Abstract
Objectives
The purpose of this study was to test the hypothesis that a small heart coupled with reduced blood volume contributes to the postural orthostatic tachycardia syndrome (POTS) and that exercise training improves this syndrome.Background
Patients with POTS have marked increases in heart rate during orthostasis. However, the underlying mechanisms are unknown and the effective therapy is uncertain.Methods
Twenty-seven POTS patients underwent autonomic function tests, cardiac magnetic resonance imaging, and blood volume measurements. Twenty-five of them participated in a 3-month specially designed exercise training program with 19 completing the program; these patients were re-evaluated after training. Results were compared with those of 16 healthy controls.Results
Upright heart rate and total peripheral resistance were greater, whereas stroke volume and cardiac output were smaller in patients than in controls. Baroreflex function was similar between groups. Left ventricular mass (median [25th, 75th percentiles], 1.26 g/kg [1.12, 1.37 g/kg] vs. 1.45 g/kg [1.34, 1.57 g/kg]; p < 0.01) and blood volume (60 ml/kg [54, 64 ml/kg] vs. 71 ml/kg [65, 78 ml/kg]; p < 0.01) were smaller in patients than in controls. Exercise training increased left ventricular mass and blood volume by approximately 12% and approximately 7% and decreased upright heart rate by 9 beats/min [1, 17 beats/min]. Ten of 19 patients no longer met POTS criteria after training, whereas patient quality of life assessed by the 36-item Short-Form Health Survey was improved in all patients after training.Conclusions
Autonomic function was intact in POTS patients. The marked tachycardia during orthostasis was attributable to a small heart coupled with reduced blood volume. Exercise training improved or even cured this syndrome in most patients. It seems reasonable to offer POTS a new name based on its underlying pathophysiology, the "Grinch syndrome," because in this famous children's book by Dr. Seuss, the main character had a heart that was "two sizes too small."Free full text

Cardiac Origins of the Postural Orthostatic Tachycardia Syndrome
Abstract
Objectives
To test the hypothesis that a small heart coupled with reduced blood volume contributes to the Postural Tachycardia Syndrome (POTS), while exercise training improves this syndrome.
Background
Patients with POTS have marked increases in heart rate during orthostasis. However, the underlying mechanisms are unknown and the effective therapy is uncertain.
Methods
Twenty-seven POTS patients underwent autonomic function tests, cardiac MRI, and blood volume measurements. Twenty-five of them participated in a 3-mo specially designed exercise training program with 19 completing the program; these patients were reevaluated after training. Results were compared with those of 16 healthy controls.
Results
Upright heart rate and total peripheral resistance were greater, while stroke volume and cardiac output were smaller in patients than controls. Baroreflex function was similar between groups. Left ventricular mass (median [25%, 75%], 1.26 [1.12, 1.37] vs 1.45 [1.34, 1.57] g/kg; P<0.01) and blood volume (60 [54, 64] vs 71 [65, 78] ml/kg; P<0.01) were smaller in patients than controls. Exercise training increased left ventricular mass and blood volume by ~12% and ~7%, and decreased upright heart rate by 9 [1, 17] bpm. Ten out of 19 patients no longer met POTS criteria after training, while patients’ Quality of Life assessed by Short-Form 36 was improved in all patients after training.
Conclusions
Autonomic function was intact in POTS patients. The marked tachycardia during orthostasis was attributable to a small heart coupled with reduced blood volume. Exercise training improved or even cured this syndrome in the majority of patients. It seems reasonable to offer POTS a new name based on its underlying pathophysiology – “The Grinch Syndrome”, because in this famous children’s book by Dr. Seuss, the main character had a heart that was “two sizes too small.”
INTRODUCTION
Young women are more susceptible to orthostatic intolerance than similarly aged men (1–3), and this sex difference is more dramatic in the Postural Orthostatic Tachycardia Syndrome (POTS, also called Chronic Orthostatic Intolerance), in which patients are unable to stand or remain upright for prolonged periods of time due to intolerable light-headedness, weakness, and near-syncope. This disorder affects over 500,000 Americans (3), the vast majority of whom are premenopausal women. Severely affected patients are unable to work, attend school, or participate in recreational activities resulting in substantial morbidity. However, the underlying mechanisms remain unknown and the effective therapy is uncertain.
Sex differences in orthostatic tolerance become more dramatic after spaceflight or a period of bed rest (4,5), in which “deconditioning” occurs. Numerous studies have shown that real or simulated microgravity exposure can elicit a “POTS-like” syndrome even in healthy fit individuals. The induced tachycardia during orthostasis has been found to be associated with reduced stroke volume, which is attributable to cardiac atrophy and hypovolemia (6,7). Indeed, with chest roentgenographic and echocardiographic techniques, it was observed that the heart was much smaller in patients with chronic fatigue syndrome, a condition with substantial overlap with POTS, than healthy controls (8). Decreases in blood volume have been reported in many POTS patients (9,10). In addition, the majority of these patients have significant limitations to even low-intensity physical activity (11–13). Based on these observations, we speculated that POTS per se may be a consequence or signature of “deconditioning”, namely, cardiac atrophy and hypovolemia. However, this speculation needs to be verified.
Clarifying the pathophysiology of POTS is essential for determining optimal evidence based therapy, particularly since many of these patients have disabling side effects with drug treatment. One non-drug therapy that has shown some promise is exercise training. For example, increased orthostatic tolerance following mild to moderate exercise training was reported in patients with unexplained syncope or orthostatic hypotension (14,15). Physical exercise training has been shown to increase plasma and blood volume (16). However, its effect on orthostatic tolerance in healthy individuals is controversial (17–19). Whether exercise training can be regarded as an effective therapy for patients with POTS needs to be determined.
The primary objective of this study was to test the hypothesis that POTS would be attributable to a small heart coupled with reduced blood volume (i.e., “deconditioning”), while exercise training could improve or even cure this syndrome by targeting the underlying pathophysiology.
