Abstract
Free full text

Hepatocyte growth factor inhibits CNS autoimmunity by inducing tolerogenic dendritic cells and CD25+Foxp3+ regulatory T cells
Associated Data
Abstract
Immune-mediated diseases of the CNS, such as multiple sclerosis and its animal model, experimental autoimmune encephalitis (EAE), are characterized by the activation of antigen-presenting cells and the infiltration of autoreactive lymphocytes within the CNS, leading to demyelination, axonal damage, and neurological deficits. Hepatocyte growth factor (HGF) is a pleiotropic factor known for both neuronal and oligodendrocytic protective properties. Here, we assess the effect of a selective overexpression of HGF by neurons in the CNS of C57BL/6 mice carrying an HGF transgene (HGF-Tg mice). EAE induced either by immunization with myelin oligodendrocyte glycoprotein peptide or by adoptive transfer of T cells was inhibited in HGF-Tg mice. Notably, the level of inflammatory cells infiltrating the CNS decreased, except for CD25+Foxp3+ regulatory T (Treg) cells, which increased. A strong T-helper cell type 2 cytokine bias was observed: IFN-γ and IL-12p70 decreased in the spinal cord of HGF-Tg mice, whereas IL-4 and IL-10 increased. Antigen-specific response assays showed that HGF is a potent immunomodulatory factor that inhibits dendritic cell (DC) function along with differentiation of IL-10–producing Treg cells, a decrease in IL-17–producing T cells, and down-regulation of surface markers of T-cell activation. These effects were reversed fully when DC were pretreated with anti-cMet (HGF receptor) antibodies. Our results suggest that, by combining both potentially neuroprotective and immunomodulatory effects, HGF is a promising candidate for the development of new treatments for immune-mediated demyelinating diseases associated with neurodegeneration such as multiple sclerosis.
Hepatocyte growth factor (HGF), also called “scatter factor,” is a polypeptide growth factor that belongs to the plasminogen family and consists of a 62-kDa alpha subunit and a 34-kDa beta subunit that form a disulfide-linked heterodimer (1). The molecule was discovered independently as a growth factor in the liver (2, 3) and as a fibroblast-derived effector of epithelial movement and cell–cell interaction (4–6). HGF is a pleiotropic factor that can trigger motility, proliferation, morphogenesis, and organ regeneration in a variety of epithelial cells (7). HGF is able to induce various responses in development (1) and in pathological situations, including tumor progression (8) and suppression of fibrosis (9). The receptor for HGF, cMet, is a tyrosine kinase encoded by the cMet proto-oncogene (10, 11). Mice lacking either HGF or its receptor die during embryogenesis, with defects in placenta, liver, and muscle development (12–14).
Both HGF and its receptor cMet are expressed during brain development and persist in the adult (15, 16). cMet is expressed in neurons but also in other brain-resident cells such as oligodendrocytes, astrocytes, and microglia (17–22). HGF promotes axonal outgrowth and regulates the differentiation of various neuronal populations, including sensory, sympathetic, and motor neurons (23, 24). The ability of HGF to promote survival of neurons is as potent as that of several neuroprotective factors, including brain-derived neurotrophic factor, ciliary neurotrophic factor, glial cell line-derived neurotrophic factor, and neurotrophin-3 (23). In addition, HGF is able to induce proliferation and migration of oligodendrocyte precursor cells (OPC) (17, 18, 22) as well as inhibition of the proapoptotic caspase-3 pathway in oligodendrocytes (21). Therefore, HGF could be involved in the processes of neuroprotection, attenuation of oligodendrocyte degeneration, and/or remyelination.
In animal studies, overexpression of HGF in the CNS delays disease progression and prolongs life span in a mouse model of amyotrophic lateral sclerosis (20), a neurodegenerative disease of the nervous system. In addition, HGF is involved in the process of postischemic brain repair (25). Increased concentrations of HGF are detected in the cerebrospinal fluid of patients with inflammatory and demyelinating diseases such as acute demyelinating encephalomyelitis and multiple sclerosis (26).
In addition to its action on the CNS, HGF shows immunomodulatory effects: on the one hand, HGF originally was reported to promote adhesion of B cells (27) and migration of T cells (28) as well as recruitment of dendritic cells (DC) (29). Moreover, HGF was reported to inhibit secretion of TGF-β (30), a potent antiinflammatory cytokine known to inhibit the progression of experimental autoimmune encephalomyelitis (EAE) (31). On the other hand, HGF was identified more recently as having protective effects in animal models of inflammatory-mediated diseases including myocarditis (32, 33), glomerulonephritis (30, 34), inflammatory bowel disease (35), collagen-induced arthritis (36), and pulmonary fibrosis (37).
