Abstract
Free full text

Chronic ingestion of 2-deoxy-D-glucose induces cardiac vacuolization and increases mortality in rats
Abstract
Calorie restriction (CR), the purposeful reduction of energy intake with maintenance of adequate micronutrient intake, is well known to extend the lifespan of laboratory animals. Compounds like 2-deoxy-D-glucose (2DG) that can recapitulate the metabolic effects of CR are of great interest for their potential to extend lifespan. 2DG treatment has been shown to have potential therapeutic benefits for treating cancer and seizures. 2DG has also recapitulated some hallmarks of the CR phenotype including reduced body temperature and circulating insulin in short-term rodent trials, but one chronic feeding study in rats found toxic effects. The present studies were performed to further explore the long-term effects of 2DG in vivo. First we demonstrate that 2DG increases mortality of male Fischer-344 rats. Increased incidence of pheochromocytoma in the adrenal medulla was also noted in the 2DG treated rats. We reconfirm the cardiotoxicity of 2DG in a 6-week follow-up study evaluating male Brown Norway rats and a natural form of 2DG in addition to again examining effects in Fischer-344 rats and the original synthetic 2DG. High levels of both 2DG sources reduced weight gain secondary to reduced food intake in both strains. Histopathological analysis of the hearts revealed increasing vacuolarization of cardiac myocytes with dose, and tissue staining revealed the vacuoles were free of both glycogen and lipid. We did, however, observe higher expression of both cathepsin D and LC3 in the hearts of 2DG-treated rats which indicates an increase in autophagic flux. Although a remarkable CR-like phenotype can be reproduced with 2DG treatment, the ultimate toxicity of 2DG seriously challenges 2DG as a potential CR mimetic in mammals and also raises concerns about other therapeutic applications of the compound.
Introduction
An expansive body of research has shown that calorie restriction (CR) can produce numerous beneficial health and functional effects in a diverse array of organisms (Masoro, 2002). CR involves long-term reduced intake of a nutritious diet about 20–50% below the ad libitum, or unrestricted, level of consumption and health benefits ranging from improved cardiovascular health to reduced tumorigenesis have been demonstrated in laboratory rodents on such regimens (Guo et al., 2002; Hursting et al., 2003). A few well-controlled clinical trials as well as studies of humans voluntarily practicing a CR lifestyle are beginning to indicate that CR could also have beneficial effects on human health (Weiss et al., 2006; Civitarese et al., 2007; Fontana et al., 2007; Larson-Meyer et al., 2008; Lefevre et al., 2008). In addition to its myriad health benefits, CR produces robust increases in lifespan in many species and has been predicted to be able to confer at least a modest effect on human lifespan (Ingram et al., 2006a; Everitt and Le Couteur, 2007). However, concerns about the practicality of lifelong human CR including issues of compliance and potential negative impact to quality of life have prompted the search for CR mimetics (CRM). Whether dietary constituents or pharmacological products, CRM would be selected by their ability to stimulate signaling pathways involved in CR and reproduce the benefits of CR without necessitating a chronic reduction in food consumption (Ingram et al., 2006b).
One aspect of physiology that is profoundly altered by CR and therefore may be a target for CRM research is energy metabolism. CR exerts several metabolic effects indicating a shift in energy processing. In general, CR induces a shift from carbohydrate to lipid metabolism (Jensen et al., 1987). In particular, CR reduces circulating blood glucose and insulin while upregulating pathways associated with glucose synthesis (e.g., gluconeogenesis and lipid and amino acid catabolism) (Hagopian et al., 2003) and downregulating pathways associated with glucose catabolism (e.g., glycolysis and the Krebs cycle) (Feuers et al., 1989). Given these observations, it is reasonable to hypothesize that treatments reducing glycolytic activity independent of reduced caloric intake may trigger CR-like responses and qualify as potential CRMs (Hipkiss, 2007).
The first proposed CRM was 2-deoxy-D-glucose (2DG) (Lane et al., 1998). 2DG is an analog of glucose with a hydrogen atom instead of a hydroxyl group at its C2 position, and it can be absorbed by the intestines, circulated, and taken up by cells via glucose transport mechanisms. Within cells 2DG is converted to 2-deoxyglucose 6-phosphate by hexokinase in the first step of glycolysis. This is as far as glycolysis with 2DG can proceed, as 2-deoxyglucose 6-phosphate inhibits phosphoglucose isomerase at the second step of glycolysis and impedes processing of glucose to fructose 6-phosphate. Because of this inhibition, 2DG has been used for over fifty years as an experimental tool to evaluate glycolysis in organisms from bacteria to humans (Wick et al., 1955; Brown, 1962). Additionally, fluorodeoxyglucose (FDG), where a hydrogen atom on 2DG is replaced with the positron-emitting isotope fluorine-18, is routinely used in medical imaging (PET scanning) in living systems (Basu and Alavi, 2008).
