Abstract
Free full text

2-hydroxypropyl-β-cyclodextrin raises hearing threshold in normal cats and in cats with Niemann-Pick type C disease
Abstract
2-hydroxypropyl-β-cyclodextrin (HPβCD) is a promising experimental therapy for Niemann-Pick type C disease that improved intracellular cholesterol transport, substantially reduced neurodegeneration and hepatic disease, and increased lifespan in npc1−/− mice. Based on the favorable treatment outcome in mice, HPβCD is being evaluated as a therapy in children with NPC disease. We evaluated the efficacy of HPβCD in the feline model of NPC disease and recognized a dose-dependent increase in hearing threshold associated with therapy as determined by brain stem auditory evoked response (BAER) testing. To further assess the effect of HPβCD on hearing threshold, normal cats were administered the drug subcutaneously at either 4000 mg/kg or 8000 mg/kg body weight, or intrathecally at a dose of 4000 mg/kg brain weight. HPβCD caused a significant increase in hearing threshold following one dose of 8000 mg/kg subcutaneously or 120 mg intrathecally and the effect was maintained for at least twelve weeks. Repeated weekly subcutaneous administration of 4000 mg/kg HPβCD resulted in a similar increase in hearing threshold. These studies are the first to describe a specific negative effect of HPβCD on the auditory system and suggest the need for auditory testing in patients receiving similar doses of HPβCD.
Introduction
Cyclodextrins are cyclic oligosaccharides with hydrophobic interiors used as formulation vehicles to increase the amount of drug, including hormones and vitamins, which can be solubilized in aqueous vehicles (1). 2-hydroxypropyl-β-cyclodextrin (HPβCD) was extensively studied in rodents, dogs, and monkeys where it was generally well tolerated at low doses (1,2). Daily intravenous administration of greater than 200 mg/kg caused reduced body weight, foamy macrophage infiltration of the lungs, elevations in hepatic enzymes, increased Kuppfer cells in the liver, and renal cortical tubular vacuolization in rodents (1,3,4). All of these changes were reversible following cessation of HPβCD administration (1).
Niemann-Pick type C (NPC) disease is an incurable lysosomal storage disorder characterized by the intralysosomal accumulation of unesterified cholesterol, hepatosplenomegaly, progressive neurological dysfunction, and early death (5,6). Weekly intraperitoneal administration of 1500 mg/kg of HPβCD to npc−/− mice resulted in improvement in hepatic disease with no effect on neurological disease or lifespan (7). In contrast, the administration of a single subcutaneous dose of 4000 mg/kg of a 20% solution of HPβCD to 7-day–old npc−/− mice reversed the defect in the lysosomal transport of cholesterol and significantly improved hepatic dysfunction, decreased neurodegeneration, and prolonged lifespan (8). Every other day subcutaneous administration of 4000 mg/kg of a 20% solution of HPβCD to npc−/− mice was the most effective treatment regimen at ameliorating clinical disease and increasing lifespan, and also significantly decreased neuronal cholesterol, ganglioside and sphingosine accumulation, and decreased neuroinflammation (9). It was hypothesized that high doses of HPβCD were needed to ameliorate neurologic dysfunction because higher blood levels allowed more drug to cross the blood-brain barrier. An alternative hypothesis was that increased serum levels of HPβCD could bind enough circulating sterols to result in enhanced cholesterol egress from the central nervous system by an undefined mechanism (9). In each of these animal studies, no significant toxicity was observed following the administration of HPβCD except for increased macrophage infiltration of the lungs found at post mortem examination (9). Based on these data from the murine model, HPβCD has been approved for use in a group of children with NPC disease by the Food and Drug Administration (FDA).
Naturally-occurring NPC disease occurs in cats which have a missense mutation in NPC1 (2864G-C) with clinical, neuropathological, and biochemical abnormalities similar to those present in juvenile-onset patients making this model homologous to the most common form of the disease seen in human patients (10,11). Brain stem auditory evoked response testing (BAER) of cats with NPC disease showed a prolongation in central conduction time with no significant alteration in hearing threshold compared to wild type cats (11). While evaluating the efficacy of HPβCD to treat NPC disease in cats, we noted a significant elevation of hearing threshold in animals receiving repeated subcutaneous doses of 4000 mg/kg. To our knowledge, a negative effect of HPβCD on auditory function has not been evaluated in any species. This study investigated the effects of the subcutaneous and intrathecal administration of HPβCD treatment on the BAER of both normal cats and cats with NPC disease.