METHODS
Study population
The patient population consisted of 54 consecutive patients referred to our tertiary Autonomic Function Clinic between December 2004 and April 2008. Eight patients refused immediately after we contacted them, because they were not interested in participating in research. Forty-six patients were screened, while 18 of them declined as they were not willing to be off medications for several months and to undergo all the comprehensive assessments prior to treatment. Twenty-eight patients (27 women, 1 man) were eventually enrolled in the study. The severity of POTS was not different between those who declined participation and those who were enrolled in the study. Among these 28 patients, one was diagnosed with Ehlers Danlos Syndrome after baseline evaluations and was thereafter excluded from the study, because this syndrome may affect cardiac size and function (20). All patients met the inclusion without exclusion criteria for POTS (12), and had a heart rate (HR) rise > 30 bpm or a rate that exceeded 120 bpm that occurred after 10 min of standing without any evidence of orthostatic hypotension (9). About 55% of them had mild POTS (i.e., an increase in HR ≤ 35 bpm), while 45% had moderate to severe POTS (i.e., an increase in HR > 35 bpm). Most patients had been treated at some point with “standard” medications such as β-blockers, volume expanders, and α1-adrenergic agonists. Patients had stopped taking medications that could affect the autonomic nervous system ≥ 2 wks before screening and testing.
Healthy controls were recruited from the Dallas-Fort Worth area. Approved flyers were posted in an advertisement format in locations such as local recreational centers, churches, grocery stores, colleges, and shopping centers. Potential subjects were asked to contact our recruiting staff to inquire about the study. Our experienced recruiting nurse performed initial telephone screening. If they did not have any exclusion criteria and appeared to have a sincere interest after explanation of the study purpose and requirements, they were invited to our laboratory for a formal screening. Sixteen healthy controls (15 women, 1 man) were eventually enrolled. “Matching of Groups” was employed in this study with the goal for the patient group and the control group to be comparable with regard to demographics and confounders; the groups had approximately the same mean age, sex, height, weight, and body mass index.
All participants were non-smokers. None was an endurance-trained athlete (19,21). All were screened with a careful medical history, physical examination, 12-lead electrocardiogram (ECG), and a 10-min stand test. All participants were informed of the purpose and procedures used in the study and gave their written informed consent to a protocol approved by the Institutional Review Boards of the University of Texas Southwestern Medical Center and Texas Health Presbyterian Hospital Dallas.
Study design
Baseline assessments
All participants completed baseline evaluations, which included (1) autonomic function tests and blood volume measurements; (2) neurohumoral regulation during 2-h standing (data to be reported elsewhere); and (3) cardiac magnetic resonance imaging (MRI) assessments. Ten female POTS patients and 11 healthy women who had normal menstrual cycles and were not on or had not been on oral contraceptives for ≥ 6 mo were studied twice; once during the early follicular phase (when both estrogen and progesterone are low) and once during the mid-luteal phase (when both sex hormones are high), with the order counterbalanced. Patients were then randomly assigned to a β-blocker versus placebo drug intervention trial prior to participation in exercise training (22). For the sake of simplicity, the β-blocker trial and the effect of the menstrual cycle data will be reported separately.
Short-term exercise training
Twenty-five patients (24 women, 1 man) participated in an “optimized” exercise training program for 3 mo with 19 completing the program, and these patients were evaluated again after exercise training during the mid-luteal phase of the menstrual cycle.
Due to the complexity of the overall study design, we only report here data obtained from baseline assessments in all participants and after exercise training in POTS patients in this manuscript.
Autonomic function tests
All participants were on an isocaloric constant diet consisting of 200 mEq sodium, 100 mEq potassium, and 1000 mg calcium, while fluid intake was ad libitum 2 days prior to testing. Women were tested during the mid-luteal phase (i.e., 19 to 22 days after the onset of menstruation) of their menstrual cycles. They took a pregnancy test and showed negative results on testing day.
The experiments were performed in the morning ≥2 h after a light breakfast, and ≥48 h after the last caffeinated or alcoholic beverage in a quiet, environmentally controlled laboratory with an ambient temperature of ~25 °C. An intravenous catheter was inserted into an antecubital vein of the non-dominant arm, and a small recording electrode was placed in the peroneal nerve at the popliteal fossa for obtaining muscle sympathetic nerve activity (MSNA) signals (23). After ≥30 min of quiet rest in the supine position, baseline data were collected for 6 min. After that, a Valsalva maneuver (40 mmHg, 20 s) was performed, followed by a cold pressor test for 2 min, and static handgrip sustained to fatigue at 40% maximal voluntary contraction force (dominant arm) with 2 min of post-exercise circulatory arrest. After a sufficient recovery, the subject was tilted passively to 30° upright for 6 min and 60° upright for 45 min or till presyncope.
HR (ECG), blood pressure (BP, Portapres), MSNA (microneurography) (23), and respiratory rate (nasal cannula) were recorded continuously. Arm BP (SunTech) was measured intermittently for the calculation of steady-state hemodynamics. Cardiac output (acetylene rebreathing) (24) was measured supine, at the end of 30° tilt, and after 5, 10, 20, 30 and 40 min of 60° tilt. Both stroke volume and total peripheral resistance were calculated (1,25). Blood samples were taken in the supine position, and after 5 and 20 min of 60° tilt. The subject was returned to supine for recovery either after completing 45 min of tilting or the development of presyncope. Blood volume was measured by a modified carbon monoxide rebreathing technique (26,27), with a typical error (expressed as coefficient of variation) of 4–5% in our laboratory.
Cardiac MRI
Cardiac MRI was acquired by a 1.5-T Phillips MRI scanner. After completing the standard imaging protocol for the assessment of mass and volume (28), gradient echo, cine long- and short-axis MRI sequences with a temporal resolution of ~42 ms, a repetition time of ~4 ms, an echo time of ~2 ms and a flip angle of 55° were obtained. The heart was sectioned in 6 mm slices with a gap of 4 mm spanning from the apex to the base and the image resolution was 256 × 256 with a 330-mm field of view. One observer who was blinded to the study read the MRI results. Short axis slices were used for left ventricular volume and mass calculations using the MRI-MRI Analytical Software System (MEDIS, Leiden, The Netherlands). The typical error of the intra-operator variability of the manual planning of cardiac MRI in our laboratory was 1.6% for left ventricular mass and 1.3% for left ventricular end-diastolic volume assessments.
