Abstract
Free full text

Recognition of Peptidoglycan from the Microbiota by Nod1 Enhances Systemic Innate Immunity
Introductory Paragraph
Humans are colonized by a large bacterial flora (the microbiota) essential for the development of the gut immune system1-3. A broader role for the microbiota as a major modulator of systemic immunity has been proposed4,5, however, evidence and mechanism have remained elusive. We show that the microbiota is a source of peptidoglycan that systemically primes the innate immune system, enhancing killing by bone marrow-derived neutrophils of two important pathogens: Streptococcus pneumoniae and Staphylococcus aureus. This requires signaling via the pattern recognition receptor Nod1 (which recognizes mesoDAP-containing peptidoglycan found predominantly in Gram-negative bacteria), but not Nod2 (which detects peptidoglycan found in Gram-positive and Gram-negative bacteria) or Tlr4 (which recognizes lipopolysaccharide)6,7. We demonstrate translocation of peptidoglycan from the gut to neutrophils in the bone marrow and show levels in sera correlate with neutrophil function. In vivo administration of Nod1 ligands is sufficient to restore neutrophil function after microbiota depletion. Nod1−/− mice show increased susceptibility to early pneumococcal sepsis, demonstrating a role for Nod1 in priming innate defenses facilitating a rapid response to infection. These data establish a mechanism for systemic immunomodulation by the microbiota and highlight potential adverse consequences of microbiota disruption, by broad-spectrum antibiotics, on innate immune defense to infection.
Humans are colonized by approximately 1013–1014 bacteria, residing primarily on the mucosal surfaces of the host8. Understanding host-bacteria relationships has generally focused on pathogenesis, however, infections are relatively rare and the predominant host-bacteria interactions are at worst benign, and often beneficial. Local effects of the microbiota, on gut homeostasis9 and gut immune development10,11, have been delineated, however, few studies have addressed the systemic effect of the microbiota12. A broader influence of the microbiota in the regulation of systemic immunity, due to the translocation of microbial products from the luminal side of the mucosa into the circulation, has been proposed4,13. However, evidence to support such an effect remains preliminary and an understanding of the mechanisms involved is lacking. Neutrophils are a critical component of the innate immune system and are often the initial defense against extracellular pathogens14. We have previously shown that local recognition of peptidoglycan, by the pattern recognition receptor Nod1 enhances killing of complement-opsonized S. pneumoniae by neutrophils15 (Fig. 1a). To ascertain whether this was a general mechanism of neutrophil activation we investigated opsonophagocytic killing of another major pathogen, S. aureus, which in contrast to S. pneumoniae, requires the oxidative burst for effective killing16. Neutrophils from the peritoneal cavity of mice administered (i.p.) heat-killed Haemophilus influenzae, a Gram-negative bacterium with mesoDAP-containing peptidoglycan, showed enhanced killing of S. aureus in an ex vivo opsonophagocytic assay, compared to neutrophils from unstimulated control mice (Fig. 1b). However, neutrophils from Nod1−/− mice were refractory to stimulation with heat-killed H. influenzae, confirming that local recognition of peptidoglycan by Nod1 enhances opsonophagocytic killing of both S. pneumoniae and S. aureus. This demonstrates that Nod1 has a broad influence on neutrophil function activating both oxidative and non-oxidative mechanisms of killing. Interestingly, we observed that neutrophils from Nod1−/− mice