Abstract
Free full text

Glucocorticoids are critical regulators of dendritic spine development and plasticity in vivo
Abstract
Glucocorticoids are a family of hormones that coordinate diverse physiological processes in responding to stress. Prolonged glucocorticoid exposure over weeks has been linked to dendritic atrophy and spine loss in fixed tissue studies of adult brains, but it is unclear how glucocorticoids may affect the dynamic processes of dendritic spine formation and elimination in vivo. Furthermore, relatively few studies have examined the effects of stress and glucocorticoids on spines during the postnatal and adolescent period, which is characterized by rapid synaptogenesis followed by protracted synaptic pruning. To determine whether and to what extent glucocorticoids regulate dendritic spine development and plasticity, we used transcranial two-photon microscopy to track the formation and elimination of dendritic spines in vivo after treatment with glucocorticoids in developing and adult mice. Corticosterone, the principal murine glucocorticoid, had potent dose-dependent effects on dendritic spine dynamics, increasing spine turnover within several hours in the developing barrel cortex. The adult barrel cortex exhibited diminished baseline spine turnover rates, but these rates were also enhanced by corticosterone. Similar changes occurred in multiple cortical areas, suggesting a generalized effect. However, reducing endogenous glucocorticoid activity by dexamethasone suppression or corticosteroid receptor antagonists caused a substantial reduction in spine turnover rates, and the former was reversed by corticosterone replacement. Notably, we found that chronic glucocorticoid excess led to an abnormal loss of stable spines that were established early in life. Together, these findings establish a critical role for glucocorticoids in the development and maintenance of dendritic spines in the living cortex.
Prolonged, excessive glucocorticoid exposure has potent effects on the architecture of neuronal connectivity in diverse regions of the brain. Chronic stress paradigms and repeated glucocorticoid injections lead to dendritic branch atrophy and reduced spine density on the apical dendrites of hippocampal CA3 and medial prefrontal pyramidal cells (1–5) and parallel increases in orbitofrontal and basolateral amygdala cells (6–8). These structural alterations have been linked to learning and memory impairments and heightened anxiety in rats (6, 8–11), and they may contribute to cognitive deficits and affective symptoms in states of chronic stress and neuropsychiatric illness.
Studies in fixed tissue indicate that stress and glucocorticoid effects on spine density become detectable after 10–21 d (1–8). Although changes in spine density are not evident in rat hippocampus and amygdala 1 d after stress or glucocorticoid treatment (7, 12), others have reported a loss of dendritic spines in mouse CA3 hippocampal cells 5–6 h after restraint stress (13, 14) and an increase in spine elimination rates in hippocampal cell culture hours after treatment with corticotropin releasing hormone, an upstream regulator of glucocorticoids (13). These studies in fixed preparations and cell culture suggest that glucocorticoids are important for dendritic spine maintenance but do not provide information on the dynamics of spine formation and elimination in the living cortex. Thus, it remains unclear whether and over what time scale glucocorticoids affect the process of dendritic spine remodeling in vivo. It is also unknown whether the diminished spine densities observed after chronic glucocorticoid exposure are because of changes in spine formation, spine elimination, or some combination of these two factors. Furthermore, relatively few studies have examined the effects of stress or glucocorticoids on spine maturation during the critical postnatal and adolescent periods (15), which are characterized by rapid spinogenesis followed by a protracted process of spine pruning that culminates in the loss of up to 50% of synaptic connections (16–19). Therefore, it is unknown whether the effect of glucocorticoids on dendritic spine remodeling varies at different developmental stages across the lifespan.
To better understand the role of glucocorticoids in dendritic spine development and remodeling, we used transcranial, two-photon microscopy to track the formation and elimination of individual dendritic spines on layer V pyramidal neurons hours to days after treatment with glucocorticoids in developing [postnatal day (P) 21–30] and adult (~P120) mice (20, 21). We found that glucocorticoids enhance both spine formation and elimination rates in multiple cortical areas and are required for spine remodeling during both development and adulthood. Additionally, we found that chronic exposure to excessive levels of glucocorticoids leads to net spine loss, eliminating both new spines and stably maintained spines formed early in development.
Results
Glucocorticoids Enhance Dendritic Spine Remodeling in the Living Cortex.
