we identified that miR-148a potentially regulates Thioredoxin interacting protein (TXNIP), while both miR-196a and miR-196b potentially target carboxypeptidase E (CPE). Real-time PCR analysis of these ten microRNA-gene target pairs revealed that miR-200b – MATR3 pair was the highest inversely correlated in our MMAD tissues (r =-0.984) (Table 3).
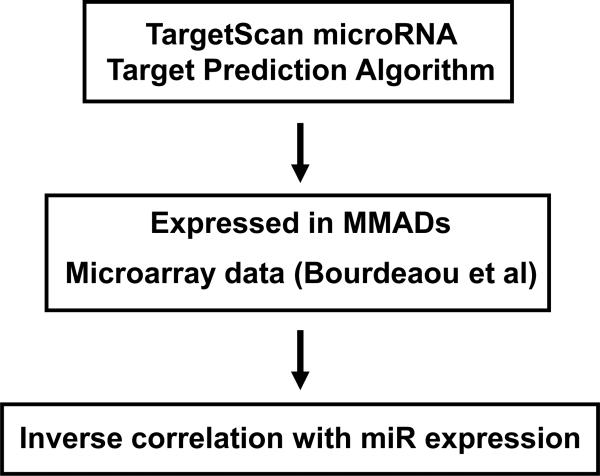
We used TargetScan prediction algorithms in order to identify potential gene targets for the differentially expressed microRNAs between MMADs and normal adrenal tissues. From the predicted gene targets we selected only those that were differentially expressed in MMADs according to the cDNA microarray data (Bourdeau I et al, Oncogene, 2004). The last step was to select only the inverse correlated microRNA - gene target pairs, because microRNAs are negative regulators of gene expression.
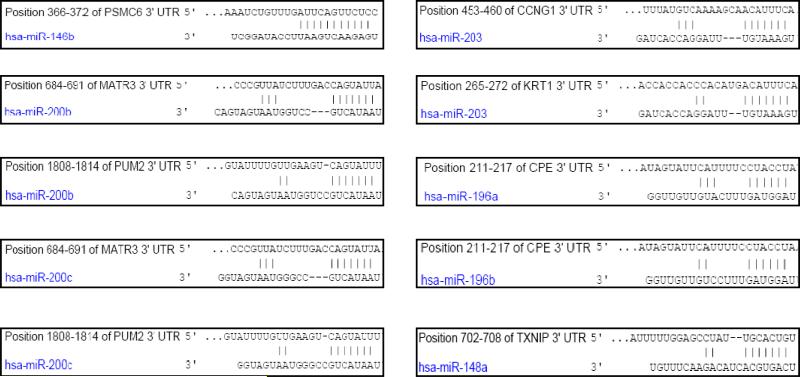
Identification of the specific sequences in the 3’UTR of microRNA gene target genes according to TargetScan Program Analysis.
Table 2
MicroRNA – gene target pairs in MMAD
MicroRNA | Chromosomal location1 | Putative Targets2 | Description |
---|---|---|---|
miR-146b | 10 : 104186259-104186331 | PSMC6 | Proteasome (prosome, macropain) 26S subunit, ATPase, 6 |
miR-200b | 1 : 1092347-1092441 | MATR3 | Matrin 3 |
PUM2 | Pumilio homolog 2 (Drosophila) | ||
miR-200c | 12 : 6943123-6943190 | MATR3 | Matrin 3 |
PUM2 | Pumilio homolog 2 (Drosophila) | ||
miR-203 | 14 : 103653495-103653604 | CCNG1 | Cyclin G1 |
KRT1 | Keratin 1 | ||
miR-148a | 3 : 189889263-189889348 | TXNIP | Thioredoxin interacting protein |
miR-196a | 7 : 27175624-27175707 | CPE | Carboxypeptidase E |
miR-196b | 12 : 52671789-52671898 | CPE | Carboxypeptidase E |
Table 3
Correlation coefficient between microRNA and gene target expression The previous analysis indentified ten potential microRNA-gene target pairs. For each microRNA and gene target we performed real-time PCR analysis in order to identify their expression levels in MMAD tissues. Due to the fact that microRNAs are negative regulators of gene expression, we were interested in the microRNA-gene target pairs with very high inversely correlated expression levels. In the table above we present the correlation coefficient between microRNA and gene target expression levels, starting from the most significant. The most significant inverse correlation was between miR-200b and MATR3 expression levels. We have identified miR-200b as the most highly down-regulated (-9.36 fold) microRNA in MMAD (according to microRNA microarray data) while MATR3 was highly up-regulated (5.56 fold) in MMAD tissues (cDNA microarray data from Bourdeau et al study).