Exercise training
A modified Astrand-Saltin incremental treadmill protocol was used to determine each patient’s peak exercise capacity prior to training. Based on the maximal steady-state HR and resting HR, three training zones were determined (i.e., recovery, base pace, and maximal steady-state). To quantify the training stimulus, we used the method of Banister et al (29), for the calculation of the training impulse.
The majority of the training sessions, particularly during the early phases were prescribed as “base training” with target HR equivalent to ~75–85% of maximal. Initially, patients trained 2 to 4 times per wk for 30–45 min/session by using a recumbent bike, rowing, or swimming. The use of only semi-recumbent exercise at the beginning was a critical strategy, allowing patients to exercise while avoiding the upright posture which elicits their symptoms. As the patients became relatively fit, the duration of the base training sessions was prolonged, and subsequently sessions of increased intensity (i.e., maximal steady-state) were added first once and then twice per week, and were always followed by recovery sessions. Upright exercise was added gradually as tolerated, though usually not until the second or third month. By the end of the training, patients were exercising 5–6 h per wk, and they were encouraged to use an upright bike, or walk on the treadmill, or jog. In addition to the endurance training, resistance training using weight lifting was also undertaken. Weight lifting started from once a week, 15–20 min/session, and gradually increased to twice a week, 30–40 min/session. Additionally, patients were encouraged to increase their diary salt intake to 6–8 g per day and water intake of 3–4 liters per day, and elevate the head of the bed during sleeping at night. Patients were encouraged to continue to train at the same level indefinitely after the study so as to maintain their fitness and heart health. They were required to return to our laboratory after 1 year to see how they did on their own without intensive follow up from us.
Patients’ Quality of Life was assessed using the 36-item Short-Form Health Survey (SF-36) (30) before and after training.
Data analysis
Sympathetic bursts were identified by a computer program (31) and were then confirmed by a microneurographer. The integrated neurogram was normalized by assigning a value of 100 to the largest amplitude of a burst during baseline. All bursts for that trial were then normalized against that value (32). Burst areas of the integrated neurogram, and systolic and diastolic pressures were measured simultaneously on a beat-to-beat basis. Total activity of the burst was defined as the burst area of the rectified and integrated neurogram. The number of bursts per minute (burst frequency), the number of bursts per 100 heart beats (burst incidence), and total activity were used as quantitative indexes.
Sympathetic baroreflex sensitivity was assessed by relating all sympathetic bursts occurring during the 20 s straining period of the Valsalva maneuver to the maximum fall of diastolic pressure (33), and cardiovagal baroreflex sensitivity was assessed during early phase II and phase IV (34). Data were averaged for 1 min at baseline and during the cold pressor test. Data were averaged for 1 min of the initial handgrip, the last 30 s of handgrip prior to fatigue, the intermediate period after the initial 1 min and before the last 30 s of handgrip, and for each 1 min of post-exercise circulatory arrest. During 30° upright tilt, data were averaged from the 2nd to 5th min. During 60° tilt, data were averaged from the 2nd to 5th min, 7th to 10th min, 17th to 20th min, 26th to 29th min, 36th to 39th min, and 42nd to 45th min. Since some subjects had presyncope during tilting, and the tilt test was terminated at different time points, we used the ‘last stable data carry forward’ method for imputing missing values (35).
Statistical analysis
Data are expressed as median [25%, 75%]. Physical characteristics, baseline variables, and baroreflex sensitivity between groups were compared using non-parametric Mann-Whitney rank sum test. Sympathetic and cardiovascular responses during the autonomic function tests were analyzed using Friedman repeated measures analysis of variance (ANOVA) on ranks, and the Student-Newman-Keuls Method was used post hoc for multiple comparisons within groups. Two way repeated measures ANOVA was used to verify whether responses to various interventions during autonomic function tests were different between groups, and the interaction in the model was used for comparisons of “group responses”. In the case of a significant difference between groups, the Holm-Sidak method was used post hoc for multiple comparisons. Survival analysis with the log-rank test was used to compare time without presyncope during 45-min 60° tilt between groups. Effects of training on hemodynamic variables, blood volume, cardiac size/mass, and SF-36 scores were analyzed using Wilcoxon signed rank tests. All statistical analyses were performed with a personal computer-based analysis program (SigmaStat, SPSS). A P value of < 0.05 was considered statistically significant.
RESULTS
Physical characteristics
Table 1 depicts physical characteristics of POTS patients and healthy controls. Both supine and 10-min standing HR as well as changes in HR were significantly greater in patients than controls. Approximately 55% of the patients had an increase in HR ≤ 35 bpm, while 45% had greater increases (> 35 bpm), with 7 patients having increases ≥ 40 bpm; the largest increase in HR was 60 bpm, and the highest standing HR was 165 bpm. Blood and plasma volumes were markedly reduced in patients. POTS patients had much smaller left ventricular mass compared with healthy sedentary controls.
Table 1
Subjects’ characteristics
Variables | POTS Patients (26 women, 1 man) | Healthy Controls (15 women, 1 man) |
---|---|---|
Age (yr) | 26 [21, 33] | 28 [23, 35] |
Height (cm) | 163 [161, 172] | 166 [162, 172] |
Weight (kg) | 64 [57, 70] | 63 [56, 68] |
Body Mass Index (kg/m2) | 23 [22, 26] | 22 [21, 24] |
Screening | ||
![]() | 88 [77, 93]†† | 72 [64, 77] |
![]() | 114 [108, 131]†† | 89 [77, 99] |
![]() | 32 [24, 38]†† | 17 [10, 22] |
Supine Hematocrit (%) | 39 [37, 40] | 38 [36, 40] |
Blood Volume (ml/kg) | 60 [54, 64]†† | 71 [65, 78] |
Plasma Volume (ml/kg) | 39 [36, 43]†† | 49 [44, 52] |
Left Ventricular Mass (g/kg) | 1.26 [1.12, 1.37]†† | 1.45 [1.34, 1.57] |
Left Ventricular End-Diastolic Volume (ml/m2) | 60 [52, 65] | 64 [57, 70] |
Values are presented as median [25%, 75%].