Previous studies have shown that chronic glucocorticoid excess alters dendritic arbors and spine density in diverse cortical regions in fixed brain tissue after weeks of excess exposure (1–8). To determine whether and how glucocorticoids modulate the formation and elimination of dendritic spines in vivo, we used time-lapse, transcranial two-photon microscopy (20) to study spine turnover in barrel cortex after a single i.p. injection of corticosterone, the principal murine glucocorticoid. We repeatedly imaged the same YFP-labeled layer V pyramidal cells, tracking the spines of apical dendrites for hours to days after the initial injection (Fig. 1A). We found that a single corticosterone injection caused a dose-dependent (2.5 and 15 mg/kg) increase in spine formation and elimination in the barrel cortex of adolescent mice (P30) (Fig. 1 B and C and Table S1 shows statistics). Significant increases in spine turnover were detectable 5 h after a single injection and occurred independently of changes in filopodia, which exhibited higher baseline turnover rates that were not significantly modified by corticosterone (Fig. S1). These effects plateaued over a period of days, with additional daily injections eliciting marginal increases in spine turnover.
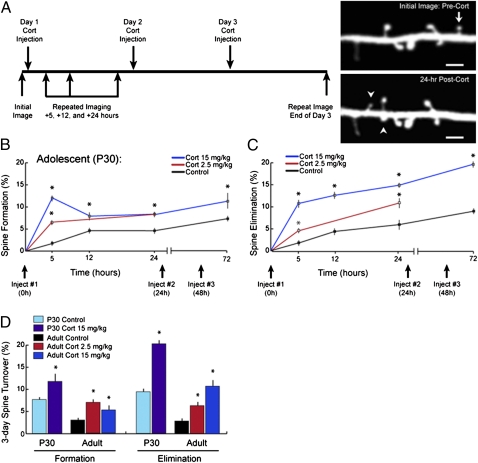
Glucocorticoids rapidly and potently enhance dendritic spine turnover in vivo. (A) Schematic depiction of experimental paradigm. Images of barrel cortex at P30 were obtained followed by a single i.p. injection of corticosterone (2.5 or 15 mg/kg). Eliminated (arrows) and formed (arrowheads) spines were identified by acquiring repeated images of the same dendritic segments over 24 h or after 3 d of daily corticosterone injections. The images depicted here were acquired from barrel cortex before and 24 h after corticosterone injection (15 mg/kg). (Scale bar: 2 μm.) (B) Corticosterone (2.5 and 15 mg/kg) increased spine formation in barrel cortex within hours in a dose-dependent manner. Significant effects were detectable 5 h after either dose. Comparable effects of smaller magnitude were observed after a 2.5-mg/kg injection. (C) Corticosterone rapidly increased spine elimination as well, with effects detectable 5 h after injection. Diminishing marginal effects occurred with additional injections over 3 d. (D) Corticosterone also increased spine elimination and formation rates over 3 d in adult mice (~5 mo old) in a dose-dependent manner, although plasticity was diminished in adults relative to P30 adolescents. Error bars = SEM. *Significantly different relative to corresponding control (P < 0.05). Tables S1–S3 show statistics and additional details.
Prior studies suggest that spine plasticity is reduced in adults and aged subjects relative to adolescents (19, 22–24). Consistent with these reports, we found that 72-h spine turnover rates were significantly diminished in adult mice relative to P30 adolescents (Fig. 1D). Like in P30 mice, corticosterone also increased spine formation and elimination rates in a dose-dependent manner in adults. Although the absolute increase in spine turnover was smaller in adulthood than adolescence (Fig. 1D), the relative changes were slightly larger, owing to the low baseline spine turnover in untreated adults (Fig. S2 and Tables S2 and S3 show statistics). These findings suggest that the adult brain retains a diminished capacity for spine plasticity under basal conditions and that glucocorticoids could significantly enhance this capacity.
Previous reports have examined the structural effects of glucocorticoids primarily in limbic brain regions. To better understand how glucocorticoids affect spine remodeling, we studied spine turnover in other cortical areas in P30 mice (Fig. 2A). Glucocorticoids enhanced elimination and formation rates in barrel cortex, primary motor cortex, and secondary motor cortex to a similar degree (Fig. 2 B and C), indicating a generalized modulation of cortical spine dynamics (Table S4 shows statistics). Together, these results show that glucocorticoids have potent effects on dendritic spine formation and elimination within a period of several hours in the living cortex.