Methods
Animals
Cats were raised in the animal colony of the School of Veterinary Medicine, University of Pennsylvania, under NIH and USDA guidelines for the care and use of animals in research. The experimental protocol was approved by the University of Pennsylvania Institutional Animal Care and Use Committee.
All animals examined were produced from the same line bred to produce cats with autosomal recessively inherited Niemann-Pick type C (NPC) disease. All cats were housed at 21°C with ad libitum food and water, 12-hour light cycles, with 12–15 air changes per hour. Peripheral blood leukocytes from all cats were tested at one day of age for the NPC1 missense mutation using a PCR-based DNA test (10). Cats with two copies of the missense mutation were classified as affected with NPC disease while cats with one or no copies of the mutation were classified as normal. Heterozygote to heterozygote breedings produced ~25% affected and ~75% normal offspring.
Study groups
Normal cats were placed in one of five study groups (Table 1). Group 1 (n=7) were six month old and received no drug. Group 2 (n=3) received one dose of 4000 mg/kg body weight HPβCD subcutaneously at six months of age. Group 3 (n=3) received one dose of 8000 mg/kg body weight HPβCD subcutaneously at six months of age. Group 4 (n=3) received one dose of 4000 mg/kg brain weight (120 mg for a 30 gm brain weight) HPβCD intrathecally at six months of age. Intrathecal adminstration was achieved by anesthetizing cats with intravenous propofol (up to 6 mg/kg; Abbott Laboratories, Chicago, IL). A 20 gauge spinal needle was placed into the cerebellomedullary cistern and 1.0 ml of spinal fluid was removed. 0.6 ml of 20% HPβCD in saline was injected intrathecally over a two minute time period into the cerebellomedullary cistern. Group 5 (n=3) received weekly 4000 mg/kg body weight HPβCD subcutaneously beginning at eight weeks of age for a total of seven doses.
Table 1
Summary of groups of normal cats treated with hydroxypropyl-beta-cyclodextrin (HPβCD)
Group | Number of cats | Dose and method of administration of HPβCD |
---|---|---|
1 | 7 | NA |
2 | 3 | 4000 mg/kg body weight subcutaneously once |
3 | 3 | 8000 mg/kg body weight subcutaneously once |
4 | 3 | 4000 mg/kg brain weight intrathecally once |
5 | 3 | 4000 mg/kg body weight subcutaneously weekly for 7 doses |
NA = not applicable
Cats affected with NPC disease were already involved in a clinical study evaluating the efficacy of HPβCD to treat disease. All cats were first administered the drug at three weeks of age, prior to the onset of clinical signs of disease, and continued to receive the drug weekly thereafter. Cats were placed into one of five groups (Table 2). Group 6 (n=8) received no HPβCD and served as the control group for cats with NPC disease. Groups 7 (n=5), 8 (n=2), and 9 (n=5) received a weekly dose of 1000 mg/kg HPβCD body weight subcutaneously, 4000 mg/kg body weight HPβCD subcutaneously, and 8000 mg/kg body weight HPβCD subcutaneously respectively. Group 10 (n=2) received 4000 mg/kg brain weight (120 mg for a 30 gm brain weight) HPβCD intrathecally every 2 weeks (intrathecal administration methods described above).
Table 2
Summary of groups of cats with NPC disease treated with hydroxypropyl-beta-cyclodextrin (HPβCD)
Group | Number of cats | Dose and method of administration of HPβCD |
---|---|---|
6 | 8 | NA |
7 | 5 | 1000 mg/kg body weight subcutaneously weekly |
8 | 2 | 4000 mg/kg body weight subcutaneously weekly |
9 | 5 | 8000 mg/kg body weight subcutaneously weekly |
10 | 2 | 4000 mg/kg brain weight intrathecally every two weeks |
NA = not applicable
HPβCD formulations
All HPβCD was administered in a 20% (weight/volume) solution dissolved in 0.9% sodium chloride. HPβCD was received from Sigma and the powdered form (HPβCD-H107; Sigma Aldrich, St Louis MO) was used in all subcutaneous administrations and the cell-culture tested form (HPβCD-C0926; Sigma Aldrich, St Louis MO) was used for all intrathecal administrations. As a control for the saline injection, addition normal cats were injected one time subcutaneously (n=2) and intrathecally (n=2) with similar volumes of saline.
To control for possible differences between HPβCD available from Sigma, the product used in published mouse studies (8,9) and the FDA-approved formulation for use in patients (Trappsol – Pharm grade, Cyclodextrin Technologies Development, Inc, High Springs, FL), Trappsol was administered to four cats: one dose of 8000 mg/kg body weight subcutaneously (n=2) and one dose of 4000 mg/kg brain weight intrathecally (120 mg for a 30 gm brain weight; n=2).
