Abstract
Purpose
To clarify the role of bone morphogenetic proteins (BMP-2,-4,-5) in sclera remodeling during myopia induction and their effect on sclera fibroblasts in cell culture.Methods
Reverse transcription and polymerase chain reaction (RT-PCR) as well as immunofluorescence were used to detect the expression of the BMPs in human and guinea pig posterior sclera. In guinea pig form-deprivation myopia (FDM) model, RT-PCR and western blotting were used to investigate changes of BMP expression in the posterior sclera. Human sclera fibroblast (HSF) was primarlly cultured and treated with various doses of BMP-2. Cell proliferation was evaluated by the MTT assay. RT-PCR and western-blot were used to determine the changes of collagen I, aggrecan, and possible activated signal pathway. Cell phenotype and activated signal pathway, especially for α-smooth muscle actin (α-SMA) and phospho-smad1/5/8 were then further investigated by cytoimmunofluorescence staining.Results
Both human and guinea pig sclera express BMP-2, -4, and -5. In FDM eyes, BMP-2 and BMP-5 expression were reduced in the posterior sclera. Cell proliferation increased significantly (p<0.05) and more cells differentiated into myofibroblast when incubated with 100 ng/ml BMP-2 . The expressions of collangen I, aggrecan, and phospho-smad1/5/8 significantly increased (p<0.05 respectively) as well.Conclusions
Various BMPs were expressed in human and guinea pig sclera. In the posterior sclera, the expressions of BMP-2 and BMP-5 decreased in FDM eyes. BMP-2 might be able to promote HSF proliferation and differentiation, as well as to help extracellular matrix synthesis potentially through classical Smad pathway.Free full text

Role of bone morphogenetic proteins in form-deprivation myopia sclera
Abstract
Purpose
To clarify the role of bone morphogenetic proteins (BMP-2,-4,-5) in sclera remodeling during myopia induction and their effect on sclera fibroblasts in cell culture.
Methods
Reverse transcription and polymerase chain reaction (RT–PCR) as well as immunofluorescence were used to detect the expression of the BMPs in human and guinea pig posterior sclera. In guinea pig form-deprivation myopia (FDM) model, RT–PCR and western blotting were used to investigate changes of BMP expression in the posterior sclera. Human sclera fibroblast (HSF) was primarlly cultured and treated with various doses of BMP-2. Cell proliferation was evaluated by the MTT assay. RT–PCR and western-blot were used to determine the changes of collagen I, aggrecan, and possible activated signal pathway. Cell phenotype and activated signal pathway, especially for α-smooth muscle actin (α-SMA) and phospho-smad1/5/8 were then further investigated by cytoimmunofluorescence staining.
Results
Both human and guinea pig sclera express BMP-2, −4, and −5. In FDM eyes, BMP-2 and BMP-5 expression were reduced in the posterior sclera. Cell proliferation increased significantly (p<0.05) and more cells differentiated into myofibroblast when incubated with 100 ng/ml BMP-2 . The expressions of collangen I, aggrecan, and phospho-smad1/5/8 significantly increased (p<0.05 respectively) as well.
Conclusions
Various BMPs were expressed in human and guinea pig sclera. In the posterior sclera, the expressions of BMP-2 and BMP-5 decreased in FDM eyes. BMP-2 might be able to promote HSF proliferation and differentiation, as well as to help extracellular matrix synthesis potentially through classical Smad pathway.
Introduction
Myopia is the most common visual disorder which affects approximately half of the world's young adult population [1-4]. The prevalence of myopia and the degree of severity is rapidly increasing, especially in some Asian communities [5]. Despite years of intensive research, the precise mechanisms which control ocular growth and development of refractive errors are still not well known. Postnatal scleral growth, like other connective tissues, is under the control of growth hormone or its downstream effectors, and there is a net loss of matrix in myopic sclera [6,7]. The role of transforming growth factor beta (TGF-β) in myopia was well investigated [8-12] during the past fifteen years. Bone morphogenetic protein (BMP), which belongs to transforming growth factor-beta superfamily, might play an important role in myopia development. BMPs were found in several ocular tissues and play an important role in eye development and differentiation [13]. Their other cellular functions, such as morphogenesis, cell proliferation, apoptosis, extracellular matrix synthesis etc., were reported [13] as well. However, to our best knowledge, the role of BMPs in homeostasis of the sclera has not been fully documented. The relationship between BMPs and axial elongation in myopia also remains obscure.
It is well known that sclera remodeling occurs during axial elongation in myopia [14,15] and scleral fibroblasts are the key cells in this process. The thinned posterior scleral in high myopia is associated with a general loss of type I collagen and aggrecan which accounting for most of scleral extra cellular matrix (ECM) [6,16]. Studies suggested that scleral cells comprised a subset of myofibroblasts [12,17]. One study showed that cultured with TGF-β brings about a rapid differentiation of sclera fibroblasts into α-smooth muscle actin (α-SMA)-expressing myofibroblasts [12].
In this study, we aim to clarify the role of BMPs (BMP-2, −4, and −5) by examining any changes during myopia induction in guinea pigs sclera, and in vitro the effect of BMPs on sclera fibroblasts differentiation, ECM, and its potential signal pathway.