2DG is a potential CRM because its effects on metabolism may upregulate stress responses that protect against many aging processes to enhance longevity without a reduction in food intake. Supporting data for this hypothesis include studies where 2DG has been shown to recapitulate key hallmarks of CR such as reduced body temperature (Ingram et al., 2006), increased torpor (Dark et al., 1994), reduced heart rate (Wan et al., 2003), and reduced circulating levels of glucose and insulin (Lane et al., 1998; Wan et al., 2003; Wan et al., 2004). 2DG has also been shown to confer functional benefits that parallel those seen with CR including inhibition of tumor growth (Gridley et al., 1985; Zhu et al., 2005) and increased stress resistance to neurotoxins and cold shock (Duan and Mattson, 1999; Yu and Mattson, 1999; Guo and Mattson 2000; Wan et al., 2004). 2DG has also shown potential benefits in the treatment of seizures in rodents (Stafstrom et al., 2008). While the downstream mechanisms elicited by 2DG-induced glycolytic inhibition have not been fully established, animals treated with 2DG have been observed to show increased levels of glucocorticoids (Weidenfeld et al., 1994; Wan et al., 2004) and heat shock proteins (Wan et al., 2003)—again paralleling effects of CR (Sabatino et al., 1991; Selsby et al., 2005).
Ultimately the validation of a potential CRM depends on its ability to extend lifespan. To this end, a recent study in nematodes (C. elegans) demonstrated that 2DG could extend both mean and maximum lifespan (Schulz et al., 2007). While longevity studies in lower organisms are commonly conducted as a screen prior to testing in mammals, it is important not to extrapolate such findings to higher organisms without further investigation. Indeed, an earlier pilot evaluation of 2DG as a CRM found that when young male Fischer-344 (F344) rats were fed diets supplemented with 2DG for 12 weeks, some doses resulted in deaths with evidence of cardiac pathology (Lane et al., 1998). Given that other studies have reported favorable preliminary toxicity results with 2DG (Stafstrom et al., 2008), and the promising findings regarding the potential efficacy of 2DG as a CRM, the current studies were conducted to further evaluate chronic consumption of 2DG in rats. First, we conducted a survival study in male F344 rats and found evidence of cardiotoxicity at two doses and increased mortality at a dose of 0.2 g/kg (0.4% by weight in the diet). To rule out source-specific and strain-specific effects, a second study evaluated 2DG from two different sources in both Brown Norway (BN) and F344 rats. This study further confirmed our initial results with additional evidence of cardiac toxicity occurring in 2DG-treated rats of both strains and from both sources.
Materials and Methods
Materials
Animals
In the lifespan study male F344 rats were obtained from Charles River Laboratories (Raleigh, NC). Male F344 and BN rats were obtained for the second and third study from Harlan Sprague-Dawley (Indianopolis, IN).
2DG
For the first study, 2DG was obtained from Sigma/Aldrich (St. Louis, MO; 2DG-S). For the second study, 2DG was obtained again from Sigma/Aldrich and also from a second source, Regent Chemical Products (Quebec, Canada; 2DG-R). The two sources differ in their production methods; 2DG-S is synthetically derived while 2DG-R is naturally derived from the exoskeletons of crayfish. For the third study, 2DG was obtained from Sigma/Aldrich.
Methods
Animals
For the survival study rats were individually housed in stainless steel cages with ad libitum access to a standard chow diet (Teklad NTP-2000, Harlan Teklad, Madison, WI) and tap water until 6 months of age. This study was conducted under contract at TherImmune Research Corporation, Gaithersburg, MD. The room was maintained at 65–75 °F with 30–70% relative humidity and a 12:12h light:dark cycle. At 6 months of age, the animals were randomized into three diet groups: 1) Control (n = 45); 2) 0.25% 2DG (n = 45); and 3) 0.4% 2DG (n = 45). All groups were granted ad libitum access to their diets. 2DG (Grade II, CAS #154-17-6; ≥ 98% pure by gas chromatography) was mixed with the diet at the indicated dry weight percentages (0.25% or 0.40%). Body weight and food intake were measured weekly during weeks 1–14 and every 4 weeks thereafter. Core body temperature (CBT) was measured by thermistor probe or subcutaneous implant (BioMedic Data System, Inc. Monitoring System) when body weights were collected. Blood glucose and insulin levels (collected by orbital sinus puncture under CO2/O2 anesthesia) were acquired during weeks 1, 13, and 39 at 06:00 h (±1:00 h).