In the present report, we assess the effect of an overexpression of HGF in the CNS of C57BL/6 mice carrying a HGF transgene under the control of a neuron-specific enolase (NSE) promoter (HGF-Tg mice) leading to selective overexpression of HGF by neurons in the CNS. In contrast, HGF serum levels were similar to those in WT littermate controls. Introduction of HGF under the control of the NSE promoter into mice leads to expression of HGF specifically in postnatal neurons of the CNS and subsequent extracellular secretion of HGF in the CNS, where it can act both on neurons and on other types of postnatal cells, such as glial and immune cells. In this experimental setup, the neural as well as the glial system have been found to be physiologically normal during development and in the adult (20, 38, 39).
EAE induced either by immunization with myelin oligodendrocyte glycoprotein (MOG) peptide consisting of amino acids 35–55 [MOG(35-55)] or by adoptive transfer of T cells from 2D2 transgenic mice that express a T-cell receptor (TCR) specific for MOG(35-55), (TCRMOG) was inhibited before peak disease was reached in HGF-Tg mice. Notably, the level of inflammatory cells infiltrating the CNS decreased in these mice except for CD25+Foxp3+ regulatory T (Treg) cells, which increased. In addition, a strong T-helper cell type 2 (Th2) cytokine bias was observed: TNF-α, IFN-γ, and IL-12p70 were decreased in the spinal cord of HGF-Tg mice, whereas IL-4 and IL-10 were increased. Antigen-specific response (ASR) assays showed that HGF is a potent immunomodulatory factor that inhibits DC function through down-regulation of their CD40 expression together with a decrease in IL-12p70 secretion. Furthermore, DC treated in vitro with recombinant mouse HGF (rHGF) induced differentiation of IL-10–producing Treg cells, along with a decrease in IL-17–producing T cells and a down-regulation of surface markers of T-cell activation.
Collectively, our data strongly suggest that HGF can inhibit the clinical course of EAE through DC tolerization and induction of Treg-cell population.
Results
MOG-Induced EAE Is Inhibited in HGF-Tg Mice.
The HGF content in the spinal cord of HGF-Tg mice, as assessed by real-time PCR and ELISA, was significantly increased (ca. 2.5-fold) compared with WT littermates. In contrast, serum levels of HGF, determined by ELISA, did not differ significantly in HGF-Tg and WT littermate mice (Fig. 1A) (20). EAE was induced in HGF-Tg and WT littermate C57BL/6 mice using MOG(35-55) peptide. The clinical course of EAE was inhibited in HGF-Tg mice before peak disease was reached [day postimmunization (dpi) 18] until the chronic phase (dpi 45) (Fig. 1B). Histopathological analysis of spinal cord performed at peak disease (dpi 25) showed that there was less inflammatory infiltrate (determined by H&E staining) and demyelination (determined by luxol fast blue staining) in HGF-Tg mice, as demonstrated by a decrease in the mean number (± standard error of the mean, SEM) of lesions per slide (Fig. 1 C and D). Fewer inflammatory CD4+ T-cell, CD8+ T-cell, CD11b, and CD11c cell subtypes were observed in the spinal cord of HGF-Tg mice than in the spinal cord of WT mice (Fig. 1E). In addition, Bielschowsky’s silver staining indicated a trend toward a decrease in axonal damage in HGF-Tg mice, but the difference did not reach statistical significance (Fig. 1 C and D).
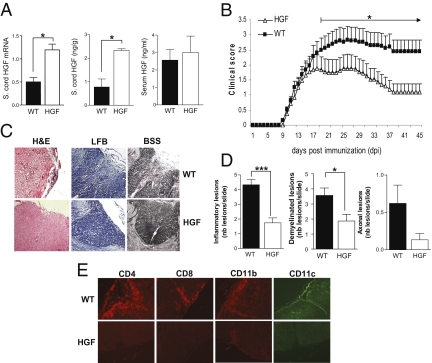
MOG(35-55)-induced EAE is inhibited in HGF-Tg mice. (A) HGF in the spinal cord of HGF-Tg mice versus WT littermates (n = 3 per group) as measured (Left) by real-time PCR (relative HGF mRNA expression) or (Center) by ELISA (ng/g of spinal cord) (mean ± SEM). *, P < 0.05. (Right) HGF measured in the serum by ELISA (ng/ml; mean ± SEM). (B) EAE scores were determined daily after disease onset in HGF-Tg mice (triangles; n = 17) and WT littermates (squares; n = 18). Shown are mean EAE score ± SEM. The difference was significant before peak disease was reached (dpi 18) and persisted until the chronic phase (dpi 45). *, P < 0.05. (C) Histopathology of paraffin-embedded spinal cord sections from PBS-perfused WT and HGF-Tg mice at peak disease (dpi 25). Sections were stained with H&E, luxol fast blue (LFB), or Bielschowsky’s silver staining (BSS) (magnification 200×). (D) Fifteen sections of spinal cord per mouse (n = 6 per group) were analyzed, and the mean number of lesions per slide (±SEM) are presented as histograms. *, P < 0.05; ***, P < 0.001. (E) Representative sections of spinal cord were analyzed. Shown are T-lymphocyte (CD4 and CD8) (red), macrophage (CD11b) (red), and DC (CD11c) (green) infiltration (magnification: 100×).
