Abstract
Free full text

The role of matrix metalloproteases in cystic fibrosis lung disease
Abstract
Significant airway remodeling is a major component of the increased morbidity and mortality observed in cystic fibrosis (CF) patients. These airways feature ongoing leukocytic inflammation and unrelenting bacterial infection. In contrast to acute bacterial pneumonia, CF infection is not cleared efficiently and the ensuing inflammatory response causes tissue damage. This structural damage is mainly a result of free proteolytic activity released by infiltrated neutrophils and macrophages. Major proteases in this disease are serine and matrix metalloproteases (MMPs). While the role of serine proteases, such as elastase, has been characterized in detail, there is emerging evidence that MMPs could play a key role in the pathogenesis of CF lung disease. This review summarizes studies linking MMPs with CF lung disease and discusses the potential value of MMPs as future therapeutic targets in CF and other chronic lung diseases.
Proteolytic activity in cystic fibrosis lung disease
Chronic progressive destruction of the lung is the major cause of death in patients with cystic fibrosis (CF) [1]. While gastrointestinal symptoms are treated efficiently by established medications, such as pancreatic enzyme replacement therapy, the progression of pulmonary symptoms with destruction of pulmonary tissue cannot be controlled sufficiently thus far. CF airways are characterized by airway surface liquid (ASL) depletion producing mucus obstruction and chronic inflammation with persistent leukocyte accumulation, mainly of neutrophils and macrophages. Despite the presence of millions of activated phagocytes, bacterial pathogens such as Pseudomonas aeruginosa, Staphylococcus aureus and Haemophilus influenzae flourish in the CF airway microenvironment, resulting in chronic infection. Since recruited leukocytes are impaired in their antibacterial capacity due to decreased mucociliary clearance [2], CXCR1 receptor cleavage [3], excessive but inefficient neutrophil extracellular trap (NET) formation [4] and other mechanisms, the dehydrated mucus and extracellular DNA obstruct the airways and other toxic products, such as proteases and oxidants, cause host tissue damage. Major proteases in this disease situation are serine and matrix metalloproteases (MMPs). While the role of serine proteases such as neutrophil elastase (NE), cathepsin G and proteinase 3 have been characterized in detail in murine and human studies (including natural and synthetic antagonists), there is emerging evidence that MMPs could play a key role in the pathogenesis of CF lung disease. The amount of free proteolytic activity increases over the lifetime of CF patients [3] and overwhelms the antiprotease shield of the airspaces. These enzymes damage cellular and pulmonary structures, including cilia, elastin, fibronectin, surfactant proteins A and D, immunoglobulins, and CXCR1 on neutrophils and CD4 on lymphocytes [5]. In the following chapters, we will introduce the MMP family and will highlight the potential of several members as future targets in CF lung disease.
MMPs: Structure and function
MMPs are one of four subfamilies within the superfamily of metalloendopeptidases known as the metzincins [6]. There are > 20 MMPs that have been identified and, based on structure and substrate specificity, are divided into different groups. All MMPs exhibit an N-terminal signal sequence, a prodomain region, and active catalytic domain (with a zinc-binding region). Another major domain observed is a hemopexin-like C-terminal domain (found in all MMPs except -7 and -22), which is thought to mediate substrate specificity of the MMP for components of the ECM. Additional domains include a transmembrane domain (found in MMP-14, -15, -16, -24) and GPI anchor (MMP-17 and MMP-25), important to cell-bound MMPs. Furin-recognition motifs are seen in multiple MMP isoform’s prodomain region and type II fibronectin-repeats are seen in gelatinase (MMP-2 and MMP-9) catalytic domains. These regions seem to have important implications to the interaction of the MMP with its substrate (Reviewed in [7]).