microRNA | Gene Target | Correlation coefficient |
---|---|---|
miR-200b | MATR3 | -0.984 |
miR-203 | KRT1 | -0.877 |
miR-196a | CPE | -0.813 |
miR-196b | CPE | -0.807 |
miR-203 | CCNG1 | -0.673 |
miR-200c | MATR3 | -0.554 |
miR-200b | PUM2 | -0.534 |
miR-200c | PUM2 | -0.512 |
miR-148a | TXNIP | -0.451 |
miR-146b | PSMC6 | -0.404 |
MiR-200b regulates MATR3 expression in adrenocortical cancer cells
Due to the fact that miR-200b was the highest down-regulated microRNAs in MMAD and predicted to regulate MATR3, we further studied the role of miR-200b – MATR3 interaction in adrenocortical carcinogenesis using H295R cell line. MiR-200b over-expression in H295R cells resulted in >50% inhibition in MATR3 mRNA expression (Figure 6A). To identify if miR-200b regulated directly MATR3 through binding in the 3’UTR we performed luciferase assay which revealed that miR-200b inhibited >45% MATR3 3’UTR luciferase activity in HEK293 cells, suggesting that miR-200B regulates directly MATR3 expression (Figure 6B).
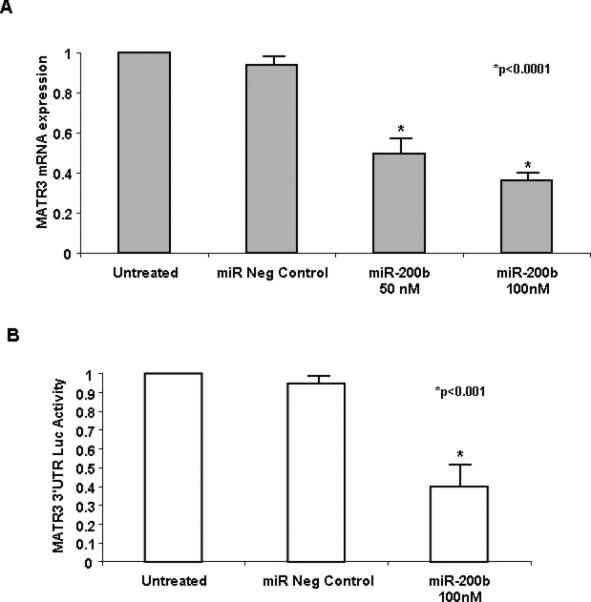
A, qRT-PCR analysis of MATR3 expression levels in an adrenocortical cancer cell line (H295R cell line). Transfection of miR-200b in the H295R cell line (50nM, 100nM) reduced MATR3 expression (>50% and 60% suppression of mRNA expression respectively). B, Luciferase assay after transfection of miR-200b (100 nM) together with luciferase vector harboring the 3’UTR of MATR3 gene in HEK293 cells. More than 45% of MATR3 3’UTR luciferase activity was inhibited by miR-200b. The data are presented as mean ± SD of three independent experiments.
Discussion
The present study identified 37 microRNAs which were statistically different in terms of their expression in patients with MMAD in comparison to normal adrenal tissue. We found reduced expression of several microRNAs (16/37) which have been also shown to be altered in various cancers: miR-200b and miR-200c are down-regulated in metastasis and have an important role in epithelial-mesenchymal transition (18) although they are over-expressed in human ovarian cancer (19). MiR-203 is down-regulated in several types of cancer, including oral squamous cell carcinoma (20); miR-203 may act as a tumor suppressor and its putative target gene is ABL1, a well known oncogene that is activated in chronic myelogenous leukemia (21). MiR-203 also targets the transcription factor p63 involved in proliferation and differentiation of epithelial cells (22).
Similarly, over-expressed microRNAs in MMAD (21/37) included several that have been implicated in tumorigenesis in other studies. MiR-210 expression has been found up-regulated in several cancer types and is associated with hypoxia- related pathways. Specifically, miR-210 is induced by HIF1A and hypoxic conditions; HIF1A binds in the promoter of miR-210 and induces its expression. The central role of HIF in the upregulation of miR-210 has been confirmed with studies in several types of cancer. In our previous study (7) HIF1A was found over-expressed (3.68-fold); our new finding, that miR-210 is also over-expressed in MMAD tissues, is consistent with the cDNA expression and other data such as fumarate hydratase (FH) mutations and adrenocortical tumors (23,24) and the hypothesis that activation of the hypoxia pathway may be involved in MMAD pathogenesis (23). Studies in a renal carcinoma cell line showed that inactivating mutations in the VHL gene had as a result the suppression of HIF degradation and the induction of hypoxia and miR-210 upregulation (25). Hypoxia is a common feature in tumors with poor prognosis and insufficient response to drugs and miR-210 has been suggested as a prognostic factor for breast cancer (26). MiR-484 has not been associated to any type of carcinogenesis yet and its function remains unknown.
We also identified two microRNAs, miR-130a and miR-382 with an expression pattern that correlated with the midnight cortisol levels of our patients, an index of the severity of their disease. MiR-130a has been found to be involved in cancer progression by regulating angiogenesis in endothelial cells through the suppression of two antiangiogenic homebox genes, GAX and HOXA5 (27). MiR-382 has been studied in acute myeloid leukemia where a positive correlation between the leukemic subtype t(15;17)/PML-PARA and over-expression of miR-382 was detected (28). Both miR-130a and miR-382 were found positively correlated to the midnight cortisol levels.