Autonomic function
Upright tilt
MSNA increased at 30°, and further increased at 60° tilt in all subjects (both P < 0.01). Total activity was significantly greater (P = 0.04) in patients than controls after 10 min of 60° tilt, while burst frequency followed a similar trend (P = 0.07) (Figure 1). Table 2 shows hemodynamic responses during upright tilt. Systolic BP increased in patients but remained unchanged in controls, while diastolic BP increased in all subjects during tilting; these responses did not differ between groups. Both supine and upright HR was markedly greater in patients than controls. Patients had smaller cardiac output and stroke volume in both supine and upright postures. Total peripheral resistance was greater in patients than controls in the supine position and during tilting. Fourteen of 27 patients (52%) and 6 of 16 controls (38%) developed presyncope during the 45-min 60° tilting, but the time to presyncope was not significantly different between groups (log-rank test, P = 0.31).
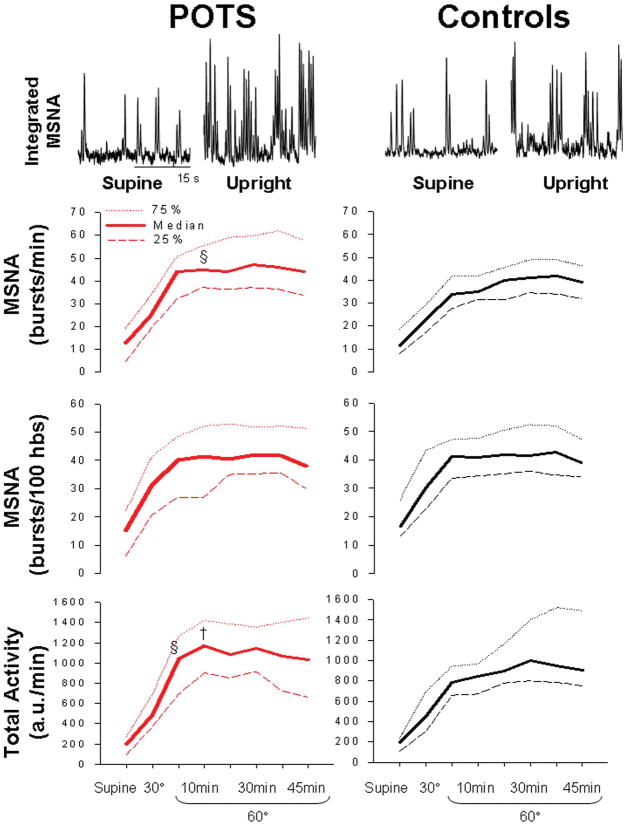
Values are expressed as median [25%, 75%]. §P < 0.10 and †P < 0.05 compared to controls after 5 and 10 min of 60° upright tilt.
Table 2
Hemodynamic responses during graded upright tilt
Variables | POTS Patients (n = 27) | Healthy Controls (n = 16) | ||||||||
---|---|---|---|---|---|---|---|---|---|---|
Supine | 30° Tilt | 60° Tilt | Supine | 30° Tilt | 60° Tilt | |||||
5 min | 20 min | 40 min | 5 min | 20 min | 40 min | |||||
SBP (mmHg) | 105 [98, 114] | 112* [105, 120] | 113* [109, 124] | 112* [105, 118] | 114* [106, 120] | 109 [106, 115] | 116 [105, 123] | 116 [109, 122] | 114 [107, 116] | 112 [109, 115] |
DBP (mmHg) | 66 [60, 69] | 70** [63, 74] | 77** [67, 81] | 76** [68, 83] | 72** [67, 81] | 63 [59, 69] | 69** [66, 75] | 72** [70, 82] | 73** [70, 77] | 75** [71, 77] |
HR (bpm) | 77†† [70, 90] | 84**†† [75, 94] | 104**†† [96, 111] | 107**†† [102, 122] | 110**†† [103, 125] | 68 [61, 76] | 70** [65, 82] | 83** [77, 93] | 87** [82, 99] | 87** [83, 108] |
CO (l/min) | 5.0†† [4.3, 5.4] | 4.4**†† [4.0, 5.0] | 3.6**†† [3.3, 4.4] | 3.5**†† [3.1, 3.8] | 3.2**†† [2.9, 3.8] | 6.8 [5.9, 7.3] | 5.9** [5.0, 6.9] | 5.1** [4.5, 6.1] | 4.4** [4.0, 5.2] | 4.2** [3.9, 4.7] |
SV (ml) | 59†† [50, 66] | 47**†† [38, 56] | 36**†† [28, 42] | 30**†† [25, 37] | 29**†† [23, 35] | 86 [74, 100] | 76** [60, 102] | 60** [45, 76] | 47** [39, 64] | 42** [36, 56] |
TPR (dyn·s·cm−5) | 1284†† [1106, 1501] | 1566**†† [1328, 1735] | 1867**†† [1573, 2314] | 1959**†† [1788, 2336] | 2137**†† [1805, 2493] | 944 [859, 1057] | 1063** [957, 1504] | 1309** [1130, 1623] | 1475** [1346, 1799] | 1635** [1362, 1790] |
Values are expressed as median [25%, 75%]. SBP, systolic blood pressure; DBP, diastolic blood pressure; HR, heart rate; CO, cardiac output; SV, stroke volume; TPR, total peripheral resistance.
Valsalva maneuver
Sympathetic baroreflex sensitivity, as assessed by relating all sympathetic bursts occurring during the 20 s straining period of the Valsalva maneuver to the maximum fall in diastolic pressure, did not differ between patients and controls (−0.75 [−1.05, −0.55] vs −0.83 [−1.21, −0.45] bursts/20 s/mmHg; P = 0.85). Cardiovagal baroreflex sensitivity assessed during early phase II (i.e., a hypotensive stimulus) of the Valsalva maneuver was comparable in patients and controls (4.61 [3.01, 5.76] vs 4.45 [3.42, 6.88] ms/mmHg; P = 0.80). It was not different between groups during phase IV (i.e., a hypertensive stimulus, 10.01 [6.91, 14.05] vs 10.80 [7.48, 13.43] ms/mmHg; P = 0.83). These results are consistent with the findings of Masuki et al (36), suggesting that tachycardia in POTS patients is not due to abnormal baroreflex control of HR.