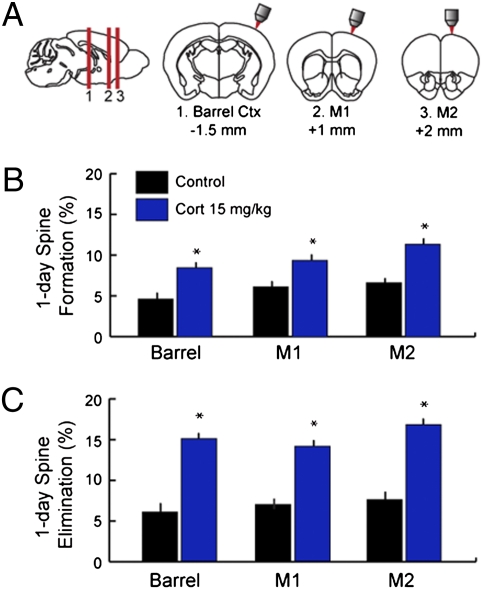
Glucocorticoids enhance spine turnover in multiple cortical regions. (A) Images were acquired from barrel cortex, primary motor cortex (M1), or secondary motor cortex (M2) immediately before and 24 h after treatment with corticosterone (15 mg/kg, i.p.) in adolescent (P30) mice. (B) Corticosterone increased 24-h spine formation rates to a comparable degree in all three regions studied. (C) Similar effects were observed for spine elimination rates over 24 h. Error bars = SEM. *Significantly different relative to corresponding control (P < 0.05). Table S4 shows statistics and additional details.
Endogenous Glucocorticoids Are Required for Spine Remodeling in the Developing Cortex.
The potent, generalized effects depicted in Figs. 1 and and22 raise the possibility that endogenous glucocorticoids play a critical role in regulating spine dynamics. To test this theory, we suppressed endogenous corticosterone release using repeated low-dose dexamethasone injections (100 μg/kg) to mimic adrenalectomy (Fig. 3A). Dexamethasone is a synthetic glucocorticoid that inhibits adrenal corticosterone synthesis by binding peripheral receptors in the anterior pituitary with high affinity (25, 26). Unlike corticosterone, dexamethasone does not appreciably penetrate the blood–brain barrier at low doses because of active transport out of the brain by the mdr1a P-glycoprotein (27, 28). Low-dose dexamethasone treatment is, therefore, used as a minimally invasive means of inducing glucocorticoid insufficiency in the central nervous system (25, 26, 29, 30).
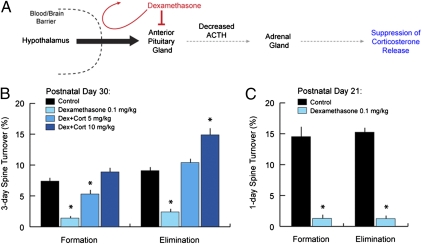
Glucocorticoid deprivation blocks dendritic spine remodeling in the developing cortex. (A) Dexamethasone suppression schematic. Dexamethasone is a synthetic glucocorticoid that does not appreciably penetrate the brain at low doses. Systemic administration of low-dose dexamethasone inhibits the anterior pituitary, inhibiting its production of adrenocorticotropic hormone (ACTH) and thereby suppressing corticosterone release from the adrenal gland. (B) Two times daily treatment with low-dose dexamethasone (0.1 mg/kg i.p.) for 3 d reduced spine turnover rates in P30 mice from 7–9% to ~2%. Spine turnover was restored by exogenous administration of corticosterone in a dose-dependent manner (5 and 10 mg/kg i.p. one time daily). (C) Spine turnover rates were ~15% over 1 d at P21. Low-dose dexamethasone reduced 1-d spine turnover rates to less than 2%. Error bars = SEM. *Significantly different relative to corresponding control (P < 0.05). Tables S5 and S6 show statistics and additional details.