In the second study 3-month old male F344 rats (n = 44, initial weight range = 242–304g) and BN (n = 44, initial weight range = 201–285g) rats were housed individually in clear plastic cages on a 12:12h light:dark schedule at the Holabird NIA/NIH research facility in Baltimore, MD, with food and water available ad libitum. After a 6-day habituation period, the rats were weighed and randomly assigned to dietary treatment groups whereby 6 each of the F344 and BN rats were designated to receive NIH-31 standard rodent chow (Harlan Teklad, Indianapolis, IN, USA) supplemented by dry weight with 0.6%, 0.2% or 0.04% Sigma 2DG. Six more animals from each strain were assigned to equivalent dose levels of the diet supplemented with the Regent 2DG, and eight animals of each type were allocated as control animals to receive unsupplemented NIH-31 chow. Throughout the duration of the study, all rats had ad libitum access to weighed amounts of their prescribed diet and water. At the end of the 6-week feeding period, the rats were euthanized. Blood was collected into vials with EDTA. Hearts and kidneys were sliced longitudinally and placed into Streck fixative before further processing.
In the third study 3-month old male F344 rats (n = 24, initial weight range = 265–330g) were housed individually in clear plastic cages on a 12:12h light:dark schedule at the Gerontology Research Center NIA/NIH in Baltimore, MD, with food and water available ad libitum. After a 7-day habituation period, the rats were weighed and randomly assigned to dietary treatment groups whereby 12 each of the rats were designated to receive NIH-31 standard rodent chow (Harlan Teklad, Indianapolis, IN, USA) or NIH-31 supplemented by dry weight with 0.6% Sigma 2DG. Throughout the duration of the study, all rats had ad libitum access to weighed amounts of their prescribed diet and water.
All animal protocols were approved by the Gerontology Research Center Animal Care and Use Committee (339-DKI-Ra) of the National Institute on Aging, NIH.
Survival study
Rats were inspected daily for health issues, and deaths were recorded for each animal. Moribund animals were euthanized and recorded. Every animal found dead or euthanized was necropsied. Rats removed at interim points during the study for other reasons were not included in survival analysis. The totals of the euthanized and natural deaths for the groups were n = 36 (control), n = 45 (0.25% 2DG), and n = 45 (0.4% 2DG).
Histopathology
Survival Study
Pathological analysis of all major organs was conducted by a veterinary pathologist blinded to group identity on most of the rats that died naturally and all of those designated in advance for scheduled sacrifice and tissue collection. Hematoxylin and eosin (H&E) stained slides were prepared by Experimental Pathology Laboratories (Herndon, VA) for the following tissues: brain, heart, liver, kidneys, spleen, lungs, pituitary, thyroid/parathyroid, salivary glands, pancreas, testes, stomach, and all gross lesions identified at necropsy. Neoplasms, cysts, and other non-gradable findings were indicated as present.
Source and Strain Study
Rats were fasted overnight and euthanized by decapitation. Following euthanasia, the hearts and kidneys were dissected out and preserved in Streck STF fixative (Streck Laboratories, Omaha, NE) and subsequently sent to Histoserv, Inc. (Germantown, MD) for paraffin embedding and hematoxylin and eosin or PAS (periodic acid Schiff) staining. The hearts were graded for pathological changes by a blinded pathologist on a scale of 0 to 4. A score of 0 denoted a normal cardiac section; a score of 4 denoted the presence of severe cardiomyopathic changes in the section. For a section to warrant a categorization of 4, assessment of the section had to include observations such as very thin fibers or loss of fibers, distinct vacuolations dispersed among many fibers, distinct loss of striations and general architecture, expanded interstitial space and infiltration of inflammatory cells and fibroblasts.
Short-Term Study
Rats were euthanized and the hearts were flash-frozen in liquid nitrogen. Portions of frozen heart were sent to Histoserv, Inc. for hematoxylin and oil red O staining. Western blotting was performed on remaining portions of frozen heart tissue.
