Abstract
Purpose
Humanized antibody hPAM4 specifically binds a mucin glycoprotein expressed in pancreatic adenocarcinomas. This phase I study evaluated a single dose of (90)Y-clivatuzumab tetraxetan ((90)Y-labeled hPAM4) in patients with advanced pancreatic cancer.Experimental design
Twenty-one patients (4 stage III; 17 stage IV) received (111)In-hPAM4 for imaging and serum sampling before (90)Y-hPAM4. Study procedures evaluated adverse events, safety laboratories, computed tomography (CT) scans, biomarkers, pharmacokinetics, radiation dosimetry, and immunogenicity (HAHA).Results
(111)In-hPAM4 showed normal biodistribution with radiation dose estimates to red marrow and solid organs acceptable for radioimmunotherapy and with tumor targeting in 12 patients. One patient withdrew before (90)Y-hPAM4; otherwise, 20 patients received (90)Y doses of 15 (n = 7), 20 (n = 9), and 25 mCi/m(2) (n = 4). Treatment was well tolerated; the only significant drug-related toxicities were (NCI CTC v.3) grade 3 to 4 neutropenia and thrombocytopenia increasing with (90)Y dose. There were no bleeding events or serious infections, and most cytopenias recovered to grade 1 within 12 weeks. Three patients at 25 mCi/m(2) encountered dose-limiting toxicity with grade 4 cytopenias more than 7 days, establishing 20 mCi/m(2) as the maximal tolerated (90)Y dose. Two patients developed HAHA of uncertain clinical significance. Most patients progressed rapidly and with CA19-9 levels increasing within 1 month of therapy, but 7 remained progression-free by CT for 1.5 to 5.6 months, including 3 achieving transient partial responses (32%-52% tumor diameter shrinkage).Conclusion
(90)Y-Clivatuzumab tetraxetan was well tolerated with manageable hematologic toxicity at the maximal tolerated (90)Y dose, and is a potential new therapeutic for advanced pancreatic cancer.Free full text

Treatment of Advanced Pancreatic Carcinoma with 90Y-Clivatuzumab Tetraxetan: A Phase I Single-Dose Escalation Trial
Abstract
Purpose
Humanized antibody hPAM4 specifically binds a mucin glycoprotein expressed in pancreatic adenocarcinomas. This phase I study evaluated a single dose of 90Y-clivatuzumab tetraxetan (90Y-labeled hPAM4) in patients with advanced pancreatic cancer.
Experimental Design
Twenty-one patients (4 stage III; 17 stage IV) received 111In-hPAM4 for imaging and serum sampling before 90Y-hPAM4. Study procedures evaluated adverse events, safety laboratories, computed tomography (CT) scans, biomarkers, pharmacokinetics, radiation dosimetry, and immunogenicity (HAHA).
Results
111In-hPAM4 showed normal biodistribution with radiation dose estimates to red marrow and solid organs acceptable for radioimmunotherapy and with tumor targeting in 12 patients. One patient withdrew before 90Y-hPAM4; otherwise, 20 patients received 90Y doses of 15 (n = 7), 20 (n = 9), and 25 mCi/m2 (n = 4). Treatment was well tolerated; the only significant drug-related toxicities were (NCI CTC v.3) grade 3 to 4 neutropenia and thrombocytopenia increasing with 90Y dose. There were no bleeding events or serious infections, and most cytopenias recovered to grade 1 within 12 weeks. Three patients at 25 mCi/m2 encountered dose-limiting toxicity with grade 4 cytopenias more than 7 days, establishing 20 mCi/m2 as the maximal tolerated 90Y dose. Two patients developed HAHA of uncertain clinical significance. Most patients progressed rapidly and with CA19-9 levels increasing within 1 month of therapy, but 7 remained progression-free by CT for 1.5 to 5.6 months, including 3 achieving transient partial responses (32%–52% tumor diameter shrinkage).
Conclusion
90Y-Clivatuzumab tetraxetan was well tolerated with manageable hematologic toxicity at the maximal tolerated 90Y dose, and is a potential new therapeutic for advanced pancreatic cancer.
Introduction
PAM4 is a monoclonal antibody developed against a human pancreatic cancer cell line which binds to a mucin produced primarily in pancreatic adenocarcinomas (1–4). Immunohistologic studies have indicated that PAM4-reactive mucin is present in early transformation, such as PanIn (pancreatic intraepithelial neoplasia) and IPMN (intraductal papillary mucinous neoplasm) lesions, but not in normal pancreatic ducts, pancreatitis, or other normal tissues (1). This antigen can also be detected in the serum, with evidence of a high specificity and sensitivity for pancreatic cancer detection (3), suggesting that it is a potentially important biomarker for malignant transformation of the pancreas.
The specificity of PAM4 also makes it an attractive agent for pancreatic cancer imaging and therapy (2). Pilot clinical studies using 131I-labeled murine PAM4 in 5 patients with suspected pancreatic cancer showed promising localization in lesions in 4 patients subsequently confirmed to have pancreatic cancer at surgery. No targeting was noted in a patient with pancreatitis (5). Preclinical studies in nude mice bearing human pancreatic carcinoma xenografts indicated that 131I- and 90Y-labeled PAM4 could significantly control tumor growth, even in well-established subcutaneous implants, with the best responses occurring with 90Y- PAM4 (6, 7). When combined with gemcitabine, further therapeutic improvements were observed (8–10).