Methods
Animals
All experiments undertaken conformed to the ARVO Statement for the Use of Animals in Ophthalmic and Vision Research and the United States NIH document “Guide for the Care and Use of Laboratory Animals 1996” were followed. Twenty pigmented guinea pigs (approximately 10~15 days old) were reared as previously reported [18]. Animals were housed in groups in a temperature controlled room (25 °C) in open topped cages, on a 12 h light (provided by ceiling fluorescent tubes 36W) and 12 h dark cycle, with free access to food (guinea pig pellets and fresh vegetables) and water.
Experimental design and myopia induction
The animals were randomly assigned to 2 groups: diffusers (n=10) and normal controls (n=10). Diffuser-wear techniques employed in our experiments are similar to McFadden’s techniques [18]. The diffusers (white translucent hemispheric occluder with a diameter of 12 mm) were mounted on a matching plastic ring and glued to the periorbital fur of the eye for the whole two-week period of this experiment. The deprived eye in diffusers was randomly selected among animals and the fellow eye was untreated as a contralateral control.
Biometric measurements
Biometric measurements include streak retinoscopy and ultrasonography. Retinoscopy was performed in a dark room by using a streak retinoscope and trial lenses when the eyes had been fully cyclopleged with 2 drops of tropicamide for approximately 15 min. The refraction was recorded as the mean value of the horizontal and vertical meridians. Axial length of the eye was measured under topical anesthesia by an A-scan ultrasonagraph with 11 MHz which had been used by Lu et al. [19]. Ocular refraction and axial length were collected at the beginning and end of the experiment.
Tissue preparation
Human scleral tissues were excised from healthy adult human donor eyes (n=3; ages of 18, 19, and 25 years) obtained from the Eye Bank of the Affiliated Hospital of Medical College, Qingdao University, with the approval of Ethics Committee of Qingdao University (Qingdao, China) and complied with the tenets of the Declaration of Helsinki for biomedical research involving human subjects. Then each sclera was cut into equal halves along the optic disc and macula. The head of the optic nerve was discarded. One half was embedded with Optimum Cutting Temperature Compound (OTC; Sigma, St. Louis, MO), and cut into 8 μm sections at −20 °C. The other half was stored at −80 °C and used for RT–PCR. Sections were subsequently tiled onto slides (Corning Ltd, Tokyo, Japan), fixed with cool acetone for 15 min, air-dried, and kept frozen at −20 °C until use. Normal (n=10) and form deprived (n=10) guinea pigs were killed by overdoses of Chloral Hydrate. The eyeballs were removed and cut into halves about 1 mm posterior to the ora serrata on the ice plate. The anterior segment of the eye was discarded. The posterior sclera was excised by using a 6 mm-diameter trephine around the head of the optic nerve. The head of the optic nerve was discarded. The left sclera was flapped and stored at −80 °C.
Human scleral cell isolation and culturing
Human scleral tissues were excised from surgical specimens collected during treatment for retinoblastoma (n=2, ages of 1.5 and 2 years) under signed informed consent obtained from donors and was also approved by the Ethics Committee of Qingdao University (Qingdao, China) and complied with the Tenets of the Declaration of Helsinki for biomedical research involving human subjects. The scleral tissue was cut into small pieces (about 1 mm3) and cultured in 25 mm2 flasks in Dulbecco’s modified Eagle’s medium (DMEM; Invitrogen, Carlsbad, CA)/Nutrient mixture F12 (1:1) with high glucose supplemented, 10% fetal bovine serum (FBS; Gibco) and incubated at 37 °C in a humidified incubator containing 5% CO2. When achieve a heavy primary monolayer, the cells were trypsinized for 3 min at room temperature in 0.25% trypsin/EDTA solution in phosphate buffered saline (PBS, Gibco) and subcultured at a split ratio of 1:3 in a 25 mm2 flask (Corning Ltd., Lowell, MA). The third passage of fibroblasts was used for this experiment.
Cell proliferation assays
Cell proliferation was measured by 3-(4, 5-dimethylthiazol-2-yl) −2,5- diphenyltetrazolium bromide (MTT, Gibco) assay. Cells were plated in 96 well plates (Corning Ltd) with DMEM in 10% FBS at a 4×103/cm2 density. Before treated with BMP-2, cells were synchronized by replacing the medium with serum-free medium for 24 h. Then cells were incubated with different concentrations (1 to 100 ng/ml) of BMP-2 at 37 °C for 1 to 6 successive days. This dose range of BMP-2 was commonly used in culture for in vitro studies although the normal physiologic levels are lower in bone at 1 to 2 ng/g. The medium was semi-replaced daily. On the seventh day, cells were washed twice with 10 mmol/l PBS (pH 7.2) and incubated with 0.5 mg/ml MTT at 10 μl/well for the last 4 h before the reaction was terminated with the addition of 150 μl dimethyl sulfoxide (DMSO, Sigma). The absorbance was determined at 490 nm using an enzyme linked immunosorbent assay (ELISA) reader (BIO-TEK Instruments, Winooski, VT).