Western blotting
Frozen heart tissue was homogenized in RIPA buffer. Western blot analysis was performed using the following antibodies and dilutions: beclin-1 at 1:3000 (Novus Biologicals, Littleton, CO), cathepsin D at 1:500 (Santa Cruz Biotechnology, Santa Cruz, CA), LAMP1 at 1:3000 (Stressgen Bioreagents, Ann Arbor, MI), LAMP-2B at 1:5000 (generated in the Cuervo lab in rabbits), LC3 at 1:1000 (Cell Signaling Technology, Danvers, MA) or p62 at 1:2000 (Biomol International, Plymouth Meeting, PA). Following incubation with the appropriate secondary antibodies (Santa Cruz Biotechnology, Santa Cruz, CA), signals were detected using the ECL Plus Western Blotting Detection System (GE Healthcare).
Statistical analysis
Data are expressed as means ± standard error of the mean. Single-factor analyses of variance followed by Fisher’s post-hoc tests were used for all comparisons. Mortality during the survival study was assessed by using the logrank test to compare the Kaplan-Meier survival curves. Differences in prevalence of specific pathology across treatment groups were assessed using two-sided Chi-square analysis. Statistical significance was set at p ≤ 0.05.
Results
Study 1 (Survival Study)
Dietary 2DG reduces glucose, insulin and body temperature
Throughout the survival study body weights (Fig. 1A) and food intake (Fig. 1B) were similar for the control and 0.25% 2DG groups. Body weight and food intake in the 0.4% 2DG group declined starting after 50 weeks on the diets. Blood glucose levels (Fig. 1C) in the 2DG groups were reduced at week 13 (100.3 ± 3.6 (0.25% 2DG) and 100.9 ± 2.1 (0.4% 2DG) versus 113.6 ± 2.1 mg/dl (control)) and week 39 (103.2 ± 2.0 (0.25% 2DG) and 101.3 ± 2.2 (0.4% 2DG) versus 121.2 ± 3.5 mg/dl (control)) on the diets. Insulin levels (Fig. 1D) were likewise reduced at weeks 13 (2.6 ± 0.2 (0.25% 2DG) and 2.2 ± 0.2 (0.4% 2DG) versus 3.7 ± 0.3 ng/ml (control)) and 39 (2.5 ± 0.2 (0.25% 2DG) and 2.2 ± 0.3 (0.4% 2DG) versus 3.9 ± 0.6 ng/ml (control)). Body temperature (Fig. 1E) was also lowered by 2DG, but this effect was not seen until 39 weeks on the diets (34.2 ± 0.1 (0.25% 2DG) and 34.1 ± 0.1 (0.4% 2DG) versus 34.5 ± 0.2 °C (control)).

A) Body weight over time (n = 45 per group at diet onset).
B) Food consumption over time (n = 45 per group at diet onset).
C) Blood glucose was measured at 6am (n ≥ 43 for all groups and time points except n = 42 for 0.4% 2DG-S at week 13 and n = 30 for 0.4% 2DG-S at week 39).
D) Insulin was measured simultaneously with glucose in a subset of the rats (n = 12 per group at each time point).
E) Body temperature (n ≥ 44 for all groups and time points except n = 40 for 0.4% 2DG-S at week 13 and n = 30 for 0.4% 2DG-S at week 39).
F) Kaplan-Meier analysis of the survival curves showed 0.4% 2DG-S survival was significantly reduced from control (χ2 = 48.5, p < 0.0001; logrank test). n = 31 (Control), 40 (0.25% 2DG-S), and 40 (0.4% 2DG-S) at the beginning of the experiment.
G) Histopathology demonstrating vacuoles in the myocardium of a representative F344 rat treated with 0.40% 2DG (ii) compared to control (i).
Data are shown as means ± SEM. † = p < 0.001; * = p < 0.05.
Chronic ingestion of 2DG increases mortality and induces cardiac pathology
Regarding survival (Fig. 1F), F344 rats consuming 0.4 % 2DG in the diet showed decreased median survival by 45% (352 vs. 641 days) and maximum lifespan—calculated from the survival of the longest-living 10% of each group—by 43% (487 vs. 859 days; χ2 = 48.5, p < 0.0001, logrank test). Median survival in rats consuming the low dose of 0.25% 2DG was not significantly reduced (650 vs. 641 days) and although maximum lifespan was reduced by 10% (775 vs 859 days), overall there was no significant difference from control in the survival curves (χ2 = 1.3, p = 0.245, logrank test).