These preclinical studies provided the impetus to humanize PAM4 (hPAM4) so that clinical studies could be conducted to assess 90Y-hPAM4 for the treatment of advanced (stage III/IV) pancreatic carcinoma by using a DOTA (1,4,7,10-tetraazacyclododecane-1,4,7,10-tetraacetic acid) conjugate of hPAM4 (clivatuzumab tetraxetan), which had been developed in kit form for 15-minute 90Y radiolabeling. Our ultimate goal was to test a fractionated dosing regimen in combination with gemcitabine given in an amount to enhance radiation sensitization. However, before initiating this protocol, an open-label, multicenter phase I clinical trial in patients with stage III/IV pancreatic adenocarcinoma was carried out. The primary objective was to determine dose-limiting toxicity (DLT) and the maximum tolerated dose (MTD) for 90Y-hPAM4 given as a single infusion. Organ distribution, pharmacokinetics (PK), tumor targeting, and dosimetry were also obtained in all patients by a pretherapy imaging study by using 111In-labeled hPAM4 as a surrogate to predict 90Y-hPAM4 clearance and distribution. Because hPAM4 binds a mucin detected in the serum, patients also received 1 of 2 hPAM4 protein doses with their radiolabeled injections to determine whether the antigen's presence would alter PK and organ distribution.
Materials and Methods
Patients
Patients were required to be 18 years or older with histologically or cytologically confirmed stage III/IV pancreatic adenocarcinoma. Patients with stage III disease must have progressed after initial therapy, whereas those with stage IV disease should not have received more than 1 prior chemotherapy regimen. All patients were required to be 4 weeks beyond chemotherapy, radiotherapy, major surgery, or other experimental treatments, and to have recovered from all acute toxicities (at study entry, ≥grade 1). Patients previously receiving nitrosureas, actinomycin D, prior radioimmunotherapy, other antibody-based therapies, or prior radiation to more than 30% of the red marrow or to maximal tolerable level for any organ were excluded. Additional requirements included an adequate performance status [≤70 Karnofsky performance status (KPS) or Eastern Cooperative Oncology Group (ECOG) ≥1) with life expectancy 3 months ore more, adequate hematologic parameters without ongoing transfusional support (hemoglobin ≤10 g/dL, ANC ≤1.5 109/L, platelets 150 × 109/L), no known history of active coronary artery disease, unstable angina, myocardial infarction, or congestive heart failure present within 6 months or cardiac arrhythmia requiring antiarrhythmia therapy, no known history of active chronic obstructive pulmonary disease or other moderate-to-severe respiratory illness present within 6 months, and adequate renal and hepatic function (creatinine and bilirubin ≥1.5 × institutional upper limit of normal, AST and ALT ≥2.0 × IULN). Patients with CNS metastatic disease, a single tumor mass more than 10 cm in its greatest diameter, anorexia (>grade 2), nausea, vomiting or signs of intestinal obstruction, known HIV, hepatitis B, or hepatitis C positivity, known autoimmune disease or presence of autoimmune phenomena (except rheumatoid arthritis requiring only low-dose maintenance corticosteroids), infection requiring intravenous antibiotic use within 1 week, use of corticosteroids within 2 weeks, and other concurrent medical or psychiatric conditions that, in the investigator's opinion, could confound study interpretation or prevent completion of study procedures, were excluded. The Institutional Review Board approved the study at each participating site, and written informed consent was obtained from all patients.
Radiolabeling
The DOTA conjugate of hPAM4 was prepared by the sponsor (Immunomedics, Inc.). Quality control testing included using an anti-idiotypic antibody after radiolabeling the DOTA conjugate to ensure immunoreactivity of the final product was maintained. For patient use, radiolabeling of DOTA-conjugated hPAM4 with 111InCl3 (sterile solution; Mallinckrodt/Covidien Imaging Solutions) or 90YCl3 (MDS Nordion) was carried out by a local radiopharmacy by using 5 mg or less protein for 111In or 2 mg/mCi or less for 90Y, as reported previously (11). Instant thin layer chromatography testing was done and was required to show less than 10% unbound radio-nuclide to be released for patient use. Using a precalibrated dose calibrator, the prescribed radiolabeled doses were prepared as an 8-mL volume in a 10-mL syringe and delivered to the investigational sites for use within 2 hours of preparation.
Treatment
All patients received 3 to 5 mCi of 111In-hPAM4 at least 1 week before 90Y-hPAM4 to assess PK and ensure that biodistribution and radiation dosimetry estimates for therapy were acceptable. The prepared 111In- and 90Y-hPAM4 each contained less than 10 mg total antibody and both were administered intravenously by slow injection over 10 minutes. Initially, cohorts of 3 to 6 patients received a single dose of 90Y-hPAM4 with 90Y doses starting at 15 mCi/m2 (555 MBq/m2) and escalated in 5-mCi/m2 (185 MBq/m2) increments. DLT was defined as grade 4 hematologic toxicity lasting 7 days or more; a grade 3 nonhematologic toxicity of any duration; or failure of platelets, absolute neutrophil count (ANC), or hemoglobin to recover to grade 1 within 12 weeks, with platelet and red blood cell (RBC) transfusions, as well as growth factors, permitted to aid hematologic recovery. Escalation continued as long as 0 of 3 or 1 of 6 patients at a particular 90Y dose encountered DLT, but ceased if there were 2 or more DLTs. The highest 90Y dose with 6 patients and at most 1 DLT was determined to be the MTD, with several additional patients then treated at or below the MTD. Prior to each radiolabeled antibody injection, additional unconjugated hPAM4 was administered intravenously over 30 to 40 minutes (10% infused in 10 minutes and if vital signs were stable, remaining dose over 20–30 minutes) during dose escalation (100 mg total antibody doses), but not after the MTD was determined (<10 mg total antibody doses). All antibody administrations were given without steroids or any other premedication.
