Abstract
Rationale
Thrombospondin (TSP)-4 is an extracellular protein that has been linked to several cardiovascular pathologies. However, a role for TSP-4 in vascular wall biology remains unknown.Objective
We have examined the effects of TSP-4 gene (Thbs4) knockout on the development of atherosclerotic lesions in ApoE(-/-) mice.Methods and results
Deficiency in TSP-4 reduced atherosclerotic lesions: at 20 weeks of age, the size of the aortic root lesions in Thbs4(-/-)/ApoE(-/-) mice was decreased by 48% in females and by 39% in males on chow diets; in mice on Western diets, lesions in the descending aorta were reduced by 30% in females and 33% in males. In ApoE(-/-) mice, TSP-4 was abundant in vessel areas prone to lesion development and in the matrix of the lesions themselves. TSP-4 deficiency reduced the number of macrophages in lesions in all groups by ≥ 2-fold. In addition, TSP-4 deficiency reduced endothelial cell activation (expression of surface adhesion molecules) and other markers of inflammation in the vascular wall (decreased production of monocyte chemoattractant protein-1 and activation of p38). In vitro, both the adhesion and migration of wild-type macrophages increased in the presence of purified recombinant TSP-4 in a dose-dependent manner (up to 7- and 4.7-fold, respectively). These responses led to p38-MAPkinase activation and were dependent on β(2) and β(3) integrins, which recognize TSP-4 as a ligand.Conclusions
TSP-4 is abundant in atherosclerotic lesions and in areas prone to development of lesions and may influence the recruitment of macrophages by activating endothelial cells and directly interacting with macrophages to increase their adhesion and migration. Our observations suggest an important role for this matricellular protein in the local regulation of inflammation associated with atherogenesis.Free full text

Thrombospondin-4 regulates vascular inflammation and atherogenesis
Abstract
Rationale
Thrombospondin-4 (TSP-4) is an extracellular protein that has been linked to several cardiovascular pathologies. However, a role for TSP-4 in vascular wall biology remains unknown.
Objective
We have examined the effects of TSP-4 gene (Thbs4) knockout on the development of atherosclerotic lesions in ApoE−/− mice.
Methods and Results
Deficiency in TSP-4 reduced atherosclerotic lesions: at 20 weeks of age, the size of the aortic root lesions in Thbs4−/−/ApoE−/− mice was decreased by 48% in females and by 39% in males on chow diets; in mice on Western diets, lesions in the descending aorta were reduced by 30% in females and 33% in males. In ApoE−/− mice, TSP-4 was abundant in vessel areas prone to lesion development and in the matrix of the lesions themselves. TSP-4 deficiency reduced the number of macrophages in lesions in all groups by ≥ 2 fold. In addition, TSP-4 deficiency reduced endothelial cell activation (expression of surface adhesion molecules) and other markers of inflammation in the vascular wall (decreased production of MCP-1 and activation of p38). In vitro, both the adhesion and migration of wild-type macrophages increased in the presence of purified recombinant TSP-4 in a dose-dependent manner (up to 7 and 4.7 fold, respectively). These responses led to p38-MAPkinase activation and were dependent on β2 and β3 integrins, which recognize TSP-4 as a ligand.
Conclusions
TSP-4 is abundant in atherosclerotic lesions and in areas prone to development of lesions and may influence the recruitment of macrophages by activating endothelial cells and directly interacting with macrophages to increase their adhesion and migration. Our observations suggest an important role for this matricellular protein in the local regulation of inflammation associated with atherogenesis.
INTRODUCTION
Thrombospondin-4 (TSP-4) is one of five members of the thrombospondin (TSP) family of secreted matrix proteins (TSP-1 through TSP-5)1, 2. Within this family, three homologous TSPs, TSP-3, TSP-4 and TSP-5, form a subfamily (subgroup B) based on their shared secondary and tertiary structure3, 4. While the functions of the anti-angiogenic TSP-1 and TSP-2 (subgroup A) in the vascular wall have been studied extensively4–6, the role of TSPs in subgroup B in vascular physiology and pathology remains unknown. In subgroup B, evidence to suggest a role of TSP-4 in vascular pathology is particularly strong. TSP-4 is expressed by endothelial (EC) and smooth muscle cells (SMC) of large blood vessels and is expressed abundantly in capillaries7. Cell biology experiments in vitro suggest that TSP-4 promotes adhesion and migration of neutrophils8, and proliferation of smooth muscle cells7. In vivo, expression of TSP-4 increases dramatically in response to pressure overload9, in failing hearts and heart hypertrophy10, 11, and in response to ischemia12. Furthermore, multiple reports of various populations have documented a genetic association between TSP-4 and accelerated atherogenesis13–18. The effect of TSP-1 deficiency on the development of atherosclerotic lesions in a mouse model has been recently reported19, namely promoting development of atherosclerotic lesions at the initial stages, but exerting a beneficial effect in the well-developed lesions by promoting the phagocytic activity of macrophages.
In view of the reported effects of TSPs in the vascular wall, the genetic information linking TSP-4 to atherogenesis, and yet the lack of information on the functions of TSP-4, we have investigated the effect of TSP-4 deficiency on the development of atherosclerotic lesions in ApoE−/− mice. We report that TSP-4 is expressed in atherosclerotic lesions, and the size of the lesions and the inflammation in vascular wall is reduced in Thbs4−/−/ApoE−/− mice. Our data further suggest that the ability of TSP-4 to support macrophage adhesion, migration and pro-inflammatory signaling is an important contributor to the mechanism of its vascular effects. In addition to the direct interaction with monocytes/macrophages, TSP-4 is found to affect EC activation, as evidenced by their production of surface adhesion molecules and chemoattractants. These effects of TSP-4 on macrophage recruitment in atherosclerotic lesions suggest that TSP-4, which is abundant in the lesions in areas prone to lesion development, and in the subendothelial matrix of capillaries7, is a local regulator of inflammation.
MATERIALS AND METHODS
The detailed descriptions of methods are provided in Online Supplemental Material.
Thbs4−/− mice
The Thbs4 gene was inactivated by replacing 14 nucleotides in the first intron of its genomic sequence with a LacZ-neomycin resistance cassette (Lac0-SA-IRES-lacZNeo555G/Kan). The mouse was created at Deltagen, Inc. (San Mateo, CA), and the resulting mice were genotyped and maintained at the Cleveland Clinic. Thbs4−/− mice were backcrossed into a C57BL/6 background for 12 generations. The absence of TSP-4 mRNA and protein was confirmed by Northern and Western blots (Online Fig.I) and the absence of immunostaining of various tissues from Thbs4−/− mice with anti-TSP-4 antibody (lack of staining in aortic lesions) is shown in Online Fig.Vb.
ApoE−/− and Thbs4−/−/ApoE−/− mice were kept on a Western diet (42% calories from fat) or regular mouse chow diet, starting at 4 weeks of age, and they were sacrificed at 20 weeks of age. All animal procedures were performed according to NIH guidelines under protocols approved by the Institutional Animal Care and Use Committee of the Cleveland Clinic.
Antibodies are described in the Online Supplemental Material.
An MCP-1 ELISA kit was purchased from R&D (Minneapolis, MN) and used according to the manufacturer's directions. Aortic tissue extracts were prepared from isolated aortic fragments, obtained from the aortic arch and descending aorta, down to the iliac bifurcation.
Quantitative analysis of lesions in aortic root was performed as described by Baglione and Smith20.
Quantitative Atherosclerosis Measurements in aortic arch and descending aorta
At 20 weeks of age, the circulatory system of anesthetized mice was perfused with 0.9% NaCl by cardiac intraventricular canalization. Surface lesion area was determined by an en face method. Oil Red O-stained areas of lesions were quantified using ImagePro 6.3.
Immunohistochemistry
Hearts and aortas were harvested and placed in Tissue-Tek O.C.T. Compound (Sakura Finetek U.S.A Inc., Torrance, CA), frozen in liquid nitrogen and stored at −80°C until processed. Frozen sections (10 –12 μm) were incubated with primary antibodies for 2 h at 4°C, followed by incubating sections in Rhodamine Red-X (1:200)- or FITC (1:100)-conjugated secondary antibodies for 45 min at 4°C.
Confocal Microscopy
Z-stacks of images were collected using a Leica TCS-SP3-AOBS Laser Scanning Confocal Microscope (Leica Microsystems Inc., Bannockburn, IL) with an HCX Plan Apo 40×/1.25 NA oil immersion objective. Image stacks from the z-series were reconstructed and analyzed using Volocity 4.1.0 software (Improvision Inc., Lexington, MA).
Quantification of stained area of lesions was performed using Adobe Photoshop CS2 and ImagePro6.3. At least 3 animals per group were used, and 4 or more sections of aortic root per animal were examined.
Plasma Cholesterol Analysis
LDL/VLDL cholesterol was measured in mouse plasma using an HDL &LDL/VLDL Cholesterol Quantification Kit (BioVision Research Products).
Induction of peritonitis and isolation of peritoneal macrophages
Murine peritoneal macrophages were collected utilizing a thioglycollate inflammation model. Sterile 4% Brewer thioglycollate medium solution was injected intraperitoneally and after 72 hours, when macrophage recruitment is maximal in this model21, mice were sacrificed and macrophages were harvested by lavage of the peritoneal cavity with sterile PBS.
Migration and adhesion assays
Cell adhesion, migration and MAPK phosphorylation were measured as described previously7 using the RAW 264.7 macrophage cell line or thioglycollate-elicited peritoneal macrophages derived from wild-type C57BL/6 mice, as described elsewhere21. In inhibition experiments, cells were pretreated for 20 min at 37°C prior to addition to the assays of the following blocking antibodies: rabbit anti-integrin β3, rat anti-α5β1, anti-α4 (Chemicon) and anti-αM (clone M1/70, ATCC), mouse anti-CD36 (Chemicon) and control anti-MHC-1 (W6/32, ATCC).