The biological significance of microRNAs is determined by the identification of their presumed gene targets. The available software-based algorithms predict 20-50 gene targets for each microRNA without taking into consideration the biological relevance of their interaction. For this reason it is important to match microRNA with expression data. In our study, we were able to match 10 microRNA-target gene pairs using our previously published expression data (7). According to RT-PCR analysis, the miR-200b - MATR3 pair had the highest inverse correlation in our samples, suggesting the putative role of MATR3 in adrenal tumorigenesis. MATR3 is a nuclear matrix protein, that interacts with other nuclear proteins and regulates transcription. It has been shown that MATR3 is the main substrate of PKA after activation of NMDA (N-methyl-D-aspartate) receptors and that PKA mediated phosphorylation leads to the degradation of MATR3 in cerebellar neurons in culture inducing neuronal death (29). Studies in 5-fluorouracil (5-FU) - resistant colon cancer cell lines suggested the implication of Matrin 3 in differential resistance response to 5-FU in these cell lines (30). MiR-200b is known to suppress the protein tyrosine phosphatase gene (PTPN12) (31) and has been found down-regulated in human clear cell renal cell carcinoma (32) and in liver carcinogenesis in the rat model (33). The data indicate that miR-200b directly regulates the expression of MATR3 and suggest a putative interaction between microRNAs, MATR3 and the PKA signaling pathway.
We conclude that MMAD has a specific microRNA signature as other proliferative disorders. Studies, like the present, will identify more pathways involved in MMAD pathogenesis and, as the clinical correlation above indicated, may even identify new biological markers of disease activity and or therapeutic targets in patients with ACTH-independent CS caused by BAHs.
Acknowledgements
This work was supported by NIH intramural project Z01-HD-000642-04 to Dr. C.A. Stratakis and, in part, by the University of Crete, School of Medicine, Post-Graduate Program (to Drs. Bimpaki and Stratakis). We would like to thank Dr Maya Lodish for her assistance in formatting the tables.
References
Full text links
Read article at publisher's site: https://doi.org/10.1111/j.1365-2265.2009.03725.x
Read article for free, from open access legal sources, via Unpaywall:
https://europepmc.org/articles/pmc3003432?pdf=render
Citations & impact
Impact metrics
Article citations
Heterogeneous circulating miRNA profiles of PBMAH.
Front Endocrinol (Lausanne), 13:1073328, 13 Dec 2022
Cited by: 1 article | PMID: 36583003 | PMCID: PMC9792611
MicroRNAs and Long Non-Coding RNAs in Adrenocortical Carcinoma.
Cells, 11(14):2234, 18 Jul 2022
Cited by: 3 articles | PMID: 35883677 | PMCID: PMC9324008
Review Free full text in Europe PMC
Characterization of Adrenal miRNA-Based Dysregulations in Cushing's Syndrome.
Int J Mol Sci, 23(14):7676, 12 Jul 2022
Cited by: 5 articles | PMID: 35887024 | PMCID: PMC9320303
Circulating MicroRNAs as Non-invasive Biomarkers for Canine Cushing's Syndrome.
Front Vet Sci, 8:760487, 17 Nov 2021
Cited by: 7 articles | PMID: 34869733 | PMCID: PMC8635510
MicroRNA expression signature and target prediction in familial and sporadic primary macronodular adrenal hyperplasia (PMAH).
BMC Endocr Disord, 22(1):11, 05 Jan 2022
Cited by: 2 articles | PMID: 34986816 | PMCID: PMC8729020
Go to all (42) article citations
Other citations
Similar Articles
To arrive at the top five similar articles we use a word-weighted algorithm to compare words from the Title and Abstract of each citation.
MicroRNA signature of primary pigmented nodular adrenocortical disease: clinical correlations and regulation of Wnt signaling.
Cancer Res, 69(8):3278-3282, 07 Apr 2009
Cited by: 51 articles | PMID: 19351815 | PMCID: PMC3124768
The role of microRNA deregulation in the pathogenesis of adrenocortical carcinoma.
Endocr Relat Cancer, 18(6):643-655, 27 Oct 2011
Cited by: 123 articles | PMID: 21859927 | PMCID: PMC3201061
Hereditary leiomyomatosis associated with bilateral, massive, macronodular adrenocortical disease and atypical cushing syndrome: a clinical and molecular genetic investigation.
J Clin Endocrinol Metab, 90(6):3773-3779, 01 Mar 2005
Cited by: 59 articles | PMID: 15741255
Clinical and genetic analysis of primary bilateral adrenal diseases (micro- and macronodular disease) leading to Cushing syndrome.
Horm Metab Res, 30(6-7):456-463, 01 Jun 1998
Cited by: 66 articles | PMID: 9694579
Review
Funding
Funders who supported this work.
Intramural NIH HHS (2)
Grant ID: Z01 HD000642-11
Grant ID: Z01 HD000642
NICHD NIH HHS (1)
Grant ID: Z01-HD-000642-04