Study assessments
After 111In-hPAM4, blood samples were collected at 0.5, 1, 2, and 4–6 hours and then on days 1, 2, 3, 4–5, and 6–7 for 111In radioactivity serum levels which were measured at each site by using a precalibrated g-scintillation counter. Whole-body anterior/posterior planar 111In scintigraphy was also carried out repeatedly over 7 days by using a precalibrated g-camera with an 111In-calibration standard in the field of view. The initial 6 patients also had total urine collections over 3 days starting 4 hours after administration of 111In-hPAM4. All images were evaluated for antibody biodistribution and tumor localization by the local nuclear medicine physician. To proceed to treatment, the biodistribution had to be acceptable with no abnormal pattern of uptake among the major organs that could not be explained by normal variability or incomplete radiolabeling, but demonstrable evidence of tumor targeting was not required. Antibody PK used SAAM II version 1.1.1 software (SAAM Institute, Inc.) to generate multiexponential fits to decay-corrected 111In serum levels. The radiation dosimetry methodology has been described previously (12, 13). Briefly a Co-57 patient transmission scan allowed 111In counts from organ regions of interest (ROI) on each whole-body image to be corrected for radiation attenuation losses, whereas a computed tomography (CT) image allowed for organ-specific subtraction of background counts from overlapping tissue. Using standard male and female phantom organ masses, solid organ radiation dose estimates were determined by MIRDOSE3.1 software (14) from the geometric mean of corrected anterior and posterior ROI counts, whereas red marrow radiation dose estimates were generated according to the method of Sgouros (15). To proceed with treatment, the dose estimates were required to be below standard limits for external beam radiation therapy (16), but often used for nonmyeloablative radioimmunotherapy trials (<300 cGy for red marrow, <3,000 cGy for liver, and <2,000 cGy for kidney and lung).
Adverse events were classified according to the Med-DRA (Medical Dictionary for Regulatory Affairs, Chantilly, VA) and graded according to the National Cancer Institute Common Toxicity Criteria, version 3.0. Serum chemistries, physical exams, vital signs, and urinalysis were conducted over 12 weeks after treatment, with weekly blood counts or more frequently for grade 3 or higher cytopenias. Blood samples were drawn at baseline and then at 4, 8, and 12 weeks posttreatment for determination of human anti-hPAM4 antibodies (HAHA) by ELISA, using an anti-idiotype antibody as a standard. Values greater than 50 ng/mL were considered positive. Serum levels of CA19-9 were obtained at baseline and then at 1, 4, 8, and 12 weeks posttreatment. Serum levels of the PAM4-reactive mucin were evaluated at the same time points by ELISA assay according to Gold and colleagues (3, 4). CT scans were obtained at baseline and 4, 8 and 12 weeks after 90Y-hPAM4, with the best treatment response at any of these time points classified by Response Evaluation Criteria in Solid Tumors (RECIST) criteria (17) as a complete response (CR), partial response (PR), stable, or progressive disease.
Statistical analyses
Safety analyses focused primarily on the treatment day and the 12-week posttreatment evaluation period, but included any potentially treatment-related toxicities occurring after 12 weeks. Response rates were summarized by descriptive statistics. Progression-free survival (PFS) was calculated from the 90Y-hPAM4 administration to the earliest occurrence of progression, death, or last contact. Duration of response from the onset of an objective response (OR; i.e., CR, or PR) to the same events and overall survival (OS) from the 90Y-hPAM4 administration to the earliest occurrence of death or last contact were also summarized.
Results
Twenty-one patients with pancreatic adenocarcinoma (17 with stage IV metastatic disease and 4 with stage III locally advanced disease) were enrolled. Three patients with metastatic disease were treatment-naive, whereas all the other 18 patients had received prior chemotherapy (±external patients radiation ± primary tumor resection). All had CT-measurable disease, primarily involving the pancreas and/or liver, and most had elevated baseline serum levels of both CA19-9 and PAM4-reactive mucin. Demographics and patient characteristics are summarized in Table 1. The 21 patients received 111In-hPAM4 and underwent pretherapy evaluations of antibody biodistribution and PK, tumor targeting, and radiation dosimetry. All patients had normal biodistribution, and radiation dosimetry estimates acceptable for treatment. One patient withdrew prior to 90Y-hPAM4 after developing gastric outlet obstruction from disease progression. All the other 20 patients received their dose of 90Y-hPAM4.
Table 1
Demographics/baseline data (N = 21)
Sex, male/female; n | 9/12 |
Age, median (range); y | 62 (34–77) |
ECOG, 0/1 | 12/9 |
Disease stage at study entry | |
III (locally advanced) | 4 |
IV (metastatic) | 17 |
Time from diagnosis, median (range), mo | 9 (0.1–34) |
Prior therapy, n | 18 |
Modalities | |
Chemotherapya | 18 |
External radiation therapy | 8 |
Surgeryb | 6 |
Time from last therapy, median (range), mo | 1.7 (0.9–27.6) |
CT identified tumor locations, n | |
Pancreas/pancreatic bed | 16 |
Liver | 11 |
Chest/lungs | 4 |
Other | 4 |
Largest target lesion, median (range); cm | 4.5 (2.1–6.3) |
Hematology, median (range) | |
Hemoglobin, g/dL | 12.4 (10.1–15) |
Neutrophils, K/μL | 4.6 (2.8–10) |
Platelets, K/μL | 261 (149–958) |
CA19-9, median (range); U/mL | 338 (5–33,359) |
90Y-hPAM4 dose level | |
15 mCi/m2 (555 MBq/m2) | 8 |
20 mCi/m2 (740 MBq/m2) | 9 |
25 mCi/m2 (925 MBq/m2) | 4 |
Treatment administration
All DOTA-hPAM4 radiolabeled products met release criteria (median unbound isotope: 0.7% 111In, 1.1% 90Y). During the initial dose escalation portion of the study, 15 patients received 90Y-hPAM4 at 90Y doses of 15 mCi/m2 (555 MBq/m2; n = 4), 20 mCi/m2 (740 MBq/m2; n 7), and 25 mCi/m2 (925 MBq/m2; n = 4). After the MTD = was determined, 6 additional patients were entered to provide more experience (3 at 15 mCi/m2, 2 at 20 mCi/m2, 1 withdrawing after 111In-hPAM4). All administrations of study drug occurred without interruption or premature termination, with 111In- and 90Y-hPAM4 both given at total antibody doses of 100 mg for the initial 15 patients and less than 10 mg for the final 6 patients.