Foam Cell Formation was measured as described22.
Microvascular endothelial cell isolation and culture
Microvascular Endothelial Cells from murine lung were isolated as described23. To assess the expression of E-selectin (CD62E), ICAM (CD54), and VCAM (CD106), cells were stimulated with 100ng/ml LPS (Sigma-Aldrich, St. Louis, MO) in the same medium for 4h (for CD62E), and 17h (for CD10 6and CD54).
Aortic endothelial cell isolation and culture
Endothelial cells from murine aorta were isolated as described23.
FACS analysis
Endothelial cells were trypsinized. Cells were harvested into DMEM/F12 medium and washed. 0.6 – 0.8×106 cells were incubated with FITC-, PE-, biotin-conjugated antibodies for one hour at 4°C, washed and analyzed with a FACS Calibur (Becton Dickinson, San Jose, CA) using CellQuest Pro software (BD Biosciences, San Jose, CA). Isotype-matched control antibodies were used as negative controls.
Statistical analysis
All data are presented as means ± SE or SD as indicated. Shapiro-Wilk test was used to evaluate normality of distribution in each data group. With the exception of data from females in Fig.1a and b and Fig. 2a, all data showed a normal distribution. Unpaired Student's t-test was used to compare the means between two independent groups with a single variable and normal distribution of data. The Mann-Whitney-Wilcoxon test was used to compare groups that were not normaly distibuted. Two-way ANOVA was used to compare groups with more than one variable. The significance level (p) was set at <0.05.
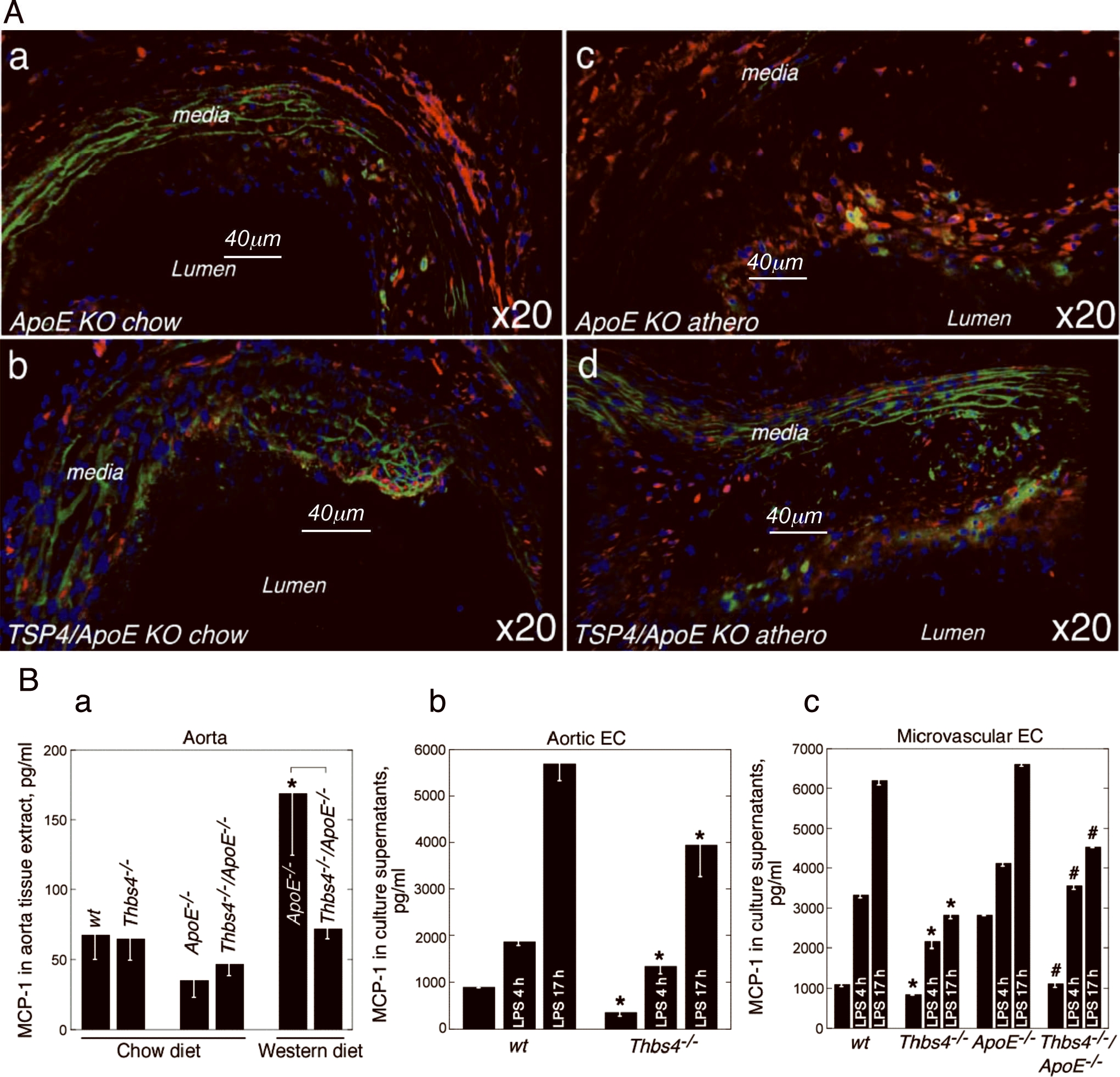
The Oil-Red O stained area in aortic root and aorta was quantified as described in Methods: , n ≥ 11. *Statistically significant changes in the area of lesions (p<0.05) a – lesions in aortic root of mice on the regular chow, μm2; b – lesions in aortic arch and descending aorta of mice on the Western diet, % of the total area; c – cholesterol in VLDL/LDL fraction of blood plasma, mg/dL. Mean ± SEM, n ≥ 11, *statistically significant changes in the area of lesions (p<0.05).
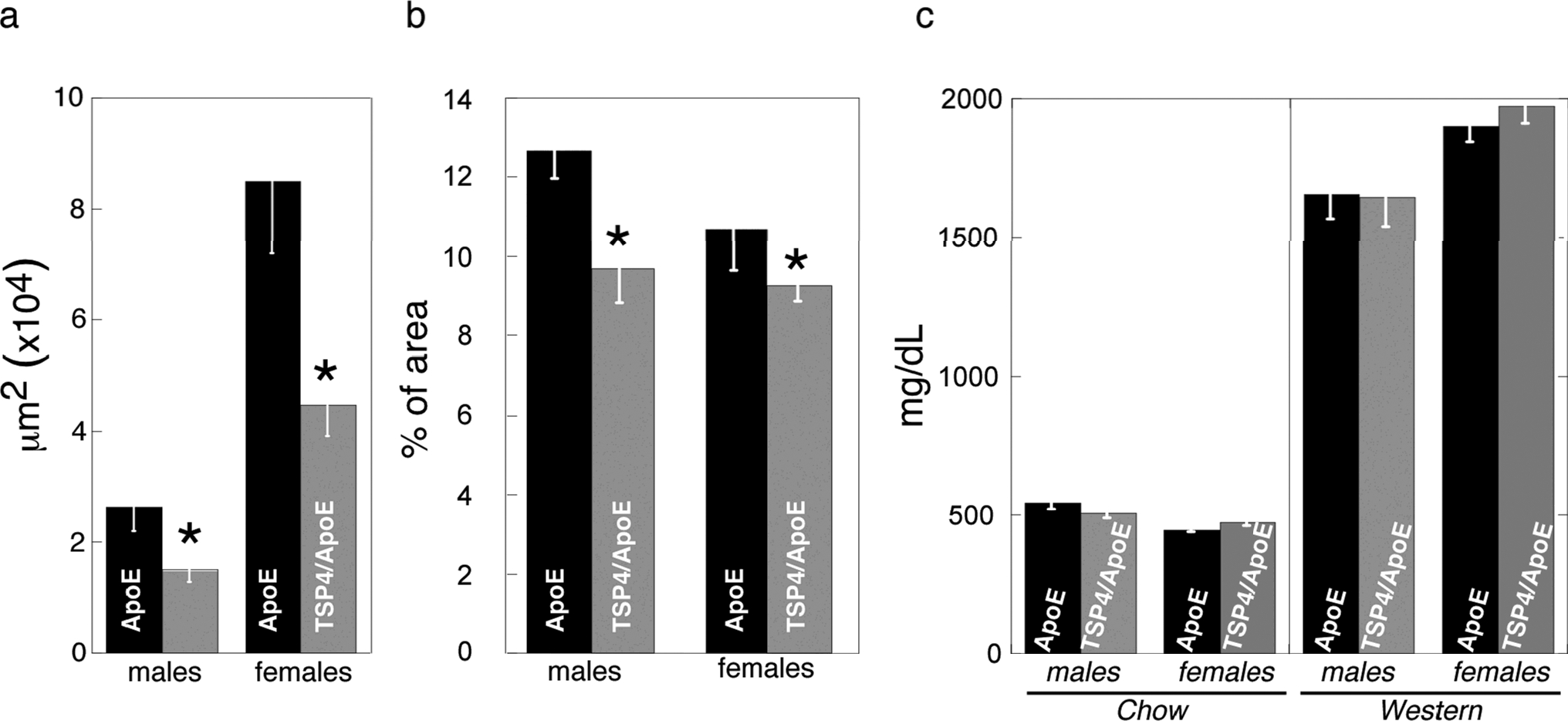
A: reduced cellularity of Thbs4−/−/ApoE−/− lesions. Areas of lesions occupied by nuclei were quantified in aortic root of all animal groups, mean ± SEM. B,C: Immunohistochemical staining or the aortic root sections with MOMA-2 antibody (brown color) was done as described in Methods, and the area of the specific staining was quantified as a percent of the lesion area (pixels). For each value, the quantification of at least 4 sections from at least 3 20-week-old mice was performed. B: percent of stained lesion area, pxl, mean ± SEM, *statistically significant changes (p<0.05). C: a – chow diet, ApoE−/−, male; b - chow diet, Thbs4−/−/ApoE−/−, male; c – 16 week of Western diet, ApoE−/−, male; d – 16 weeks of Western, Thbs4−/−/ApoE−/−, male. DKO = Thbs4−/−/ApoE−/− double KO mice.