were not only unresponsive to stimulation by exogenous peptidoglycan, but also showed defects in their basal level of killing in the absence of additional stimulation (comparison of S. pneumoniae and S. aureus killing by neutrophils from unstimulated control wild-type and Nod1−/− mice) (Fig. 1a,b). We hypothesized that recognition of microbial products under basal conditions (in the absence of infection) systemically primes the innate immune system, enhancing neutrophil function, and that the host microbiota provides this stimulus. To investigate this hypothesis, mice were administered broad-spectrum antibiotics and the systemic effect of microbiota depletion on bone marrow-derived neutrophil function assessed. We confirmed by flow cytometry that, analogously to neutrophil-enriched PECs, the cell population enriched from the bone marrow interacting with bacteria were Ly6G+ neutrophils, and this interaction was dependent on opsonization of bacteria by complement (data not shown). Neutrophils isolated from the bone marrow of antibiotic treated mice and tested in an ex vivo opsonophagocytic assay, showed a significant reduction in killing of S. pneumoniae and S. aureus, compared to those from non-antibiotic treated control mice (Fig. 1c,d). Bone marrow-derived neutrophils from mice maintained germ-free also showed reduced killing of S. pneumoniae and S. aureus, compared to those from previously germ-free conventionalized mice, confirming the systemic role of the microbiota in enhancing neutrophil function (Fig. 1e,f). To define the mechanism by which the microbiota exerts this effect, we treated mice deficient in pattern recognition receptors for different microbial products (Nod1−/−, Nod2−/− and Tlr4−/−) with broad-spectrum antibiotics. Bone marrow-derived neutrophils from antibiotic treated Tlr4−/− and Nod2−/− mice showed a significant reduction in the killing of both S. pneumoniae and S. aureus compared to non-antibiotic treated mice, showing that neither lipopolysaccharide nor MDP from the microbiota are important for systemic innate immune activation in this model (Fig. 1g,h). In contrast, bone marrow-derived neutrophils from Nod1−/− mice were not only defective in killing S. pneumoniae and S. aureus but were also unresponsive to signals from the microbiota, showing that recognition of peptidoglycan from the microbiota by Nod1 is critical for maintaining a basal level of immune activation. Next we addressed how the microbiota could communicate systemically to sites distal to the gut, and whether peptidoglycan from the gut microbiota was translocated from the luminal side of the mucosa into the host circulation under basal conditions (in the absence of infection). The translocation of microbial products has been documented previously, however, their effects have generally been considered in the context of compromised barrier function due to viral or bacterial infection17,18. In addition, specific mechanisms for the uptake of peptidoglycan fragments from the colonized mucosa have been proposed19,20. Formerly germ-free mice were colonized with E. coli with [3H]-DAP-labeled peptidoglycan. E. coli colonized the gut stably over three days (Fig. 2a) and peptidoglycan was detected systemically in sera and levels correlated with those in feces (Fig. 2a,b). This indicates that during colonization peptidoglycan is constantly turned over and either excreted or translocated across the gut mucosa into the circulation. Furthermore, during colonization peptidoglycan accumulated in the bone marrow (Fig. 2b), and could be detected in the neutrophil fraction (72 hours post-oral inoculation, 0.043 ± 0.014% of total CPM of inoculum per 109 neutrophils). To demonstrate the activity of translocated peptidoglycan, sera