Adverse events and dose-limiting toxicity
There were no infusion reactions to administration of study drug. Other than events typically occurring in patients with pancreatic cancer and advanced disease (primarily gastrointestinal complications, pain, and constitutional complaints), the adverse event profile reflected cytopenias that were expected following radioimmunotherapy. The most frequent events (>10% of patients) were vomiting (35%); fatigue, anemia, nausea (30% each); abdominal pain, leukopenia (25% each), constipation, anorexia, thrombocytopenia, diarrhea (20% each), dehydration, back pain, ascites, asthenia, fever, and dyspnea (15% each). Eleven patients had 19 serious adverse events. Of these, 7 events were cytopenias attributed to radio-immunotherapy, 1 event of vomiting was considered possibly related to study drug but with multiple other likely explanations, whereas all other events (dehydration, pain, dyspnea, gastric outlet obstruction, asthenia, confusion) were attributed to complications of underlying disease and were unrelated to study drug. No bleeding episodes occurred, and other than 1 upper respiratory infection and 1 urinary tract infection, none of the patients developed infections. No abnormal pattern of changes occurred in standard serum chemistries, with no grade 3 to 4 events except for several isolated elevations of transaminase, alkaline phosphatase, or bilrubin consistent with hepatic involvement, biliary obstruction, and other abdominal complications expected in this population (data not shown). Grade 3 or 4 anemia was rare at any dose level and required few transfusions of RBCs, but the incidence of severe thrombocytopenia and neutropenia, as well as platelet transfusions and growth factor support, increased with total 90Y dose (Table 2). For patients with grade 3 or 4 events, the median time from 90Y-hPAM4 therapy to nadir value was 4.8 weeks (range, 3.8–6.6 weeks) for platelets and 4.8 weeks (range, 2.9–5.7 weeks) for neutrophils.
Table 2
Hematologic toxicity stratified by 90Y dose
90Y Dose level (mCi/m2) | |||
---|---|---|---|
15 (n = 7) | 20 (n = 9) | 25 (n = 4) | |
Patients with grade 3 or 4 nadirs (%) | |||
Hemoglobin | |||
Grade 3 | 0 (0) | 2 (22) | 0 (0) |
Grade 4 | 0 (0) | 0 (0) | 0 (0) |
Platelets | |||
Grade 3 | 0 (0) | 4 (44) | 0 (0) |
Grade 4 | 0 (0) | 1 (11) | 3 (75) |
Neutrophils | |||
Grade 3 | 0 (0) | 2 (22) | 0 (0) |
Grade 4 | 0 (0) | 2 (22) | 3 (75) |
Patients administered hematologic support (%) | |||
RBC | 0 (0) | 4 (44) | 2 (50) |
Platelets | 0 (0) | 1 (11) | 3 (75) |
G-CSF | 0 (0) | 4 (44) | 0 (0) |
EPS | 1 (14) | 1 (11) | 2 (50) |
Abbreviations: G-CSF, granulocyte-colony stimulating factors; EPS, erythropoiesis-stimulating agents.
The MTD was declared to be 20 mCi/m2, because during dose escalation there were no DLTs at 15 mCi/m2, 1 DLT among 7 patients (6 evaluable for DLT assessment, 1 inevaluable after entering hospice 5 weeks posttreatment) at 20 mCi/m2, and 3 patients at 25 mCi/m2 with DLTs. No DLTs occurred in the 5 patients treated after dose escalation at or below the MTD. All DLTs were due to grade 4 thrombocytopenia and/or neutropenia (thrombocytopenia was generally the more severe) lasting more than 7 days. There was no hematologic DLT due only to prolonged recovery beyond 12 weeks. This was unable to be assessed in 4 patients (3 patients entered hospice with early study terminations prior to recovery and 1 patient had platelet recovery to near grade 1 levels but then developed disseminated intravascular coagulation). Otherwise, the median recovery time from grade 3 or 4 nadirs to grade 1 levels was 2.7 weeks (range, 1.7–4.1 weeks) for platelets and 1.9 weeks (range, 1.0–2.8 weeks) for neutrophils.
Pharmacokinetics and biomarkers
Serum levels of 111In-hPAM4, after correction for 111In physical decay, generally decreased monoexponentially over 7 days, with a half-life (mean ± SD) of 4.6 ± 0.9 days (range, 3.5–7.0) for the initial 15 patients (100 mg total antibody dose) and 3.8 ± 0.5 days (range, 3.2–4.6) for the final 6 patients (<10 mg total antibody dose). Serum antibody levels were also measured by ELISA assay over 7 days after 111In-hPAM4. The median Cmax was 21 mg/mL (range, 10–41) with a half-life of 5.1 days (range, 3.9–13.2) for the initial 15 patients, and the median Cmax was 4 mg/mL (range, 2–7) for the final 6 patients who had measurable levels over 7 days, but too low for half-life determinations. CA19-9 serum levels were elevated in 18 of the 20 treated patients at study entry. By 4 weeks after 90Y-hPAM4, CA19-9 levels had increased more than 25% from baseline in 15 patients and were either stable (n = 3) or had decreased more than 25% (n = 2) in the other 5 patients (2 with no further evaluations, 2 with >25% increases at next evaluation, and 1 with levels still decreased at last evaluation at 12 weeks). In 18 treated patients with measured serum levels of hPAM4-reactive mucin, the median value at study entry was 21 units/mL (range, 1.5–1,398), including 14 patients (78%) with levels above upper limits established in patients without pancreatic cancer (>2.4 units/mL). Posttreatment mucin levels could not be reliably measured due to interference in the ELISA assay from administrated hPAM4.