RESULTS
Thbs4−/− mice
Thbs4−/− mice appeared normal at birth and displayed no obvious phenotype during their early and adult development. Their lifespan was similar to that of wild type mice. Since β-galactosidase expression was detected in the ovary, seminal vesicles, prostate and uterus of Thbs4−/− mice (http://www.informatics.jax.org/external/ko/deltagen/2006.html), the breeding status of these mice was evaluated. The duration and frequency of pregnancy were similar in WT and Thbs4−/− mice and changed similarly with age. The fertility of males and females was also the same as assessed by mating Thbs4−/− males with WT females and WT males with Thbs4−/− females (data not shown). There was no difference in weight between Thbs4−/−/ ApoE−/− mice and ApoE−/− mice at the age of 20 weeks when all mice used in this study were killed. A slight decrease (10–15%) in body weight of the Thbs4−/− mice was detected in mice of 87–90 weeks of age. The skeletal system of Thbs4−/− and WT control mice was imaged using a custom-built microcomputed tomography system (Cleveland Clinic, Cleveland, OH) at 30 μm voxel resolution, and visualized using the VolSuite 3D rendering software (Ohio SuperComputer Center, Columbus, OH). The resulting data suggested that the abnormality in bone density and structure observed in Thbs3−/− mice24 was not present in the Thbs4−/− mice. Furthermore, skeletal anatomic aberrations were absent by visual inspection. Thbs4−/− mice were bred with ApoE−/− mice to obtain Thbs4−/−/ApoE−/− mice. These mice also displayed no overt phenotype. With the absence of a spontaneous phenotype, we began our analyses of atherosclerotic lesions in Thbs4−/−/ApoE−/− mice.
Atherosclerotic lesion area is decreased in the aortic root and descending aorta of Thbs 4−/−/ApoE−/− mice
For the analysis of atherosclerotic lesions, four groups of mice (n ≥ 11) were analyzed; ApoE−/− and Thbs4−/−/ApoE−/− mice were maintained either on a regular chow or a Western diet (42% calories from fat). All mice were analyzed for development of atherosclerosis at 20 weeks of age, 16 weeks after being placed on the two diets. In mice on the Western diet, lesions developed in the aortic root, aortic arch and the descending aorta and were measured at these sites; in mice on the chow diet, in which atherosclerosis develops slower, and lesions at the age of 20 weeks are restricted to the aortic root, only lesions in the aortic root were measured (Fig.1a). These measurements revealed that a deficiency in TSP-4 has a protective effect on the development of lesions. As measured by lipid staining (Oil Red O), the area of aortic root lesions was significantly decreased in Thbs4−/−/ApoE−/− mice on a chow diet, where lesions were small (Fig. 1a), but not on a Western diet, where the lesions in the aortic root were large (not shown). The lesions in the arch and descending aorta were significantly reduced in the Thbs4−/−/ApoE−/− mice compared to the ApoE−/− mice (Fig.1b). Thus, when lesions were small, either due to diet or to genetic background, TSP-4 deficiency suppressed lesion development. In larger, more developed lesions, the difference between lipid staining of lesions was not evident. However, despite the similar size of Oil Red O-stained areas in these larger lesions, inflammation and the number of inflammatory cells in the lesions were dramatically different, as described below.
The effect of the genotype on the size of the lesions was influenced by gender in the groups in Fig.1a and in the groups presented in Fig. 1b as was demonstrated by a two-factor ANOVA.
LDL/VLDL levels in Thbs4−/−/ApoE−/− mice
The cholesterol in the LDL/VLDL lipoprotein fraction was measured as described in Methods (Fig.1c). There was no difference in LDL/VLDL levels between genotypes, suggesting that the effect of TSP-4 on development of atherosclerotic lesions is determined by its functions in the vascular matrix and in cell interactions with the matrix, rather than on global changes in lipoprotein metabolism. Total cholesterol and HDL cholesterol levels were similar in both genotypes (not shown).
Cellular content of lesions in Thbs4−/−/ApoE−/− mice
The atherosclerotic lesions in aortic root of both genotypes were examined microscopically. Matrix deposition in the lesions was assessed by Masson trichrome staining, and no difference was observed between genotypes (Online Fig. IIA, a–d). Collagens 1, 3 and 4 were visualized individually, and no differences were observed between the two genotypes (Online Fig. IIB, a–f). However, microscopic evaluation suggested that the number and distribution of cells in the lesions were significantly different: Thbs4−/−/ApoE−/− mice had fewer cellular lesions with large acellular matrix depositions, and cells (apparently macrophages) concentrated closer to the luminal surface of the lesion. In contrast, lesions in ApoE−/− mice contained visibly higher numbers of cells, and these cells were more uniformly distributed throughout the lesion. The number of cells in the lesions was quantified as the area of lesions occupied by the nuclei (hematoxylin staining, fibrous cap and EC included, Fig.2A). The quantification confirmed that the lesions of Thbs4−/−/ApoE−/− mice were less cellular in all groups: 14.5±3.1% of area versus 38.3±11.5% in males on chow (p = 0.09); 12.6±4.1% versus 23.1±2.8% in females on chow (p = 0.02); 9.3±1.3% versus 14.2±1.3 in males on Western diet (p = 0.002) and 7.4±0.8% versus 15.3±1.2% in females on Western diet (p = 0.0008).
To determine which cell type(s) account for these differences in cellularity, we examined EC, smooth muscle cells and macrophages by immunohistochemistry. We did not detect significant numbers of CD31-positive cells (EC) in the lesions. α-Actin-positive smooth muscle cells were detected in the lesions of mice on the Western diet, both in ApoE−/− and in Thbs4−/−/ApoE−/− mice (Online Fig.III), but their numbers were not different between the two genotypes: 11.7±2.33% of lesion area in male ApoE−/− mice versus 15.7±2.6% in male Thbs4−/−/ApoE−/− mice (p = 0.13) and 25.2±3% in female ApoE−/− mice versus 25.9±2.2% in male Thbs4−/−/ApoE−/− mice (p = 0.4). The difference in the total cellularity of the lesions was due primarily to the difference in the number of macrophages in the lesions (Fig. 2B and C). The area of lesions occupied by macrophages was reduced ≥2 fold in all the groups (Fig. 2B). Unlike for lipid accumulation (Fig.1), the effect of the genotype on cellularity and macrophage content was not influenced by the gender as determined by two-factor ANOVA analysis.
Furthermore, while the lesions of ApoE−/− mice (including smaller, less developed lesions) had a large number of macrophages evenly distributed throughout the lesion, most Thbs4−/−/ApoE−/− mice had lesions with fewer macrophages and these cells were restricted to the surface of the lesions with low cellular infiltration into the matrix-rich body of the plaque. This pattern was obvious in both smaller and larger lesions (Fig.2C, a–d). No correlation was observed between lesion size, as determined by lipid staining, and the accumulation of macrophages in the lesions in Western-diet-fed mice. Thus, while the quantification of lipid staining indicated an equal lesion size in the aortic root of mice of the two genotypes (Fig.1a), the area stained for macrophages in the lesions was twice smaller in Thbs4−/−/ApoE−/− mice than in the ApoE−/− mice, suggesting that macrophage recruitment was influenced by TSP-4 despite the equal lipid content of the lesions in mice of both genotypes.
TSP-4 in atherosclerotic lesions and blood vessels of ApoE−/− mice
We examined the patterns of TSP-4 expression in the lesions and the adjoining area of blood vessels of ApoE−/− and in the aortas of wild-type mice. Remarkably, strong expression of TSP-4 was detected in areas of branching of intercostal arteries of ApoE−/− mice, sites especially prone to development of atherosclerotic lesions (Fig. 3a). Such localization of TSP-4 was only detected in ApoE−/− mice but not in wild type C57BL6 mice (Fig. 3b). TSP-4 was found in the tunica adventitia of both genotypes (Fig. 3, a–c).