We found that central glucocorticoid deprivation by low-dose dexamethasone prevented normal, physiological spine turnover in barrel cortex at P30, reducing elimination and formation rates from 7–9% to ~2% over 3 d (Fig. 3B and Tables S5 and S6 show statistics). Normal spine turnover was restored in a dose-dependent manner by coadministration with exogenous corticosterone (Fig. 3B), indicating a necessary role for glucocorticoids in generating spine remodeling during development. To further understand this effect, we studied it in P21 mice, which exhibit heightened spine plasticity, with turnover rates averaging 14–15% over 24 h. Remarkably, dexamethasone suppression reduced elimination and formation rates from 14–15% to less than 2% over 24 h (Fig. 3C).
It is well-established that corticosterone acts by binding two types of soluble receptors: the type I corticosteroid or mineralocorticoid receptor (MR; named for another ligand) and the type II corticosteroid or glucocorticoid receptor (GR) (25). We found that treatment with either receptor antagonist alone significantly disrupted spine dynamics in P30 mice (Fig. 4 A and B and Table S7 shows statistics). Spironolactone (20 mg/kg i.p.), an MR antagonist, reduced elimination and formation rates to ~1% over 24 h, significantly less than the 5–6% observed in vehicle-treated controls. Mifeprisone (20 mg/kg i.p.), a GR-selective antagonist, reduced formation rates but had no effect on elimination. Again, spine formation rates were ~1% over 24 h. Coadministration of corticosterone (15 mg/kg) and either receptor antagonist blocked corticosterone effects on spine turnover in a similar manner (Fig. 4 A and B). Together, these data provide strong support for a necessary, permissive role for endogenous glucocorticoids in regulating dendritic spine dynamics, with spine formation requiring activation of both receptor pathways.
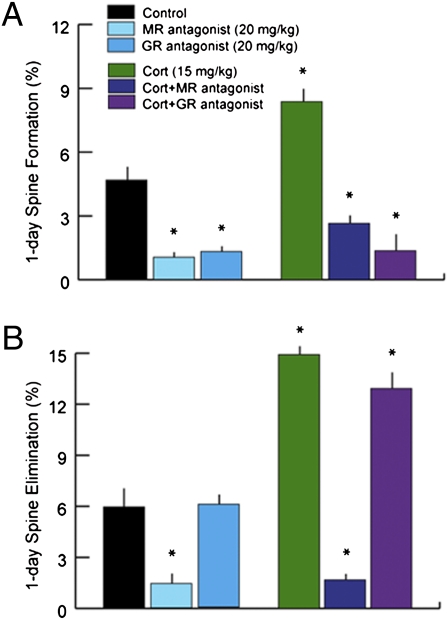
Corticosteroid receptor antagonists disrupt spine dynamics. (A) Corticosterone binds two types of corticosteroid receptors known as the MR and GR. Treatment with an MR antagonist alone (spironolactone; 20 mg/kg i.p.) reduced spine formation from 4.6% to 1.0% over 24 h. Treatment with a selective GR antagonist (mifepristone; 20 mg/kg i.p.) had an equivalent effect. Coadministration of corticosterone and either antagonist blocked the enhancing effect of corticosterone on spine formation rates. (B) The MR antagonist also reduced 24-h spine elimination to less than 2% and interfered with the enhancing effect of corticosterone. The GR antagonist had no significant effect on spine elimination rates. Error bars = SEM. *Significantly different relative to corresponding control (P < 0.05). Table S7 shows statistics and additional details.
Chronic Glucocorticoid Excess Leads to Loss of Spines Formed Early in Development.
The results above indicate that glucocorticoids have potent but diminishing marginal effects on spine elimination over a period of hours to days, and therefore, most spines are stably maintained after brief exposures to glucocorticoids. Previous work has shown that chronic glucocorticoid excess for 10–21 d reduces spine density by as much as 20% in the hippocampus and medial prefrontal cortex, shrinks dendritic arbors, and impairs associated memory processes (1–11). Thus, brief and chronic exposure to excessive glucocorticoids may have distinct effects on the processes of dendritic spine formation and elimination in the cortex. Recent studies indicate that new spines are more prone to elimination than spines that have been maintained in circuits for many days (19, 24). This finding suggests that older spines may be differentially susceptible to elimination by brief and chronic glucocorticoid exposure. To address this question, we acquired images of barrel cortex on P23 and P30 to differentiate new spines that formed after P23 and spines that formed early in life (before P23) and persisted for at least 1 wk at P30 (Fig. 5A). A single high-dose corticosterone injection (15 mg/kg) on P30 enhanced both spine formation and elimination (Fig. 5B), with the latter increasing from 7.0% in the control group to 12.4% in the corticosterone-treated group over 24 h (Tables S8 and S9 show statistics). However, this injection affected primarily recently formed spines: elimination rates increased from 30.3% to 59.8% over 24 h in the pool of recently formed spines (Fig. 5C) but did not differ significantly from controls for older spines (Fig. 5D). In both groups, a disproportionate majority of eliminated spines were new spines, present on P30 but not on P23, and the vast majority (> 95%) of old spines established before P23 were spared.