MMPs have the combined ability to degrade essentially all connective tissue components. While MMPs are involved in many normal homeostatic mechanisms, they are commonly elevated in their expression and activities in conditions where inflammation and tissue remodeling/repair are operative [8, 9]. MMPs are regulated at various points. They are regulated at the level of transcription (through their induction by various cytokines such as IL-1 and TNF-alpha) and may involve the activation of a diverse group of intracellular signaling cascades (such as p38 MAPK or ERK 1/2 MAPK) leading to activation of nuclear signaling factors such as AP1, NF-kappa B, and STAT to induce MMP transcription [10]. Most MMPs are translated to a zymogen (inactive) form. These enzymes have a pro-domain region which is cleaved through a "cysteine switch" mechanism and is then activated. Activation can be induced by various triggers, including protease-mediated activation (either by another protease or auto-activation by the active form of that MMP), oxidant-mediated activation, or matrix-mediated activation. In addition to regulation of activation, there are numerous inhibitors of MMPs. Although tissue inhibitor of metalloproteases (TIMPs) is often thought of as the predominant group of inhibitors for MMPs, they are really the most specific endogenous MMP inhibitors. Rather, the majority of MMP-related inhibition in vivo occurs through relatively non-specific MMP inhibitors, such as alpha-2 macroglobulin [8, 11]. TIMPs are a group of four small (20-24 kDa) MMP-specific inhibitors which bind to MMPs in a 1:1 stoichiometric relationship [12-15]. Animal studies, in addition to human studies in adults, support a role for MMPs and an imbalance between MMPs and TIMPs in the pathogenesis of several well-recognized pulmonary disorders such as COPD [16, 17] and asthma [18-20].
MMPs perform numerous biologic functions, including degradation of matrix components and remodelling of tissues, release of cytokines, growth factors and chemokines, and modulation of cell mobility and migration [11]. Data suggests that dysregulated cellular production, secretion and activation of MMPs, and/or dysfunction of their inhibitors are involved in pathologic conditions within the lung parenchyma. Through degradation of extracellular matrix components, MMPs can destroy the alveolar epithelium, as well as disrupt reorganization during the repair process [8, 9]. Although previously well-studied in other pulmonary disorders, the role of MMPs in CF lung disease has only recently been emerging and suggests important downstream effects of this proteolytic dysregulation.
MMPs in cystic fibrosis lung disease
MMPs were first found to be upregulated in the airways of CF patients in 1995 when Delacourt et al described the increase of a 95/88 kDa gelatinase isoform (presumably MMP-9) in the sputum of patients with CF lung disease (n=27) compared to asthmatic controls (n=9) with an associated imbalance of TIMP [21]. Since this finding, there has been a burgeoning of MMP literature in CF lung disease, focusing not only on relative protease/antiprotease imbalance, but also increasing evidence for downstream effects of this proteolytic environment. These potential biological effects with regards to CF lung disease are shown in Figure 1.
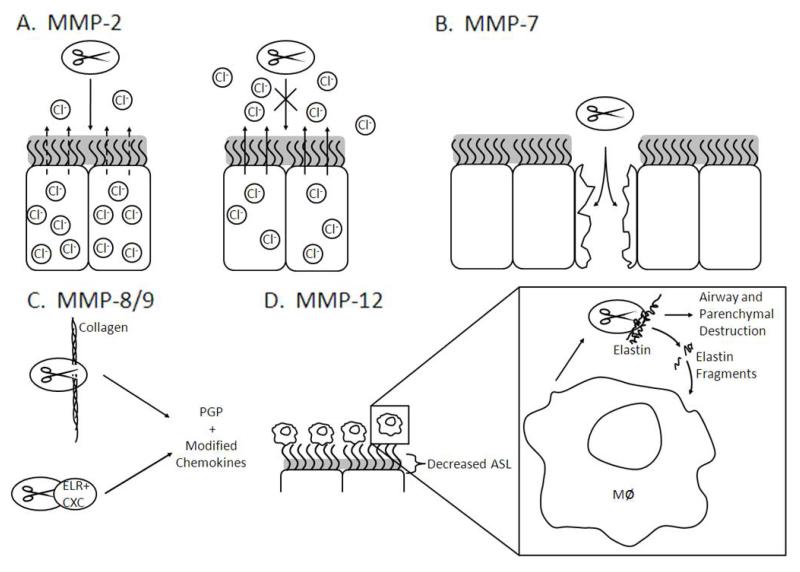
Predicted effects of MMPs (depicted as ovals with scissors) activities in the context of cystic fibrosis. A) MMP-2 disrupts chloride current, and inhibition of MMP-2 abrogates this effect. B) MMP-7 functions to repair damage in airway epithelia. C) MMP-8/9 are able to cleave collagen and modify ELR+ CXC chemokines which modulate inflammation in the airways. D) Decreased airway surface liquid (ASL) activates macrophages and increases expression of MMP-12, which cleaves elastin resulting in degradation of the airway and lung parenchyma. These elastin fragments may also increase the recruitment of monocytes and activation of macrophages.
