Abstract
Free full text

Rad51 and BRCA2 - New Molecular Targets for Sensitizing Glioma Cells to Alkylating Anticancer Drugs
Abstract
First line chemotherapeutics for brain tumors (malignant gliomas) are alkylating agents such as temozolomide and nimustine. Despite growing knowledge of how these agents work, patients suffering from this malignancy still face a dismal prognosis. Alkylating agents target DNA, forming the killing lesion O6-alkylguanine, which is converted into DNA double-strand breaks (DSBs) that trigger apoptosis. Here we assessed whether inhibiting repair of DSBs by homologous recombination (HR) or non-homologous end joining (NHEJ) is a reasonable strategy for sensitizing glioma cells to alkylating agents. For down-regulation of HR in glioma cells, we used an interference RNA (iRNA) approach targeting Rad51 and BRCA2, and for NHEJ we employed the DNA-PK inhibitor NU7026. We also assessed whether inhibition of poly(ADP)ribosyltransferase (PARP) by olaparib would enhance the killing effect. The data show that knockdown of Rad51 or BRCA2 greatly sensitizes cells to DSBs and the induction of cell death following temozolomide and nimustine (ACNU). It did not sensitize to ionizing radiation (IR). The expression of O6-methylguanine-DNA methyltransferase (MGMT) abolished all these effects, indicating that O6-alkylguanine induced by these drugs is the primary lesion responsible for the formation of DSBs and increased sensitivity of glioma cells following knockdown of Rad51 and BRCA2. Inhibition of DNA-PK only slightly sensitized to temozolomide whereas a significant effect was observed with IR. A triple strategy including siRNA and the PARP inhibitor olaparib further improved the killing effect of temozolomide. The data provides evidence that down-regulation of Rad51 or BRCA2 is a reasonable strategy for sensitizing glioma cells to killing by O6-alkylating anti-cancer drugs. The data also provide proof of principle that a triple strategy involving down-regulation of HR, PARP inhibition and MGMT depletion may greatly enhance the therapeutic effect of temozolomide.
Introduction
Glioblastoma multiforme (GBM, WHO grade IV) is the deadliest form of malignant brain tumors. Complete surgical resection of this tumor is hampered by its inherent invasiveness into the surrounding healthy brain tissue. As the tumor cannot be removed completely, adjuvant chemo-radiotherapy plays a major role in the treatment of patients. Nevertheless, despite the best available therapeutic approach, the survival rate for patients with this malignancy is below 1.5 years after diagnosis [1]. Temozolomide (TMZ) is the current first-line chemotherapeutic for gliomas. Similar to other methylating chemotherapeutics such as procarbazine, dacarbazine and streptozotocine, TMZ-induced cell-kill is mainly due to O6-methylguanine (O6MeG), which is a minor lesion induced by these agents in DNA. The suicide enzyme O6-methylguanine-DNA methyltransferase (MGMT) repairs O6MeG and thereby renders cells resistant to methylating agents [2]. Consequently, MGMT activity and the promoter methylation status of the MGMT gene (indicators of O6MeG repair capacity and MGMT protein expression, respectively) are used as predictive markers for the response of gliomas to TMZ [3], [4]. As a result of the protective role of MGMT in methylating and chloroethylating agent based therapy, MGMT inhibitors are in trials for use in MGMT expressing tumors [5].
O6MeG is processed into DNA double-strand breaks (DSBs) in a DNA mismatch repair (MMR)-dependent manner, which requires two rounds of DNA replication [6], [7], [8], [9]. These DSBs then trigger apoptotic cell death in gliomas [10]. For chloroethylating agents such as nimustine (ACNU), O6-chloroethylguanine forms secondary interstrand crosslinks that in turn give rise to DSBs (for review see [2]). Cells can protect against DSBs via two repair pathways, namely non-homologous end joining (NHEJ) and homologous recombination (HR).
NHEJ is a error-prone process that relies on the coordinated action of Ku70/Ku80, DNA-PKcs, Artemis, XRCC4 and DNA ligase IV, among other factors, to rejoin the two ends of a broken DNA molecule [11], [12]. HR employs sequence homology to perform an error-free break correction that preserves the original DNA sequence. The central reaction of the HR pathway, namely the homology search and strand invasion, is performed by Rad51-coated 3′- single stranded DNA (ssDNA) tails generated by DNA end resection of the break [13], [14]. The formation of this nucleoprotein filament at ssDNA is promoted and stabilized by BRCA2 [15], [16]. Both Rad51 and BRCA2 are essential for HR in mammalian cells. A significant function of Rad51 and BRCA2 in other repair pathways has not been described.