Total RNA isolation, reverse transcription and polymerase chain reaction (RT–PCR)
Human sclera cells were seeded in 6-well culture plates at 4×105 cells/well and cultured for 24 h. After synchronized with serum-free DMEM for another 24 h, cells were incubated in DMEM containing BMP-2 (100 ng/ml). The culture without BMP-2 acted as controls. On day 2, the culture media was changed with the same concentration of BMP-2 in each well. After 72 h incubation, cells were harvested for RNA extraction. Sclera was grinded in liquid nitrogen. Total RNA were extracted from sclera (normal human sclera n=3;normal guinea pigs sclera n=3; form deprived guinea pig sclera n=5; contralateral control sclera n=5) and cells with TRIzol reagent (TaKaRa, Dalian, China) and contaminating DNA was digested using DNase I (Promega, Madison, WI) according to the manufacturer's protocol, and quantified using ultraviolet spectrophotometry by measuring OD260 and OD280 (optical density 260/280 higher than 1.9). cDNA was synthesized with 5 µg total RNA, 2 µl random 6 mers, 0.5 µl Oligo dT Primer, 2 µl PrimeScriptTM Buffer, and 0.5µl PrimeScriptTM RT Enzyme Mix I (TaKaRa) at 37 °C for 15 min. The reaction was suspended at 85 °C for 5 s. Conditions for PCR were 95 °C for 30 s, 35 cycles of 95 °C for 5 s, 55 °C (56 °C for BMP-3, BMPR-lB, and Smad1, respectively) for 30 s, and 72 °C for 30 s. The final extension step was at 72 °C for 7 min. The PCR products were then electrophoresed on a 2% agarose gel containing 10× Gelred reagents in parallel with 50 bp DNA markers. The nucleotide sequences of the primers used in the experiments are denoted in Table 1. β-actin (Actb) was used as an internal control. All band intensities were evaluated by densitometry (Gel-Pro Analyzer 4.5; Gel-Pro, Bethesda, MD). The ratio of a target gene versus Actb was computed as a series of numbers where there might be the smallest number. To make the expression trend clear, the value in the figures was the ratio of the series of numbers versus the smallest one. Because guinea pigs nucleotide sequences haven’t been reported, we designed probes to cover regions where the human and rat BMP sequences show high homology. It is therefore reasonable to expect that our primers specifically recognize the examined BMPs. To prove the identity, all the experiments were performed at least 3 times and the products were sequenced by TaKaRa.
Table 1
Gene | Upstream primer | Downstream primer | Tm (°C) | Size (bp) | NCBI accession number (rat and human) |
---|---|---|---|---|---|
BMP-2 | GCGGAAACGCCTTAAGTCCA | GTGGAGTTCAGATGATCAGC | 55 | 156 | NM_017178.1 , NM_001200.2 |
BMP-3 | TTGGCTGGAGCGAATGGATTA | GCTCAGGAATCCCAGAGACGAC | 56 | 159 | NM_017105.1 , NM_001201.2 |
BMP-4 | TTTGTTCAAGATTGGCTCCCAAG | AAACGACCATCAGCATTCGGTTA | 55 | 101 | NM_012827.2 , BC020546.2 |
BMP-5 | TCCACAGAACAATTTGGGCTTACA | ACCATGAACGGCTGCTTTGAC | 55 | 120 | NM_001108168.1 , NM_021073.2 |
BMP-6 | CGCCTTCCTCAACGACCGCGG | GGAATCTGGGATAAGTTGAA | 55 | 120 | NM_013107.1 , NM_001718.4 |
BMP-7 | TCCGGTTTGATCTTTCCAAGA | CCCGGATGTAGTCCTTATAGATCCT | 55 | 81 | NM_001191856.1 , NM_001719.2 |
BMPR-IA | GCCGTTTTGAAGCTGATGTCA | TCTTTGCGAGCGTCTTCTTGA | 55 | 88 | S75359.1 , NM_004329.2 |
BMPR-IB | TGGCTGACATGTACAGCTTTGG | GGCACTCGTCACTGCTCCAT | 56 | 198 | NM_001024259.1 , D89675.1 |
BMPR-ll | ACTGCAGATGGACGCATGG | AATCTCGATGGGAAATTGCAG | 55 | 199 | NM_080407.1 , NM_001204.6 |
Smad1 | CTCCAATGTTAACCGGAACTCCAC | CTCTGCACGAAGATGCTGCTG | 56 | 129 | NM_013130.2 , U59423.1 |
Collagen I | TGCTGGCAAGAATGGCGATC | CTGTCTCAGCCTTGTCACCAC | 55 | 122 | NM_000088.3 , AF169346.1 |
aggrecan | GAAGTGATGCATGGCATTGAGG | ATGATGGCGCTGTTCTGAAGG | 55 | 146 | NM_022190.1 , BC036445.1 |
β-actin | GGCACCACACTTTCTACAATG | GGGGTGTTGAAGGTCTCAAAC | 55 | 133 | NM_031144.2 , NM_001101.3 |
Western blot analysis