CNS Overexpression of HGF Does Not Influence Splenocyte Function During EAE.
Splenocytes of HGF-Tg mice and WT littermates were isolated at peak disease (dpi 25), and cell surface and intracellular markers were analyzed by flow cytometry. No differences between the two groups of mice were observed in the frequency of CD4+, CD8+, or CD4+CD25+Foxp3+ T cells, CD11c-CD11b+ macrophages, or CD11c+ DC (Fig. 2A). When proliferation assays were performed to evaluate the possible influence of CNS-specific overexpression of HGF on peripheral T cells, increased T-cell proliferation was observed with escalating concentrations of MOG peptide, but no difference was observed between HGF-Tg and WT mice (Fig. 2B). Analysis of splenocytes by FACS at EAE peak disease was performed with distinction of CD4+ T cells for IFN-γ (T-helper type 1, Th1), IL-10 (Th2), and IL-17 (T-helper type 17, Th17) subsets. No distinction was observed in the spleen for these three CD4+ T-cell subsets when WT and HGF-Tg mice were compared (Fig. 2C). To confirm the absence of specific splenic HGF increase in HGF-Tg mice, we analyzed the HGF content from whole spleen in WT and HGF-Tg mice. The results showed no difference in the splenic HGF content in normal and EAE peak-disease conditions (Fig. S1 A and B). To confirm further that HGF had no effect on splenocyte proliferation during EAE, a proliferation assay with increasing doses of HGF was performed. No proliferation of splenocytes was observed in either WT or HGF-Tg mice at various HGF concentrations (Fig. S1C).
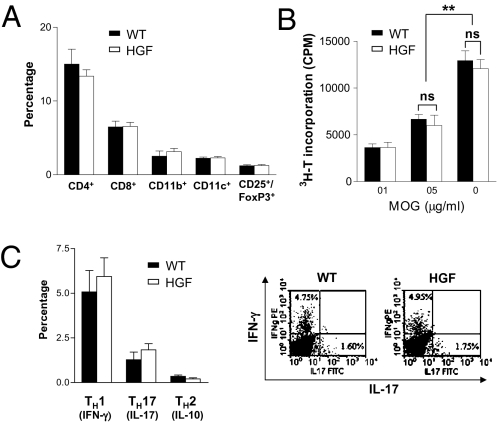
Unchanged characteristics of splenocyte populations in HGF-Tg mice during EAE. (A) Splenocytes were isolated from HGF-Tg mice and WT littermates during EAE and stained for CD4, CD8, CD11b, CD11c, and CD25-FoxP3. Bars show percentages of total splenocytes ± SEM (n = 3 per group). (B) Proliferation assay with MOG(35-55) of splenocytes from HGF-Tg mice and WT littermates (mean cpm ± SEM of triplicate experiments; n = 3). **, P < 0.01; ns, not significant. (C) Analysis by intracellular cytokine staining of the production of IFN-γ (TH1), IL-10 (TH2), and IL-17 (TH17) in splenocytes at peak dis-ease. (Left) Bars show percentages of CD4 subtypes ± SEM (n = 6 per group). (Right) Representative dot blots of IFN-γ and IL-17 analyses.
Increase of CD25+Foxp3+ Treg Cells and Induction of a Th2 Cytokine Bias in the Spinal Cord of HGF-Tg Mice During EAE.
Inflammatory cell infiltrates from pooled spinal cords of HGF-Tg (n = six mice) and WT littermates (n = five mice) were isolated at peak disease (dpi 25) by Percoll gradient, stained for surface markers, and analyzed by flow cytometry. Compared with WT littermates, a decrease of CD4+ and CD8+ T cells, CD11c−CD11b+ macrophages, and CD11c+ DC was observed in HGF-Tg spinal cords (Fig. 3A). In contrast, an increase of CD25+Foxp3+ Treg cells was observed in the spinal cords of HGF mice (Fig. 3B). Intracellular staining for IL-17 production in CD4+ T cells was lower in the spinal cord of HGF-Tg mice than in WT littermates (Fig. 3C). In a subsequent experiment, we analyzed by ELISA the cytokines detected in the supernatant of spinal cord homogenate of the two groups of mice. We observed a decrease in TNF-α, IFN-γ, and IL-12p70 in HGF-Tg mice, whereas IL-4 and IL-10 were increased (Fig. 3D). The sharp increase in IL-10 (ca. 8-fold) was confirmed further by real-time PCR (Fig. 3E).