Brain stem auditory evoked response testing (BAER)
All measurements of the BAER were obtained from cats given atropine sulfate (0.02 mg/kg; Butler Animal Health Supply, Dublin, OH) and then anesthetized with intravenous propofol (up to 6 mg/kg). The BAER data were recorded using 12 mm, 29 gauge subdermal needle electrodes and a Nicolet Viking Quest signal analyzer (Nicolet Biomedical, Madison, WI). The active electrode was placed over the osseous bulla of the stimulated ear, the reference electrode was situated over the vertex of the skull, and the ground electrode over the contralateral osseous bulla. All recording electrodes were placed subcutaneously. Alternating rarefaction and condensation clicks were produced by connecting a square wave pulse (0.1 ms in duration) to a speaker (Model TIP-300; Nicolet Biomedical, Madison, WI). The stimuli were presented monaurally at a rate of 11.1 Hz using a 25 cm plastic tube of 1.5 mm diameter which was connected to the speaker at one end, with the other end laid in the unsealed ear canal (an open field stimulus). The stimulator delivered a 125 dB pSPL click to the recorded ear and delivered a 85 dB SPL white noise to the contralateral ear. The high pass filter on the amplifier was 20 Hz and the low pass cutoff was 3 kHz. A sensitivity of 1 uV/cm was used to record the responses and the averaging epoch was 10 ms with a sampling resolution of .01 ms. One thousand evoked responses were averaged for each BAER response obtained. Central conduction time was defined as the time between the first and the fifth peak. Wave V/I amplitude was determined by dividing the amplitude of the fifth wave by the amplitude of the first wave and multiplying by 100; amplitude was measured from peak to trough and expressed as microvolts. A modified method of limit procedure was used to estimate threshold. When a clearly defined BAER was identified at the reference stimulus of 125 dB, the attenuator was then increased in 3 dB steps and a signal averaged response was sought at each step. If an evoked response was observed, the attenuator was then increased by another 3 dB and the BAER response again observed. This continued until a sound level was reached at which an averaged evoked response could not be identified.
In normal cats, BAER studies were performed every other week following the administration of HPβCD in Groups 2, 3, and 4 for a total of 12 weeks, and were performed every week in group 5 for a total of 12 weeks. In cats with NPC disease, BAER studies were performed at sixteen weeks of age.
Statistical methods
The mean and standard deviation of the thereshold, central conduction time, and wave V/I ratio estimates in each group were calculated to describe the data and an unpaired 2-tailed t test was used to compare data between various groups. Significance values of p<0.05 (*) are given. Threshold difference between groups were considered statistically reliable if the probability of chance occurrence was 0.05 or less.
Results
Normal cats
None of the normal animals that received either subcutaneous or intrathecal HPβCD injections showed evidence of loss of balance or ataxia at any point during the study. No clinical signs were attributable to HPβCD administration aside from pain at the subcutaneous injection site which was common in cats receiving weekly doses. Subjective evaluation of hearing was difficult to perform since normal, untreated colony-bred animals frequently do not respond repeatably to sound. Detailed behavioral testing was not performed.
A single subcutaneous dose of 4000 mg/kg HPβCD evoked waveforms the same as in cats which received no HPβCD (Figure 1, Groups 1 and 2). In contrast, a single subcutaneous dose of 8000 mg/kg HPβCD resulted in diminished waveform amplitude with changes severe enough to make specific waveforms difficult to identify (Figure 1, Group 3). Similarly, a single intrathecal dose of 120 mg HPβCD resulted in altered evoked responses characterized by reduced amplitude (Figure 1, Group 4). A single injection of intrathecal saline left the BAER unchanged in two cats (data not shown).