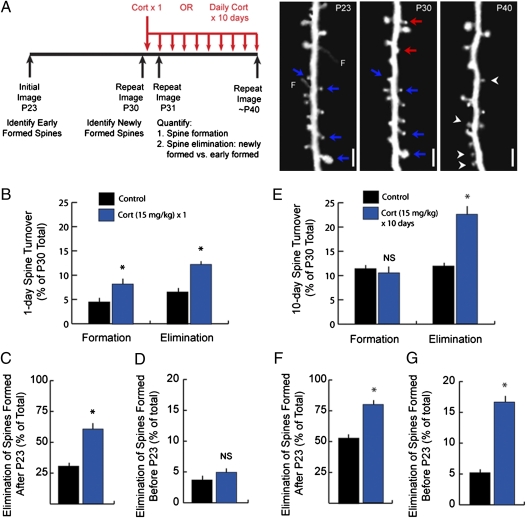
Chronic glucocorticoid excess leads to loss of spines formed early in development. (A) In one experiment, subjects were imaged at P23 and P30, and then, they were reimaged on P31 at 24 h after a single injection of corticosterone (15 mg/kg) or vehicle. Spine formation and elimination were quantified for the 24-h interval after corticosterone treatment as previously described. In a second experiment, subjects were again imaged at P23 and P30, and then, they were reimaged at P40 after 10 d of daily injections of corticosterone (15 mg/kg) or vehicle. Spine formation (arrowheads) and elimination (arrows) were quantified over the 10-d period from P30 to P40. In both experiments, eliminated spines that were formed early in development before P23 (blue arrows) were distinguished from those spines that were formed after P23 (red arrows) and were absent in the initial image. Right depicts a typical quantification for the chronic glucocorticoid treatment. Note that, to avoid overestimating the effect on spines established early in development, eliminated spines were considered recently formed if a filopodium (F) but not a mature spine was observed at P23. (Scale bar: 2 μm.) (B) A single corticosterone injection significantly increased formation and elimination of spines over 24 h. (C) Accordingly, corticosterone significantly increased the elimination of younger spines that were formed after P23 and were absent from the initial image. (D) In contrast, a single corticosterone injection had no significant effect on spines established early in development, before P23. (E) Chronic glucocorticoid exposure increased elimination rates but had no significant effect on formation. (F) Chronic corticosterone treatment significantly increased the elimination of spines formed after P23, eliminating 78.6% of this group. (G) Spines formed early in development were also significantly affected, with 10-d elimination rates increasing threefold for these spines. Error bars = SEM. *Significantly different relative to corresponding control (P < 0.05). Tables S8 and S9 show statistics and additional details.
In contrast, chronic corticosterone treatment did not spare older spines. Here, we acquired images on P23 and P30, and then, we initiated a 10-d course of daily injections of corticosterone (15 mg/kg) or vehicle and reimaged the same spines on day 40 (Fig. 5A). Repeated daily corticosterone increased spine elimination from 12.1% to 22.7% over 10 d; formation was not significantly affected (Fig. 5F). Importantly, the chronic corticosterone treatment increased the elimination of both young spines that formed after P23 (Fig. 5F) as well as older spines that formed before P23 (Fig. 5G). Elimination rates for these older spines increased more than threefold (16.5% vs. 5.1% in vehicle-treated controls). A majority (65.0%) of the spines eliminated after prolonged exposure were older spines that were formed early during development. In contrast, a single glucocorticoid injection did not affect older spines (Fig. 5D), consistent with previous studies showing that the vast majority of spines that have persisted for at least 1 wk will remain stable for many months thereafter (24). Thus, transient and chronic glucocorticoid exposures have distinct effects on dendritic spine dynamics and the stability of cortical connections; both increase spine elimination rates, but only the latter has a significant effect on the maintenance of stable spines established early during cortical development.