Cold pressor test and static handgrip
Cardiovascular and sympathetic responses during the cold pressor test did not differ between groups, even though HR was consistently greater in patients (Table 3). These results indicate that the integrity of central vasomotor processes and their efferent pathways was intact in POTS patients. Table 4 depicts responses to handgrip. At the same relative fatiguing force, the peak BP during handgrip did not differ between groups. Even though absolute values of HR were consistently greater in patients than controls, the contraction-induced rises in HR were not different between groups (P = 0.67). Contraction-evoked peak MSNA responses were similar in patients and controls (P = 0.85 for total activity). MSNA during post-exercise circulatory arrest was also similar between groups (P = 0.64). These data suggest that modulation of the sympathetic nerve system by the muscle metaboreflex, mechanoreflex, and central command was intact in POTS patients.
Table 3
Cardiovascular and sympathetic responses during the cold pressor test
Variables | POTS Patients (n = 27) | Healthy Controls (n = 16) | ||||||
---|---|---|---|---|---|---|---|---|
Baseline | CPT-1min | CPT-2min | Recovery | Baseline | CPT-1min | CPT-2min | Recovery | |
SBP (mmHg) | 110 [99, 118] | 120** [113, 130] | 127** [119, 137] | 112 [101, 117] | 114 [110, 121] | 126** [121, 133] | 130** [115, 138] | 114 [110, 120] |
DBP (mmHg) | 66 [63, 70] | 80** [71, 88] | 78** [73, 85] | 66 [63, 68] | 68 [65, 72] | 82** [72, 86] | 77** [71, 83] | 69 [65, 73] |
HR (bpm) | 79† [69, 87] | 82*† [72, 97] | 82† [70, 93] | 72† [66, 86] | 67 [62, 72] | 79* [69, 87] | 71 [63, 79] | 64* [58, 70] |
MSNA-BF (bursts/min) | 9 [4, 15] | 22** [16, 47] | 30** [16, 36] | 16* [6, 18] | 7 [4, 13] | 29** [15, 36] | 24** [9, 38] | 13* [6, 17] |
MSNA-BI (Bursts/100 heart beats) | 9 [6, 18] | 30** [15, 55] | 38** [16, 44] | 19** [7, 27] | 11 [6, 19] | 32** [20, 50] | 35** [14, 47] | 18* [11, 26] |
Total Activity (a.u./min) | 170 [99, 265] | 560** [336, 1098] | 612** [324, 876] | 265 [121, 346] | 123 [65, 211] | 725** [216, 935] | 533** [255, 808] | 225* [169, 325] |
Values are expressed as median [25%, 75%]. CPT, the cold pressor test; SBP and DBP, systolic and diastolic blood pressure; HR, heart rate; MSNA-BF, muscle sympathetic nerve activity burst frequency; MSNA-BI, muscle sympathetic nerve activity burst incidence; a.u. arbitrary unit.
Table 4
Cardiovascular and sympathetic responses during static handgrip to fatigue
Variables | POTS Patients (n = 27) | Healthy Controls (n = 16) | ||||||
---|---|---|---|---|---|---|---|---|
Baseline | HG-fatigue | PECA | Recovery | Baseline | HG-fatigue | PECA | Recovery | |
SBP (mmHg) | 117 [111, 132] | 145** [133, 154] | 137** [128, 148] | 117 [109, 130] | 129 [125, 138] | 158** [131, 170] | 148** [130, 159] | 131 [121, 137] |
DBP (mmHg) | 69 [60, 73] | 91** [78, 98] | 80** [68, 88] | 67* [57, 73] | 68 [63, 71] | 85** [73, 93] | 75** [73, 83] | 66* [61, 70] |
HR (bpm) | 75† [65, 84] | 94**† [81, 107] | 79† [67, 89] | 76 [64, 85] | 65 [61, 72] | 79** [73, 92] | 65 [60, 73] | 64 [61, 74] |
MSNA-BF (bursts/min) | 9 [5, 19] | 28** [24, 38] | 23** [16, 31] | 12* [7, 21] | 10 [5, 24] | 28** [20, 39] | 23** [16, 28] | 10 [8, 20] |
MSNA-BI (Bursts/100 heart beats) | 13 [6, 25] | 32** [25, 42] | 31** [19, 43] | 19** [9, 28] | 15 [7, 31] | 37** [25, 51] | 35** [22, 44] | 14 [12, 28] |
Total Activity (a.u./min) | 192 [107, 313] | 774** [593, 974] | 471** [372, 627] | 274* [175, 366] | 172 [102, 303] | 814** [476, 1121] | 542** [368, 692] | 173* [157, 360] |
Values are expressed as median [25%, 75%]. HG-fatigue, static handgrip at fatigue; PECA, post-exercise circulatory arrest; SBP and DBP, systolic and diastolic blood pressure; HR, heart rate; MSNA-BF, muscle sympathetic nerve activity burst frequency; MSNA-BI, muscle sympathetic nerve activity burst incidence; a.u. arbitrary unit.
Exercise training effects
Twenty-five patients initiated the exercise training program including all of the sickest and most debilitated patients, with 19 completing the 3-mo training. The dropout rate was 24% in this study. The reasons for these dropouts were that (1) two patients were too busy with work and no longer had time to exercise; (2) two patients got injured – one injured her ankle falling down the stairs, and the other one was in a car accident and injured her legs; (3) one patient was diagnosed with seizures in addition to POTS; and (4) one patient had an arrhythmia, which was not related to training. HR was similar between those who dropped out and who completed the program in the supine position (80 [72, 90] vs 85 [72, 90] bpm; P = 0.98) and after 10 min of standing (114 [108, 126] vs 120 [103, 122] bpm; P = 0.85), suggesting that the severity of POTS was not the cause for the dropout.