from either antibiotic treated, non-antibiotic treated, germ-free or previously germ-free conventionalized mice, was added to a bioassay using HEK293T cells carrying an NF-κB-luciferase reporter, co-transfected with either a Nod1 or Nod2 construct. In this bioassay, addition of mouse sera elicited both Nod1 and Nod2-dependent NF-κB activation, confirming the presence of translocated peptidoglycan (Fig. 2c,d). Sera from germ-free and antibiotic treated mice elicited significantly less Nod1 and Nod2-dependent NF-κB activation than sera from previously germ-free conventionalized and non-antibiotic treated mice, respectively (Fig. 2c,d). This demonstrates that the decrease in Nod1-dependent neutrophil killing correlated with systemic levels of Nod1-activating peptidoglycan fragments. To determine whether Nod1 ligands alone were sufficient to restore innate immunity after microbiota depletion, mice were treated with broad-spectrum antibiotics and then administered (i.p.) MurNAcTriDAP (the Nod1 ligand), MDP, or vehicle controls. Bone marrow-derived neutrophils from mice treated with MurNAcTriDAP, but not MDP, showed increased killing of S. pneumoniae, compared to vehicle control, confirming that fragments of peptidoglycan found systemically, recognized by Nod1, are sufficient to activate neutrophils after microbiota depletion (Fig. 3a). Additionally we confirmed the expression of Nod1 in bone marrow-derived neutrophils by RT-PCR (data not shown). We have previously shown that neutrophils are required to control sepsis from pneumococcal infection of the upper respiratory tract21. To investigate the importance of Nod1 in priming innate immunity, WT and Nod1−/− mice were challenged intranasally with S. pneumoniae. Nod1−/− mice were more susceptible to early sepsis (day 3, P = 0.0176; and day 4, P = 0.0437) than WT, however, there was no difference in the overall survival between Nod1−/− and WT animals (>day 5) (Fig. 3b). This suggests that Nod1−/− mice have delayed responses to infection due to a lack of innate immune priming rather than any intrinsic defects. We then determined if neutrophils could be directly activated by peptidoglycan. HL-60 cells differentiated to a neutrophil-like phenotype were treated with MurNAcTriDAP, MDP, or vehicle controls. Treatment with MurNAcTriDAP, but not MDP, increased killing of S. pneumoniae, compared to vehicle control (Fig. 3c), demonstrating that peptidoglycan recognized by Nod1, but not Nod2, can directly enhance neutrophil function. This enhancement by MurNAcTriDAP required the ability of Nod1 to activate the transcription factor NF-κB (Fig. 3c). Neutrophils isolated from human whole blood also showed a significant increase in killing of S. pneumoniae after treatment with MurNAcTriDAP (Fig. 3d).
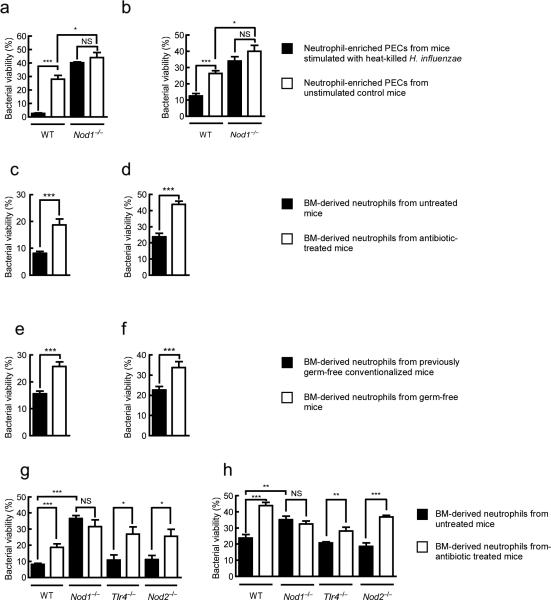
(a,b) Killing of S. pneumoniae (a) and S. aureus (b) by neutrophil-enriched PECs obtained from WT and Nod1−/− mice stimulated with heat-killed H. influenzae (i.p.) and unstimulated control mice. (c,d) Killing of S. pneumoniae (c) and S. aureus (d) byneutrophils harvested from bone marrow of WT mice treated with broad-spectrum antibiotics and untreated control mice. (e,f) Killing of S. pneumoniae (e) and S. aureus (f) by neutrophils harvested from bone marrow of germ-free WT mice and previously germ-free conventionalized mice. (g,h) Killing of S. pneumoniae (g) and S. aureus (h) by neutrophils harvested from bone marrow of WT, Nod1−/−, Tlr4−/−, and Nod2−/− mice treated with broad-spectrum antibiotics and untreated control mice. For all assays bacterial viability is expressed relative to control assays without neutrophils. Values represent at least three independent experiments performed in triplicate ± SEM. *P< 0.05, **P<0.01 and ***P<0.001.