Discussion
It was once widely assumed that the brain is impervious to the influence of environmental stressors. This assumption changed with the discovery over 40 y ago of hippocampal glucocorticoid receptors mediating neurochemical and behavioral adaptations to stressors and the peripheral neuroendocrine response that they elicit (31, 32). Subsequent work has shown that chronic stress and prolonged glucocorticoid exposure have potent effects on the architecture of cortical connectivity, particularly in brain regions that modulate the hypothalamic/pituitary/adrenal (HPA) response to stress, and have been implicated in neuropsychiatric illness (1–8). Here, we have shown that glucocorticoids play an unexpectedly general and necessary role in generating dendritic spine plasticity in multiple areas of cortex in vivo in the adolescent and adult brain alike. This report examines glucocorticoid effects on the dynamics of dendritic spine remodeling in the living cortex during postnatal and adolescent development. Remarkably, glucocorticoid deprivation by dexamethasone suppression or treatment with exogenous corticosteroid antagonists reduced spine turnover rates from 15% to less than 2% in the cortex at P21, supporting a critical and necessary role for glucocorticoids in establishing normal patterns of synaptic connectivity in the developing cortex.
Notably, the observed effects were smaller in adults. Baseline turnover rates over 3 d were ~3% in adults vs. 7–9% in adolescents. This result is consistent with prior studies that suggest a markedly diminished capacity for spine remodeling in adults (22–24). However, this capacity could still be enhanced by glucocorticoids in adulthood. Daily low-dose corticosterone injections (2.5 mg/kg) were associated with a twofold increase in spine turnover (Fig. 1D). Because the effects on elimination and formation were comparable, there was no net change in spine density at this dose, a finding in agreement with fixed tissue studies of spine density changes after chronic stress in aged adults (23). With greater glucocorticoid exposure (15 mg/kg), elimination rates increased to 10% over 3 d and exceeded spine formation rates, suggesting that chronic, high-dose glucocorticoid exposure may lead to spine loss in adults as well. Together, our results confirm that spine turnover is reduced in adulthood, but they also show that the adult brain retains a substantial capacity for spine remodeling, which was, in turn, enhanced by glucocorticoids.
Previous studies have linked chronic stress and excess glucocorticoid exposure to changes in spine density and dendritic arborization in brain regions that are known regulators of the HPA axis, including the hippocampus, medial prefrontal cortex, and amygdala (1–8). Our results show that glucocorticoid effects on spine dynamics also occur in primary somatosensory cortex as well as primary and secondary motor cortex—areas that are devoted to sensorimotor processing and are not directly linked to HPA axis regulation. Importantly, chronic glucocorticoid exposure disrupts cortical connections in a manner that is distinct from the remodeling observed acutely after a transient exposure, which preserves the vast majority of established spines. In contrast, prolonged exposure disrupted stably maintained spines that were established early in life (Fig. 5). These findings underscore the importance of maintaining existing synaptic connections in the healthy brain and suggest that abnormal loss of synaptic connections established during early development may contribute in unexpected ways to pathophysiology in neuropsychiatric illness.
Glucocorticoids have been shown to regulate multiple genes implicated in spine plasticity after chronic glucocorticoid exposure, causing changes in extracellular glutamate, calcium currents, and expression of adhesion molecules and NMDA and GABA receptors as well as altering the rho-kinase/LIM kinase/cofilin signaling pathway and other pertinent signaling activity (26, 33–37). Glucocorticoids also have complex effects on sleep architecture, tending to increase rapid eye movement sleep and decrease slow wave sleep with chronic exposure (38–40). Sleep and prolonged wakefulness, in turn, are associated with contrasting changes in the expression of genes associated with cortical plasticity (41, 42). Future studies are needed to address whether glucocorticoids regulate spine plasticity through localized effects on cortical pyramidal cells, interneurons, or glial cells—all of which express corticosteroid receptors (43, 44)—or through generalized effects on global patterns of neural activity.
Materials and Methods
Experimental Animals.