Exercise training increased peak oxygen uptake (26.8 [24.1, 29.0] pre vs 28.9 [26.7, 32.7] ml/kg/min post-training; P < 0.01), blood volume (Figure 2), and plasma volume (39 [34, 42] vs 41 [37, 43] ml/kg; P < 0.01) in these patients. Left ventricular mass also increased significantly after training in POTS patients, and was no longer different from controls (Figure 2). Both supine and 10-min standing HR decreased markedly after short-term exercise training (Figure 3). Ten out of 19 patients no longer met POTS criteria after training, even though as a group standing HR was still greater in these patients compared to controls (Figure 3). Patients’ Quality of Life was improved significantly after training in all patients (Figure 4).
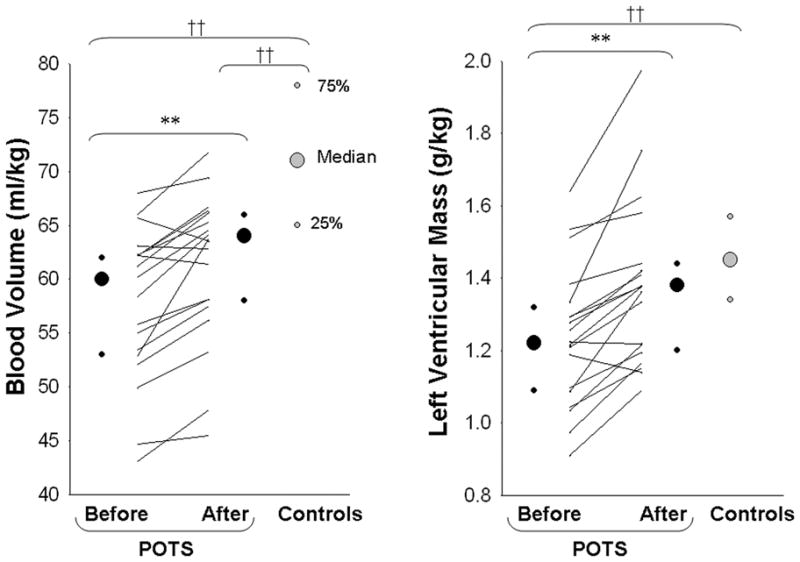
Values are expressed as individuals and median [25%, 75%]. **P < 0.01 compared to before training in POTS. ††P < 0.01 compared to controls.
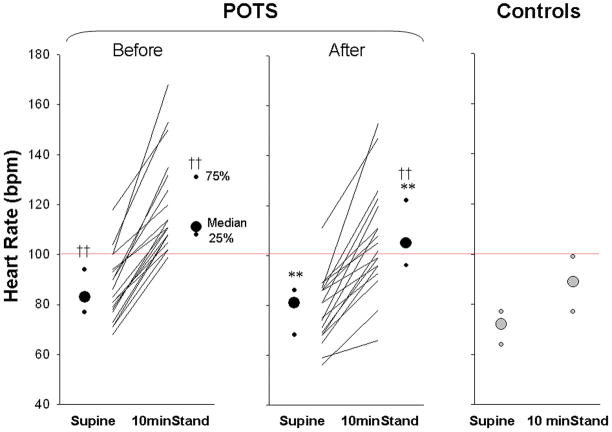
Values are expressed as individuals and median [25%, 75%]. **P < 0.01 compared to before training in POTS in the same posture. ††P < 0.01 compared to controls in the same posture.
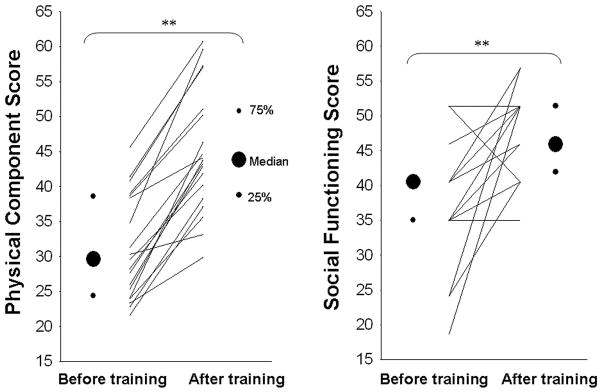
Values are expressed as individuals and median [25%, 75%]. **P < 0.01 compared to before training in POTS.
Exercise training lowered HR significantly during upright tilt in all patients (P = 0.02). Based on the median value of MSNA burst frequency for healthy controls during acute upright tilt (i.e., 5th min of 60° tilt), we separated patients into two subgroups before training, namely, hyperadrenergic group (i.e., MSNA ≥ 45 bursts/min) and normadrenergic group (i.e., MSNA < 45 bursts/min). Upright MSNA decreased in the hyperadrenergic group (n = 9; 5th min of 60° tilt, 51 [50, 60] pre- vs 41 [38, 47] bursts/min post-training, P = 0.02), but not in the normadrenergic group (n = 10; 36 [24, 42] vs 35 [31, 44] bursts/min, P = 0.57) after training. Exercise training did not alter BP responses during tilting. Both cardiovagal (P = 0.55 and 0.89 during early phase II and phase IV) and sympathetic (P = 0.17) baroreflex sensitivity remained unchanged after training. Even though the absolute values of HR were markedly lowered by exercise training, the responses of MSNA and BP during the cold pressor test and static handgrip did not change.
DISCUSSION
The major findings from this study are that (1) cardiac size and mass, and blood volume were much smaller in POTS patients compared with healthy sedentary controls; (2) HR was greater, while stroke volume was smaller in patients than controls during upright posture; (3) the function of the autonomic nervous system was intact in POTS patients; and (4) exercise training increased cardiac size and mass, expanded blood volume, and thus improved or even cured POTS syndrome. These results suggest that POTS per se is indeed a consequence of “deconditioning” (i.e., cardiac atrophy and hypovolemia), and carefully prescribed exercise training can be used as a non-drug treatment for patients with POTS.