MMP-2
MMP-2 (EC 3.4.24.24) is a protease with gelatinolytic activity (hence its alternate name, Gelatinase A), which is found to be constitutively expressed in various cell types found in the lungs. This enzyme has a broad spectrum of substrates and is involved in modulating diverse cellular functions including angiogenesis [22], tissue remodeling [9], and potentiation of inflammatory response [23]. MMP-2 is activated in a unique MT-MMP dependent manner, demonstrating a classic example of MMP-to-MMP activation [24]. MMP-2 is thought to contribute to the pathogenesis of a variety of pulmonary disorders including COPD, asthma, lung cancer, and interstitial pulmonary fibrosis [25].
MMP-2 has not been extensively studied in CF lung disease but there are some interesting insights into the potential role of this protease in CF. For example, one study has shown that inhibition of MMP-2 in Calu-3 cells (which constitutively express MMP-2) leads to augmentation of chloride transport in these cells, suggesting autocrine regulation of ion transport via this protease [26]. In addition, McElvaney and colleagues have recently demonstrated that NE may augment MMP-2 expression from epithelial cells, potentially leading to increased remodeling and inflammatory response in CF [27]. Interestingly, although MMP-2 elevations have not been consistently observed in pulmonary specimens from individuals with CF [28, 29], a recent manuscript has found that MMP-2 decreases in serum during CF exacerbation [30].
MMP-7
MMP-7 (EC 3.4.24.23), or matrilysin, is the smallest known MMP, but exhibits broad substrate specificity with the capacity to cleave multiple components of the matrix [31]. In addition, MMP-7 has been observed in playing a role in diverse biological responses such as airway re-epithelialization, inflammation [32], host defense [33], and cell growth via cleavage of IGF binding proteins [34]. MMP-7 has been shown to be involved in IPF, as knockout mice are protected from bleomycin-induced injury [35] and MMP-7 is overexpressed in lung tissues from patients with IPF [36].
MMP-7 may play an important role in the injury/repair response in CF lung disease. Dunsmore et al have shown increased MMP-7 expression in the airway epithelia of CF patients, and that it is differentially released either apically or basally from these cells. The authors hypothesize that this regulation is important in the injury response and show that human airways treated with an MMP-7 inhibitor fail to re-epithelialize after injury [37]. To our knowledge, specific studies of the importance of MMP-7 expression in CFTR knockout mice after injury have not yet been published.
MMP-8
MMP-8 (EC 3.4.24.34), or neutrophil collagenase, is one of 3 collagenase MMPs (MMP-1, -8, and -13), and is highly expressed in neutrophils. These collagenases have common cleavage sites for type I, II, and III collagens, producing a three-fourths N-terminal fragment and a one-fourth C-terminal fragment [38-40]. This suggests a potential redundancy for these proteases in disease. MMP-8 has also been show to cleave aggrecan in vitro [41], and may play a role in arthritis [42]. Two specific isoforms of pro-MMP-8 have been described in humans: one which is approximately 80 kDa and is highly expressed in PMNs, and a 55 kDa isoform which is expressed from mesenchymal cells [43]. Beyond the capacity to cleave extracellular matrix, MMP-8 has diverse biological effects including modulation of chemokines [44], regulation of repair response [45], and innate immunity [46]. Increased MMP-8 expression has been characterized in various lung diseases including COPD [47], IPF [43], bronchiectasis [48], and asthma [49].