Mice expressing YFP in pyramidal cells predominantly in cortical layer 5 were purchased from Jackson Laboratory (YFP-H line) and group-housed with a 12-h light to dark cycle (lights on at 7:00 AM and lights off at 7:00 PM) in the Skirball Institute Central Animal Facility at New York University. Most experiments involved mice at P30, but younger mice (P21) and adult mice (~P120) were used in some experiments as described in the text. They were afforded ad libitum access to food and water for all experiments. All experimental protocols were conducted in accordance with institutional guidelines.
In Vivo Transcranial Two-Photon Imaging.
This procedure has been described in detail elsewhere (20). In brief, surgical anesthesia was achieved with an i.p. injection (5–6 μL/g) of a mixture of ketamine (20 mg/mL) and xylazine (3 mg/mL). A midline incision of the scalp exposed the periosteum, which was manually removed with a microsurgical blade. The area to be imaged was identified in terms of stereotactic coordinates: 1.1 mm posterior to Bregma and 3.4 mm lateral from midline for barrel cortex, 1.3 mm anterior and 1.2 mm lateral for primary motor cortex, and 2.0 mm anterior and 1.0 mm lateral for secondary motor cortex. These coordinates were confirmed to be within the specified regions in previous reports (45, 46). The head was then immobilized, and a high-speed microdrill and microsurgical blade were used to thin a circular area of skull over the area of interest to a thickness of ~20 μm. This procedure was performed under a dissection microscope. Image stacks of dendritic segments projecting to superficial cortical layers were obtained using a two-photon microscope tuned to 920 nm with a 60× objective immersed in an artificial cerebrospinal fluid solution and a 3× digital zoom yielding high-magnification images suitable for quantification of dendritic spines. For repeated imaging over intervals of hours to weeks, the procedure above was repeated, and localization of the same region was facilitated by low-magnification images stacks at 1× digital zoom and with reference to vascular landmarks in light microscopic photographs of the thinned area of skull.
Manipulations of Glucocorticoid Activity.
All of the reagents described below are commercially available from Sigma Aldrich (www.sigmaaldrich.com). Corticosterone and various synthetic glucocorticoid receptor modulators were injected i.p. in a DMSO vehicle; doses and timing are described above. Where relevant, vehicle-treated control animals were injected with DMSO alone. Corticosterone was administered at doses of 15 mg/kg to facilitate comparisons with the relatively high doses used in much of the existing literature (4, 47) and at a lower dose of 2.5 mg/kg to yield an exposure that would be more comparable with the exposure observed after a stressor. It is worth noting that doses in this range have been found to influence memory as well (48).
Dexamethasone suppression of endogenous glucocorticoids.
Dexamethasone is a synthetic glucocorticoid that suppresses endogenous corticosterone synthesis by inhibiting the anterior pituitary gland, but it does not cross the blood–brain barrier at low doses (26–28, 49). Accordingly, low-dose dexamethasone (100 μg/kg i.p.) was administered approximately 2 h before the initial imaging session to study the effects of suppressing endogenous glucocorticoid activity in the brain. Repeated doses were administered every 12 h for 24 (Fig. 3C) or 72 h (Fig. 3B). This dose has been shown to reduce plasma corticosterone to undetectable levels within hours (50). In related experiments, dexamethasone was coadministered with corticosterone at varying doses to study the reversibility of these effects by exogenous glucocorticoids. In these experiments, dexamethasone was administered every 12 h to maintain the suppression of endogenous glucocorticoid activity, whereas corticosterone was administered one time every 24 h.
Corticosteroid receptor studies.
Corticosterone at physiological concentrations binds two receptors: the type I (MR) and type II (GR) corticosteroid receptors. To dissociate these effects, spironolactone (20 mg/kg i.p.) and mifepristone (20 mg/kg i.p., formerly known as RU-486) were used to selectively antagonize MR and GR, respectively, with doses selected on the basis of previously published reports (51–53).
Data Analysis.