Biodistribution and tumor targeting
All 111In-hPAM4 images were evaluated by the investigators as having normal biodistribution and with no evidence of fixed gastrointestinal or genitourinary uptake. 111In-hPAM4 whole-body planar imaging is suboptimal for detecting pancreatic tumors or metastases in the liver. Nonetheless, the investigators reported visual evidence of tumor targeting in 12 patients, including pancreatic tumors or residual tumor in the pancreatic bed (n = 9) or other abdominal sites of confirmed involvement (n = 3). All the visualized pancreatic targeting occurred in patients with the higher serum levels of hPAM4-reactive mucin (28–332 U/mL), and involved 40% (6 of 15) of patients receiving 100 mg total antibody compared with 50% (3 of 6) receiving <10 mg. An internal review by the sponsor of all images found no qualitative differences in biodistribution or intensity of tumor uptake between patients receiving 100 mg or <10 mg total antibody. An example of antibody biodistribution and tumor targeting with 111In-hPAM4 imaging is provided in Figure 1.
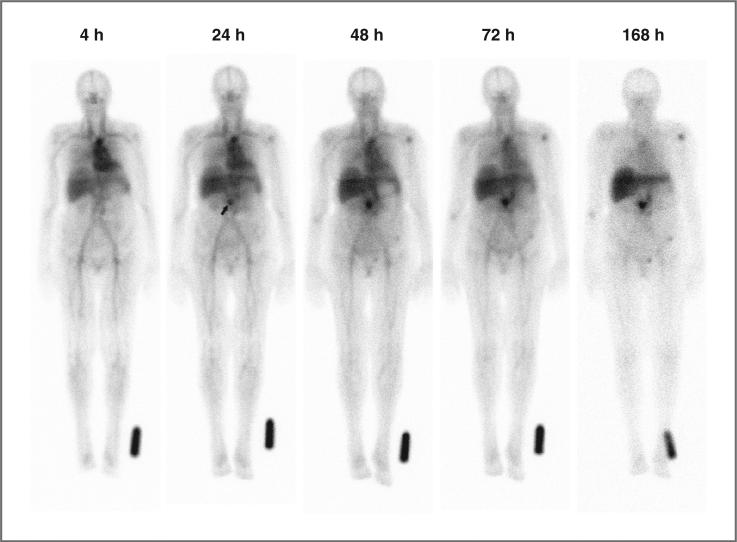
Serial anterior whole-body planar scintigraphic images showing normal antibody biodistribution and tumor targeting in a patient with a recurrent mass in the pancreatic bed after undergoing a Whipple procedure. CT imaging had revealed a 1.5 × 2.1-cm mass surrounding the distal superior mesenteric artery. The images were acquired sequentially over 1 week, beginning 4 hours after administration of 111In-hPAM4. Image intensities were normalized by using an 111In standard near the left foot to correct for 111In physical decay and different acquisition times over this period. Uptake at the pancreatic site is clearly seen at 24 hours (arrow), and becomes progressively more prominent on subsequent images. Mild decreased intensity across the upper aspect of the liver is due to attenuation from breast tissue. Mild focal uptake in the left shoulder and pelvic area is of uncertain significance.
Radiation dosimetry and total body clearance
Table 3 provides estimates of the radiation dose that each mCi of 90Y-hPAM4 delivers to the whole body and organs. There are no substantial differences between patients receiving 100 mg or less than 10 mg total antibody, although the liver radiation dose seems modestly decreased in patients receiving additional antibody. Combining the 2 groups, the estimated radiation doses received from treatment are summarized in Table 4. The median dose to the whole body and each individual organ increases with each 90Y dose level, as expected. However, even at 25 mCi/m2, which exceeds the MTD of 20 mCi/m2, the highest radiation dose received by any patient remains well below the defined organ radiation dose limits (red marrow <300 cGy; liver <3,000 cGy, lungs and kidneys <2,000 cGy). Pooled urine was collected in 6 patients at intervals over 3 days after 111In-hPAM4 and the counts were corrected for 111In-physical decay. The median estimated percent of the administered antibody eliminated in the urine was 3.7% (range, 2.5%–7.5%), with a median of 1.2% excreted by 4 hours, 0.8% from 4 to 24 hours, 0.7% from 24 to 48 hours, and 0.9% over 48 to 72 hours. From this, the median half-life for whole-body clearance of the antibody by urinary excretion was 63 days (range, 33–94 days) compared with 60 days (range, 39–91 days) determined by whole-body scintigraphy for these 6 patients.
Table 3
Radiation dose estimates (mGy/mCi) stratified by antibody dose
Total antibody administered | ||
---|---|---|
100 mg (n = 15)a | <10 mg (n = 5) | |
Whole body | 2.7 ± 0.4 | 2.5 ± 0.4 |
Red marrow | 4.1 ± 1.2 | 3.8 ± 0.9 |
Liver | 15.0 ± 5.0 | 20.8 ± 1.9 |
Spleen | 20.6 ± 12.9 | 22 ± 13.8 |
Lungs | 14.8 ± 5.7 | 11.7 ± 4.6 |
Kidneys | 16.0 ± 5.0 | 18.7 ± 4.8 |
Testesb | 6.9 ± 5.2 | 8.2 ± 3.3 |
Ovariesc | 2.1 ± 0.2 | 1.9 ± 0.3 |
Lens of eye | 2.0 ± 0.2 | 1.6 ± 0.4 |
Urinary bladder | 1.4 ± 0.4 | 1.7 ± 0.3 |
NOTE: Mean ± SD of the estimated radiation dose to whole body and organs from 90Y-hPAM4 per mCi of 90Y administrated.