Spinal cord inflammation of HGF-Tg mice is characterized by a Th2 bias and an increase in Treg cells. (A) Inflammatory cells were isolated by Percoll gradient from pooled spinal cords of HGF-Tg (n = 6) versus WT littermates (n = 5) at EAE peak disease (dpi 25) and were stained for CD4, CD8, CD11b, and CD11c. Bars show percentages of total cells. Spinal cord inflammatory cells were stained further (B) for CD4-CD25 (histogram; percentage of total CD4+ T cells) and CD4-CD25-FoxP3 (dot blots) or (C) for IL-17. (D) Spinal cord supernatants from HGF-Tg mice and WT littermates (n = 3 per group) from a subsequent EAE were analyzed by ELISA for cytokines. Shown are the mean ± SEM of experiments performed in triplicate. *, P < 0.05; **, P < 0.01; ***, P < 0.001. (E) The increase of IL-10 detected by ELISA in the spinal cord of HGF-Tg mice at peak disease was confirmed by real-time PCR analysis. *, P < 0.05.
HGF Inhibits Antigen-Presenting Function of DC in Vitro and Induces a Th2 Cytokine Bias.
To assess the ability of HGF to influence DC function and T-cell proliferation, we performed a mixed lymphocyte reaction with DC obtained from BALB/c splenocytes and T cells from C57BL/6 spleen cells. In parallel, we confirmed that the cMet receptor was present on the surface of CD11c+ DC of C57BL/6 or BALB/c mice but was not expressed by CD4+ T cells (Fig. S2). After incubation with rHGF at various concentrations, DC were cocultured with T cells, and a proliferation assay was performed using 3H-thymidine incorporation. T-cell proliferation was inhibited in a dose-dependant manner when allogeneic DC were pretreated with rHGF (Fig. S3). We further evaluated the ability of rHGF to inhibit DC function in an in vitro model closer to EAE, i.e., an ASR assay performed with MOG(35-55). In this experiment, DC were purified from C57BL/6 mouse splenocytes and then were treated with rHGF at various concentrations (1–100 ng/mL) or were left untreated and finally were pulsed with MOG(35-55) peptide (20 μg/mL). In parallel, TCRMOG T cells were obtained from splenocytes of 2D2 mice. After 48 h of coculture, T-cell proliferation was measured. A significant inhibition of T-cell proliferation was observed with increasing concentrations of rHGF (10–100 ng/mL), with the maximum effect starting at 30 ng/mL (Fig. 4A). To confirm that the inhibition of DC function was indeed mediated through the HGF–cMet pathway, an anti-HGF receptor (α-cMet) neutralizing antibody (10 μg/mL) was added to the DC before incubation with rHGF. Inhibition of T-cell proliferation was abrogated completely by preincubation of DC with the α-cMet antibody across the entire range of tested rHGF concentration (Fig. 4A). Furthermore, we examined the expression of T-cell activation markers during ASR assays and found that rHGF-treated DC pulsed with MOG(35-55) were not capable of activating T cells, which remained in a low state of activation (CD44lowCD62L+ and CD44intermediateCD62L+). In contrast, when the ASR assays were performed without rHGF pretreatment, T cells were highly activated (CD44highCD62L+ and CD4highCD62L−) (Fig. 4B). We then analyzed by ELISA the cytokine secretion profiles in the ASR assay supernatants after DC had been cocultured with TCRMOG T cells. We observed a decrease in TNF-α, IFN-γ, and IL-12p70, whereas IL-4, IL-10 and, to a lesser extent, TGF-β were increased when DC were pretreated with rHGF at 30 ng/mL (Fig. 5A). In a subsequent ASR assay, we analyzed intracellular IFN-γ and IL-17 production in CD4+ T cells by flow cytometry and found that IL-17 production was inhibited when CD4+ T cells were cocultured with rHGF-treated DC. In contrast, there was no change in T-cell production of IFN-γ (Fig. 5B). Finally, the expression of costimulatory surface molecules on DC (CD40, CD80, CD86, and MHC class II) was measured by flow cytometry during ASR assays. Unlike CD80, CD86, and MHC class II, a decrease in CD40 expression was observed under rHGF influence and was reversed when DC were pretreated with the α-cMet receptor antibody (Fig. S4).
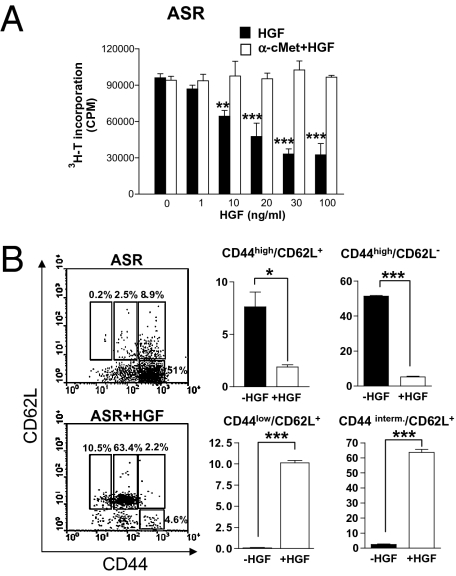
HGF inhibits in vitro CD11c+ DC function and T-cell activation markers. (A) ASR assay performed with C57BL/6 CD11c+ cells stimulated with MOG(35-55) and coincubated with TCRMOG T cells. T-cell proliferation was analyzed after CD11c+ cells were pretreated with α-cMet-blocking antibody or were left untreated and then were preincubated with increasing concentrations of rHGF. **, P < 0.01; ***, P < 0.001. (B) FACS staining for CD44 and CD62L coreceptors of CD4+ T cells after CD11c+ cells were preincubated with rHGF (30 ng/mL) or were not preincubated. Results shown are the mean of three to five independent experiments ± SEM. *, P < 0.05; ***, P < 0.001.