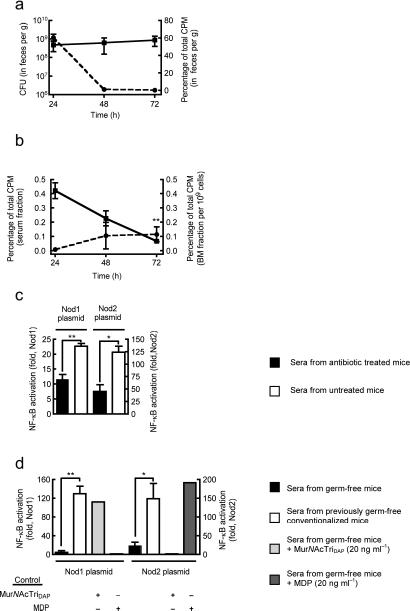
(a,b) Detection of translocated peptidoglycan in blood, feces and bone marrow cell samples from mice inoculated with [3H]-DAP labeled E. coli via oral gavage, by liquid scintillation counting. CFU in feces (—) and CPM in feces (– –) (a); CPM in serum fraction (—) and CPM in bone marrow fraction (– –) (b). Data is relative to CPM of total inoculum, time is post-oral inoculation. Statistical comparison of peptidoglycan accumulation in bone marrow cells at 72 hour is relative to 24 hour time point. (c,d) A HEK293T cell bioassay to detect peptidoglycan recognized by Nod1 and Nod2 in the sera of antibiotic treated and non-antibiotic treated WT mice (c); and conventionalized and germ-free WT mice (d). HEK293T cells were co-transfected with a NF-κB-luciferase reporter and either a Nod1 or Nod2 construct; luciferase expression was measured 24 h post-transfection and considered a measure of NF-κB activation. The specificity of NF-κB activation was confirmed using sera from germ-free mice with MDP and MurNAcTriDAP added in vitro to control Nod1 and Nod2 bioassays. Values represent fold increase in luciferase expression above empty vector controls, and are based on three independent experiments performed in triplicate ± SEM. *P< 0.05, **P<0.01.
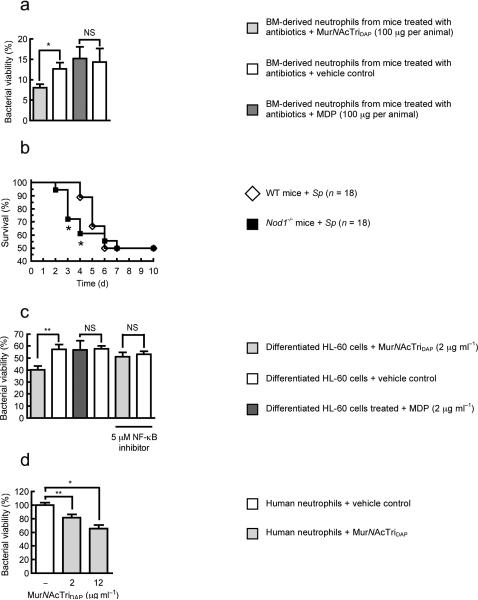
(a) Killing of S. pneumoniae P1121 by neutrophils from WT mice treated with broad-spectrum antibiotics and then administered (i.p., day 7) either MurNAcTriDAP, MDP, or vehicle controls. 24 hours after stimulation neutrophils were harvested from the bone marrow and their ability to kill S. pneumoniae compared. Bacterial viability is expressed relative to control assays without neutrophils. (b) Survival of Nod1−/− mice and WT mice challenged intranasally with 5 × 107 CFU of S. pneumoniae P1547. (c) Killing of S. pneumoniae by differentiated HL-60 cells treated with either MurNAcTriDAP, MDP, or vehicle controls for 4 hours. For NF-κB inhibition, cells were pretreated with 6-amino-4-(4-phenoxyphenylethylamino) quinazoline for 2 hours at 37 °C prior to stimulation with peptidoglycan. Bacterial viability is expressed relative to control assays without neutrophils. (d) Killing of S. pneumoniae by human neutrophils treated with MurNAcTriDAP for 2 hours, bacterial viability is shown relative to vehicle control treatment. Values represent at least three independent experiments performed in triplicate ± SEM. *P< 0.05, **P<0.01.