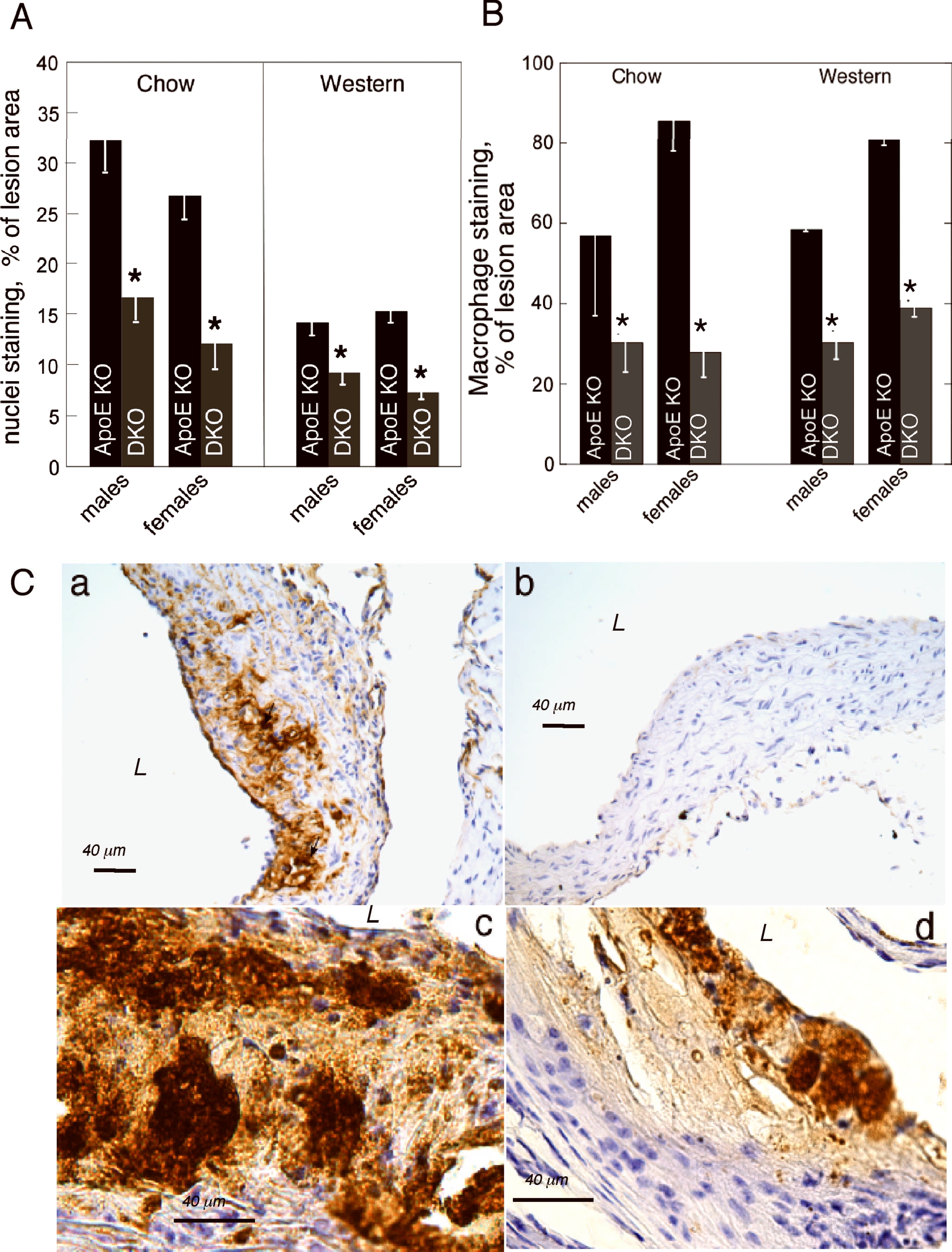
TSP-4 (red, anti-TSP-4 antibody) was found in tunica adventitia (a–c), at branches of intercostals arteries of ApoE−/− (a) but not wild type mice (b), and in the matrix of atherosclerotic plaques (d, e). TSP-4 associated with EC is shown by arrows in d and e. A = atherosclerotic lesion; L = lumen of aorta; m = tunica media of aorta, elastic lamina have green autofluorescence; solid arrows identify macrophages in a, d, and e (anti-F4/80 antibody, green); dashed arrows indicate the lumens of intercostal arteries, nuclei are stained with DAPI (blue color). a, d, e: ApoE KO, 20 week-old male, ×60, ×20 ×20, respectively; b,c: wild type C57BL6, 20 week-old male, ×60 and ×20, respectively.
TSP-4 was detected in all atherosclerotic lesions, regardless of their size (Fig. 3 d,e). It was abundant in the lesion matrix (Fig. 3 d,e) and was present within EC, as shown by immunostaining (Fig. 3 d,e). Macrophages in the lesions of ApoE−/− mice were observed in close proximity to TSP-4 (Fig. 3 a,d,e). However, no intracellular TSP-4 was detected in macrophages (unlike in EC), suggesting that macrophages within lesions do not produce TSP-4. Blood monocytes or peritoneal macrophages of wild-type or ApoE−/− mice on chow or Western diet did not produce detectable TSP-4, as was documented in Western blotting and by ELISA (not shown).
TSP-4 is homologous to two other thrombospondins, TSP-3 and TSP-5, and we were concerned that the observed effects of TSP-4 deletion might be a consequence of compensatory expression and function of another TSP family member. To examine the relationships among the three TSPs in the lesions, we stained blood vessels of ApoE−/− mice for all three TSPs. Like TSP-4, both TSP-3 and TSP-5 were also present in the lesions. However, while TSP-3 was abundant in the tunica media and tunica adventitia, on the surface of luminal EC and in the atherosclerotic lesions, TSP-5 was limited to the tunica media and was associated with cells of the media and with a few cells in the lesion (Online Fig. IV a–d). Furthermore, the expression patterns of the three TSPs were different; they appeared at different locations in the blood vessels and in atherosclerotic lesions, and, hence are likely to have distinct functions in the vascular wall. The specificity of observed immunostaining was confirmed by the lack of staining in lesions of Thbs4−/−/ApoE−/− mice (anti-TSP-4 antibody, Online Fig. V a,b), the lack of staining in lesions with the secondary antibody only (Online Fig. V c,d), and the lack of staining in aortas of Thbs3−/−/Thbs4−/−/Thbs5−/− mice (Online Fig. V e–h). TSP-1 was also abundantly present in the lesions of both ApoE−/− mice (Online Fig. IVe) and Thbs4−/−/ApoE−/− mice (Online Fig. IVf) in equal amounts, but only traces of TSP-2 were detected in the lesions of both genotypes (Online Fig. IV g,h).
As an independent approach to corroborate these findings, Western blotting was performed with extracts of aortas of wild-type and Thbs4−/− mice, and with extracts of aortas of ApoE−/− and Thbs4−/−/ApoE−/− mice on both diets. This analysis revealed that the levels of TSP-3 and TSP-5 were not increased in Thbs4−/− vessel tissue or in Thbs4−/−/ApoE−/− aortas from mice on chow diet (Online Fig.VI). Western diet increased the levels of all three TSPs. Of the various comparisons, only the level of TSP-3 was slightly higher in Thbs4−/−/ApoE−/− aortas from mice on a Western diet as compared to aortas of ApoE−/− mice on a Western diet.
TSP-4 and macrophage function
Despite the absence of detectable TSP-4 in macrophages (Fig. 3 d,e), we considered that TSP-4 might influence macrophage function, and thus selected responses were evaluated.
Effect of TSP4 on the uptake of acetylated LDL by macrophages
The uptake of acetylated LDL is a function that is critical to the formation of atherosclerotic lesions. Peritoneal macrophages from WT mice were cultured on 0–50 μM recombinant TSP-4. TSP-4 had no effect on the formation of foam cells (data not shown).
Migration and adhesion of Thbs4−/− peritoneal macrophages
Peritoneal macrophages were isolated from peritoneal fluid of ApoE−/− and Thbs4−/−/ApoE−/− mice on a chow or Western diet, 72 hours after injection of thioglycollate to induce peritonitis. At this time point, 95% of cells in peritoneal fluid were identified by microscopy or FACS as macrophages. The cells were counted to assess the in vivo migratory ability of macrophages, but no difference in cell counts was found between the two genotypes (Online Fig. VIIA).
The effect of TSP-4 deletion on adhesion and migration of peritoneal macrophages isolated from wild type and Thbs4−/− mice was assessed. No difference was found between macrophages from the two genotypes to adhere or migrate in vitro in response to MCP-1 and Gro1α (Online Fig. VIIB).
Since we were unable to detect differences in these macrophage responses and we could not detect TSP-4 in macrophages, we assessed the effect of exogenously added recombinant TSP-4 on the adhesion and migration of macrophages.
Adhesion and migration of monocytoid cells to purified recombinant TSP-4
RAW 264.7 cells, a widely used murine macrophage-like cell line, were employed initially to assess the effects of TSP-4 on adhesive and migratory responses. TSP-4 supported both the adhesion of RAW 264.7 cells in a dose-dependent manner, and a stimulus, MCP-1, did not affect the extent of adhesion (Fig. 4A, a). RAW264.7 cells migrated onto TSP-4 in a dose-dependent manner (Fig. 4A, b), and MCP-1 increased the migration almost two-fold. Maximum migration was reached at 5 μg/ml of rTSP-4 and decreased at higher concentrations, i.e., increased adhesion may impede migration as is often observed on matrix proteins. Macrophage adhesion and migration to extracellular matrix proteins such as the TSPs are integrin-mediated responses. We utilized a series of blocking antibodies to candidate integrins, as well as to non-integrins as controls. As shown in Fig. 4A, c and d, only two of the antibodies tested were effective inhibitors of macrophage adhesion and migration on TSP-4. These were antibodies to integrins αMβ2 and to the β3 subunit. Antibodies to other integrins, α5β1 and the β4 subunit, and to non-integrins, CD36 and MHC, had little effect.