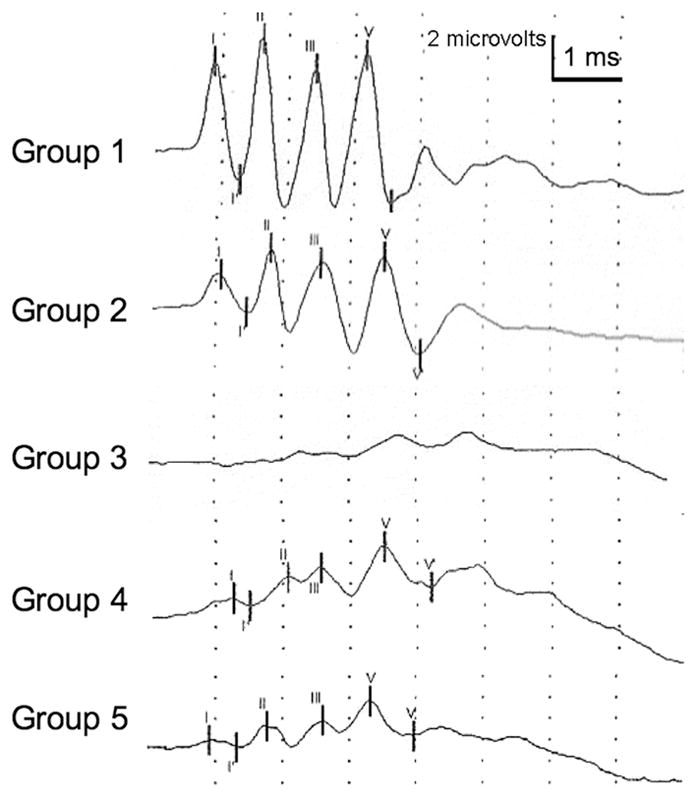
Representative brainstem auditory evoked responses for normal cats following the administration of HPβCD (Group 2–5). Recordings for Groups 2–4 were made two weeks after a administering a single drug dose. The recording for Group 5 was made one week after the sixth weekly drug dose. A decrease in waveform amplitude was noted in Groups 3, 4, and 5 compared to untreated cats (Group 1). Changes in Group 3–5 were severe enough that waveforms could not always be reliably identified (an example is given for Group 3).
Hearing threshold, wave V/I amplitude, and central conduction time were measured for groups 1–5 (Table 3). Cats in groups 3 and 4 showed a significant increase in hearing threshold two weeks following injection compared to uninjected cats (Group 1). The average click BAER threshold in control cats was 66 dB, whereas in Groups 3 and 4 that were treated with 4000 mg/kg and 8000 mg/kg HPβCD, repectively, the threshold increased to 79 and 81 dB respectively. This approximately 13 dB difference between groups was statistically reliable (p< 0.05). Differences in wave V/I amplitude or in central conduction time were due to random sampling and were statistically insignificant among the groups. The BAER of cats in groups 2, 3, and 4 remained unchanged, neither improving or worsening, during the 12 week study following a single administration of HPβCD (data not shown).
Table 3
Hearing threshold, wave V/I amplitude and central conduction time two weeks after administering a single dose of HPβCD to normal cats (Groups 2–4) and one week following the sixth dose (Group 5).
Hearing threshold dB SPL) | Wave V/I amplitude | Central conduction time (ms) | |
---|---|---|---|
Group 1 (n=7) | 66.4 +/− 3.2 | 181 +/− 16.7 | 2.41 +/− 0.09 |
Group 2 (n=3) | 63.0 +/− 5.2 | 212 +/− 24.0 | 2.43 +/− 0.02 |
Group 3 (n=3) | 79.0 +/− 4.6* | 545 +/− 402 | 2.36 +/− 0.02 |
Group 4 (n=3) | 81.0 +/− 3.0* | 525/− 127 | 2.37 +/− 0.05 |
Group 5 (6 doses of HPβCD) (n=3) | 80.0 +/− 1.7* | 469 +/− 423 | 2.38 +/− 0.09 |
Cats in Group 5 received seven weekly injections of 4000 mg/kg HPβCD subcutaneously. Interestingly, while these cats showed no significant elevation in hearing threshold two weeks after the first injection (data not shown), repeated weekly injections of the same dose resulted in a progressive elevation of hearing threshold with the threshold on the fourth through seventh week significantly greater than the hearing threshold observed prior to injection (week 0) (Table 3; Figure 2).