Working with rodent cells mutated in these DSB repair pathways, we were able to show that HR, but not NHEJ, is responsible for resistance to DSBs formed in response to TMZ-induced O6MeG [17]. Similar data were obtained for cells treated with chloroethylating agents like ACNU and lomustine (CCNU), which are also used in glioma therapy (Nikolova et al., unpublished data). These findings suggest that HR might be a promising target in methylating and chloroethylating agent based glioma therapy.
This work was aimed at assessing whether knockdown of HR in glioma cells by interference RNA (iRNA) leads to improved killing of glioma cells following treatment with TMZ, ACNU and ionizing radiation (IR), all part of the standard care for patients with this malignancy.
Results and Discussion
Knockdown of the homologous recombination proteins Rad51 and BRCA2 sensitizes glioma cells to alkylating anti-cancer drugs
O6-alkylating agents (O6AA) are widely used as standalone agents or as part of regimes for the treatment of glioma, malignant melanoma, Hodgkin's and non-Hodgkin lymphoma, sarcoma and islet cell carcinoma of the pancreas. To determine whether down-regulation of HR would lead to increased sensitivity of glioma cells to O6AA, stable and transient iRNA transfections targeting HR proteins were performed in these cells. LN-229 glioma cells stably knocked-down for Rad51 (Fig. 1A demonstrating parental cells and four stable Rad51sh transfectants), which is the protein responsible for homology search and strand invasion, showed a profound increase in both TMZ (Fig. 1B) and ACNU (Fig. 1C) induced cell death. Remarkably, this increase in sensitivity was proportional to the increase in Rad51 knockdown (Fig. 1D; a similar quantitative correlation was found for ACNU (not shown)). One may suppose that knockdown of Rad51 causes a general sensitization that pertains to all genotoxins including IR. To