MMP-8 expression and activity has also been found to be elevated in the airway secretions of patients with CF lung disease [50]. This enzyme is also elevated in the serum of patients with CF, and levels correlate with lung function (MMP-8 level vs. %FEV1, r=-0.468, p<0.001) [30]. Unfortunately, beyond these characterizations, there is little else known regarding the impact of this dysregulation on CF pathogenesis. Recently, a novel neutrophil chemokine (proline-glycine-proline) was found in the sputum of CF patients and MMP-8 was found to be an important enzyme involved in its generation [51]. Further examination of the impact of MMP-8 on progression of CF lung disease is certainly warranted.
MMP-9
Perhaps one of the most extensively-studied proteases, MMP-9 (EC 3.4.24.35), or gelatinase B, is broadly expressed in a variety of cells in the lung including inflammatory (PMNs, macrophages), epithelial, and endothelial cells. This observed redundancy belies important location-specific functions of MMP-9, some of which seem in opposition to other MMP-9 functions. For example, MMP-9 has observed important pro-inflammatory effects by generating PGP [51] and increasing chemokine potency of IL-8 [52], but MMP-9 also belies important role in the regulation of granuloma formation in tuberculosis [53]. Similarly, MMP-9 has been predominately thought to lead to matrix breakdown but recently it has been suggested that MMP-9 may have a role in matrix repair [54, 55].
MMP-9 has been extensively examined in the lower airway secretions of CF patients and has been found to be increased in both quantity and activity [28, 50]. One study found a correlation with lung function (FEV1) and MMP-9 with r value of −0.78 (p = 0.001) [56]. In addition, MMP-9 expression is also elevated in the serum of patients with CF lung disease [30].
MMP-12
MMP-12 (EC 3.4.24.65), also known as macrophage elastase, is a protease secreted by macrophages with the capacity to degrade elastin. MMP-12 was first cloned by Shapiro et al [57] and seems to have partial regulation by proteases such as thrombin and plasmin and can be released from macrophages by a variety of inflammatory cytokines such as TNF-a and IL-1b [58]. This protease has emerging biological effects in modulating cytokine and chemokine networks, including cleavage of pro-TNF-α [59] and ELR+ CXC chemokines [60]. The physiological role of MMP-12-mediated proteolysis remains poorly understood and may be related to remodelling of connective tissue during growth and development, and migration of macrophages into tissues. Interestingly, recent data demonstrated that MMP-12 has direct antimicrobial activity and plays an important role in macrophage-mediated killing of both gram-negative and gram-positive bacteria in the lung and other organs [61]. Increased proteolytic activity of MMP-12 has been shown to play an important role in the pathogenesis of emphysema induced by either cigarette smoke [17] or overexpression of the Th-2 cytokine IL-13 in mice [62].
A recent human study identified an SNP producing a functional variant in the MMP-12 promoter that is associated with lung function in asthmatic children and the risk of COPD in adult smokers, suggesting that MMP-12 plays an important role in the pathogenesis of asthma and COPD [63]. Recently, the expression of MMP-12 in the sputum of CF individuals has been demonstrated [28]. The role of increased MMP-12 activity in CF lung disease has not been studied. However, recent observations in transgenic mice with airway-specific overexpression of the amiloride-sensitive epithelial Na+ channel (βENaC-Tg mice) that exhibit ASL depletion and phenocopy CF lung disease [64] suggest that airway surface dehydration and mucostasis cause macrophage activation and MMP-12-dependent emphysema [65]. Further elucidation of the role of MMP-12 in the human CF lung should be facilitated by the recent development of FRET probes designed to assess MMP-12 activity on leukocytes and biological fluids [66]. Based on their high sensitivity (with a detection limit in the sub-nanomolar range for human MMP-12), these novel MMP-12 FRET sensors should be suitable to measure MMP-12 activity in clinical specimens such as BAL or sputum and determine its role in the pathogenesis and as a potential biomarker of CF and other chronic inflammatory lung diseases.