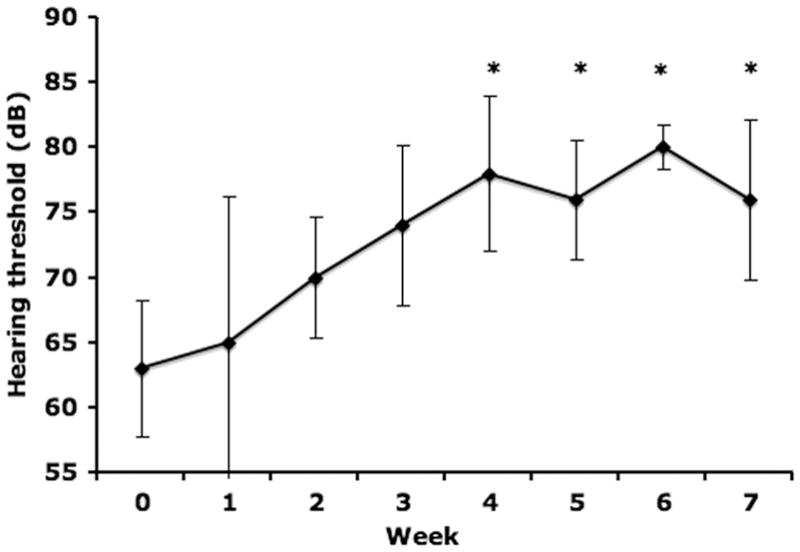
Weekly hearing thresholds following 4000 mg/kg weekly HPβCD administration (Group 5; n=3). The first dose was administered immediately after Week 0 threshold testing. Repeated HPβCD administration resulted in progressive elevation of the hearing threshold with a statistically significant (p<0.05) increase from weeks 4 to 7 when compared to week 0.
Cats with NPC disease
Affected cats began weekly subcutaneous therapy with HPβCD at three weeks of age in an attempt to ameliorate disease progression. At 16 weeks of age, hearing thresholds were statistically the same in normal cats (Group 1, 66.4 dB +/− 3/2 dB) and cats with NPC disease (Group 6, 71.9 dB +/− 7.9 dB). Interestingly, significant differences were absent between cats treated subcutaneously with 1000 mg/kg HPβCD (65 dB +/− 7.5 dB; Group 7) and untreated cats (Group 6) (Figure 3). However, both animals treated with weekly subcutaneously administered 4000 mg/kg (Group 8) had higher hearing thresholds than any untreated cats with NPC disease. Similarly, cats treated with weekly subcutaneously administered 8000 mg/kg (91.8 dB +/− 16.4 dB; Group 9) had significantly greater hearing threshold compared to untreated cats with NPC disease. Finally, both cats given HPβCD intrathecally (every other week) had no click evoked waveforms even at the highest sound intensity of 125 dB. Where waveforms could be reliable discerned, the wave V/I amplitude and the central conduction time did not differ between untreated cats with NPC disease and cats treated with HPβCD.
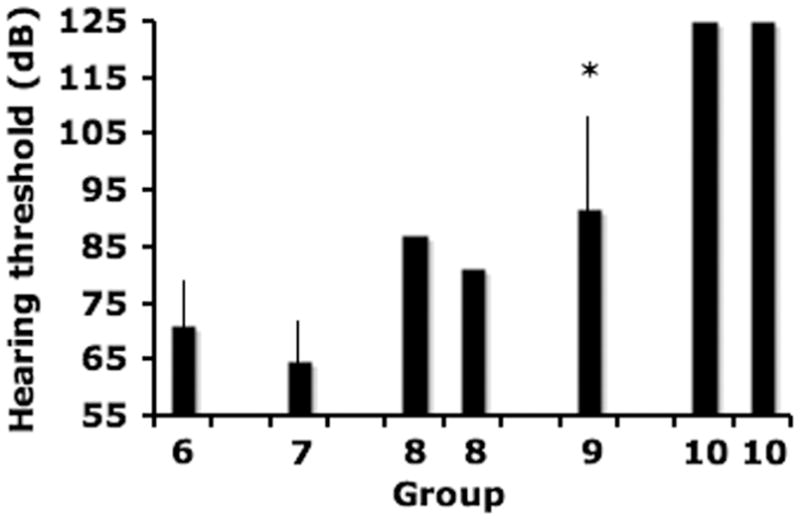
Cats with NPC disease cats showed no increase in hearing threshold following weekly administration of 1000 mg/kg HPβCD (Group 7; n=5) compared to untreated affected cats (Group 6; n=8). Both cats treated with weekly 4000 mg/kg (Group 8; n=2) showed an increase in hearing threshold and cats treated with weekly 8000 mg/kg (Group 9; n=5) had a statistically significant (p<0.05) increase in threshold. No waveforms were evoked at the maximum stimulus intensity of 125 dB from two cats treated with every other week intrathecal HPBCD (Group 10).