determine whether this is the case, LN-229 Rad51 knockdown cells were exposed to increasing doses of γ-radiation, which contrary to O6AA induce DSBs directly. A slight increase in sensitivity in the Rad51 knockdown lines compared to the control line was observed (Fig. 1E), although not as dramatic as the sensitization towards O6AA (compare with Fig. 1B and 1C).
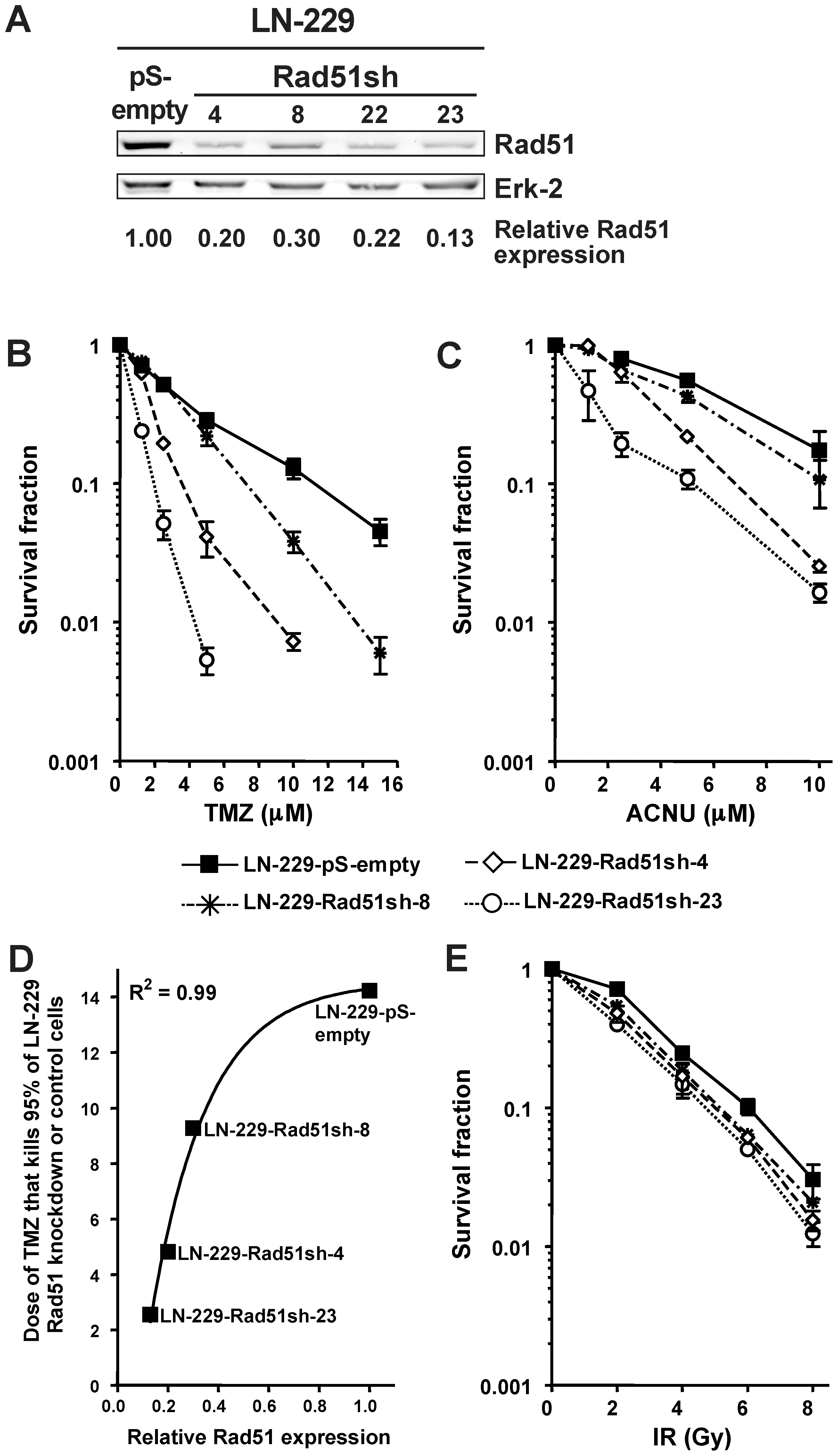
(A) Rad51 protein expression in knockdown clones and control assessed by western blot, quantified, corrected for loading (Erk-2) and expressed relative to control. (B) Clonogenic survival for stable Rad51 knockdown glioma cells (LN229-Rad51sh) compared to empty vector transfected cells (LN-229-pS-empty) following TMZ treatment. (C) Clonogenic survival for stable Rad51 knockdown glioma cells compared to empty vector transfected cells following ACNU treatment. (D) Correlation between the relative Rad51 expression, determined from A, and TMZ concentration that kills 95% of glioma cells, determined from B. Line was fitted using the equation y=
minimum + (maximum-minimum)×(1-exp(-kx)). (E) Clonogenic survival for stable Rad51 knockdown glioma cells compared to empty vector transfected cells following ionizing radiation (IR).
To determine whether the sensitization of glioma cells to O6AA chemotherapeutics is not exclusive for Rad51 knockdown, BRCA2, the scaffold protein that promotes and stabilizes the presynaptic filament formation during HR repair, was transiently down-regulated (Fig. 2A). Similar to Rad51, BRCA2 knockdown also caused sensitization of glioma cells to TMZ and ACNU (Fig. 2B and 2C). Again, for ionizing radiation the increase in cell kill after BRCA2 knockdown was less prominent (Fig. 2D). These results suggest that HR plays a protective role in glioma cells treated with TMZ or ACNU and that saturation of HR must occur before O6AA chemotherapeutics trigger cell death.