Outlook: Targeting MMPs to treat cystic fibrosis lung disease
As more information has been found regarding the presence and activity of MMPs in CF lung disease, there has been increased interest in modulating MMP activity to improve disease outcome. Evidence supporting the involvement of MMPs in CF lung disease is summarized in Table 1.
Table 1
IntEnz Number | Alternative Name | Molecular Weight | Location of Expression in Airways | Expression in Cystic Fibrosis | Predicted Role in Cystic Fibrosis | References | |
---|---|---|---|---|---|---|---|
MMP-2 | EC 3.4.24.24 | Gelatinase A | 72 kDa pro, 64 active | Structural cells (epithelia, endothelium) and macrophages | Detected in BAL fluid, not detected in sputum, decreased in serum during exacerbation | Regulation of ion transport, increasing remodeling and inflammatory response | [26], [27], [28], [29] |
MMP-7 | EC 3.4.24.23 | Matrilysin | 28 kDa pro, 19 active | Epithelial cells predominately | Elevated in airway epithelia | Possible role in injury/repair | [37] |
MMP-8 | EC 3.4.24.34 | Neutrophil Collagenase | 75 pro, 65 active | Neutrophils and epithelia | Elevated in serum and levels are negatively correlated with lung function, elevated in BAL fluid | Modulation of inflammatory response, airway remodeling, generation of bioactive collagen fragments | [30], [50], [51] |
MMP-9 | EC 3.4.24.35 | Gelatinase B | 92 pro, 82 active | Predominately neutrophils but also structural cells (epithelia, endothelium) and macrophages | Elevated in lower airway secretions and negatively correlated with lung function, elevated in serum | Modulation of inflammatory response , airway remodeling, generation of bioactive collagen fragments | [30], [51], [52], [56] |
MMP-12 | EC 3.4.24.65 | Macrophage Elastase | 54 pro, 45 active | Macrophages | Detected in sputum and serum of CF patients | Possible role in airway and parenchymal remodeling, possible role in generating bioactive elastin fragments | [28], [30], [65] |
MMP inhibition has been extensively studied as a potential therapeutic in cancer, and although there has been significant promise for these drugs, two previous phase III trials have shown no clinical benefit [67]. In addition, an MMP inhibitor (PG-116800) has been studied in post-myocardial infarction cardiac remodelling and has shown no benefit. To date, the clinical use of MMP inhibitors in the setting of inflammatory disorders such as osteoarthritis has been largely disappointing [68]. Unfortunately, many synthetic MMP inhibitors either have been limited from either off-target effects or inability to tolerate side effect profiles. Despite difficulties of translation in other conditions, interest has turned to the potential of MMP inhibition in lung disease such as CF. Various approaches have been entertained for inhibition of these activities and detailed below.
Tissue inhibitors of metalloproteases (TIMPs)
The tissue inhibitors of metalloproteases are naturally occurring inhibitors of MMPs in vivo. While TIMP binding blocks MMP activity, TIMPs themselves can be inactivated by proteases like NE by direct proteolytic degradation [69] or excessive MMP-12 activity [70]. Though this kind of TIMP/MMP dysregulation has been shown to contribute to the pathology of the disease and to correlate to disease severity in animal and human studies, there have not, to our knowledge, been attempts to replete TIMPs as a treatment for CF. Though TIMPs serve as fairly potent inhibitors of MMP activity, the increased quantities of TIMP required to overcome a pro-proteolytic environment would likely make TIMP therapy cost-prohibitive.
Antibiotics
As previously mentioned, the current standard of long term clinical care of patients with CF lung disease often involve 3 times per week dosing of a macrolide antibiotic [71]. While these antibiotics have multiple biological effects, a potent effect is the inhibition of MMP activities. Currently, tetracycline antibiotics have not been systematically examined for similar anti-protease or anti-inflammatory activities in CF. Previous data indicates that tetracyclines have intrinsic anti-inflammatory properties. Doxycycline, a member of the tetracycline family is able to reduce neutrophil chemotaxis [72] and nitric oxide production from murine lung epithelial cells [73]. More pertinently, doxycycline can also inhibit MMP synthesis from human endothelial cells [74]. As MMPs have been well regarded as targets for tetracycline antibiotics in disease [75], there is increasing interest for inhibiting MMPs by these agents. Recent ex vivo data suggests that this class of antibiotic may effectively reduce MMP activity in CF sputum [28]. A single centre randomized-controlled clinical trial is currently planned to examine these effects in an adult inpatient CF population in the United States (Clin Trials.gov #NCT01112059, PI Gaggar).