Normal cats receiving Trappsol
Trappsol was administered subcutaneously to two normal cats as a single dose of 8000 mg/kg body weight and intrathecally to two normal cats as a single dose of 4000 mg/kg brain weight (120 mg for a 30 gm brain weight) in the same manner as the Sigma product was administered. BAER recordings were first made within 15 minutes of subcutaneous and intrathecal administration and no changes in the waveforms were noted (data not shown). Figures 4A and 4C show the BAER recordings prior to Trappsol administration in two cats. Figure 4B shows the BAER recording one week following the administration of HPβCD intrathecally and figure 4D shows the tracing obtained one week following the administration of subcutaneous HPβCD. The administration of Trappsol to normal cats resulted in changes to the BAER similar to that seen with the Sigma product. Hearing threshold in two cats treated intrathecally increased from 69 dB and 72 dB prior to drug administration to 87 dB and 90 dB following administration. Hearing threshold in two cats treated subcutaneously increased from 69 dB and 72 dB prior to drug administration, to 87 dB in both cats following drug administration.
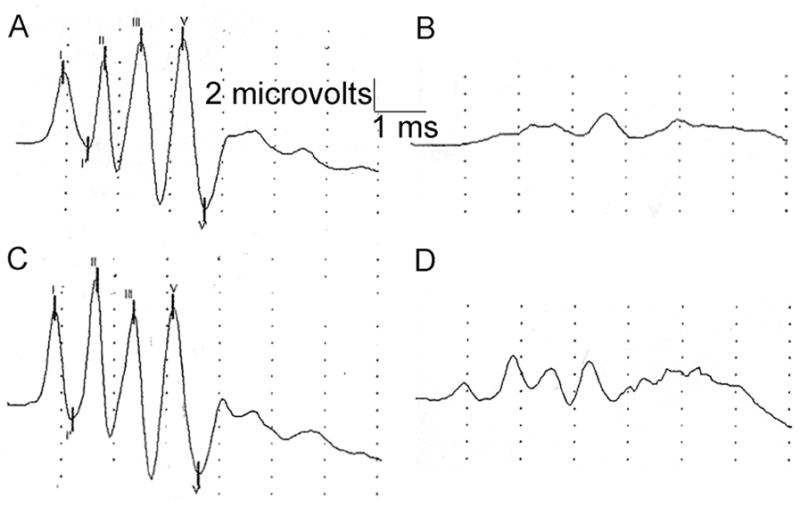
Normal cats treated with either intrathecal or subcutaneous Trappsol showed abnormal waveforms one week following administration. Figure A shows BAER tracing immediately prior to intrathecal injection of 120 mg Trappsol; Figure B shows BAER in same cat one week later. Figure C shows BAER tracing immediately prior to subcutaneous injection of 8000 mg/kg Trappsol; Figure D shows BAER in same cat one week later.
Discussion
Niemann-Pick type C disease has an incidence of 1:150000, with over 250 disease-causing mutations identified (5). Natural history studies and therapy trials are difficult to perform on this disorder due to the relatively low incidence and the heterogeneity of disease in human patients. One particular missense mutation represents more than 20% of mutant alleles and greater than 50% of patients have a juvenile onset of neurological disease. The feline model of NPC disease has a spontaneously-occurring missense mutation in NPC1 (2864G-C) and exhibits clinical, neuropathological and biochemical abnormalities similar to those present in juvenile-onset patients. Thus the cat model is homologous to the most common form of disease seen in human patients (10, 12–17). The feline model has been useful for identifying the late endosomal/lysosomal accumulation of unesterified cholesterol and gangliosides (GM2 and GM3) (18), for evaluating the association of GM2 storage with meganeurite formation and abnormal dendritogenesis (19), for correlating neuroaxonal dystrophy with neurological dysfunction (16), and for evaluating efficacy of experimental therapies (10,13). The onset and progression of neurological dysfunction in the feline model has been well-characterized (11,15). A regular onset of progressive cerebellar and vestibular dysfunction occurred in affected cats beginning with intention tremors and ataxia at six weeks of age. This dysfunction progressed until cats could no longer maintain sternal recumbency at approximately 24 weeks of age. Changes in hearing threshold were not found although a delay in central conduction time and a decrease in wave V/I amplitude ratio was observed in 16 and 24 week old affected cats compared to wild type cats (11).
2-hydroxypropyl-β-cyclodextrins (HPβCDs) are cyclic oligosaccharides consisting of seven β-(1–4)-glucopyranose units (7). HPβCDs have a hydrophilic exterior and a hydrophobic interior making them useful for increasing the aqueous solubility of hydrophobic molecules such as cholesterol, steroids, and vitamins (20). In vitro studies using β-cyclodextrins have shown a marked removal of cholesterol from cultured neuronal (21,22) and non-neuronal cell lines (23–25). HPβCDs were shown to cross the blood brain barrier in in vitro (25) and in in vivo with difficulty (7,26). However, β-cyclodextrins were safely administered intrathecally in rodent studies and used to improve the delivery to the brain of drugs including anesthetic agents, galanin-like peptide, and estradiol (27–29).