Table 4
Radiation dose estimates (mGy) stratified by 90Y dose
90Y Dose Level (mCi/m2) | |||
---|---|---|---|
15 (n = 7) | 20 (n = 9) | 25 (n = 4) | |
Whole body | 67 (56–75) | 96 (86–109) | 129 (119–141) |
Red marrow | 108 (88–190) | 139 (118–163) | 176 (124–202) |
Liver | 418 (304–698) | 546 (339–853) | 803 (669–914) |
Spleen | 319 (89–862) | 762 (68–1,890) | 1,112 (631–1,444) |
Lungs | 334 (181–475) | 483 (400–1,036) | 534 (461–572) |
Kidneys | 409 (287–772) | 526 (306–916) | 635 (461–779) |
Testesa | 182 (80–356) | 151 (45–176) | 630 (492–769) |
Ovariesb | 49 (33–54) | 68 (60–78) | 94 (94–94) |
Lens of eye | 48 (33–54) | 68 (60–78) | 94 (83–113) |
Urinary bladder | 41 (34–52) | 48 (24–97) | 70 (59–85) |
NOTE: Median (range) estimated radiation dose to whole body and organs from 90Y-hPAM4 for patients receiving 90Y at dose levels of 15, 20, or 25 mCi/m2.
Of the 12 patients with visual tumor targeting by 111In-hPAM4 scintigraphy, the radiation doses delivered to the primary tumor could be estimated in 2 patients who had discrete focal uptake seen on both anterior and posterior images. The patient shown in Figure 1 was treated at 20 mCi/m2, with a 1.5 × 2.1-cm pancreatic mass receiving 5,035 cGy, and remaining stable in size when evaluated 4 weeks later, although new lesions were seen at that time. The other patient was treated at 15 mCi/m2, with a much larger 4.3 × 3.4-cm mass in the head of the pancreas receiving a more modest radiation dose of 457 cGy and increasing in size 4 weeks later.
Immunogenicity
Baseline levels of anti-hPAM4 antibodies (HAHA) were all at or below the 50 ng/mL cutoff of the assay. Posttreatment serum samples evaluated for at least 8 weeks in most patients were negative, except for 2 patients with positive levels (one 1,460 ng/mL at 8 weeks and one 1,193 ng/mL at 4 weeks) of uncertain significance, because there were no associated clinical sequelae.
Clinical efficacy
Of the 20 patients, 13 had progressed by the first CT-based evaluation at 4 weeks following therapy. Although none of the other 7 patients achieved CRs, 3 patients (1 treated at 15 mCi/m2 and 2 at 20 mCi/m2) met RECIST criteria for unconfirmed PRs (imaging examples are provided in Fig. 2). They achieved 32% to 52% shrinkage of the sum of the longest diameters of their target lesions at 4-week evaluations (1 patient with a 4.5-cm pancreatic mass decreasing to 3.3 cm and a 2.3-cm liver mass no longer visible, the others with locally advanced disease decreasing from 6.3 cm to 3.0 cm and 3.7 cm to 2.5-cm), but subsequently progressed with new lesions at their 8-week evaluations. The remaining 4 patients had stable disease at 4-week evaluations (2 patients with ~5-cm masses both progressing by 8 weeks, the others with 2- to 3-cm masses progressing at 12- and 24-week evaluations). The median PFS for all 20 patients was only 4.3 weeks after treatment, whereas the 7 patients who had not progressed by the first CT-based evaluation at 4 weeks following therapy remained progression-free for 1.5 to 5.6 months.
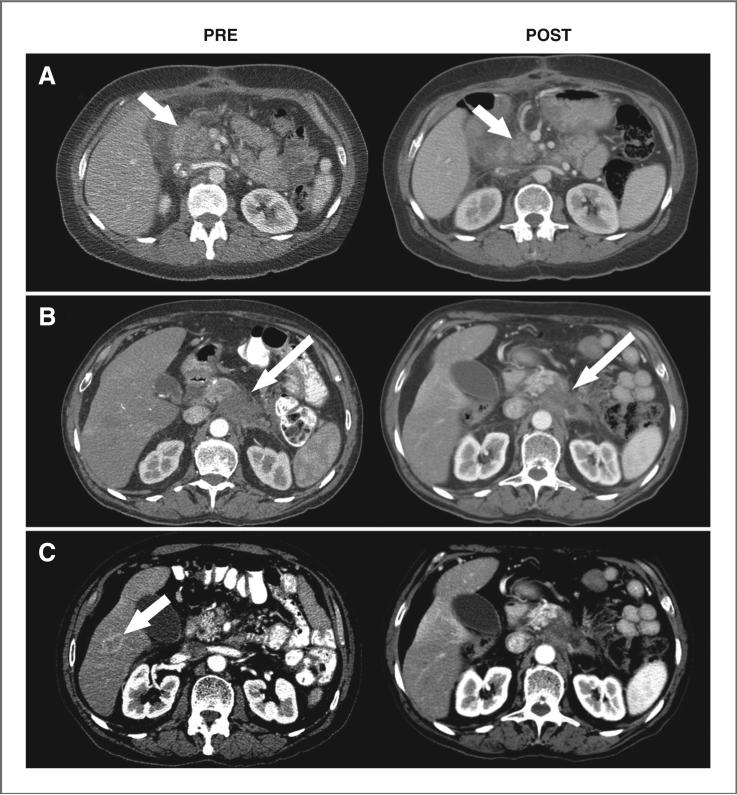
CT image examples before (PRE) and after treatment with 90Y-hPAM4 (POST). A, a 63-year-old female diagnosed 5 months earlier with locally advanced pancreatic cancer was previously treated with gemcitabine and external radiation therapy. At study entry, the patient had a 6.3-cm mass in the head of the pancreas which reduced to 3.0 cm by 4 weeks after 90Y-hPAM4 (arrows). B and C, a 70-year-old male diagnosed 4 months earlier with locally advanced disease received 5-FU and external radiation therapy initially and then gemcitabine. At study entry, the patient had a 4.5-cm mass in the body of the pancreas which reduced to 3.3 cm after treatment with 90Y-hPAM4 (long arrows) and a 2.3-cm lesion in the liver (short arrow) that was no longer measurable.