POTS and “deconditioning”
POTS is characterized by orthostatic tachycardia without significant hypotension (37). It has been demonstrated that the excessive tachycardia in POTS patients is not caused by anxiety (38). Patients with POTS often experience palpitations, light-headedness, dizziness, nausea, fatigue, exercise intolerance, or near syncope. It has been the focus of increasing clinical interest over the last 15 years (9,12,39). However, the pathophysiology of this disorder remains unclear. It is proposed that POTS is heterogeneous in presentation and mechanisms (37). Previous investigations have shown that POTS patients have altered baroreflex function (40,41), hyperadrenergic activity (42), postganglionic sympathetic denervation and inadequate peripheral vasoconstriction (43), cardiac sympathetic dysautonomia (44), norepinephrine-transporter deficiency (45), decreased blood volume (39), abnormal activation of the renin-angiotensin system (42), mast cell activation (46), muscle pump defects (47), or autoimmune autonomic neuropathy (48).
We assessed comprehensively the function of the autonomic nervous system in all patients, but did not find any evidence of abnormalities. However, blood and plasma volume was about 20% lower, while left ventricular mass was approximately 16% smaller in these patients compared with healthy sedentary controls. Similar observations can almost always be made after a period of microgravity exposure (e.g., bed rest or spaceflight) when “deconditioning” occurs (49,50). Previous work from our laboratory has demonstrated that a smaller and less “distensible” heart in young women or in healthy individuals after bed rest deconditioning can result in a larger reduction in stroke volume during orthostasis as a function of the Frank-Starling mechanism, leading to an excessive increase in HR (50). Consistent with our previous preliminary observations reported in abstract format (51), upright stroke volume was markedly smaller in POTS patients than healthy controls in this study. The orthostatic tachycardia observed in these patients thus appeared to be a physiological compensatory response to the smaller stroke volume (51,52), which was attributable to cardiac atrophy and reduced blood volume. POTS may occur alone (primary), or because of another medical condition (secondary) (53). However, almost all the patients have a history of physical inactivity (i.e., deconditioning), which subsequently elicits or exacerbates POTS symptoms.
“The Grinch syndrome” – a new name for POTS
Results from our laboratory have shown that there is a sex-specific difference in cardiac size and mass even in healthy humans, and women have a smaller (and therefore, less “distensible) heart compared with men (2,54). It is possible that such a sex difference is exaggerated in POTS patients. This notion is supported by a recent study of Miwa and Fujita (8), showing that a considerable number of chronic fatigue syndrome patients had a small heart, as assessed by roentgenography and echocardiography. POTS is a frequent finding in patients with chronic fatigue syndrome (55). It is highly likely that the small heart contributes to the development of POTS, and should probably be included in the genesis of this syndrome.
By using a cardiac MRI technique, we assessed precisely the heart size and mass in patients with POTS, and found that the heart was about 16% smaller in these patients and more than two standard deviations smaller than the true mean for healthy sedentary controls. In the famous children’s book “How the Grinch Stole Christmas” by Dr. Seuss, subsequently popularized by the movie of the same name, the main character had a heart that was “two sizes too small.” We suggest then, that a more pathophysiological name for POTS would be: “The Grinch Syndrome”, emphasizing that a small heart is the primary abnormality and target for therapy. A small heart coupled with reduced blood volume contributes to the small stroke volume, ultimately resulting in reflex tachycardia during orthostasis in these patients.
Exercise training for POTS
Treating POTS is difficult, since there are no effective pharmacological therapies for POTS patients so far. Many patients have disabling side effects with “standard” drug treatments. For example, administration of β-blockers can reduce tachycardia; but, if the excessive reduction in stroke volume during orthostasis cannot be corrected, orthostatic hypotension and severe fatigue often occurs in these patients. Administration of volume expanders increases body fluids and vascular volume, which can result in a smaller reduction in stroke volume during orthostasis in POTS patients. However, leg edema may appear and hypokalemia may develop in some patients. Administration of α1-adrenergic agonists can also decrease heart rate through the baroreflex mechanism. However, some patients may develop hypertension or other intolerable side effects, which is quite common in these patients with prominent hypervigilance (37).
To avoid the side effects with drug therapies, we applied a non-drug treatment, namely, exercise training, to patients with POTS. It is important to emphasize that even the sickest patients participated in this program and that training was facilitated by avoiding upright exercise in the early stage. Endurance training has been shown to expand blood and plasma volumes (16), increase cardiac size and mass (49), prevent cardiac atrophy and increase orthostatic tolerance in healthy women after prolonged period of bed rest (49) as well as in patients with unexplained syncope or orthostatic hypotension. We found that 3 months of progressive exercise training in POTS patients increased maximal oxygen uptake by 11%, indicating an increase in physical fitness. Left ventricular mass and end-diastolic volume increased by 12 and 8% after training, resulting in significant “cardiac remodeling”. The heart became much “bigger” and probably more distensible after exercise training. Blood and plasma volumes also increased markedly after training. Ten out of 19 patients (53%) no longer met criteria for POTS after completion of the 3-month exercise training program and thus were “cured”. More importantly, patients’ Quality of Life, as assessed by SF-36, was improved significantly after short-term exercise training in virtually all these patients, including those with persistence of orthostatic tachycardia. We speculate that more prolonged and/or intense training may be necessary to further reduce upright tachycardia in those patients.
Limitations
There are at least three limitations in this study. First, it may be difficult to attribute the improvements in POTS symptoms after exercise training only to the training program because patients were “encouraged” to increase their diary salt and water intake, and elevate the head of the bed during sleeping at night. The latter two can also expand blood volume and increase orthostatic tolerance. However, these “encouragements” are often given by clinicians to their patients, while the therapeutic effects seem to be limited in most patients. Second, the size of the control group was relatively small, and the control-to-case ratio was 16:27, namely, 1:1.7. However, adding more healthy subjects would not likely change our results and conclusions, because the power for detecting the difference between POTS patients and healthy controls was ≥ 0.80 for all major outcome variables in the present study. Third, six patients exited the 3-mo training program, and therefore, our retention and treatment completion rate was 76%. Retention in the exercise training program is one of the important measures of treatment effectiveness. Previous and recent studies have shown that the adherence to either exercise training (56), or antihypertensive drug treatment (57), or cardiac rehabilitation (58) ranges from ~35% to ~68%. Our retention rate is actually higher than the findings of these studies, suggesting that compliance with this approach is within the range of other drug or non-drug interventions in common diseases.