As outlined in Table 1, two major distinguishing pathological features of rats on the 2DG supplemented diet were severe cardiac toxicity leading to cardiac failure and neoplastic changes to the adrenal medulla. Virtually every 2DG-treated rat exhibited cardiac pathology. At a gross level, many hearts were markedly enlarged and abnormal in shape; some hearts were abnormally rounded while others were highly angled and almost box-like in shape. Common among the rats found dead before their scheduled sacrifice date, there were lesions indicative of heart failure, including discolored, dark, or mottled lungs, fluid in the thoracic cavity (indicative of left-sided cardiac failure) and fluid in the abdominal cavity (indicative of right-sided cardiac failure). Based on the pathological analysis of the H&E stained cardiac slides, we found the 2DG treated rats displayed vacuolization in their myocardial fibers (Fig. 1G), dilation of the atrial and ventricular chambers, hypertrophy of the ventricular wall, and/or atrial thrombosis. The vacuolization of the myocardial fibers occurred principally in the apical portion of the ventricles and septum. This lesion was more prominent in rats with thickened or normal ventricular walls. Once the ventricular wall became severely thinned, vacuolization was not as evident. Apparently this vacuolization was an indication of the degeneration of muscle fibers resulting in the loss of contractility of the myocardium. Subsequently, hypertrophy of the ventricular wall occurred as a compensatory mechanism. With continuing failure of the heart, dilation of the organ occurred. Stagnation of blood flow due to impaired cardiac function resulted in atrial thrombosis. Congestion of the lung and liver was frequently present in rats that died early. Chronic passive congestion of the lung, with thickened alveoli, septa, pigmented macrophages, and dilated blood vessels was a frequent feature in rats which died after a longer period of time. When selected slides were stained for periodic-acid-Schiff reaction (PAS), no evidence was found to confirm glycogen content of the vacuoles related to 2DG treatment. The vacuoles appeared as clear and empty spaces and most likely represent a degeneration of the cytoplasm of the myocardial cells.
Table 1
Incidence of Major Pathology
0.25% | 0.40% | Chi-square | p-value | |||
---|---|---|---|---|---|---|
Tissue | Group | Control | 2DG | 2DG | ||
Total Rats Examined | 36 | 45 | 45 | |||
Heart | Dilation | 0.0% | 55.6% | 75.6% | 48.00 | <0.0001 |
Ventricular Hypertrophy | 0.0% | 42.2% | 28.9% | 22.14 | <0.0001 | |
Artrial Thrombosis | 2.8% | 28.8% | 51.1% | 26.13 | <0.0001 | |
Vacuolation | 5.6% | 80.0% | 97.8% | 81.70 | <0.0001 | |
Adrenals | Pheochromocytoma (Benign) | 13.9% | 42.2% | 40.0% | 9.04 | 0.011 |
Pheochromocytoma (Malignant) | 0.0% | 2.2% | 2.2% | 0.81 | NS | |
Hyperplasia, Medullary | 5.6% | 20.0% | 26.7% | 6.12 | 0.047 |
Aside from the above noted cardiac pathology, there was also evidence of changes in the adrenal medulla of rats treated with 2DG (Table 1). For example, the incidence of pheochromocytoma (both benign and malignant) was approximately 40% in the two 2DG treated groups compared to about 14% in the untreated controls. The incidence of medullary hyperplasia was also higher in 2DG treated rats compared to the control group.
Other pathological comparisons were confounded to a degree due to the earlier deaths of the 2DG treated groups, but the 2DG treated rats had no other significant pathology that distinguished them from the other groups with one exception. Despite their accelerated mortality, the incidence of testicular atrophy among the 0.4% 2DG treated group was comparable to that observed in all the other groups, which was about 30%.
Study 2 (Source & Strain Study)
Short-term dietary 2DG from both natural and synthetic sources induces cardiac vacuolization
Figure 2 depicts body weight and food consumption throughout the feeding study. In the F344 rats, weight gain was significantly reduced in the high 2DG doses compared to control rats (43.6 ± 10.8g (S) and 41.7 ± 4.4g (R) versus 76.5 ± 5.1g) (Fig. 2A). Food consumption was also lower in the high-dose 2DG groups (Fig. 2C), as the average daily consumption throughout the feeding study was significantly lower compared to controls (14.9 ± 0.3g (S) and 13.6 ± 0.2g (R) versus 15.8 ± 0.2g). Consumption of the low-2DG-S was increased compared to controls (17.6 ± 0.3g versus 15.8 ± 0.2g, respectively).