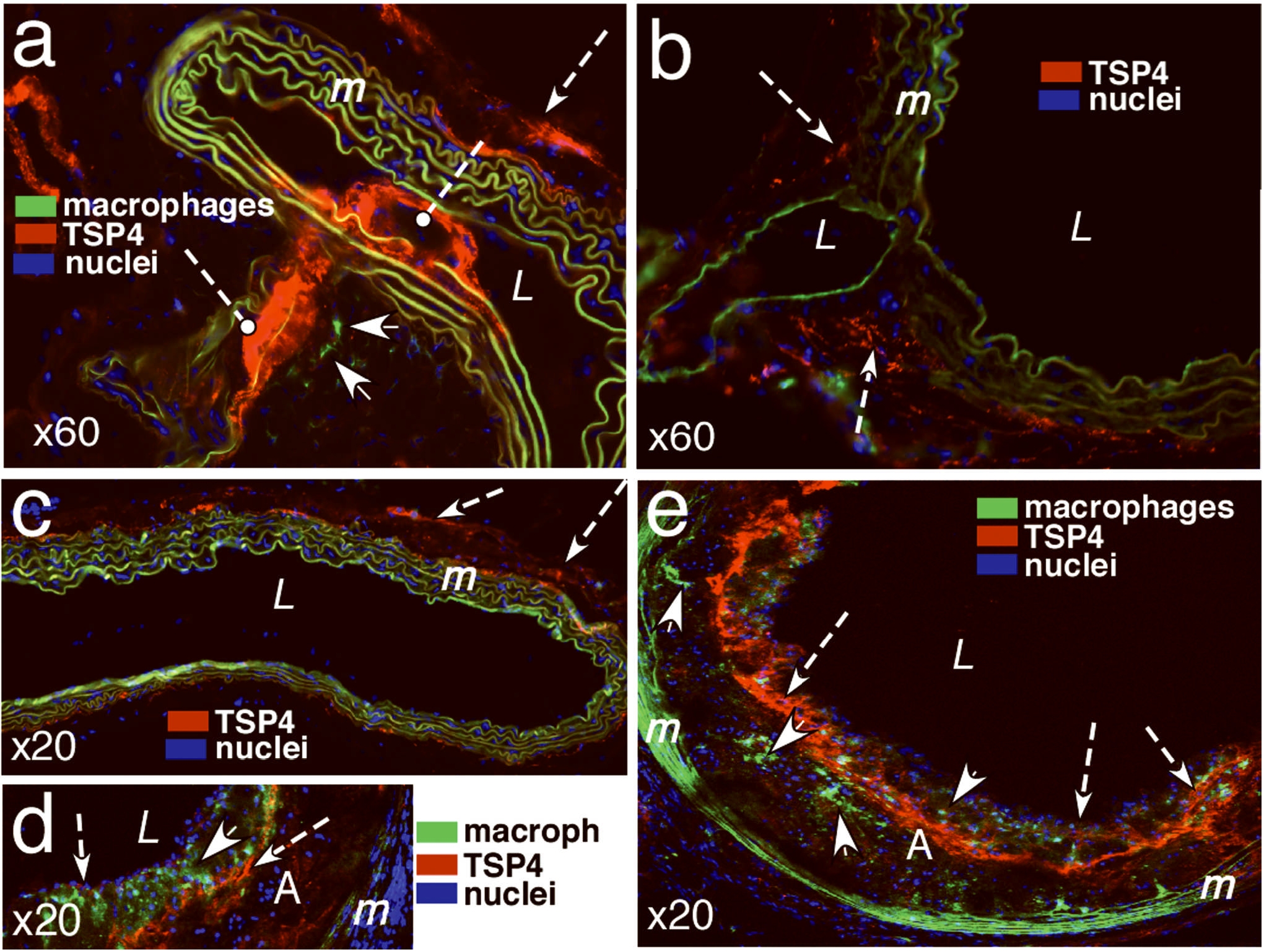
A, B: Cell adhesion, a, and cell migration, b, were measured, as described in Methods, on plates pre-coated with purified recombinant TSP-4 or through filters pre-coated with TSP-4 in the presence of MCP-1 or without MCP-1. Inhibition of adhesion, c, or migration, d, by antibodies (see Methods) that block the function of various murine adhesion receptors expressed by macrophages was observed. A: undifferentiated RAW 264.7 cells; B: peritoneal macrophages from wild type C57BL6 mice. C: Adhesion of macrophages to rTSP-4 through αMβ2 and β3 integrins induces phosphorylation of p38 MAPK. Peritoneal macrophages from WT mice were pretreated with blocking antibodies to integrins and plated on rTSP-4-coated plates. Western blots were developed with anti-phospho-p38 and anti-p38 MAPK.
Similar to the effect of TSP-4 on RAW264.7 cells, the adhesion and migration of peritoneal macrophages was supported by TSP-4 in a dose-dependent manner (Fig. 4B, a and b, respectively). Antibodies to integrins αMβ2 and to the β3 subunit again blocked both adhesion and migration while anti-α5β1 and anti-MHC failed to affect either response, confirming that the interaction of macrophages with TSP-4 depends on β2- and β3-containing integrins (Fig. 4B, c and d).
Intracellular signaling initiated by TSP-4 - macrophages interaction
We have previously reported that interaction of neutrophils and EC with TSP-4 initiates intracellular phosphorylation and activates intracellular kinases7, 8. In neutrophils adherent to TSP-4, phosphorylation of p38MAPK was particularly robust. Increased phosphorylation of p38MAPK was analyzed by Western blots with specific antibodies in peritoneal macrophages adherent to rTSP-4. A marked increase in phospho-p38MAPK was noted in the cells adherent to TSP-4 (Fig. 4C). The increase in phospho-p38MPK occurred in the absence of detectable changes in total cellular p38MAPK. The increase in phosphorylation of p38MAPK was prevented by pre-incubation with either anti-β3 or anti-αMβ2 antibodies, suggesting that the activation of p38 MAPK is a consequence of engagement of these integrins by TSP-4.
To assess the activation of this important intracellular mediator of inflammatory signaling in lesions of ApoE−/− and Thbs4−/−/ApoE−/− mice on chow or Western diets, lesions in the aortic root of mice of all groups were stained with anti-phospho-p38MAPK (Fig.5) and the area of staining was quantified (Fig.5A). The activation of p38MAPK was decreased in Thbs4−/−/ApoE−/− mice in all groups, with an exception of males on a chow diet.
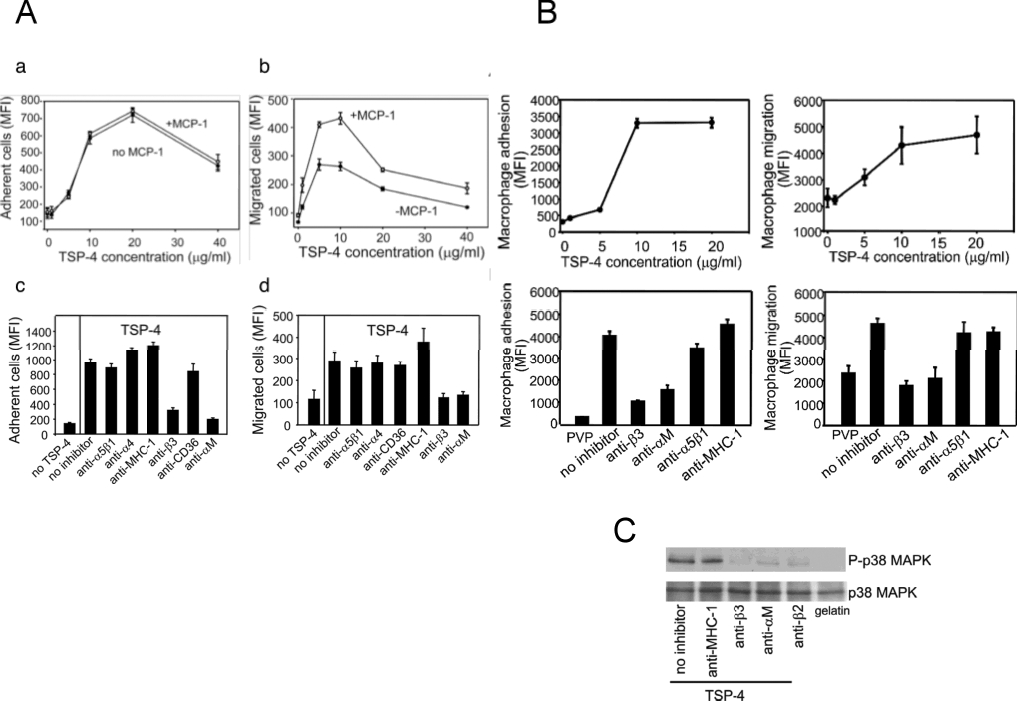
Anti-p38 antibody was used for staining of aortic root (brown color) as described in Methods and in Fig.2 legend, and the stained area was quantified as percent of lesion area. A: percent of stained lesion area, pxl, mean ± SEM. *Statistically significant changes (p<0.05). B: b – 16 weeks of Western diet, ApoE−/−, male; c - 16 weeks of Western diet, Thbs4−/−/ApoE−/−, male; d – 16 weeks of Western diet, ApoE−/−, female; e - 16 weeks of Western diet, Thbs4−/−/ApoE−/−, female. DKO = Thbs4−/−/ApoE−/− double KO mice.
The role of αMβ2 and β3 integrins in macrophage interactions with TSP-4 and initiation of pro-inflammatory signaling was confirmed using peritoneal macrophages from αMβ2 and β3 subunit KO mice (Fig.6). In both αMβ2 and β3 subunit knockout macrophages, the ability to adhere (Fig.6A,B) and activate p38MAPK (Fig.6C) was reduced, consistent with the inhibition of these functions by corresponding antibodies (Fig.4C). Macrophage migration was reduced in αMβ2-deficient macrophages, but not in β3 subunit knockout cells (Fig.6B). The lack of effect of β3 may be due to compensatory mechanisms that are known to occur in these mice25.