Recently, HPβCD was shown to release cholesterol from NPC-deficient lysosomes and allowed unesterified cholesterol to be available to the NPC cell. This resulted in the amelioration of disease and the prolongation of life in the murine model (8,9,30). However, high doses of HPβCD (at least 4000 mg/kg) however appeared necessary to retard the progression of neurological disease. Studies in npc1−/− mice showed that 1500 mg/kg HPBCD administered weekly caused a decrease in hepatic unesterified cholesterol concentrations without substantial effect on neurological signs (7). Increasing the dose to either 4000 mg/kg weekly or every other day delayed clinical disease onset, increased survival time, corrected cholesterol metabolism, and improved biochemical and histological disease (8,9). Since s-cyclodextrins do not easily penetrate the blood brain barrier (7,25), these studies suggested that parenteral administration of high doses of HPβCD are necessary to get sufficient amounts of HPβCD to cross the blood brain barrier and to have an effect on neurological disease. Unfortunately, the pharmacokinetics of HPβCD are not well understood particularly in the nervous system. A plasma elimination half-life in rats was 0.4 hours and in dogs was 0.8 hours although the concentration in cerebrospinal fluid after systemic administration was not described (1). Most recently, in mice, subcutaneously administered HPBCD was cleared by greater than 90% from the plasma six hours after administration (31). Serum and cerebrospinal measurements of unlabeled HPβCD are technically difficult to perform and these concentrations were not determined in the recent murine papers (8,9,30). Clearly, the kinetics of HPβCD in serum and spinal fluid will be necessary in order to clarify how HPβCDs effect neurological dysfunction in NPC disease and to determine what dose is most efficacious while also limiting toxicity.
As a result of the dramatic improvement in clinical signs seen in the mouse model of NPC disease, HPβCD was given recent FDA approval for use in a small number of patients with NPC disease. This has increased the urgency to more fully characterize any dose-related potential toxic effects of the drug. In humans, intravenous administration of up to 3 grams in healthy volunteers was well-tolerated and doses of 16 grams per day given with itraconazole did not result in hearing abnormalities (4). The authors could not find examples of doses of 1000 mg/kg and higher being used in human patients although these are being proposed to treat patients with NPC disease. The authors are aware of no previous study examining the effect of HPβCD on auditory function and yet we were able to determine an effect on hearing using a small number of normal cats and cats with NPC disease. Our data show that 1000 mg/kg had no effect on the BAER response when given weekly for 14 doses between the ages of three and 16 weeks of age. Doses of 4000 mg/kg body weight resulted in an increase in hearing threshold only after repeated dosing and doses of 8000 mg/kg body weight resulted in significant increases in hearing threshold in both normal cats and cats with NPC disease following the administration of a single dose. Interestingly, the doses needed to negatively impact the BAER response was the same dose necessary to retard nervous system dysfunction in mice. Our preliminary data in cats affected with NPC disease suggest a similar requirement for doses equal to or greater than 4000 mg/kg to positively affect neurological disease (data not shown). One conclusion that suggested itself is that doses of greater than 4000 mg/kg are necessary in order for HPβCD to cross the blood brain barrier and that the effect on hearing is related to the ability of the drug to enter the CNS. Our data on the effect of intrathecal administration of HPβCD on hearing threshold supported the conclusion that the drug had its negative effect on hearing only after it entered the spinal fluid. Importantly, whether the drug was given subcutaneously or intrathecally, the negative effect of HPβCD on the auditory system was not ameliorated up to 12 weeks following the cessation of drug administration suggesting that the effects may be irreversible.
The increased hearing threshold with no change in central conduction time suggested that the damage from HPβCD occurred in the peripheral auditory pathway (cochlea or eighth nerve) and that potential mechanisms of action for the hearing loss observed include a direct effect on the stria vascularis and its role in maintaining the ionic environment of the inner ear fluid space, the transduction and motility mechanisms of inner and outer hair cells, and/or the excitation patterns in the auditory nerve discharges. Identifying the site of action of HPβCD within the peripheral auditory system will likely be the first step in overcoming toxicity of HPβCD applications. Otoacoustic emission testing would be a useful method for evaluating outer hair cell function in cats but was unavailable for these studies. Histopathology of the cochlea should be performed in the future to identify any pathological changes.