Human sclera cells were treated as described in RNA extraction. After incubated with 100 ng/ml BMP-2 for 72 h, cells were harvested for protein extraction. Form deprived guinea pig sclera (n=5) and contralateral control sclera (n=5) were grinded in liquid nitrogen. Cells and sclera tissues were homogenized separately in ice-cold extraction buffer (0.01 M Tris-HCl at pH 7.4, 0.15 M NaCl, 1% w/v Triton X-100, 0.1% SDS, 1% deoxycholic acid, 1mM EDTA) as well as protease inhibitors (1μM pepstatin, 1μg/ml leupeptin, and 0.2mM PMSF). After homogenization, samples were placed on ice for 30 min and centrifuged at 4 °C 12,000× g for 15 min. The supernatant was decanted and the precipitate was discarded. Protein concentrations were determined according to the BCA method using an Enhanced BCA Protein Assay Kit (Boster, Wuhan, China). Each protein sample (30 μg) was mixed with 5× sample buffer for SDS PAGE. The mixture was boiled for three min, electrophoresed on a 10% SDS polyacrylamide gel, and transferred to nitrocellulose membranes (Pall Corporation, East Hills, NY). Protein loading and transfer efficiency were monitored by staining the membranes with 1% Ponceau S. The membranes were washed three times with TBST (pH 7.6) and soaked in a blocking solution (5% w/v skim milk powder in 2.5 mM Tris-HCl and 14 mM NaCl plus 0.05% Tween-20) for 1 h at room temperature. The membranes were incubated overnight with primary antibodies at a 1:100 dilution (rabbit anti BMP-2, −4, and −5; 0.2ml; Boster, Wuhan, China) and 1:500 dilution (rabbit anti phospho-Smad1/5/8; 0.1 ml; , Millipore, Billerica, MA) at 4 °C in blocking solution. The membranes were then washed three times with TBST and incubated with a horseradish peroxidase-conjugated secondary antibody (goat anti-rabbit) at a 1:1,000 dilution (0.4 μg/ml; Boster) for another 1 h at room temperature. The membranes were again washed three times with TBST. The reaction products were visualized with BeyoECL Plus western blotting detection reagents (Beyotime Institute of Biotechnology, Haimen, China). Images were captured with a Fuji Film LAS3000 imaging system and analyzed with MultiGauge software (Fuji Film, Tokyo, Japan). β-actin (Kang Chen, China) was used as a housekeeping protein to normalize the protein load.
Immunofluorescent staining
The slides were washed three times with PBS, nonspecific bindings were blocked by incubation in 10% (v/v) goat serum in PBS for 30 min at room temperature. Then the slides were incubated at 4 °C overnight with primary antibodies against BMP-2, −4, and-5 (BA0585; BA0662; BA0663; 1:200 dilution; Boster) diluted in PBS. The antibody-treated and negative control slides were washed with PBS three times at room temperature, then incubated with FITC labeled goat anti-rabbit IgG antibodies (BA1105; Boster) diluted in PBS at room temperature for 1 h. Fluorescent signals were detected using a fluorescent microscope (TS-100; Nikon, Tokyo, Japan).
Cells grown on 96-well plates were fixed with 4% (v/v) paraformaldehyde for 30 min and then made permeable with 0.1% Triton X-100 in PBS for 10 min. Nonspecific binding was blocked by incubation in 10% (v/v) goat serum in PBS for 30 min at room temperature. Following incubation of the cells with primary antibody against phospho-Smad1/5/8 (AB3848; Millipore), α-SMA (BM0002; Boster) diluted in PBS at 4 °C overnight. Cells were then probed with FITC-labeled (BA1105; Boster) or Cy3-labeled goat anti-rabbit (BA1032; Boster) secondary antibodies diluted in PBS and incubated at the room temperature for another 1 h. Nuclear staining with DAPI was applied. Fluorescent signals were detected using a fluorescent microscope (TS-100; Nikon).
Statistical analysis
Data were expressed as mean±standard deviation (SD). Differences between the groups were compared by using a one-way ANOVA (ANOVA) with a Tukey post hoc test or Bonferroni Correction. The Student’s t-test was performed for statistical analysis of cytometry between the experiment and control groups. Statistical analysis was performed using the SPSS 15.0 statistical software (IBM, Chicago, IL). The results were considered to be statistically significant at p<0.05.
Results
Expression of BMPs and BMP receptors in human and guinea pig sclera
In normal human and guinea pig sclera, BMP-2, −4, −5, and all the BMPRs could be detected after RT–PCR (Figure 1A). The relative expression level of BMP-5 was strongest, followed by BMP-2 and BMP-4. The expression of BMPR-IA and BMPR-IB were higher than that of BMPR-II. The expression of BMPs in human sclera were further confirmed by immunofluorescence (Figure 1B).
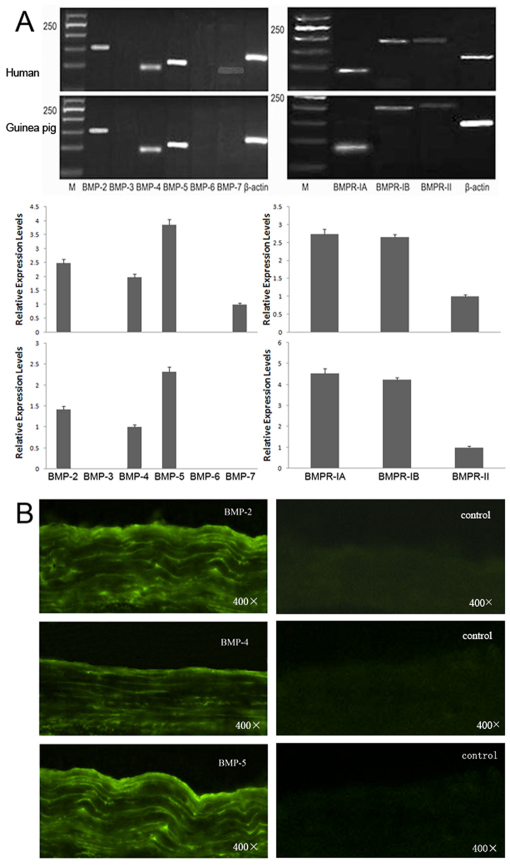
Expressions of BMPs and BMP receptors in human and guinea pig sclera. A: Semi-quantitative RT–PCR analysis of total RNA from 3 normal human sclera and 3 normal guinea pig sclera using specific primers for BMP-2 to BMP-7, BMPR-IA, BMPR-IB, and BMPR-II normalized to Actb at 35 cycles (M: molecular size marker). B: Distribution of the BMPs in human sclera by indirect immunofluorescence (FITC marked the secondary antibody).