Previous work22-24, and data presented here, has shown that microbial products derived from the microbiota are found systemically, however, there is little appreciation of how they effect the host. Depletion of the microbiota significantly reduced systemic peptidoglycan levels which correlated with a reduction in killing of S. pneumoniae and S. aureus by bone marrow-derived neutrophils. We demonstrate that peptidoglycan recognized by Nod1 was sufficient to restore neutrophil function in the absence of the microbiota, and in the absence of Nod1 signaling there is a failure to prime the innate immune system when examined both in vitro and in vivo. This reveals a novel role for peptidoglycan in priming systemic innate immunity, and for Nod1 as a homeostatic regulator. Our data challenges the traditional view of innate immunity as quiescent in the absence of infection, and activated only upon pathogen recognition25. We propose that the microbiota constantly modulates the innate immune system, priming it, thus facilitating a rapid response to pathogens, such as S. pneumoniae and S. aureus, that might otherwise quickly overwhelm innate defenses. This systemic effect of microbial products means that disruption of the microbiota, with a concomitant lowering of systemic immune activation, may have profound consequences on the host rendering it more susceptible to infections. This may be a factor in the prevalence of secondary infections which occur after broad-spectrum antibiotic therapy26. There is increasing discussion regarding the beneficial, or “probiotic”, role of the microbiota, however, the mechanism by which the microbiota exerts these effects are vague and inferred. Our data provides a direct example and mechanism for a probiotic activity of the microbiota, demonstrating that translocated microbial products found systemically benefit the host by enhancing systemic innate immune function.
Materials and Methods
Bacterial strains
S. pneumoniae P1121 (a type 23F isolate), S. pneumoniae P1547 (a type 6A isolate) and H. influenzae 636 were grown as previously described15,27. S. aureus 8325-4 was grown in tryptic soy broth at 37 °C with shaking.
Mouse strains
Mice were used at 6–10 weeks and housed in accordance with Institutional Animal Care and Use Committee protocols. Wild-type C57Bl/6 and congenic Nod2−/− (C57Bl/6) and Tlr4−/− (C57Bl/10ScN) mice were from the Jackson Laboratories. Nod1−/− mice were provided by Millennium Pharmaceuticals. C57Bl/6 mice were bred and maintained germ-free in one Trexler flexible film isolator in the University of Pennsylvania gnotobiotic facility. Germ-free mice were conventionalized by housing in non-germ-free conditions for >3 weeks.
Cell culture
HL-60 cells were cultured in RPMI 1640 medium (GIBCO) + 10% FCS (GIBCO), 100 units ml−1 penicillin, and 100 μg ml−1 streptomycin (GIBCO) in a humidified atmosphere of 5% CO2, at 37 °C. HL-60 cells were differentiated to a neutrophil-like phenotype by addition of 1.3% DMSO to medium for 7 days.
Microbiota depletion
Mice were provided with broad-spectrum antibiotics (ampicillin 1 g ml−1, Mediatech; neomycin sulphate 1 g ml−1, Calbiochem; metronidazole 1 g ml−1, Fluka; and vancomycin 0.5 g ml−1, Sigma) in drinking water for 1 week.
Isolation of neutrophils from the peritoneal cavity
We obtained neutrophils by lavage of the peritoneal cavity with PBS + 20 mM EDTA, of mice administered (i.p.) 1 ml PBS + 10% casein for 24 hours, and again 2 hours prior to cell harvest, as described previously15. PECs from unstimulated control mice were elicited with casein alone, PECs from stimulated mice were elicited with casein containing heat-inactivated (65 °C for 30 minutes) H. influenzae 636 (1×107 cells per animal), stimulation protocol modified from28. We enriched neutrophils from PECs by Ficoll density gradient centrifugation using Mono-poly resolving medium according to the manufacture's instructions (MP Biomedicals). Flow cytometry of enriched cells showed that >90% were Ly6G+.
Isolation of neutrophils from bone marrow
We isolated neutrophils from the hind limbs of mice. Bones were washed with 70% ethanol, then Hank's buffer (−Ca2+ and −Mg2+) (Mediatech) + 0.1% gelatin. Bones were flushed with Hank's buffer (−Ca2+ and −Mg2+) + 0.1% gelatin and enriched neutrophils by Ficoll density gradient centrifugation using Mono-poly resolving medium.