In summary, hearing impairment following HPβCD administration appeared to be both dose-dependent and long-lasting and may be a limiting factor in the use of this drug at high doses to treat Niemann-Pick type C disease. Auditory testing is recommended for patients receiving doses of 4000 mg/kg HPβCD or greater in order to evaluate the effect on hearing threshold in these patients.
Acknowledgments
This work was supported by grants from the Ara Parseghian Medical Research Foundation, Dana’s Angels Research Trust and NIH grant RR02512.
The authors acknowledge the critical review of the manuscript by Drs. Shel Steinberg and James Saunders. Trappsol was provided by Dr. Rick Stratton.
Abbreviations
- BAER
- brain stem auditory evoked response
- HPβCD
- hydroxypropyl-beta-cyclodextrin
- NPC
- Niemann-Pick type C
References
Full text links
Read article at publisher's site: https://doi.org/10.1203/pdr.0b013e3181df4623
Read article for free, from open access legal sources, via Unpaywall:
https://www.nature.com/articles/pr2010130.pdf
Citations & impact
Impact metrics
Citations of article over time
Alternative metrics
Smart citations by scite.ai
Explore citation contexts and check if this article has been
supported or disputed.
https://scite.ai/reports/10.1203/pdr.0b013e3181df4623
Article citations
Advances in research on potential therapeutic approaches for Niemann-Pick C1 disease.
Front Pharmacol, 15:1465872, 28 Aug 2024
Cited by: 0 articles | PMID: 39263569 | PMCID: PMC11387184
Review Free full text in Europe PMC
Differently increased volumes of multiple brain areas in Npc1 mutant mice following various drug treatments.
Front Neuroanat, 18:1430790, 16 Jul 2024
Cited by: 0 articles | PMID: 39081805 | PMCID: PMC11286580
Lysosomal cholesterol accumulation is commonly found in most peroxisomal disorders and reversed by 2-hydroxypropyl-β-cyclodextrin.
Sci China Life Sci, 66(8):1786-1799, 23 Mar 2023
Cited by: 2 articles | PMID: 36971991
Structure-function relationships of cholesterol mobilization from the endo-lysosome compartment of NPC1-deficient human cells by β-CD polyrotaxanes.
PLoS One, 17(12):e0268613, 30 Dec 2022
Cited by: 0 articles | PMID: 36584173 | PMCID: PMC9803220
Acat1/Soat1 knockout extends the mutant Npc1 mouse lifespan and ameliorates functional deficiencies in multiple organelles of mutant cells.
Proc Natl Acad Sci U S A, 119(18):e2201646119, 04 May 2022
Cited by: 14 articles | PMID: 35507892 | PMCID: PMC9170141
Go to all (74) article citations
Other citations
Data
Data behind the article
This data has been text mined from the article, or deposited into data resources.
BioStudies: supplemental material and supporting data
Diseases
- (1 citation) OMIM - 150000
Similar Articles
To arrive at the top five similar articles we use a word-weighted algorithm to compare words from the Title and Abstract of each citation.
Intracisternal cyclodextrin prevents cerebellar dysfunction and Purkinje cell death in feline Niemann-Pick type C1 disease.
Sci Transl Med, 7(276):276ra26, 01 Feb 2015
Cited by: 112 articles | PMID: 25717099 | PMCID: PMC4415615
Intrathecal 2-hydroxypropyl-β-cyclodextrin decreases neurological disease progression in Niemann-Pick disease, type C1: a non-randomised, open-label, phase 1-2 trial.
Lancet, 390(10104):1758-1768, 10 Aug 2017
Cited by: 174 articles | PMID: 28803710 | PMCID: PMC6176479
Intravenous 2-hydroxypropyl-β-cyclodextrin (Trappsol® Cyclo™) demonstrates biological activity and impacts cholesterol metabolism in the central nervous system and peripheral tissues in adult subjects with Niemann-Pick Disease Type C1: Results of a phase 1 trial.
Mol Genet Metab, 137(4):309-319, 17 Oct 2022
Cited by: 11 articles | PMID: 36279795
2-Hydroxypropyl-β-cyclodextrins and the Blood-Brain Barrier: Considerations for Niemann-Pick Disease Type C1.
Curr Pharm Des, 23(40):6231-6238, 01 Jan 2017
Cited by: 16 articles | PMID: 29065825 | PMCID: PMC5824462
Review Free full text in Europe PMC
Funding
Funders who supported this work.
NCRR NIH HHS (3)
Grant ID: P40 RR002512-228570
Grant ID: RR02512
Grant ID: P40 RR002512
NIH HHS (1)
Grant ID: P40 OD010939
NINDS NIH HHS (1)
Grant ID: R01 NS073661