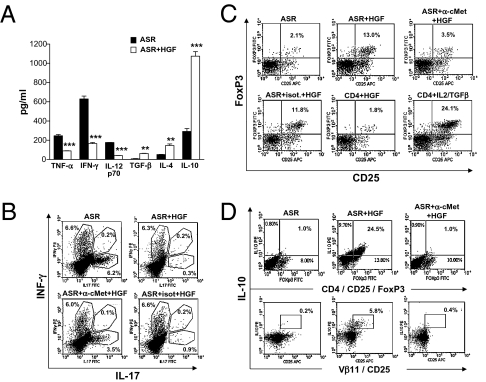
HGF-treated DC induce a Th2 cytokine bias and CD25+FoxP3+ Treg-dependant IL-10 production but decrease IL-17. (A) ELISA analysis of cytokines from ASR supernatant: TNF-α, IFN-γ, and IL12p70 are decreased, whereas TGF-β, IL-4, and IL-10 are increased. Results shown are mean ± SEM of triplicate experiments; rHGF added at 30 ng/mL. **, P < 0.01; ***, P < 0.001. (B) Intracellular staining of splenocytes from ASR experiments: CD4+ T-cell–dependant production of IL-17 was inhibited by rHGF-treated CD11c+ cells. Figures are representative of four independent experiments. (C) CD4+ T cells were stained for CD25-FoxP3 after CD11c+ cells were treated with rHGF (30 ng/mL) or left untreated. HGF-treated DC induce a strong increase in Treg cells. The effect of HGF on CD11c+ cells was inhibited by preincubation of CD11c+ cells with α-cMet–blocking antibody. HGF had no direct effect on CD4+ T cells, but IL-2/TGFβ–treated CD4+ T cells induced the production of CD25+FoxP3+ Treg cells (positive control). (D) IL-10 production (intracellular staining) was increased in CD25+FoxP3+ Treg cells and Vβ11(TCRMOG) CD25 T cells when T cells were cocultured with rHGF-treated CD11c+ cells. This effect was inhibited when CD11c+ cells were preincubated with α-cMet–blocking antibody. Figures are representative of four to six independent ASR experiments.
HGF-Treated DC Induce Expansion of CD25+Foxp3+ Treg Cells in Vitro with Increased IL-10 Production.
Because with rHGF pretreatment we observed a strong increase of IL-10 in both the spinal cord of HGF-Tg mice and the DC plus T-cell culture supernatant from ASR assays, we also examined by flow cytometry whether rHGF-treated DC could promote the induction of CD25+FoxP3+ Treg cells, known to be the major IL-10–producing subtype of T cells. Indeed, a strong increase in CD25+FoxP3+ Treg cells was observed when rHGF-treated DC, pulsed with MOG(35-55), were cocultured with TCRMOG T cells (Fig. 5C). This effect was abrogated when DC were pretreated with α-cMet antibody. Also, HGF had no direct influence on CD4+ T cells (in contrast to IL-2 plus TGF-β, which was used as positive control). Intracellular staining performed during ASR assays showed an increase of IL-10 in CD4+, CD4+CD25+ (Fig. S5), and CD4+CD25+Foxp3+ T cells (Fig. 5D) when DC were pretreated with rHGF. Again, this effect was reversed by preincubation of DC with α-cMet antibody. In addition, most IL-10+CD25+ T cells were TCRMOG T cells (TCR Vβ11+), demonstrating that MOG autoreactive transgenic T cells could be skewed toward a less inflammatory profile by rHGF-treated DC (Fig. 5D).
EAE Induced by Adoptive Transfer of TCRMOG T Cells Is Inhibited in HGF-Tg Mice.
To confirm our results further, we performed EAE experiments with adoptive transfer of TCRMOG T cells from 2D2 transgenic mice. EAE was inhibited significantly in HGF-Tg mice, compared with WT littermates, before peak disease was reached (dpi 15) (Fig. 6A). At peak disease (dpi 23), analysis of spinal cord-infiltrating inflammatory cells was performed by flow cytometry to evaluate CD25+ IL10-producing T cells as well as surface markers of T-cell activation. The results showed a strong increase in the CD25+IL10+ T-cell population in HGF-Tg mice as compared with WT mice (Fig. 6B). Analysis of T-cell activation markers at EAE peak disease confirmed our previous in vitro data (ASR; Fig. 4B) by showing that spinal cord CD4+ T cells were maintained in a state of low activation (CD44lowCD62L+) in HGF-Tg mice, whereas CD4+ T cells (CD44highCD62L−) were activated mostly in WT mice (Fig. 6C).