The procedure for quantifying spine dynamics has been described elsewhere (24). In brief, image stacks were analyzed using the freely available ImageJ software (rsbweb.nih.gov/ij/). For each dendritic segment analyzed, filopodia were identified as long, thin protrusions with a ratio of length to neck diameter >3:1. Corticosterone effects on filopodia were analyzed separately (Fig. S1). The remaining protrusions were classified as spines. Raters blinded to experimental condition compared pairs of images of the same dendritic segment and identified stable spines (present in images 1 and 2), eliminated spines (present in image 1 but not in image 2), and formed spines (present in image 2 but not in image 1). In some experiments, the age of eliminated spines was estimated by examining an image obtained before the pair in question (Fig. 5). Between group differences in spine elimination rates and formation rates were tested using one-way ANOVA and posthoc t tests. All statistical analyses were performed using the SPSS statistical software package.
Acknowledgments
We thank Gan Laboratory members for critical comments on this manuscript. C.L. is supported by a DeWitt Wallace Reader's Digest Fellowship and the Cornell Department of Psychiatry. Funding for this work was provided by National Institutes of Health Grant NS047325 (to W.-B.G.).
Footnotes
The authors declare no conflict of interest.
This article is a PNAS Direct Submission.
This article contains supporting information online at www.pnas.org/lookup/suppl/10.1073/pnas.1110444108/-/DCSupplemental.
References
Articles from Proceedings of the National Academy of Sciences of the United States of America are provided here courtesy of National Academy of Sciences
Full text links
Read article at publisher's site: https://doi.org/10.1073/pnas.1110444108
Read article for free, from open access legal sources, via Unpaywall:
https://www.pnas.org/content/pnas/108/38/16074.full.pdf
Citations & impact
Impact metrics
Citations of article over time
Alternative metrics
Smart citations by scite.ai
Explore citation contexts and check if this article has been
supported or disputed.
https://scite.ai/reports/10.1073/pnas.1110444108
Article citations
Complement and microglia activation mediate stress-induced synapse loss in layer 2/3 of the medial prefrontal cortex in male mice.
Nat Commun, 15(1):9803, 12 Nov 2024
Cited by: 0 articles | PMID: 39532876 | PMCID: PMC11557709
Effects of chronic stress on cognitive function - From neurobiology to intervention.
Neurobiol Stress, 33:100670, 02 Sep 2024
Cited by: 0 articles | PMID: 39295772 | PMCID: PMC11407068
Review Free full text in Europe PMC
Cocaine disrupts action flexibility via glucocorticoid receptors.
iScience, 27(7):110148, 28 May 2024
Cited by: 0 articles | PMID: 38989467 | PMCID: PMC11233908
Altered projection-specific synaptic remodeling and its modification by oxytocin in an idiopathic autism marmoset model.
Commun Biol, 7(1):642, 27 May 2024
Cited by: 0 articles | PMID: 38802535 | PMCID: PMC11130163
Early-life maturation of the somatosensory cortex: sensory experience and beyond.
Front Neural Circuits, 18:1430783, 08 Jul 2024
Cited by: 0 articles | PMID: 39040685 | PMCID: PMC11260818
Review Free full text in Europe PMC
Go to all (201) article citations
Data
Data behind the article
This data has been text mined from the article, or deposited into data resources.
BioStudies: supplemental material and supporting data
Similar Articles
To arrive at the top five similar articles we use a word-weighted algorithm to compare words from the Title and Abstract of each citation.
Glucocorticoid receptors modulate dendritic spine plasticity and microglia activity in an animal model of Alzheimer's disease.
Neurobiol Dis, 132:104568, 05 Aug 2019
Cited by: 33 articles | PMID: 31394203
Pyramidal Neurons in Different Cortical Layers Exhibit Distinct Dynamics and Plasticity of Apical Dendritic Spines.
Front Neural Circuits, 11:43, 19 Jun 2017
Cited by: 30 articles | PMID: 28674487 | PMCID: PMC5474458
Glucocorticoid and β-adrenergic regulation of hippocampal dendritic spines.
J Neuroendocrinol, 32(1):e12811, 05 Dec 2019
Cited by: 6 articles | PMID: 31715030 | PMCID: PMC7003927
Rapid nongenomic modulation by neurosteroids of dendritic spines in the hippocampus: Androgen, oestrogen and corticosteroid.
J Neuroendocrinol, 30(2), 01 Feb 2018
Cited by: 18 articles | PMID: 29194818
Review
Funding
Funders who supported this work.
NINDS NIH HHS (2)
Grant ID: NS047325
Grant ID: R01 NS047325