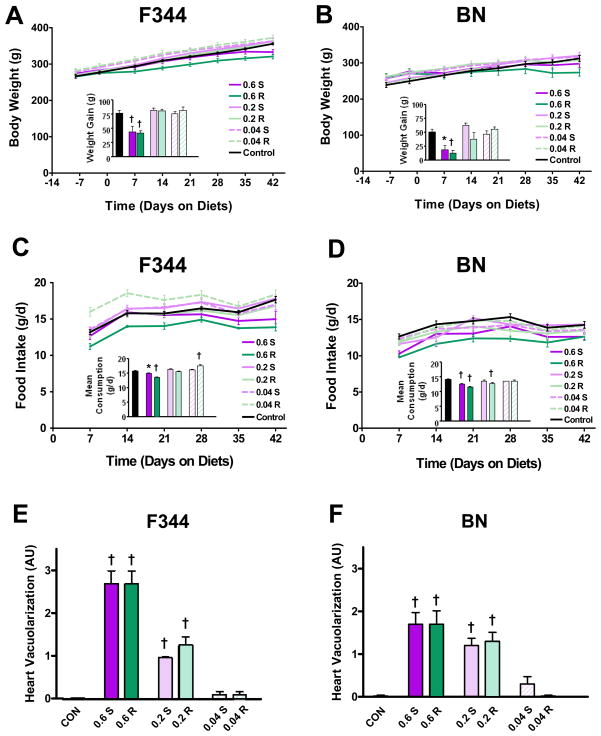
A) F344 body weight over time (n = 6 per 2DG group; n = 8 for control). Average weight gain throughout the course of the feeding study is shown in the inset.
B) BN body weight over time (n = 6 per 2DG group; n = 8 for control). Average weight gain throughout the course of the feeding study is shown in the inset.
C) F344 food consumption over time (n = 6 per 2DG group; n = 8 for control). Average daily consumption throughout the course of the feeding study is shown in the inset.
D) BN food consumption over time (n = 6 per 2DG group; n = 8 for control). Average daily consumption throughout the course of the feeding study is shown in the inset.
E) Vacuole grading in the F344 rats (n = 6 per 2DG group; n = 8 for control).
F) Vacuole grading in the BN rats (n = 6 per 2DG group; n = 8 for control).
Data are shown as means ± SEM. † = p < 0.001; * = p < 0.05.
Body weight in the BN rats followed a similar pattern with the high-dose 2DG groups showing reduced weight gain compared to controls (19.0 ± 8.0g (S) and 12.8 ± 5.6g (R) versus 50.9 ± 5.4g) (Fig. 2B). This was again reflected in food consumption (Fig. 2D) with the high-dose 2DG groups consuming less than controls on a daily basis (12.5 ± 0.3g/d (S) and 11.6 ± 0.3g/d (R) versus 14.2 ± 0.2g/d). The mid-dose 2DG-R group also consumed significantly less on average than controls (12.8 ± 0.4g/d versus 14.2 ± 0.2g/d, respectively).
Histological assessment of the kidneys revealed normal morphology (not shown); however, the hearts displayed abnormal characteristics including a dose-dependent increase in the degree of vacuole presence in both the F344 (Fig. 2E) and BN rats (Fig. 2F) fed 2DG from both sources. Only the low-dose 2DG-R BN and the control groups showed no observable cardiomyopathic changes by histopathology. Specifically, a disorganization of myofibrils associated with extensive vacuolization within myocytes was noted in the 2DG-fed rats (Fig. 3A panel ii) but not in a single control heart (Fig. 3A panel i).

A) Representative H & E staining of a control heart (i) and a high-dose 2DG-S heart. PAS-staining of a control heart (iii) and a high-dose 2DG-S heart (iv) reveal glycogen accumulation that is not intravacuolar. Likewise, oil red O staining of a control heart (v) and a high-dose 2DG-S heart (vi) show that the vacuoles are not an accumulation of lipid. All slides are from representative F344 hearts.
B) Representative immunoblots for the indicated proteins of cardiac muscle from 3 hearts of each group (6 total hearts per group were assayed and the following quantifications are the means ± SEM of all samples).
C) The mean value of the ratio of LC3II to LC3I.
D) Level of LC3II in 2DG-treated hearts relative to controls.
E) Levels of LAMP-1 and Beclin-1 in 2DG-treated hearts relative to controls.
F) Level of p62 in 2DG-treated hearts relative to controls.
G) Levels of three different isoforms of cathepsin D in 2DG-treated hearts relative to controls.
H) Presence of mature cathepsin D as a percentage of total cathepsin D levels.