EAE induced by adoptive transfer of TCRMOG-specific (2D2) T cells is inhibited in HGF-Tg mice. Adoptive transfer of TCRMOG T cells in HGF-Tg mice (n = 10) and WT littermates (n = 12). (A) EAE was inhibited in HGF-Tg mice before peak disease was reached. Shown is the mean clinical score ± SEM at day 15 after adoptive transfer. *, P < 0.05. (B and C) Inflammatory cells infiltrating the spinal cord of HGF-Tg mice and WT littermates (n = 5 per group) at EAE peak disease (day 23 after adoptive transfer) were isolated by Percoll gradient and stained by flow cytometry. (B) (Left) Representative dot blots of CD25 and IL-10 analyses. (Right) CD25-dependent IL-10 production was increased in the spinal cord of HGF-Tg mice (mean ± SEM). **, P < 0.01. (C) Staining for CD44 and CD62L coreceptors showed that CD4+ T cells in the spinal cord of HGF-Tg mice were maintained in a low state of activation. (Left) Representative dot blots of CD62L CD44 analyses on T cells. (Right) Mean ± SEM, n = 3 per group. *, P < 0.05.
Discussion
HGF is a pleiotropic factor that acts by binding to the HGF tyrosine kinase receptor, cMet (11). HGF and cMet are expressed in brain-resident cells including neurons (24), mature oligodendrocytes (21, 22), OPC (17, 18, 22), and microglia (19). In addition to its neuroprotective effect, HGF is known to influence inflammation. However, whether its global immunomodulatory effect is pro- or antiinflammatory is still unclear. On the one hand, HGF is known to increase adhesion and migration of inflammatory cells of both the adaptive and the innate immune system (27–29). On the other hand, several antiinflammatory effects of HGF have been described, including (i) a Th2/T-helper cell type 3 (Th3) bystander deviation with increase of TGF-β and IL-10 (33, 40), (ii) inhibition of antigen-presenting cell (APC) function (40, 41), (iii) down-regulation of monocyte chemotactic protein-1 (MCP-1) and regulated upon activation, normal T cell expressed and secreted (RANTES) chemokines (42), and (iv) blocking of NF-κB function (42). All these effects could contribute to the protective action of HGF in EAE and/or multiple sclerosis (43, 44).
Here we show that overexpression of HGF in the CNS of transgenic mice inhibits the EAE clinical course by using two different methods of disease induction: MOG immunization and adoptive transfer of MOG-specific T cells. This finding is confirmed by histological observations that show a decrease of inflammatory lesions as well as a lower level of demyelination and axonal loss in HGF-Tg mice. Analyses by flow cytometry of inflammatory cell phenotypes in the spinal cord show that the total number of T cells and APCs was decreased in HGF-Tg mice during EAE, with the exception of the Treg cell population, which increased. Of note, HGF was up-regulated exclusively in the CNS and not in the systemic compartment, as demonstrated by the absence of a significant influence of the transgene on spleen cells. The reduction of CNS inflammation in HGF-Tg mice was associated with a decrease of proinflammatory (Th1) cytokines including TNF-α, INF-γ, and IL12p70, whereas the antiinflammatory (Th2) cytokine IL-10 was strongly increased. In vitro assays (i.e., ASR) mimicking immune response during EAE with DC stimulated by MOG with or without HGF and coincubated with TCRMOG T cells demonstrated (i) inhibition of DC function (including a decrease in costimulatory CD40 on DC and IL12p70 secretion together with an inhibition of T-cell proliferation), (ii) a cytokine Th2 bias similar to that detected in the spinal cord of EAE mice, (iii) induction of Foxp3+ Treg cells with a high proportion of IL-10–secreting cells, and (iv) inhibition of surface markers of T-cell activation. All these effects were driven by HGF-tolerized DC, and HGF had no direct effect on CD4+ T cells. In addition, the effects of HGF on DC were abrogated when DC were pretreated with blocking antibody directed against cMet, the HGF receptor, thereby indicating that the observed effects were related specifically to DC activation through the HGF–cMet pathway. Finally, EAE experiments were repeated using adoptive transfer of TCRMOG CD4+ T cells, and the results confirmed that inhibition of EAE in HGF-Tg mice is driven specifically through an increase of IL-10–producing Treg cells and maintenance of T cells in a low state of activation.
HGF has been shown previously to be protective in various animal models of immune-mediated diseases such as myocarditis (32, 33), glomerulonephritis (30, 34), inflammatory bowel disease (35), collagen-induced arthritis (36), pulmonary fibrosis (37), allogeneic heart transplantation (45), graft-vs.-host disease (46), and asthma (47). According to these studies, HGF may ameliorate both Th1-type–dominated (33, 36) and Th2-type–dominated (40, 47) autoimmune responses. Interestingly, a recent report demonstrated that, in an experimental model of airway allergy, HGF might repress DC function without up-regulation of IL-10 (40). In contrast, our data along with recent reports on murine models of cardiac transplantation (45) and autoimmune myocarditis (33) clearly show an HGF-dependant induction of both Treg cells and IL-10.