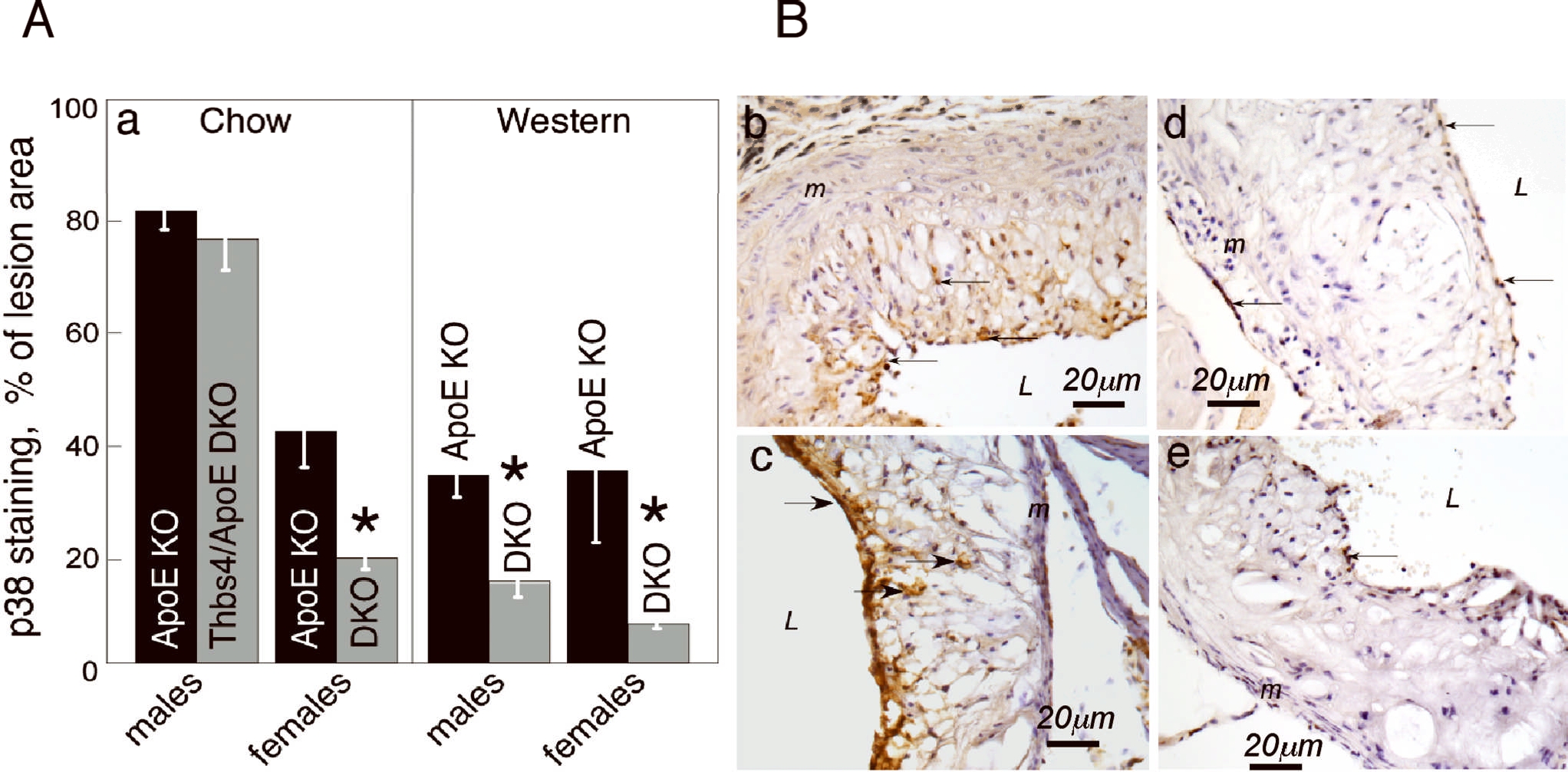
Cell adhesion, left panels in A and B, and cell migration, right panels in A and B, were measured as described in Methods and in Fig. 4 legend. Closed circles = wild type macrophages; open circles = αM-integrin deficient macrophages in a and β3-integrin-deficient macrophages in b. C: Reduced phosphorylation of p38 MAPK in αM-integrin deficient macrophages (upper panel) and β3-integrin-deficient macrophages adherent to rTSP-4 by Western blotting.
Reduced vascular inflammation and EC activation in Thbs4−/−/ApoE−/− mice
As a secreted protein, TSP-4 in the matrix is available for interactions with many cell types and may influence pro-inflammatory processes in lesions in multiple ways. To assess the effect of a deficiency of TSP-4 on the levels of inflammatory mediators and chemoattractant MCP-1 in the aorta, we visualized MCP-1 in the lesions of mice of both genotypes on chow and Western diets (Fig.7A, a–d). In both genotypes, as expected, MCP-1 levels were increased by the Western diet in all the layers of aorta, but especially in the lesions themselves. However, there was also a remarkable decrease in MCP-1 levels in the lesions, in the tunica media and tunica adventitia of Thbs4−/−/ApoE−/− mice as compared to a gender- and diet-matched control group of ApoE−/− mice.
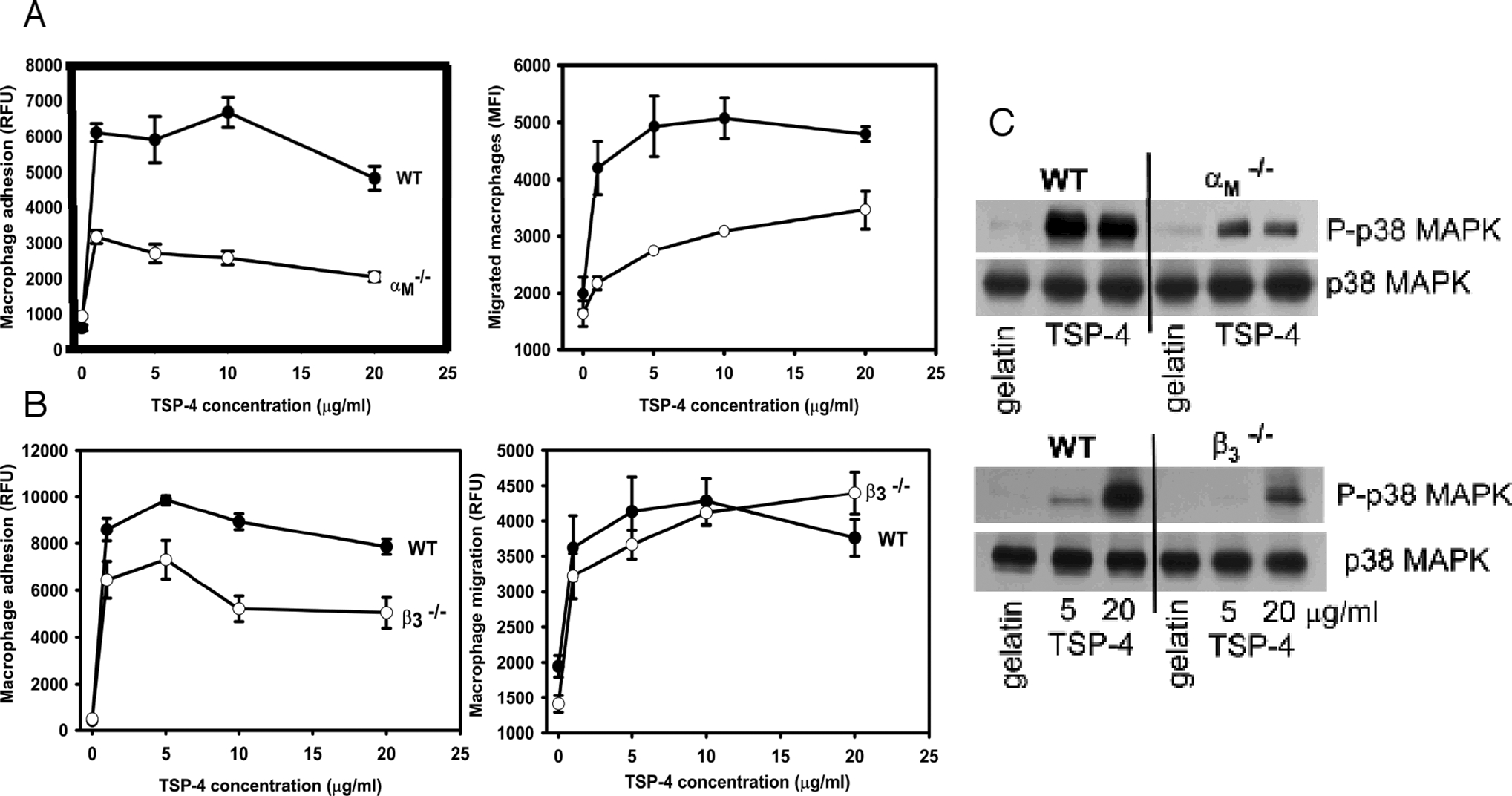
MCP-1 levels were assessed in aortas of ApoE−/− and Thbs4−/−/ApoE−/− mice by immunohistochemistry and ELISA. A: staining in the aortic root of ApoE−/− (a, c) and Thbs4−/−/ApoE−/− mice (b, d) on chow (a, b) and Western diet (c, d), anti-MCP-1 antibody, red staining. B: (a) Extracts of aortic tissue were prepared as described in Methods, and MCP-1 levels in aortic wall were measured using a MCP-1 ELISA. * - p <0.05. MCP-1 production by cultured EC was measured in culture media of aortic EC from wild type and Thbs4−/− mice (b, * - p <0.05) and in culture media of microvascular lung EC isolated from wild type, Thbs4−/−, ApoE−/−, and Thbs4−/−/ApoE−/− mice (c, * - p <0.05 in comparison between wild type and Thbs4−/−, # - p <0.05 in comparison between ApoE−/−, and Thbs4−/−/ApoE−/− cells).
MCP-1 levels were further evaluated in extracts of aortas by ELISA (Fig.7B,a). The difference observed by immunohistochemistry in aortas of mice on chow could not be confirmed by ELISA, either due to insufficient sensitivity of the assay or to the smaller size of lesions, which may contribute a large part of the total MCP-1 in aortic tissue. However, in aortas of mice on a Western diet, in which both the levels of MCP-1 were higher and the lesions were larger, the 3-fold decrease in the MCP-1 level in Thbs4−/−/ApoE−/− mice was confirmed.
As became clear from the immunohistochemical staining, the source of MCP-1 (red staining in Fig. 7A) was not limited to macrophages (green staining). We evaluated the effect of TSP-4 deficiency on production of MCP-1 by EC isolated from wild type, Thbs4−/−, ApoE−/− and Thbs4−/−/ApoE−/− mice (Fig. 7B, b and c). As measured by ELISA in culture media, both basal and LPS-induced levels of MCP-1 released into the conditioned media of EC derived from Thbs4−/−/ApoE−/− mice were consistently and significantly lower than from EC derived from ApoE−/− mice. EC of the second passage were used in these experiments. The levels of MCP-1 were normalized to the number of cells harvested from each plate at the end of this experiment.