The expression of BMPs in guinea pig sclera of form-deprivation myopia model
The differences between the two eyes of each animals in axial length were not significant for the two groups at the beginning (p=0.794, one-way ANOVA with Bonferroni correction, Figure 2). At the 14th day, monocularly deprived eyes had myopia of −0.48±0.51 D and an axial length of 8.29±0.05 mm which was significantly more myopic and longer than either the contralateral control eyes (+3.22±0.34 D; t=-12.814,df=18, p<0.001; 8.05±0.06 mm, t=7.23,df=18 p<0.001) or age-matched normal eyes (+3.07±0.54 D, t=-11.878, df=18, p<0.001; 8.06±0.06 mm, t=9.084, df=18, p<0.001, independence samples test, Figure 2).
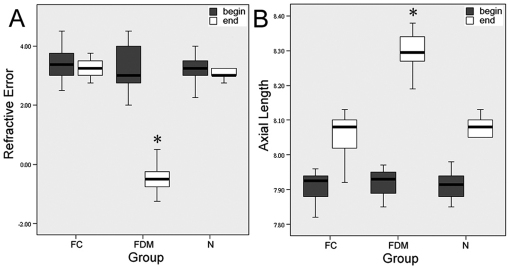
Differences in refractive error and axial length after induction of myopia. Differences in refractive error (A) and axial length (B) among form-deprived myopic (FDM), contralateral control (FC) and normal control(N) eyes of guinea pigs were illustrated after two weeks. The asterisk denotes a p<0.001 versus FC and N eyes.
The relative expression levers of FDM eyes were significantly lower than contralateral control eyes for BMP-2 (p=0.017) and BMP-5 (p=0.028). However, the expression of BMP-4 was not significantly altered in FDM eyes (p=0.162; Figure 3A). The identity of PCR products was confirmed by sequence analysis of vector-cloned cDNA fragments. Negative control experiments without template did not yield any products. The protein expressions of BMP-2, −4 and −5 could be detected by western blot (bands at about 13 kDa and 15 kDa, Figure 3B). After 14 days of visual deprivation, significant decrease was shown for BMP-2 (−32.65%, p=0.026) and BMP-5 (−25.11%, p=0.034) compared with the contralateral control eyes of the same animals, while no significant changes shown for BMP-4 (p=0.158; Figure 3B).
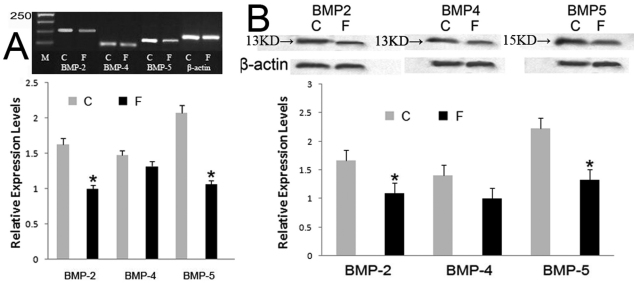
Effect of myopia induction on the expressions of BMPs in guinea pigs sclera. A: Significant decreases in mRNA expression were found in BMP-2 and BMP-5 compared with the internal control eyes. C: contralateral control, F: form-deprivation myopia. B: western blotting also showed a significant decrease for BMP-2 and BMP-5 but not for BMP-4. Bar graph shows changes in mRNA and protein expressions of BMP subtypes in the posterior sclera during form-deprived myopia in guinea pigs. Values (mean±standard error of the mean) were expressed as relative expression levels. The asterisk denotes a p<0.05.
The effect of BMP-2 on HSF in vitro
The scleral cells began growing out from pieces of sclera tissue after almost 10 days in culture. They exhibited a fibroblast-like spindle shape or polygonal shape in morphology for scleral cells, which grew in a vortex pattern cultured in monolayer. After exposed with various concentrations of BMP-2 (0, 1, 10, and 100 ng/ml) for one to seven days, cells proliferation was significantly higher on day 6 with 100 ng/ml BMP-2 (Figure 4A) than other concentrations, and the shape of cells was polygonal (Figure 4B,C). And there was a rapid differentiation of fibroblasts into α-SMA-expressing myofibroblasts with the addition of 100 ng/ml BMP-2 in cell cultures (Figure 4D,E).