The HGF receptor cMet is not expressed on CD4+ and CD8+ T cells but can be detected on APC including CD11b+ monocyte-macrophages and CD11c+ DC. Of note, DC are the most efficient APC and are crucial in the EAE model, because the presence of DC alone is sufficient to present antigen in vivo to primed myelin-reactive T cells and to mediate CNS inflammation and development of clinical disease (43). Thus, a selective tolerization of DC as demonstrated in the present report could be sufficient to explain the inhibition of EAE. However, because cMet also is expressed on other APC, we cannot exclude completely the possibility that the protective effect of HGF observed in EAE also could be mediated by tolerized monocytes/macrophages.
Two plasminogen-related growth factors have been identified so far: HGF and HGF-like/macrophage-stimulating protein (HGFl/MSP) (48). The receptor for HGFl/MSP is a tyrosine receptor kinase, recepteur d’origine nantais (RON) (49), closely related to cMet, thereby suggesting a coevolution of these growth factors and their receptors. Of note, mice lacking RON show an exacerbation of symptoms during EAE, with overall worsened disease severity, increased demyelination, axonal loss, and neuroinflammation (50). Hence, these data suggest that both HGF and HGFl/MSP are protective in EAE.
In addition to its immunomodulatory effect, the role of HGF in regulating the survival, the differentiation, and the promotion of axonal outgrowth of various neuronal populations, including sensory, sympathetic, and motor neurons, has been firmly established (24). HGF is known to be a potent neuroprotective factor and also is able to induce differentiation of OPC into new myelin-forming cells (18). We recently demonstrated that HGF can be produced by microglia under TGF-β stimulation and can act as a chemotactic factor inducing migration of myelin-forming cells (i.e., OPC) into demyelinated lesions (17). Given that c-Met is expressed and phosphorylated in both OPCs and oligodendrocytes (22) and that activation of caspase-3 in oligodendrocytes can be attenuated by HGF application in the animal model of spinal cord injury (21), we cannot exclude the possibility that the beneficial effects of HGF overexpression in the CNS might be mediated partially via the prevention of oligodendrocytic cell degeneration. The importance of these mechanisms in the attenuation of EAE lesions in HGF-Tg mice remains to be determined.
In conclusion, our data demonstrate that HGF is a strong immunomodulator that inhibits EAE, a model of CNS autoimmunity closely resembling multiple sclerosis. HGF is able to induce tolerization of DC and to inhibit T-cell function at different levels. Our data are in accordance with recent publications showing that the immunomodulatory effects of HGF are mediated through APC tolerization and induction of an antiinflammatory (Th2) cytokine pattern (33, 40, 41). Along with the previous observations that HGF is a strong neuroprotector (24) and potentially could trigger remyelination (17, 18), our results suggest that HGF might be a promising candidate for the development of treatments for immune-mediated demyelinating diseases associated with neurodegeneration, such as multiple sclerosis.
Methods
Mice, Induction of EAE, and Isolation of CNS-Infiltrating Mononuclear Cells.
NSE-HGF-Tg mice were generously provided by H. Nakamura and T. Funakoshi (University of Osaka), and genotyping was performed as described (20). The transgenic founder mice (C57BL/6J background) were bred with WT C57BL/6J mice and backcrossed at least 15 times before EAE induction. 2D2 (TCRMOG) transgenic mice were generously provided by V. Kuchroo (Harvard Medical School). All mice were bred in house under pathogen-free conditions and were used at 8–10 weeks of age. Animal experiments were approved by the local veterinary office (Geneva, Switzerland) according to Swiss ethical regulations. For active immunization, female HGF-Tg mice and WT littermates were immunized with MOG(35-55) as described (51). For adoptive transfer, spleen cells from TCRMOG transgenic mice were stimulated with MOG(35-55) and IL-12p70 (R&D Systems) for 4 days. At day 5, CD4+ T cells were isolated and injected into recipient mice (7 × 106 cells per mouse). Animals received pertussis toxin (300 ng per mouse) on days 0 and 2 after T-cell transfer. Mice were assigned clinical scores daily as described (51). CNS mononuclear cells were isolated as described (52).
ASR Assays and T-Cell Proliferation Assay.
CD4+ T cells and CD11c+ cells were selected from spleen cells using MicroBeads (Miltenyi Biotec) according to the manufacturer’s protocol. DC were incubated with rHGF (eBioscience) (1–100 ng/mL) for 24 h. As a negative control, DC were preincubated with anti-cMet antibody (10 μg/mL; eBioscience). To examine the effect of HGF on Ag presentation, DC were pulsed with MOG(35-55) (20 μg/mL). After washing with PBS, DC (1 × 105/mL) were cocultured with CD4+ T cells (1 × 106/mL) obtained from 2D2 (TCRMOG) transgenic mice. Proliferation assays were performed as described elsewhere (43).