Luminal aortic EC produce TSP-4 (Fig. 3 d,e), and the MCP-1 levels in cultured cells of the two genotypes suggested that the activation of EC may be influenced by TSP-4 and may contribute to the difference in macrophage recruitment between two genotypes. The expression of the surface adhesion molecules, E-selectin, VCAM-1 and ICAM-1, was assessed by FACS analysis in cultured EC from wild type, Thbs4−/−, ApoE−/−, and Thbs4−/−/ApoE−/− mice (Fig. 8A). The expression of all three surface proteins was dramatically decreased in LPS-stimulated Thbs4−/− EC as compared to those in wild type EC (Fig. 8A, upper panel). The differences became smaller in mice on an ApoE−/− background (Fig. 8A, lower panel). Out of three markers of endothelial activation tested in these in vitro experiments, E-selectin was the most consistently down-regulated surface protein while ICAM-1 and VCAM-1 showed smaller differences between the two genotypes on an ApoE background.
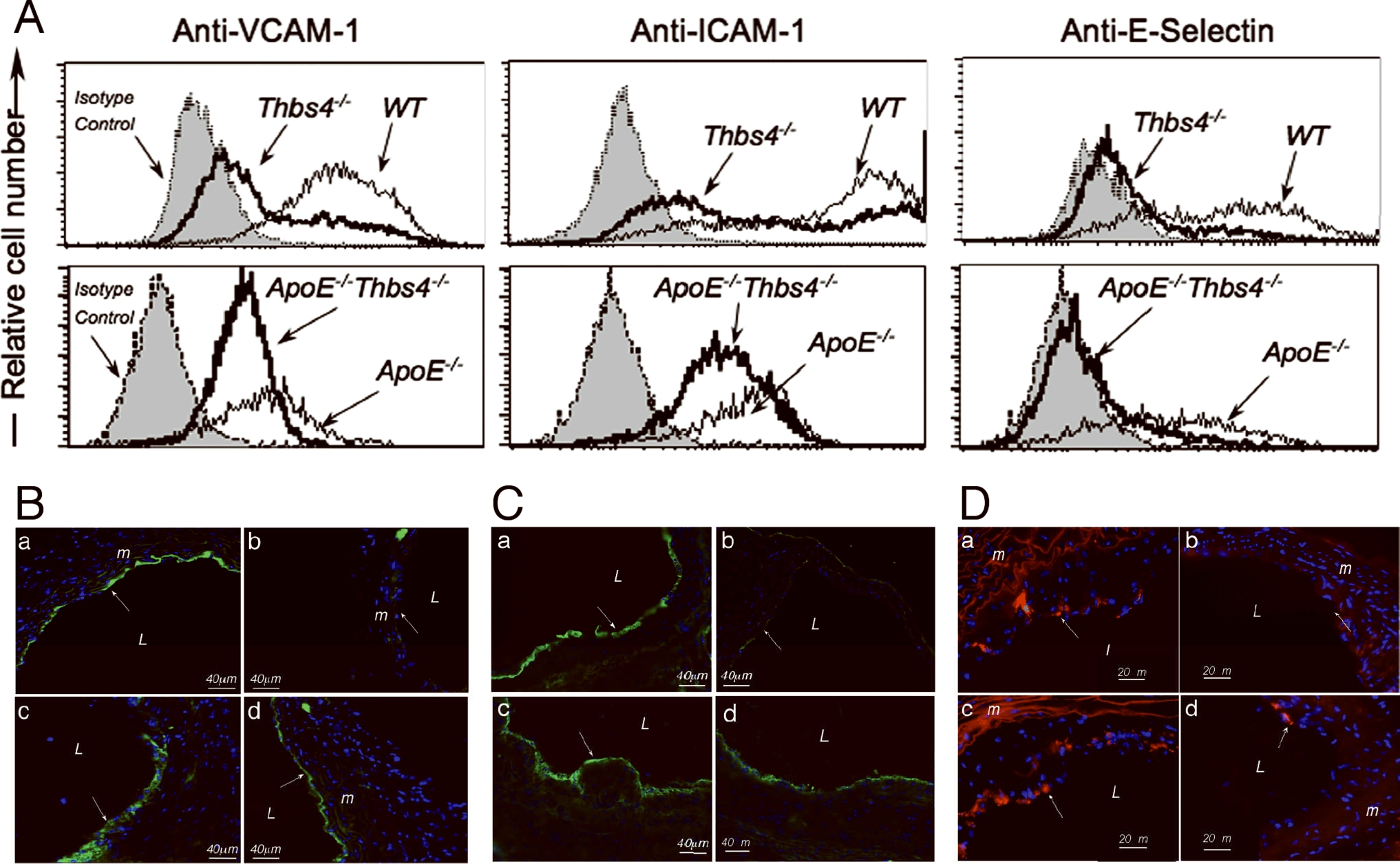
A: Cultured microvascular lung EC were stimulated with LPS for 4 hours (for E-selectin expression) or 18 hours (for VCAM-1 and ICAM-1 expression). The expression was assessed by FACS using specific antibodies. B: VCAM-1; C: ICAM1; D: E-selectin; expression in aorta of ApoE−/− (a, c) and Thbs4−/−/ApoE−/− mice (b, d) on chow (a, b) and Western diet (c, d). Immunostaining with anti-VCAM-1 antibody (green); L = lumen.
The EC environment in a cell culture model is different from the in vivo environment of luminal EC of the aortic wall. We sought to confirm the reduced activation of Thbs4−/− EC in vivo. Immunostaining of aortas of ApoE−/− and Thbs4−/−/ApoE−/− mice with anti-VCAM-1, anti-ICAM-1 and anti-E-selectin antibodies confirmed that the activation of luminal endothelial cells is reduced in Thbs4−/− animals in vivo (Fig. 8B–D). The staining was visibly reduced in double knockout mice both on chow (Fig. 8B–D,b) and on Western (Fig. 8B–D,d) diets.
DISCUSSION
TSPs are multifunctional matricellular proteins which interact with a wide variety of receptors and other binding partners to induce responses in numerous cell types, including many circulating blood cells and cells of the vessel wall1, 2, 4. The influence of TSPs on cellular responses such as adhesion, migration and proliferation of vascular cells suggests that they might exert a significant effect on atherogenesis, but very little information is available in this regard. In a recent study, deficient mice were used to show that TSP-1 influences early lesion formation and exerts a significant effect on the maturation of atherosclerotic lesions19. TSP-4 has been linked to several cardiovascular pathologies, including myocardial infarction, through an unknown mechanism.
In the present study, we examined the effect of a lack of TSP-4 on the development of atherosclerotic lesions in ApoE−/− mice. The absence of TSP-4 was protective in smaller, less developed lesions, e.g., in the aortic roots of mice on chow diet and in the aortic arches and descending aortas of mice on a Western diet. The decrease in development of lesions in the aortic root (39–48%), was dramatic in mice on a regular chow diet and was observed in both genders. These differences were highly significant and the extent of this reduction is as dramatic (or greater) as that observed in mice deficient in other molecules with well established roles in atherogenesis, such as PAI-1 (≤40% decrease in lesion area in aortic arch26), MCP-1 receptor CCR2 (~42% decrease in lesion area27) and iNOS (30–50% reduction28, 29). However, the most remarkable feature of atherogenesis in Thbs4−/−/ApoE−/− mice is the reduced vascular inflammation, characterized by decreased cellularity, decreased activation of EC, and decreased pro-inflammatory signaling within lesions. In larger, advanced lesions in the aortic root of mice on a Western diet, the effect of TSP-4 deficiency on the size of lesions, as determined by lipid staining, was lost. However, the TSP-4 effect on the composition of the lesion and the extent of inflammation in the lesion was preserved in all groups, independent of the size and lipid content of lesions. VLDL/LDL levels were identical in both genotypes on the chow or Western diet, suggesting that the protective effect of TSP-4 deficiency is due to its effects in the vascular wall rather than on metabolism.
A striking difference in the lesions of Thbs4−/−/ApoE−/− mice compared to those of ApoE−/− mice is the presence of substantially fewer cellular elements in Thbs4−/− mice, regardless of lesion size. Detailed analysis of the cells within lesions revealed that the number of macrophages was reduced, and their location in the lesion was changed. Other cell types in the lesions were not affected.
TSP-1 and TSP-4 are highly homologous in the region that in TSP-1 stimulates SMC proliferation though αvβ3 integrin30. We have observed that the proliferation of cultured SMC is increased in the presence of recombinant C-terminal fragments of TSP-47, and β3 has been identified as one of the receptors for TSP-4 in this report and previously8. However, there was no difference in SMC numbers between the lesions of Thbs4−/−/ApoE−/− and ApoE−/− mice.
We investigated further the interaction of macrophages with recombinant TSP-4, focusing on the formation of foam cells, adhesion and migration. No effect of TSP-4 on uptake of acetylated LDL and formation of foam cell was detected. However, TSP-4 supported adhesion and migration of macrophages in a dose-dependent manner. These functional activities of TSP-4 were observed with a macrophage cell line as well as with peritoneal macrophages and may account for the decrease in the accumulation of macrophages in atherosclerotic lesions in Thbs4−/−/ApoE−/− mice, as compared to that in ApoE−/− mice.