Values are means ± SEM of 6 different animals. * = p < 0.001
2DG-induced cardiac vacuolization is not associated with either glycogen or lipid accumulation but with impaired autophagy
To determine whether the vacuoles contained glycogen, the hearts were stained with PAS. Examination of the hearts showed that while the slides from the 2DG-fed animals (Fig. 3A panel iv) show increased glycogen stores compared to controls (Fig. 3A panel iii), the vacuoles themselves were free of glycogen. Next we assessed the presence of lipid in the vacuoles with oil red O staining and found neither accumulation of lipid overall nor vacuolar lipid accumulation in the 2DG-treated hearts (Fig. 3A panel vi) compared with untreated controls (Fig. 3A panel v).
We next assessed whether the vacuoles were a reflection of a disruption in autophagy by quantifying the expression levels of proteins associated with degradation (Fig. 3B). An increase in both LC3 II and the ratio of LC3 II/I was found in the hearts of 2DG-treated rats. Pro-cathepsin D was also increased in the 2DG hearts, but not the pre- or mature forms of the protein. No significant changes were seen in LAMP-1, beclin-1, or p62.
Discussion
The present study provides evidence that 2DG delivered in the diet produces cardiac toxicity in rats at doses ranging from 0.02 – 0.3 g/kg (0.04 – 0.6% 2DG by weight in the diet) and hastens mortality at doses above 0.2 g/kg (0.4% in the diet). The latter occurs as a result of heart failure despite seemingly beneficial reductions to blood glucose, insulin and body temperature, all biomarkers of an effective CRM (Figure 1).
The second study was conducted to confirm the toxic effect in another strain using a natural source of 2DG. Despite the fundamental phenotypic differences among the strains (BN eat less and are smaller than F344), their responses to the 2DG treatment in terms of food consumption and body weight were similar. While there was no mortality in any of the groups in this six-week feeding study, toxic effects of 2DG began to appear in the final weeks as the groups receiving the highest doses began consuming noticeably less than the other groups. The reduction in food intake paralleled a reduction in weight gain that resulted in the high-dose groups weighing the least of all groups at the conclusion of the feeding period. The food consumption trend from the outset where the high-dose groups consumed less of the diets while the low-dose groups consumed more could reflect a food preference curve where up to a point 2DG improves the palatability of a diet (lower doses) but after a threshold reduces it (high doses).
Cardiotoxic effects of 2DG in F344 rats have been previously reported (Lane et al., 1998). Our data confirm this effect in F344 and show it extends to BN rats as well with substantial cardiomyopathic changes seen in both strains at the highest doses although vacuole accumulation was more pronounced in the F344 hearts. This strain difference may reflect their higher levels of consumption of the drug, or alternatively it may reflect a predisposition of F344 rats to developing cardiac pathology (Weindruch and Masoro, 1991). The lower 2DG doses also induced cardiomyopathic changes in both strains with scores ranging from 0 to 2. Only the low-dose 2DG-R BN and the control groups showed no observable cardiomyopathic changes by histopathology. It would be predicted at some dose level below 0.04, there would be no evidence of cardiomyopathy.
The third study was conducted to further explore the cardiotoxic effects of 2DG and investigate the nature of the cardiac vacuoles. Because 2DG is well known as a glycolytic inhibitor, we originally hypothesized the vacuoles were a result of excessive glycogen accumulation; however, the PAS staining showed the vacuoles did not contain glycogen. We then considered whether the vacuoles may instead represent accumulation of lipids or proteins. Indeed, work by Ralser and colleagues (2008) showed that in yeast, genes beyond glucose metabolism including those involved in cell cycle control, signaling, mRNA decay, redox control, protein degradation, ribosome biogenesis, and DNA repair are also involved in the organism’s response to 2DG. Our data further support the idea that 2DG has effects on biological systems beyond glucose metabolism and suggest the extensive vacuolization observed in the cardiac muscle to be of autophagic nature. Namely, we found a significant increase in the levels of LC3-II (a well-characterized autophagosomal marker) and in the ratio of its vacuolar (II) to cytosolic (I) forms in animals subjected to 2DG treatment. Although further functional studies may be required, our observations suggest the vacuolization may result more from inefficient clearance of autophagosomes than from upregulation of macroautophagy. Thus, we did not find considerable expansion of the lysosomal compartment (LAMP-1 positive) or induction of activators of macroautophagy such as beclin-1 in the treated animals when compared with controls. Furthermore, the slight increase in p62 (a typical autophagic cargo) in the treated animals supports decreased clearance in these animals. It is possible that problems in the trafficking of lysosomal enzymes or disruption of the lysosomal pH could be behind the suggested decrease in vacuolar clearance, as processing of cathepsin D from its precursor to its mature form was reduced upon 2DG treatment.