All 20 treated patients have now died. The median OS was 4.3 months (range, 1.5–22.2), with 9 patients expiring 1.5 to 2.9 months after therapy, 5 patients surviving 4.1 to 5.6 months, and 6 patients surviving 9.3 to 22.2 months. Exploratory survival analyses were inconclusive regarding 90Y dose dependency, due to small patient numbers at each dose level. There was an apparent trend toward improved survival for patients at study entry with smaller-sized tumors [median OS 7.5 months for maximum lesions 4.5 cm (n = 10) vs. 2.2 months for larger lesions (n = 10)] and better performance status [median OS 5.6 months for ECOG 0 (n = 11) vs. 2.9 months for ECOG 1 (n 9)], and for those without progression 4 weeks after treatment [median OS = 5.6 months with PR or stable disease (n = 7) vs. 2.4 months with progression (n = 13)].
Discussion
The outlook for patients with advanced pancreatic cancer remains dismal, with the 2 approved systemic therapies (gemcitabine and erlotinib) offering only a modest survival benefit (18, 19). More recently, a 3-drug combination of fluorouracil (5-FU), oxaliplatin, and irinotecan showed improved survival compared with gemcitabine monotherapy (20). However, patients with metastatic disease are still not cured with conventional chemotherapy, and there remains an urgent need for new approaches, such as the antibody-targeted radiotherapeutic, 90Y-hPAM4 evaluated in this initial single-dose phase 1 trial. This agent is based on the humanized version of the PAM4 antibody that targets a mucin glycoprotein specifically expressed in pancreatic adenocarcinoma. Preclinical studies with 90Y-hPAM4 not only showed efficacy as a single agent in treating human pancreatic carcinoma xenografts, but also showed an enhanced response when combined with gemcitabine (8–10), which is a well-known radiosensitizing agent (21). Additionally, other radioimmunotherapies have indicated that patients were able to tolerate a higher total cumulative dose of 90Y when given in weekly fractions (22). However, before proceeding to clinical trials in combination with gemcitabine and taking advantage of a fractionated dosing regimen to increase the radiation dose, this study was undertaken to evaluate a single dose of 90Y-hPAM4 administered as a single agent to patients with advanced pancreatic cancer.
This trial showed that 90Y-hPAM4 was well tolerated and that up to 100 mg of the hPAM4 antibody could be given without infusion-type reactions. As expected with radio-immunotherapy, the only significant toxicity was transient hematologic suppression, with grades 3 and 4 neutropenia and thrombocytopenia increasing as the 90Y dose was escalated. In spite of the fact that most patients had received gemcitabine or other prior chemotherapy that can damage the bone marrow, there were no bleeding events or serious infections even at the highest 90Y dose tested, and most cytopenias recovered to grade 1 levels within 12 weeks. Although dosimetry was evaluated in each patient prior to 90Y-hPAM4 administration, the limitation of red marrow dosimetry to predict hematalogic toxicity is well known with other 90Y-labeled antibodies, likely reflecting damage from prior chemotherapy or other causes of interpatient variability in bone marrow reserve (23). Thus, even though the radiation dose estimates to the red marrow remained below standard limits (300 cGy), dose escalation stopped after 3 patients at 25 mCi/m2 encountered protocol-defined DLT with grade 4 cytopenias more than 7 days. As only 1 of 6 evaluable patients already treated at the next lower dose level of 20 mCi/m2 had a DLT with grade 4 thrombocytopenia lasting more than 7 days, 20 mCi/m2 was declared as the MTD for single administration of 90Y-hPAM4 in this population in which most patients had received at least one prior treatment. Of note, this last patient with DLT had been heavily pretreated with 4 prior chemotherapeutic agents, yet the platelet counts were still able to recover to grade 1 levels posttreatment, allowing the patient to initiate gemcitabine when disease progression subsequently occurred. Further, after the MTD was determined, 2 additional patients were treated at 20 mCi/m2 and neither encountered DLT. Thus, although limited by the relatively few number of patients treated in this study, a single administration of 90Y-hPAM4 had manageable hematologic toxicity at the MTD, and on this basis, a starting cumulative dose of 20 mCi/m2 was selected for initiating subsequent studies in which 90Y-hPAM4 is given in fractionated doses in combination with gemcitabine (24).
Most patients entered this study with elevated serum levels of PAM4-reactive mucin. There was no evidence that tumor targeting was substantially hindered, because 111In-hPAM4 visualized pancreatic tumors in patients at the higher serum levels, and all patients showed a normal antibody biodistribution pattern over 7 days with the expected retention in the liver for a radiometal-labeled antibody and with no unusual splenic uptake. As a precaution to avoid potential antibody sequestration by serum mucin or by low-level nonspecific antigen expression on some tissues, patients initially entered during dose escalation had their radiolabeled antibodies given with additional hPAM4 (100 mg total antibody dose), whereas for comparison, patients entered after the MTD was determined received their radiolabeled antibodies without any additional hPAM4 (<10 mg total antibody dose). There seems to be no advantage to adding additional antibody with this therapy, because no differences were noted between PK, biodistribution, or normal organ dosimetry estimates for the 2 groups, except for the radiation dose estimate to the liver, which appeared modestly increased in patients without additional antibody, but remained well within acceptable limits for radioimmunotherapy at all 90Y dose levels in this study. Although only a small number of patients in this study did not receive additional antibody, it did appear that tumor targeting was at least comparable in this group, and in a preliminary report from an ongoing trial of 90Y-hPAM4 administered without additional antibody to a larger group of patients, neither blood clearance nor liver uptake seemed appreciably influenced by serum mucin levels (24). Furthermore, targeting studies in animal models instead reported that uptake in human pancreatic xenografts was reduced at higher antibody doses (25), presumably a result of competitive binding at tumor sites between the unlabeled and radiolabeled antibodies, further supporting the decision to continue 90Y-hPAM4 therapy in future studies without requiring administration of additional antibody.