Opsonophagocytic killing assay
We assessed opsonophagocytic killing by neutrophils using an ex vivo assay as described previously15. A 200 μl assay in Hank's buffer (+Ca2+ and +Mg2+) + 0.1% gelatin, was composed of 1×102 PBS-washed bacteria, 1×105 neutrophils in Hank's buffer (+Ca2+ and +Mg2+) (GIBCO) + 0.1% gelatin and 20 μl of fresh serum from either mouse or baby rabbit as a source of complement. Assays were incubated for 45 minutes at 37 °C with rotation. NF-κB signaling was inhibited by 6-amino-4-(4-phenoxyphenylethylamino) quinazoline (CALBIOCHEM), as indicated. Bacterial viability was determined in serial dilutions.
Bioassay for detection of peptidoglycan
HEK293T cells were cultured in DMEM + 10% FCS, 100 units ml−1 penicillin, and 100 μg ml−1 streptomycin in a humidified atmosphere of 5% CO2, at 37 °C. Cells were seeded at a density of 6×104 cells per well and transiently transfected using FuGENE 6 Transfection Reagent (Roche) according to the manufacturer's instructions. Transfection reactions contained 25 ng pNF-κB-luc (Stratagene), and either 50 ng pcDNA3-Nod1-FLAG29, 50 ng pCMV-Tag-Nod230, or 50 ng empty vector control plasmid (pcDNA3 or pCMV-Tag2C) (Stratagene). Sera was added directly to cells with transfection reagents, at a final dilution of 1:125. 24 hours post-transfection luciferase expression was measured using a Luciferase Assay System (Promega) according to the manufacturer's instructions. Control assays using MDP and MurNAcTriDAP added to sera from germ-free mice confirmed Nod1- and Nod2-dependent NF-κB activation specificity, respectively.
Preparation of peptidoglycan fragments
MDP and MurNAcTriDAP were synthesized as described previously15,31.
Flow cytometry
For flow cytometry, we used antibodies to mouse Ly6.G (BD Biosciences), CD45 (BioLegend) and F4/80 (eBioscience). S. pneumoniae P1121 were labeled with FITC (1 mg ml−1) for 30 minutes at 37 °C for bacterial uptake assays. Cells were washed and resuspended in Hank's buffer (−Ca2+ and −Mg2+) + 0.1% gelatin and diluted 1:10.
Analysis of Nod1 expression by RT-PCR
Bone marrow-derived neutrophils, enriched by Ficol density gradient centrifugation, were further purified by FACS. The Ly6G+, CD45+ and F4/80− cell population was sorted to isolate neutrophils. RNA was isolated using an RNeasy mini kit (QIAGEN) according to the manufacture's instructions, and cDNA reverse transcribed using a high capacity reverse transcription kit (Applied Biosystems). We confirmed expression of Nod1 in bone marrow-derived neutrophils and HL-60 cells by RT-PCR (data not shown). PCR primers are described in supplementary methods.
Statistical analysis
Difference between groups was compared by the unpaired Student's t-test (GraphPad Prism 4). P-values <0.05 were considered significant. The Kaplan-Meier logrank test was used to compare survival between groups.
Acknowledgments
We thank C. G. Dowson and D. I. Roper (University of Warwick, UK) for peptidoglycan fragments, D. J. Philpott (University of Toronto, Canada) for Nod vectors, and D Kobuley for assistance with germ-free mice. This work was supported by grants AI038446 (JNW), AI044231 (JNW), AI078538 (JNW) and AI037108 (YY) from the US Public Health Service.