In summary, patients with POTS had a smaller heart coupled with reduced blood volume compared with healthy controls. We therefore propose the name “The Grinch Syndrome” to focus on this pathophysiology. The marked orthostatic tachycardia in these patients appeared to be a physiological compensatory response to a smaller stroke volume, which was attributable to cardiac atrophy and hypovolemia. The function of the autonomic nervous system was intact in POTS patients. Short-term exercise training increased cardiac size/mass, expanded blood/plasma volume, and thus improved or even cured POTS. These results suggest that POTS per se is indeed a consequence of “deconditioning”, and carefully prescribed exercise training can be used as an effective non-drug therapy for POTS patients.
Acknowledgments
The time and effort put forth by the subjects is greatly appreciated. The authors thank Robin P. Shook, Kazunobu Okazaki, Nainesh Gandhi, Beverley A. Huet, M. Dean Palmer, Daniel L. Creson, Colin L. Conner, Diane Bedenkop, Peggy Fowler, Adam Banks, Murugappan Ramanathan, Cyrus Oufi, and Dak Quarles for their valuable laboratory assistance. We also gratefully acknowledge Dr. Gordon H. Williams from Brigham & Women’s Hospital, Harvard Medical School in Boston for invaluable advice and support.
Funding sources: The National Institutes of Health K23 grant (HL075283), National Space Biomedical Research Institute grant (CA00701), and the Clinical and Translational Research Center (formerly, the General Clinical Research Center) grant (RR00633).
Abbreviations and Acronyms
- POTS
- Postural Orthostatic Tachycardia Syndrome
- HR
- heart rate
- ECG
- electrocardiogram
- BP
- blood pressure
- MSNA
- muscle sympathetic nerve activity
- MRI
- magnetic resonance imaging
- SF-36
- the 36-item Short-Form Health Survey
Footnotes
Publisher's Disclaimer: This is a PDF file of an unedited manuscript that has been accepted for publication. As a service to our customers we are providing this early version of the manuscript. The manuscript will undergo copyediting, typesetting, and review of the resulting proof before it is published in its final citable form. Please note that during the production process errors may be discovered which could affect the content, and all legal disclaimers that apply to the journal pertain.
References
Full text links
Read article at publisher's site: https://doi.org/10.1016/j.jacc.2010.02.043
Free after 12 months at content.onlinejacc.org
http://content.onlinejacc.org/cgi/content/full/55/25/2858
Free to read at content.onlinejacc.org
http://content.onlinejacc.org/cgi/content/abstract/55/25/2858
Free after 12 months at content.onlinejacc.org
http://content.onlinejacc.org/cgi/reprint/55/25/2858.pdf
Citations & impact
Impact metrics
Article citations
Management of Postural Orthostatic Tachycardia Syndrome in Pediatric Patients: A Clinical Review.
J Pediatr Pharmacol Ther, 29(5):456-467, 14 Oct 2024
Cited by: 0 articles | PMID: 39411411 | PMCID: PMC11472415
Individually tailored exercise in patients with postural orthostatic tachycardia syndrome related to post-COVID-19 condition - a feasibility study.
Sci Rep, 14(1):20017, 28 Aug 2024
Cited by: 0 articles | PMID: 39198662 | PMCID: PMC11358431
Hyperadrenergic Postural Tachycardia Syndrome: Clinical Biomarkers and Response to Guanfacine.
Hypertension, 81(11):2237-2247, 07 Aug 2024
Cited by: 0 articles | PMID: 39109428
Reduced Stroke Volume and Brain Perfusion Drive Postural Hyperventilation in Postural Orthostatic Tachycardia Syndrome.
JACC Basic Transl Sci, 9(8):939-953, 26 Jun 2024
Cited by: 0 articles | PMID: 39297140 | PMCID: PMC11405806
Suspected Diphtheria Toxoid and Tetanus Toxoid (dTdap) Booster Vaccine-Induced Postural Orthostatic Tachycardia Syndrome (POTS).
Cureus, 16(7):e64008, 07 Jul 2024
Cited by: 0 articles | PMID: 39109119 | PMCID: PMC11301768
Go to all (154) article citations
Similar Articles
To arrive at the top five similar articles we use a word-weighted algorithm to compare words from the Title and Abstract of each citation.
Postural Hyperventilation as a Cause of Postural Tachycardia Syndrome: Increased Systemic Vascular Resistance and Decreased Cardiac Output When Upright in All Postural Tachycardia Syndrome Variants.
J Am Heart Assoc, 7(13):e008854, 30 Jun 2018
Cited by: 21 articles | PMID: 29960989 | PMCID: PMC6064900
The international POTS registry: Evaluating the efficacy of an exercise training intervention in a community setting.
Heart Rhythm, 13(4):943-950, 09 Dec 2015
Cited by: 47 articles | PMID: 26690066
Effects of exercise training on arterial-cardiac baroreflex function in POTS.
Clin Auton Res, 21(2):73-80, 20 Nov 2010
Cited by: 23 articles | PMID: 21103906
Exercise in the postural orthostatic tachycardia syndrome.
Auton Neurosci, 188:86-89, 21 Nov 2014
Cited by: 25 articles | PMID: 25487551 | PMCID: PMC4336603
Review Free full text in Europe PMC
Funding
Funders who supported this work.
Clinical and Translational Research Center(formerly the General Clinical Research Center (1)
Grant ID: RR00633
NCI NIH HHS (1)
Grant ID: CA00701
NCRR NIH HHS (5)
Grant ID: M01 RR000633-347641
Grant ID: RR00633
Grant ID: M01 RR000633-335189
Grant ID: M01 RR000633-358240
Grant ID: M01 RR000633
NHLBI NIH HHS (7)
Grant ID: HL075283
Grant ID: K23 HL075283
Grant ID: K23 HL075283-01
Grant ID: K23 HL075283-02
Grant ID: K23 HL075283-04
Grant ID: K23 HL075283-05
Grant ID: K23 HL075283-03
National Institutes of Health (1)
Grant ID: HL075283
National Space Biomedical Research Institute (1)
Grant ID: CA00701