ACKNOWLEDGEMENTS
Supported by the NIH (HL102371-01A1 to AG), CF Foundation (GAGGAR07A0 to AG), German Research Foundation (DFG MA2081/3-3 and MA 2081/4-1 to M.A.M.) and EC (MEXT-CT-2004-013666 to M.A.M.)
REFERENCES
Full text links
Read article at publisher's site: https://doi.org/10.1183/09031936.00173210
Read article for free, from open access legal sources, via Unpaywall:
http://erj.ersjournals.com/content/38/3/721.full.pdf
Citations & impact
Impact metrics
Article citations
Saccharomyces cerevisiae β-glucan improves the response of trained macrophages to severe P. aeruginosa infections.
Inflamm Res, 73(8):1283-1297, 08 Jun 2024
Cited by: 0 articles | PMID: 38850343 | PMCID: PMC11282130
Advancements in preclinical human-relevant modeling of pulmonary vasculature on-chip.
Eur J Pharm Sci, 195:106709, 19 Jan 2024
Cited by: 1 article | PMID: 38246431
Review
Extracellular vesicles secreted by primary human bronchial epithelial cells reduce Pseudomonas aeruginosa burden and inflammation in cystic fibrosis mouse lung.
Am J Physiol Lung Cell Mol Physiol, 326(2):L164-L174, 12 Dec 2023
Cited by: 0 articles | PMID: 38084406 | PMCID: PMC11279747
Mechanisms by which the cystic fibrosis transmembrane conductance regulator may influence SARS-CoV-2 infection and COVID-19 disease severity.
FASEB J, 37(11):e23220, 01 Nov 2023
Cited by: 3 articles | PMID: 37801035 | PMCID: PMC10760435
Modulation of Plasmatic Matrix Metalloprotease 9: A Promising New Tool for Understanding the Variable Clinical Responses of Patients with Cystic Fibrosis to Cystic Fibrosis Transmembrane Conductance Regulator Modulators.
Int J Mol Sci, 24(17):13384, 29 Aug 2023
Cited by: 0 articles | PMID: 37686190 | PMCID: PMC10488059
Go to all (60) article citations
Data
Data behind the article
This data has been text mined from the article, or deposited into data resources.
BioStudies: supplemental material and supporting data
Clinical Trials
- (1 citation) ClinicalTrials.gov - NCT01112059
Similar Articles
To arrive at the top five similar articles we use a word-weighted algorithm to compare words from the Title and Abstract of each citation.
Inhibition of airway proteases in cystic fibrosis lung disease.
Eur Respir J, 32(3):783-795, 01 Sep 2008
Cited by: 67 articles | PMID: 18757703
Review
Proteases and cystic fibrosis.
Int J Biochem Cell Biol, 40(6-7):1238-1245, 14 Mar 2008
Cited by: 113 articles | PMID: 18395488 | PMCID: PMC2431113
Review Free full text in Europe PMC
Biochemical and pathologic evidence for proteolytic destruction of lung connective tissue in cystic fibrosis.
Am Rev Respir Dis, 132(3):529-535, 01 Sep 1985
Cited by: 118 articles | PMID: 3898942
The role of bacterial proteases in the pathogenesis of cystic fibrosis.
Am J Respir Crit Care Med, 150(6 pt 2):S118-22, 01 Dec 1994
Cited by: 36 articles | PMID: 7952646
Review
Funding
Funders who supported this work.
NHLBI NIH HHS (2)
Grant ID: R01 HL102371
Grant ID: HL102371-01A1