Despite current and previous experimental data suggesting dietary supplementation with 2DG can elicit physiological changes in rats (e.g. reduced body temperature and insulin levels) similar to those seen with CR (Ingram et al., 2006), we have demonstrated that 2DG administration induces cardiotoxic effects in two different rat strains and increases mortality in F344 rats. Whether mammalian species besides rats would exhibit toxicity to 2DG or whether different routes of administration (e.g. intravascular) would also produce the same effect are areas for future research. If similar mechanisms are operative in humans, then long-term dietary supplementation with 2DG or other glycolytic inhibitors could also prove toxic. These results seriously question 2DG’s potential as a CRM. Furthermore, ongoing research projects investigating 2DG as an anti-cancer (Mohanti et al., 1996; Maschek et al., 2004; Andringa et al., 2006) or anti-epileptic agent (Garriga-Canut et al., 2006) may need to consider the potential for long-term toxicity.
Acknowledgments
This work was supported by the Intramural Research Program of the National Institute on Aging, National Institutes of Health. We would like to acknowledge the generous gift of 2DG from Regent Chemical Products and we also want to thank Dr. Deborah A. Banas of the Experimental Pathology Laboratories, Inc., for her careful pathological analysis of the rats in the survival study. None of the authors have any conflict of interest to disclose.
References
Full text links
Read article at publisher's site: https://doi.org/10.1016/j.taap.2009.11.025
Read article for free, from open access legal sources, via Unpaywall:
https://europepmc.org/articles/pmc2830378?pdf=render
Citations & impact
Impact metrics
Citations of article over time
Alternative metrics
Smart citations by scite.ai
Explore citation contexts and check if this article has been
supported or disputed.
https://scite.ai/reports/10.1016/j.taap.2009.11.025
Article citations
Reprogramming the immunosuppressive tumor microenvironment through nanomedicine: an immunometabolism perspective.
EBioMedicine, 107:105301, 22 Aug 2024
Cited by: 0 articles | PMID: 39178747 | PMCID: PMC11388279
Review Free full text in Europe PMC
Healthy Aging in Times of Extreme Temperatures: Biomedical Approaches.
Aging Dis, 15(2):601-611, 01 Apr 2024
Cited by: 0 articles | PMID: 37450930 | PMCID: PMC10917539
Review Free full text in Europe PMC
Unveiling the veil of lactate in tumor-associated macrophages: a successful strategy for immunometabolic therapy.
Front Immunol, 14:1208870, 26 Jul 2023
Cited by: 12 articles | PMID: 37564659 | PMCID: PMC10411982
Review Free full text in Europe PMC
An immunometabolic patch facilitates mesenchymal stromal/stem cell therapy for myocardial infarction through a macrophage-dependent mechanism.
Bioeng Transl Med, 8(3):e10471, 13 Dec 2022
Cited by: 2 articles | PMID: 37206202 | PMCID: PMC10189442
Unraveling female reproductive senescence to enhance healthy longevity.
Cell Res, 33(1):11-29, 02 Jan 2023
Cited by: 18 articles | PMID: 36588114 | PMCID: PMC9810745
Review Free full text in Europe PMC
Go to all (72) article citations
Other citations
Similar Articles
To arrive at the top five similar articles we use a word-weighted algorithm to compare words from the Title and Abstract of each citation.
Caloric restriction in primates and relevance to humans.
Ann N Y Acad Sci, 928:305-315, 01 Apr 2001
Cited by: 96 articles | PMID: 11795522
Review
Enhancement of amphetamine-induced locomotor response in rats on different regimens of diet restriction and 2-deoxy-D-glucose treatment.
Neuroscience, 131(2):451-464, 01 Jan 2005
Cited by: 7 articles | PMID: 15708486
Effects of 2-deoxy-D-glucose on food and water intake and body temperature in rats.
Physiol Behav, 36(3):431-435, 01 Jan 1986
Cited by: 15 articles | PMID: 3703971
Intermittent fasting and dietary supplementation with 2-deoxy-D-glucose improve functional and metabolic cardiovascular risk factors in rats.
FASEB J, 17(9):1133-1134, 22 Apr 2003
Cited by: 83 articles | PMID: 12709404
Funding
Funders who supported this work.
Intramural NIH HHS (1)
Grant ID: Z01 AG000371-03