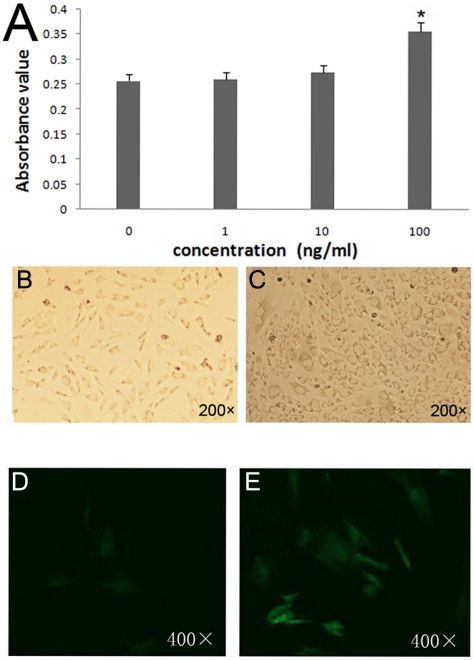
The effect of BMP-2 on HSF in vitro. A: MTT Assay of the effect of BMP-2 on HSF proliferation at different concentration on 6th day. Absorbance value at a wavelength of 490 nm. HSF proliferation in the presence of BMP-2 (100 ng/ml) was significantly higher on day 6 than BMP-2 with other concentrations or controls (p<0.05, n=6). The asterisk *stands for p<0.05. The morphology of primary cultured human scleral cells (B) the cells not exposed with BMP-2; (C) the cells cultured with 100 ng/ml BMP-2 on day 6. Cultured scleral fibroblasts were incubated without (D) or with (E) BMP-2(100ng/ml) for 6 days. The expression of the myofibroblast-marker, α-SMA was assessed using fluorescent immunocytochemistry (FITC-labeled the second anitibody).
As shown in Figure 5A,B, the expressions of aggrecan and collagen l as well as their protein expression levels were significantly increased when being cultured with 100 ng/ml BMP-2 for 72 h. The time point was selected according to Hu et al. [20] and Yoon et al. [21].
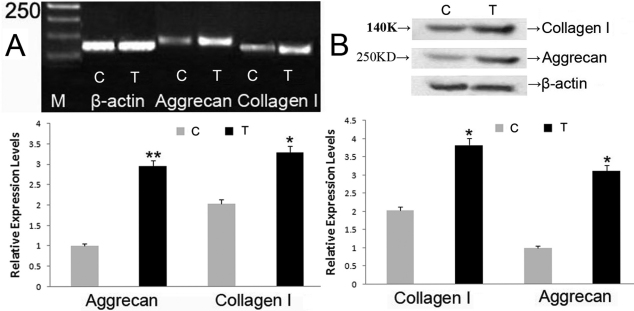
Effect of BMP-2 on production of collagen I and aggrecan. The mRNA (A) and protein (B) expression levels for collagen I and aggrecan were significantly increased with 100 ng/ml BMP-2, 72 h in vitro. Bar graphs revealed changes in mRNA and protein expressions (mean±standard error of the mean) where values were stated as relative expression levels. C: control group, T: BMP-2 treatment group. The asterisk stands for p<0.05 and the double asterisk stands for p<0.01.
Potential signal pathway of BMP effects in vitro
The expression of BMPR-IA, -IB, -II, Samd1, and BMP-2 significantly increased in vitro (Figure 6A) after cells were exposed with100 ng/ml BMP-2 for 24 h. The expression level of BMPR-IA and BMPR-IB were higher than BMPR-II.
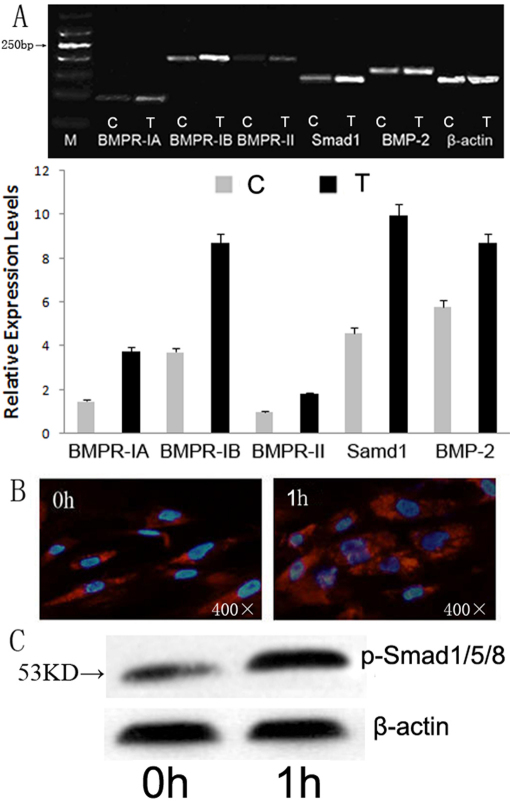
Potential effect of BMP-2 on the signal pathway in vitro. A: The mRNA expression in BMPRs, smad1, and BMP-2 after 24 h of stimulation with 100 ng/ml BMP-2. B: Immunofluorescent staining of Smad1/5/8 phosphorylation located in the cells treated by 100 ng/ml BMP-2 for 1 h.The second antibody is Cy3-labeled goat anti-rabbit (Boster) and nuclear was stained with DAPI. C: The protein level of phospho-Smad1/5/8 in the treated cells detected by western blot.
To confirm the effect of BMP-2 on signal pathway in vitro, cells were stained with immunofluorescence labeled Smad1/5/8 and detected by western blotting also. After incubated with100 ng/ml BMP-2 for 1 h, it showed more marked fluorescent signals localized in the nucleus of the treated cells than untreated cells (Figure 6B). Western blots also showed a higher phospho-Smad1/5/8 protein level in the treated cells (Figure 6C).