Methodologies are described in SI Methods.
RNA Isolation and Real-Time PCR.
RNA was extracted from spinal cords of mice. The spinal cords were flushed with ice-cold PBS, and RNA was isolated using RNAeasy Mini Kits (Qiagen) following the manufacturer’s instructions. The PCR was performed as described previously (20). Results were quantified relative to a standard curve generated with serial dilutions of a reference cDNA from a DC line and normalized using TATA-biding protein mRNA. Each experiment was repeated at least three times, and values were expressed as mean ± SEM.
Statistical Analysis.
Statistical analysis was performed using two-tailed Student’s t test. P < 0.05 was considered statistically significant.
Acknowledgments
We thank S. Izui for help in the study design and T. Moll for critically reading the manuscript. We thank C. Juillard, D. Bielser, and G. Brighouse for technical assistance. This study was supported by Grant 310000–113653 from the Swiss National Foundation to P.H.L. and by grants from the Swiss Multiple Sclerosis Society to P.H.L. and from the Alliance SEP Association to P.H.L. and M.C.
Footnotes
The authors declare no conflict of interest.
*This Direct Submission article had a prearranged editor.
This article contains supporting information online at www.pnas.org/cgi/content/full/0912437107/DCSupplemental.
References
Articles from Proceedings of the National Academy of Sciences of the United States of America are provided here courtesy of National Academy of Sciences
Full text links
Read article at publisher's site: https://doi.org/10.1073/pnas.0912437107
Read article for free, from open access legal sources, via Unpaywall:
https://europepmc.org/articles/pmc2851995?pdf=render
Free after 6 months at www.pnas.org
http://www.pnas.org/cgi/content/full/107/14/6424
Free after 6 months at www.pnas.org
http://www.pnas.org/cgi/reprint/107/14/6424.pdf
Free after 6 months at www.pnas.org
http://www.pnas.org/cgi/content/abstract/107/14/6424
Citations & impact
Impact metrics
Citations of article over time
Alternative metrics
Article citations
Impact of Neuron-Derived HGF on c-Met and KAI-1 in CNS Glial Cells: Implications for Multiple Sclerosis Pathology.
Int J Mol Sci, 25(20):11261, 19 Oct 2024
Cited by: 0 articles | PMID: 39457044 | PMCID: PMC11509024
Unveiling the Role of HGF/c-Met Signaling in Non-Small Cell Lung Cancer Tumor Microenvironment.
Int J Mol Sci, 25(16):9101, 22 Aug 2024
Cited by: 0 articles | PMID: 39201787 | PMCID: PMC11354629
Review Free full text in Europe PMC
Growth Factors and Their Application in the Therapy of Hereditary Neurodegenerative Diseases.
Biomedicines, 12(8):1906, 20 Aug 2024
Cited by: 0 articles | PMID: 39200370 | PMCID: PMC11351319
Review Free full text in Europe PMC
Elucidating the mechanism of corneal epithelial cell repair: unraveling the impact of growth factors.
Front Med (Lausanne), 11:1384500, 04 Apr 2024
Cited by: 0 articles | PMID: 38638937 | PMCID: PMC11024251
Review Free full text in Europe PMC
Umbilical cord-derived mesenchymal stem cell sheets transplanted subcutaneously enhance cell retention and survival more than dissociated stem cell injections.
Stem Cell Res Ther, 14(1):352, 10 Dec 2023
Cited by: 3 articles | PMID: 38072920 | PMCID: PMC10712142
Go to all (132) article citations
Data
Data behind the article
This data has been text mined from the article, or deposited into data resources.
BioStudies: supplemental material and supporting data
Similar Articles
To arrive at the top five similar articles we use a word-weighted algorithm to compare words from the Title and Abstract of each citation.
Hepatocyte growth factor limits autoimmune neuroinflammation via glucocorticoid-induced leucine zipper expression in dendritic cells.
J Immunol, 193(6):2743-2752, 11 Aug 2014
Cited by: 37 articles | PMID: 25114100
Involvement of regulatory T cells in the experimental autoimmune encephalomyelitis-preventive effect of dendritic cells expressing myelin oligodendrocyte glycoprotein plus TRAIL.
J Immunol, 178(2):918-925, 01 Jan 2007
Cited by: 30 articles | PMID: 17202353
Selective depletion of CD11c+ CD11b+ dendritic cells partially abrogates tolerogenic effects of intravenous MOG in murine EAE.
Eur J Immunol, 46(10):2454-2466, 01 Oct 2016
Cited by: 23 articles | PMID: 27338697 | PMCID: PMC5514845
Tolerance in the absence of autoantigen.
Endocr Metab Immune Disord Drug Targets, 7(3):203-210, 01 Sep 2007
Cited by: 6 articles | PMID: 17897047 | PMCID: PMC9811400
Review Free full text in Europe PMC