Abbreviations
BM | bone marrow |
BSA | bovine serum albumin |
CFU | colony forming units |
CPM | counts per minute |
DAP | diaminopimelic acid |
DMEM | Dulbecco's Modified Eagle Medium |
FACS | fluorescence-activated cell sorting |
FCS | fetal calf serum |
FITC | fluorescein isothiocyanate |
HEK293T | human embryonic kidney 293T cells |
HL-60 | human promyelocytic leukemia cells |
i.p. | intraperitoneal |
LB | Luria broth |
MDP | N-acetylmuramyl-l-alanyl-γ-d-glutamate |
MurNAcTriDAP | N-acetylmuramyl-l-alanyl-γ-d-glutamyl-meso-2,6-diaminopimelic acid |
NF-κB | nuclear factor-kappa B |
Nod1 | nucleotide-binding oligomerization domain containing 1 |
Nod2 | nucleotide-binding oligomerization domain containing 2 |
PBS | phosphate buffered saline |
PEC | peritoneal exudates cell |
r.p.m. | revolutions per minute |
SEM | standard error of the mean |
Tlr4 | toll-like receptor 4 |
WT | wild-type |
Footnotes
Author Contributions
T.B.C designed the research, performed the experiments, analyzed the data and wrote the manuscript, K.M.D performed experiments, analyzed data and contributed to the manuscript, E.S.L and A.Y.Z performed experiments and analyzed data, Y.Y. contributed vital reagents, J.N.W. designed the research, analyzed the data and wrote the manuscript.
References
Full text links
Read article at publisher's site: https://doi.org/10.1038/nm.2087
Read article for free, from open access legal sources, via Unpaywall:
https://europepmc.org/articles/pmc4497535?pdf=render
Citations & impact
Impact metrics
Citations of article over time
Alternative metrics
Article citations
The association between the gut microbiota metabolite trimethylamine N-oxide and heart failure.
Front Microbiol, 15:1440241, 26 Sep 2024
Cited by: 0 articles | PMID: 39391607 | PMCID: PMC11464299
Review Free full text in Europe PMC
The gut microbiota in thrombosis.
Nat Rev Cardiol, 17 Sep 2024
Cited by: 0 articles | PMID: 39289543
Review
Possible immune mechanisms of gut microbiota and its metabolites in the occurrence and development of immune thrombocytopenia.
Front Microbiol, 15:1426911, 07 Aug 2024
Cited by: 0 articles | PMID: 39171254 | PMCID: PMC11335631
Review Free full text in Europe PMC
The microbiota: a crucial mediator in gut homeostasis and colonization resistance.
Front Microbiol, 15:1417864, 06 Aug 2024
Cited by: 1 article | PMID: 39165572 | PMCID: PMC11333231
Review Free full text in Europe PMC
Gut microbiota mediates ambient PM2.5 exposure-induced abnormal glucose metabolism via short-chain fatty acids.
J Hazard Mater, 476:135096, 02 Jul 2024
Cited by: 0 articles | PMID: 38996677
Go to all (692) article citations
Data
Data behind the article
This data has been text mined from the article, or deposited into data resources.
BioStudies: supplemental material and supporting data
Similar Articles
To arrive at the top five similar articles we use a word-weighted algorithm to compare words from the Title and Abstract of each citation.
Bacterial peptidoglycan with amidated meso-diaminopimelic acid evades NOD1 recognition: an insight into NOD1 structure-recognition.
Biochem J, 473(24):4573-4592, 14 Oct 2016
Cited by: 18 articles | PMID: 27742759
Nod1 signaling overcomes resistance of S. pneumoniae to opsonophagocytic killing.
PLoS Pathog, 3(8):e118, 01 Aug 2007
Cited by: 49 articles | PMID: 17722978 | PMCID: PMC1950946
An essential role for NOD1 in host recognition of bacterial peptidoglycan containing diaminopimelic acid.
Nat Immunol, 4(7):702-707, 06 Jun 2003
Cited by: 757 articles | PMID: 12796777
The role of NOD1 and NOD2 in host defense against chlamydial infection.
FEMS Microbiol Lett, 363(17):fnw170, 14 Jul 2016
Cited by: 12 articles | PMID: 27421958
Review
Funding
Funders who supported this work.
NIAID NIH HHS (11)
Grant ID: R01 AI044231
Grant ID: AI038446
Grant ID: AI078538
Grant ID: R37 AI037108
Grant ID: AI037108
Grant ID: R01 AI038446
Grant ID: AI044231
Grant ID: R01 AI037108
Grant ID: R01 AI078538
Grant ID: R21 AI044231
Grant ID: R37 AI038446