In investigating the mechanism by which TSP-4 might influence macrophage adhesion and migration, we found that antibodies to two integrins blocked these responses in a murine macrophage cell line. Antibodies to integrin αMβ2 and the integrin β3 subunit (integrin αVβ3 is the only β3 expressed by these cells) were potent inhibitors of macrophage adhesion and migration on TSP-4. Of note, in a previous study of neutrophils, we implicated these two integrins in the adhesion and migration of these cells by using both antibody inhibition and expression approaches7. As in neutrophils, we noted that adhesion of macrophages to TSP-4 led to phosphorylation of p38MAPK, a reflection of intracellular signaling. This activation was inhibited by antibodies to αMβ2 but not to αVβ3 integrin. Deficiencies of both of these integrins and inhibition of p38MAPK have been shown to affect vascular wall remodeling, inflammation in the vascular wall and atherogenesis in mice31–34. αVβ3 integrin was athero-protective and anti-inflammatory in the published reports32,33. The discrepancy between the two models in their effects on atherogenesis may be due to compensatory mechanisms in the αVβ3 integrin KO mouse or to a predominant effect of other ligands for the integrin or other receptors for TSP-4. Indeed, our data provide direct evidence for involvement of a second integrin, αMβ2, in TSP-4-induced responses. Of note, the lack of the effect of β3 integrin knockout on migration (Fig.6B, right panel) was inconsistent with the results of experiments employing the inhibitory antibody (Fig. 4A,d and 4B,d), also suggesting a compensation in the β3 integrin knockout mouse. Such differences between antibody inhibition and β3 integrin knockout mice were previously observed in the angiogenic response35 and were ascribed to a compensatory mechanism (upregulation of VEGFR2) in the KO mice.
While antibodies specific for αMβ2 were very effective in blocking macrophage responses to TSP-4, we do not exclude that other β2 integrins might recognize TSP-4 and thereby contribute to atherogenesis. Wu et al36 reported that CD11c (αXβ2) is upregulated by hypercholesterolemia in apoE mice on a high fat diet and CD11c KO mice are resistant to diet-induced atherosclerosis. Of note, αCβ2 and αDβ2 share many ligands in common with αMβ237
The interaction of macrophages with TSP-4 induced activation of p38MAPK, a major intracellular mediator of the production of cytokines and inflammatory mediators38, as well as endothelial-leukocyte interactions39 and motility in macrophages40. p38 MAPK has been implicated in signaling, leading to the production of MCP-1, macrophage inflammatory protein 1beta and alpha (MIP-1), IL-1beta, IL-6, IL-8, IL-10, GM-CSF and TNF by macrophages41, 42. Immunostaining of lesions revealed that both p38MAPK and MCP-1 levels in the lesions are reduced in Thbs4−/−/ApoE−/− mice, which in turn would reduce inflammation and leukocyte recruitment into the lesion, in addition to the direct effect of the lack of TSP-4.
As a result of TSP-4 deficiency, vascular inflammation was reduced through multiple mechanisms, including reduced EC activation, monocyte/macrophage recruitment, macrophage migration inside the lesion and pro-inflammatory signaling in macrophages. Consequently, the cellularity and inflammation within lesions became substantially reduced, even when the lipid content within the lesions of ApoE−/− mice was similar.
Thus, we propose that TSP-4 may provide an important substrate for the recruitment of monocytes/macrophages into lesion-prone areas. The absence of TSP-4 in the Thbs4−/− mice may impede initiation and progression of atherogenesis. While some of the effects of TSP-4 and TSP-1 deficiencies on atherogenesis appear to be similar, these proteins seem to influence the advanced lesions differently. Thus, while TSP-1 deficiency results in accelerated maturation of the lesion by inhibiting phagocytosis in macrophages19, TSP-4 deficiency reduces macrophage recruitment into the lesion, their migration in the lesion and pro-inflammatory signaling in macrophages and EC. In view of differences in structure and binding partners for TSP-1 and TSP-4, it is not surprising that they have different functions in the vascular wall and differently affect the macrophages in the lesions.
Monocytes/macrophages or any other blood cell types do not produce detectable amounts of TSP-4, and Thbs4−/− macrophages do not differ from WT macrophages in their ability to adhere and migrate to chemoattractants. TSP-4 is always expressed in atherosclerotic lesions, independent of their size and location, and is clearly not solely responsible for macrophage recruitment. However, it is noteworthy that TSP-4 is abundant in areas of blood vessels that are prone to the development of lesions, e.g., sites of branching of intercostal arteries. These findings suggest that in these areas the adhesion and migration of monocytes/macrophages is enhanced, compared to these processes in adjacent areas. In other parts of aorta and in other large arteries and veins, TSP-4 was found in the tunica adventitia, but its presence there was discontinuous.
In conclusion, our results establish the effects of a deficiency in TSP-4 on atherogenesis and suggest a role for TSP-4 in the vascular wall and in inflammation. Inflammation drives the development of lesions from their initiation to the formation of the vulnerable plaque43,44. The inflammatory status of the lesion correlates with thrombosis and clinical manifestations. As suggested by our data, increased TSP-4 expression may affect local inflammation through several mechanisms: increased adhesion and migration of monocytes/macrophages through the blood vessel due to the direct interaction of monocytes/macrophages with TSP-4, abundantly present in lesions, the activation of EC and increased expression of surface adhesion proteins on their surface, and the increased inflammatory and atherogenic stimuli produced by both EC and macrophages. These observations provide a framework within which the role of TSP-4 in the development of cardiovascular disease in humans can be considered.
ACKNOWLEDGMENTS
We thank Dr. J. Barnard (Cleveland Clinic) for the review of statistical analyses, Dr. Eugene Podrez (Cleveland Clinic) for the foam cell formation assay and Dr. Judith Drazba (Cleveland Clinic, Imaging Core) for assistance with generation of imaging data. We also thank Dr. Jacqueline Hecht (The University of Texas Health Science Center at Houston) for Thbs3−/−/5−/− double knockout mice.
SOURCES OF FUNDING This work was supported by National Institutes of Health Grants P50 HL077107 (to EFP) and R01 DK067532 (to OIS).
Non-standard Abbreviations and Acronyms
ES cell line | embryonic stem cell line |
EC | endothelial cells |
DAPI | 4', 6-diamidino-2-phenylindole |
FITC | fluorescein isothiocyanate |
HDL | high density lipoproteins |
IOD | integrated optical density |
LDL | low density lipoproteins |
p38-MAPK | p38 mitogen activated phosphokinase |
PVP | polyvinylpyrrolidone |
TSP | thrombospondin |
VLDL | very low density lipoproteins |
WT | wild type |
Footnotes
DISCLOSURES: None.
Publisher's Disclaimer: This is a PDF file of an unedited manuscript that has been accepted for publication. As a service to our customers we are providing this early version of the manuscript. The manuscript will undergo copyediting, typesetting, and review of the resulting proof before it is published in its final citable form. Please note that during the production process errors may be discovered which could affect the content, and all legal disclaimers that apply to the journal pertain.
REFERENCES
Full text links
Read article at publisher's site: https://doi.org/10.1161/circresaha.110.232371
Read article for free, from open access legal sources, via Unpaywall:
https://www.ahajournals.org/doi/pdf/10.1161/CIRCRESAHA.110.232371
Free after 12 months at intl-circres.ahajournals.org
http://intl-circres.ahajournals.org/cgi/content/full/107/11/1313
Free after 12 months at intl-circres.ahajournals.org
http://intl-circres.ahajournals.org/cgi/reprint/107/11/1313.pdf
Free to read at intl-circres.ahajournals.org
http://intl-circres.ahajournals.org/cgi/content/abstract/107/11/1313
Citations & impact
Impact metrics
Citations of article over time
Alternative metrics
Article citations
The role and mechanism of thrombospondin-4 in pulmonary arterial hypertension associated with congenital heart disease.
Respir Res, 25(1):313, 17 Aug 2024
Cited by: 0 articles | PMID: 39154161 | PMCID: PMC11330619
Thrombospondin-4 deletion does not exacerbate muscular dystrophy in β-sarcoglycan-deficient and laminin α2 chain-deficient mice.
Sci Rep, 14(1):14757, 26 Jun 2024
Cited by: 0 articles | PMID: 38926599 | PMCID: PMC11208443
A proteomics-based survey reveals thrombospondin-4 as a ligand regulated by the mannose receptor in the injured lung.
J Biol Chem, 300(5):107284, 13 Apr 2024
Cited by: 0 articles | PMID: 38614208 | PMCID: PMC11107221
The molecular mechanism of thrombospondin family members in cardiovascular diseases.
Front Cardiovasc Med, 11:1337586, 07 Mar 2024
Cited by: 0 articles | PMID: 38516004 | PMCID: PMC10954798
Review Free full text in Europe PMC
Unraveling the Role of Sex in Endothelial Cell Dysfunction: Evidence From Lineage Tracing Mice and Cultured Cells.
Arterioscler Thromb Vasc Biol, 44(1):238-253, 30 Nov 2023
Cited by: 2 articles | PMID: 38031841
Go to all (68) article citations
Data
Data behind the article
This data has been text mined from the article, or deposited into data resources.
BioStudies: supplemental material and supporting data
Similar Articles
To arrive at the top five similar articles we use a word-weighted algorithm to compare words from the Title and Abstract of each citation.
Effects of thrombospondin-4 on pro-inflammatory phenotype differentiation and apoptosis in macrophages.
Cell Death Dis, 11(1):53, 23 Jan 2020
Cited by: 25 articles | PMID: 31974349 | PMCID: PMC6978349
The P387 thrombospondin-4 variant promotes accumulation of macrophages in atherosclerotic lesions.
FASEB J, 34(9):11529-11545, 20 Jul 2020
Cited by: 1 article | PMID: 32686880
Role of endothelial cell-derived angptl2 in vascular inflammation leading to endothelial dysfunction and atherosclerosis progression.
Arterioscler Thromb Vasc Biol, 34(4):790-800, 13 Feb 2014
Cited by: 87 articles | PMID: 24526691
Thrombospondins: old players, new games.
Curr Opin Lipidol, 24(5):401-409, 01 Oct 2013
Cited by: 26 articles | PMID: 23892609 | PMCID: PMC3935726
Review Free full text in Europe PMC
Funding
Funders who supported this work.
NHLBI NIH HHS (3)
Grant ID: P50 HL077107
Grant ID: R01 HL098193
Grant ID: R01 HL077781-01
NIDDK NIH HHS (2)
Grant ID: R01 DK067532-04
Grant ID: R01 DK067532