Discussion
In this study, the expression of selected BMPs were investigated in human and guinea pig sclera as well as in form-deprivation myopia sclera of guinea pigs. To our best knowledge, this is the first report about the expression and functional role of BMPs in guinea pigs sclera, and particularly for a form-deprivation myopia model.
The present study showed that both human and guinea pig sclera expressed BMP-2, −4, and −5. The additional expression of BMP receptors (BMPR-IA, -IB, and -II) in sclera indicates that BMPs play a role in normal sclera homeostasis. In the following study, there is a significant downward regulation of BMP-2 and −5 in the scleral remodeling during myopia induction, especially BMP-2. BMP-2 is expressed in the human cornea and is considered to be a heparin-binding cytokine that can modulate corneal fibroblast apoptosis [22]. It was also found to promote sclera fibroblast proliferation in vitro [20]. McGlinn et al. [23] reported that chicks following 6 h and 3 days of diffuser-wear, BMP-2 mRNA levels were significantly down-regulated in the retina and RPE. These research works show that there could be an additional role for BMP-2 in eye tissue. In a study [24]of possible mechanisms of scleral remodeling in the development of myopia found that there were mechanical stresses induced BMP-2 mRNA expression in human scleral fibroblasts after 30 min and 24 h. Another study [25] also reported that variations in the BMP2K gene are strongly correlated with high myopia in the Taiwanese population. In our study, BMP-2 was decreased in posterior sclera of myopia eyes, which would further indicate the BMP-2 is involved in the growth of human sclera.
It is reported that BMP-5 is also expressed in adult cornea [26]. BMP-5 expression increases during chondrocyte differentiation both in vivo and in vitro and this could promote proliferation and cartilage matrix synthesis through smad1/5/8 and p38 MAP kinase pathway [27]. In synovial tissue of patients with osteoarthritis and rheumatoid arthritis, the expression of BMP-5 was decreased [28]. In our study, there is a very high expression of BMP-5 in normal guinea pigs sclera and a significantly decreased expression in FDM. The decreased expression of BMP-5 indicates that BMP-5 was involved in sclera remodeling during myopia induction.
Other studies found BMP-4 could block the induction of fibronectin by TGF-β2 in TM cells [29]. In primary open angle glaucoma, elevated BMP antagonist expressed by TM cells inhibits BMP-4 antagonism of TGF-β2 and this inhibition leads to increased ECM deposition and elevated IOP [13]. In humans, mutations in BMP-4 [30] and BMP-7 [31] have been reported to be associated with eye and brain developmental anomalies, including the development of myopia in specific families. However, in our study, it did not show significant changes in BMP-4 expression during myopia induction. Further research should be focused on the relationship of BMP-4 and its antagonist with the effect of TGF-β in sclera ECM remodeling.
It was reported that HSF proliferation and scleral extracellular matrix remodeling events result in changes in biomechanical properties of the sclera and subsequent changes in axial length [24,32,33]. FDM significantly decreased total cell numbers in the region between the optic nerve and 10 degrees nasal (equivalent to myopic crescent location in humans) compared with control or normal eyes [34]. In our study, we used BMP-2 to clarify the effect of BMPs on HSF differentiation, extracellular matrix synthesis and its potential signal pathway in human sclera cells in vitro. It showed that BMP-2 significantly promoted HSF cell proliferation. Cell morphology and organization were also changed with the increasing concentration of BMP-2. The highest concentration of BMP-2 we had conducted is 100 ng/ml, for it is the most concentration used in vitro. For cell aggregation and polygonal could related to rapidly increasing cell differentiation and expression of more adhesion molecules. The increasing expression of α-SMA suggested that several HSF had differentiated into myofibroblasts. Myofibroblasts are generally defined as highly contractile cells that express smooth muscle protein, α-SMA [35] and comprise as a subset of scleral cells. One study suggested an age-dependent increase in the proportion of myofibroblasts [14,36]. Exposure of sclera cells to the reduced levels of TGF-β which was found in form-deprivation myopia sclera will decrease cell-mediated contraction and reduce α-SMA expression [12]. Myofibroblasts are capable of modifying their extracellular environment both through contraction and production of new extracellular matrix. Our result of increased myofibroblasts indicates that there are increased cell-mediated contractions with BMP-2.
Type I collagens constitute the majority of the collagens (approximately 99%) in human sclera and aggrecan is a major component of sclera proteoglycans [6]. In myopia, the posterior sclera remodeling was characterized by physical loss of the scleral extracellular matrix which were mainly due to the reduced production and increased degradation of type I collagen and aggrecan [6,37]. Previous studies had shown an increased expression of tissue inhibitor of matrix metalloproteinase (TIMP-2) and a decreased expression of MMP-2 in HSF after incubation with BMP-2 [20]. It implies that BMP-2 could decrease the degradation of the extracellular matrix of sclera. In this study, we observed significantly increased expression of aggrecan and collagen when cells incubated with 100 ng/ml BMP-2. This is different from previous studies which BMP-2 could not change mRNA of Type I collagen at any doses in osteoinductive cells such as intervertebral disc cells or chondrocyte [38,39]. Our results further suggested that there was an increased extracellular matrix production with 100 ng/ml BMP-2, which were similar to the results of Seko et al. [40].