Although animal models have been highly supportive of the antitumor activity of radiolabeled antibodies in solid tumors, ORs have been infrequently reported clinically (26). Thus, we are encouraged by the antitumor activity observed in this initial small study, with 3 transient PRs and 4 patients achieving at least temporary disease stabilization by CT-based criteria, and 2 patients having decreases in serum levels of CA19-9, albeit short-lived. These results, obtained with a single dose of 90Y-hPAM4 alone in this difficult-to-treat population, support a more promising treatment regimen now underway in which 90Y-hPAM4 is fractionated into several weekly doses to enhance the total radiation dose delivered to tumors and also administered in combination with radiosensitizing doses of gemcitabine to increase the potency of the radiation delivered (24).
In conclusion, radioimmunotherapy with a single dose of 90Y-clivatuzumab tetraxetan is well tolerated without the need for premedication or additional antibody, and with manageable hematologic toxicity at the maximal tolerated 90Y dose. Further study of this promising treatment strategy for pancreatic cancer is ongoing.
Acknowledgments
We thank Henry Founds, Preeti Trisal, and Kiril Gordeyev for contributions to the pharmacokinetic and immunoassay analyses; Dion Yeldell for performing radiation dosimetry assessments; and Susan Garl for clinical trial coordination.
Footnotes
Presented in part at the 54th Annual Meeting of the Society of Nuclear Medicine, Washington, DC, June 2–6, 2007.
Current address for S.A. Gulec: The Herbert Wertheim College of Medicine, Florida International University Miami, Florida.
Disclosure of Potential Conflicts of Interest
H. Horne, W.A. Wegener, N. Teoh, and D.M. Goldenberg are employed by or have financial interests with Immunomedics, Inc. S.A. Gulec, S.J. Cohen, K.L. Pennington, L.S. Zuckier, and R.J. Hauke received research support from Immunomedics, Inc. for this study.
References
Full text links
Read article at publisher's site: https://doi.org/10.1158/1078-0432.ccr-10-2579
Read article for free, from open access legal sources, via Unpaywall:
https://aacrjournals.org/clincancerres/article-pdf/17/12/4091/1998350/4091.pdf
Citations & impact
Impact metrics
Article citations
Radiolabeled Antibodies for Cancer Imaging and Therapy.
Cancers (Basel), 14(6):1454, 11 Mar 2022
Cited by: 23 articles | PMID: 35326605 | PMCID: PMC8946248
Review Free full text in Europe PMC
Molecular Targeted Positron Emission Tomography Imaging and Radionuclide Therapy of Pancreatic Ductal Adenocarcinoma.
Cancers (Basel), 13(24):6164, 07 Dec 2021
Cited by: 7 articles | PMID: 34944781 | PMCID: PMC8699493
Review Free full text in Europe PMC
Radioimmunotherapy Combined With Low-Intensity Ultrasound and Microbubbles: A Potential Novel Strategy for Treatment of Solid Tumors.
Front Oncol, 11:750741, 22 Oct 2021
Cited by: 2 articles | PMID: 34745976 | PMCID: PMC8570127
Review Free full text in Europe PMC
Radioimmunotherapy in Oncology: Overview of the Last Decade Clinical Trials.
Cancers (Basel), 13(21):5570, 07 Nov 2021
Cited by: 10 articles | PMID: 34771732 | PMCID: PMC8583425
Review Free full text in Europe PMC
Understanding the Clinical Impact of MUC5AC Expression on Pancreatic Ductal Adenocarcinoma.
Cancers (Basel), 13(12):3059, 19 Jun 2021
Cited by: 16 articles | PMID: 34205412 | PMCID: PMC8235261
Review Free full text in Europe PMC
Go to all (39) article citations
Similar Articles
To arrive at the top five similar articles we use a word-weighted algorithm to compare words from the Title and Abstract of each citation.
Fractionated radioimmunotherapy with (90) Y-clivatuzumab tetraxetan and low-dose gemcitabine is active in advanced pancreatic cancer: A phase 1 trial.
Cancer, 118(22):5497-5506, 08 May 2012
Cited by: 53 articles | PMID: 22569804 | PMCID: PMC4215160
(90)Y-clivatuzumab tetraxetan with or without low-dose gemcitabine: A phase Ib study in patients with metastatic pancreatic cancer after two or more prior therapies.
Eur J Cancer, 51(14):1857-1864, 14 Jul 2015
Cited by: 15 articles | PMID: 26187510
Anti-CD22 90Y-epratuzumab tetraxetan combined with anti-CD20 veltuzumab: a phase I study in patients with relapsed/refractory, aggressive non-Hodgkin lymphoma.
Haematologica, 99(11):1738-1745, 22 Aug 2014
Cited by: 12 articles | PMID: 25150258 | PMCID: PMC4222463
A Phase I trial of 90Y-anti-carcinoembryonic antigen chimeric T84.66 radioimmunotherapy with 5-fluorouracil in patients with metastatic colorectal cancer.
Clin Cancer Res, 9(16 pt 1):5842-5852, 01 Dec 2003
Cited by: 46 articles | PMID: 14676105
Review