BMPs exert their biologic effects through binding to the cell-surface serine-threonine kinase receptors BMP-RI and BMP-RII. This activation leads to phosphorylation of intracellular signaling molecules including Smad1, Smad5, and Smad8 [41,42]. Smad1/5/8 are receptor-activated Smads (R-Smads) which were phosphorylated by BMP type I receptors. After phosphorylation, R-Smads are released rapidly from the Type-I receptor to interact with Co-Smads (Smad4) and form hetero-oligomeric complexes which then translocate into the nucleus to regulate the transcription of various target genes [43]. In our study, BMPRs were known to be functional because Smad1/5/8 phosphorylation was observed following addition of BMP-2 (100 ng/ml).
A increased expression of mRNA for Smad1 and protein levels of phospho-Smad1/5/8 indicate a classic Smad signal pathway might be activated in HSF with BMP-2 stimulation. To further prove it, more experiments should be done as other proteins may be involved in the effects of activated BMP receptors on cell proliferation or matrix synthesis.
During myopia development, the biomechanical properties of the sclera are altered. Although scleral matrix remodelling was considered to be the sole determinant of this kind of change, but the importance of scleral cells, particularly scleral myofibroblasts should not be overlooked [7]. Our data suggest that BMP-2 could influence fibroblast proliferation and differentiation as well as extracellular matrix synthesis, which contributes to the development of human myopia. Future research is needed to investigate how BMP-2 controls ocular growth in animal models and influences on the remodeling of the sclera.
In conclusion, BMP-2, -4, and -5 are expressed in human and normal guinea pigs sclera. For myopia induction, the changes of their expression were found in posterior sclera of guinea pig. It suggests that BMPs might play an important role in sclera homeostasis and be potential candidates for myopia control.
Acknowledgments
The authors thank Xiangtian Zhou for his technical assistance in animal model. This study was supported in part by grants (BS2010SF009) from Shandong Provincial Education Department and the Affiliated Hospital of Medical College, Qingdao University. No authors have any commercial interest in the subject of the manuscript or in entities discussed in the manuscript.
References
Articles from Molecular Vision are provided here courtesy of Emory University and the Zhongshan Ophthalmic Center, Sun Yat-sen University, P.R. China
Citations & impact
Impact metrics
Citations of article over time
Article citations
Crosstalk between Myopia and Inflammation: A Mini Review.
Int J Med Sci, 21(9):1589-1603, 03 Jun 2024
Cited by: 0 articles | PMID: 39006849
Review
Gene Expression Signatures of Contact Lens-Induced Myopia in Guinea Pig Retinal Pigment Epithelium.
Invest Ophthalmol Vis Sci, 63(9):25, 01 Aug 2022
Cited by: 4 articles | PMID: 36006019 | PMCID: PMC9424971
Culture and identification of multipotent stem cells in guinea pig sclera.
Int Ophthalmol, 43(1):113-120, 06 Jul 2022
Cited by: 0 articles | PMID: 35794406
Scleral remodeling in myopia development.
Int J Ophthalmol, 15(3):510-514, 18 Mar 2022
Cited by: 14 articles | PMID: 35310066 | PMCID: PMC8907044
Review Free full text in Europe PMC
Advances in myopia research anatomical findings in highly myopic eyes.
Eye Vis (Lond), 7:45, 02 Sep 2020
Cited by: 24 articles | PMID: 32905133 | PMCID: PMC7465809
Review Free full text in Europe PMC
Go to all (29) article citations
Data
Data behind the article
This data has been text mined from the article, or deposited into data resources.
BioStudies: supplemental material and supporting data
Nucleotide Sequences (Showing 6 of 6)
- (1 citation) ENA - BC020546
- (1 citation) ENA - S75359
- (1 citation) ENA - D89675
- (1 citation) ENA - AF169346
- (1 citation) ENA - BC036445
- (1 citation) ENA - U59423
Show less
RefSeq - NCBI Reference Sequence Database (Showing 8 of 8)
- (1 citation) RefSeq - NM_001101.3
- (1 citation) RefSeq - NM_001201.2
- (1 citation) RefSeq - NM_001024259.1
- (1 citation) RefSeq - NM_021073.2
- (1 citation) RefSeq - NM_001718.4
- (1 citation) RefSeq - NM_080407.1
- (1 citation) RefSeq - NM_001719.2
- (1 citation) RefSeq - NM_013107.1
Show less
Similar Articles
To arrive at the top five similar articles we use a word-weighted algorithm to compare words from the Title and Abstract of each citation.
Form-deprivation myopia induces decreased expression of bone morphogenetic protein-2, 5 in guinea pig sclera.
Int J Ophthalmol, 8(1):39-45, 18 Feb 2015
Cited by: 9 articles | PMID: 25709905 | PMCID: PMC4325239
The Role of the RhoA/ROCK Signaling Pathway in Mechanical Strain-Induced Scleral Myofibroblast Differentiation.
Invest Ophthalmol Vis Sci, 59(8):3619-3629, 01 Jul 2018
Cited by: 21 articles | PMID: 30029249
BMP-2 Is Involved in Scleral Remodeling in Myopia Development.
PLoS One, 10(5):e0125219, 12 May 2015
Cited by: 25 articles | PMID: 25965995 | PMCID: PMC4429026
Bone morphogenetic proteins.
Growth Factors, 22(4):233-241, 01 Dec 2004
Cited by: 1246 articles | PMID: 15621726
Review