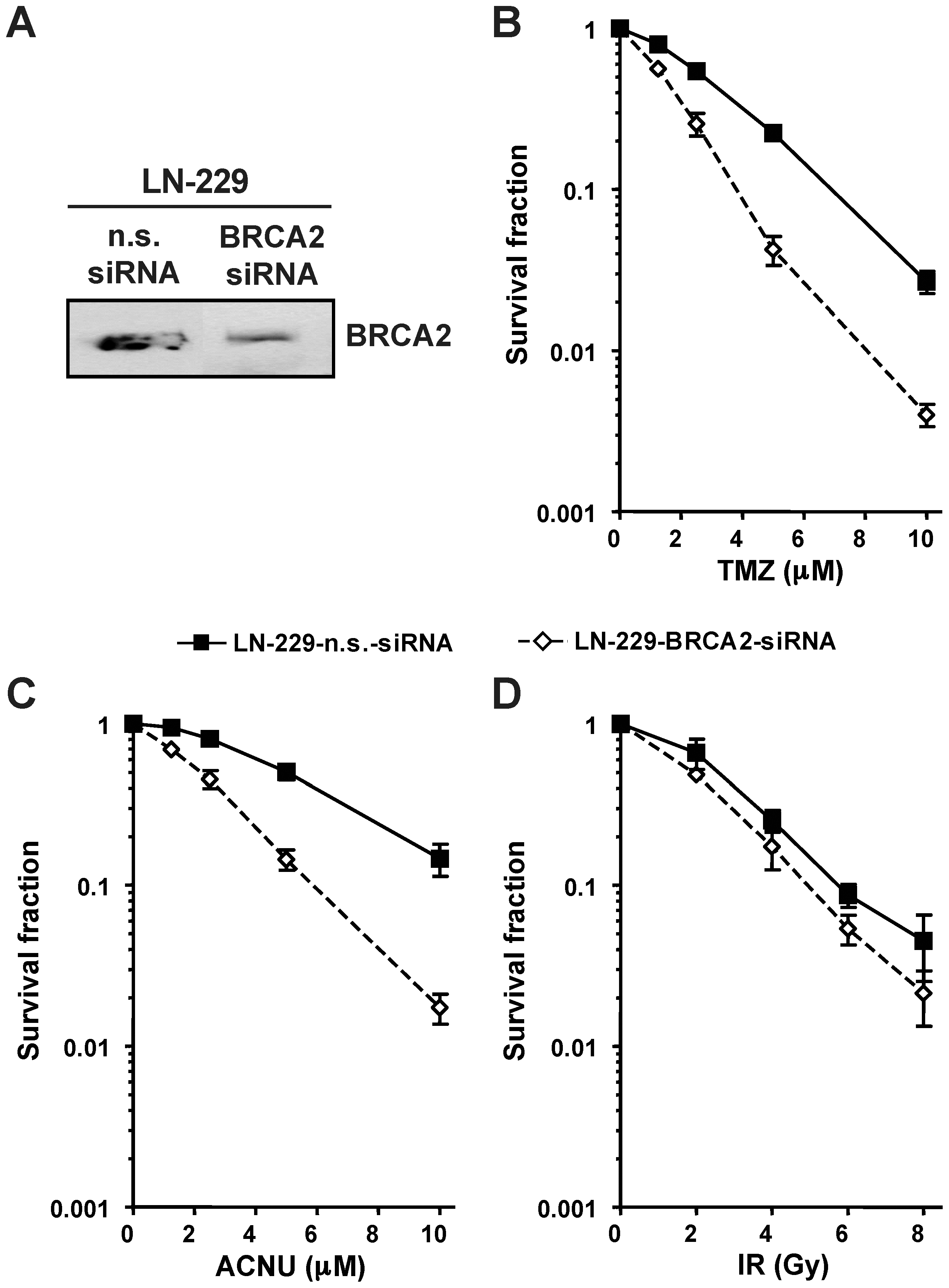
(A) BRCA2 protein expression following knockdown was assessed by western blot. (B) Clonogenic survival following TMZ treatments in transient BRCA2 or non-sense (n.s.) siRNA transfected glioma cells. (C) Clonogenic survival following ACNU treatments in transient BRCA2 or non-sense (n.s.) siRNA transfected glioma cells. (D) Clonogenic survival following ionizing radiation (IR) in transient BRCA2 or non-sense (n.s.) siRNA transfected glioma cells.
Cell death in stable Rad51 down-regulated cells showed a strong increase in apoptosis following TMZ treatment compared to control as determined by annexin V/PI flow cytometry (Fig. 3A). This is in line with previous data obtained with glioma cells showing that apoptosis is the major cell death pathway following TMZ [10]. If Rad51 represents a general mechanism for TMZ resistance in glioma cells, then knockdown of Rad51 in other glioma cells should also lead to increased sensitivity towards TMZ triggered apoptosis. To prove this, Rad51 was transiently knocked-down in the glioma cell lines U87MG and T98G (Fig. 3B). TMZ induced apoptosis was determined in these cells and compared to the response of LN-229 cells transfected with the pS-empty and Rad51sh vector (Fig. 3C). We should note that for the cell line T98G, MGMT was depleted by O6BG, while LN-229 and U87MG does not express MGMT and therefore O6BG pretreatment was not necessary. All three cell lines showed a significant increase in TMZ triggered apoptosis following Rad51 knockdown (Fig. 3C). These results support the view that the chemo-sensitizing effect of HR down-regulation is a general phenomenon that is not restricted to a particular glioma cell line.
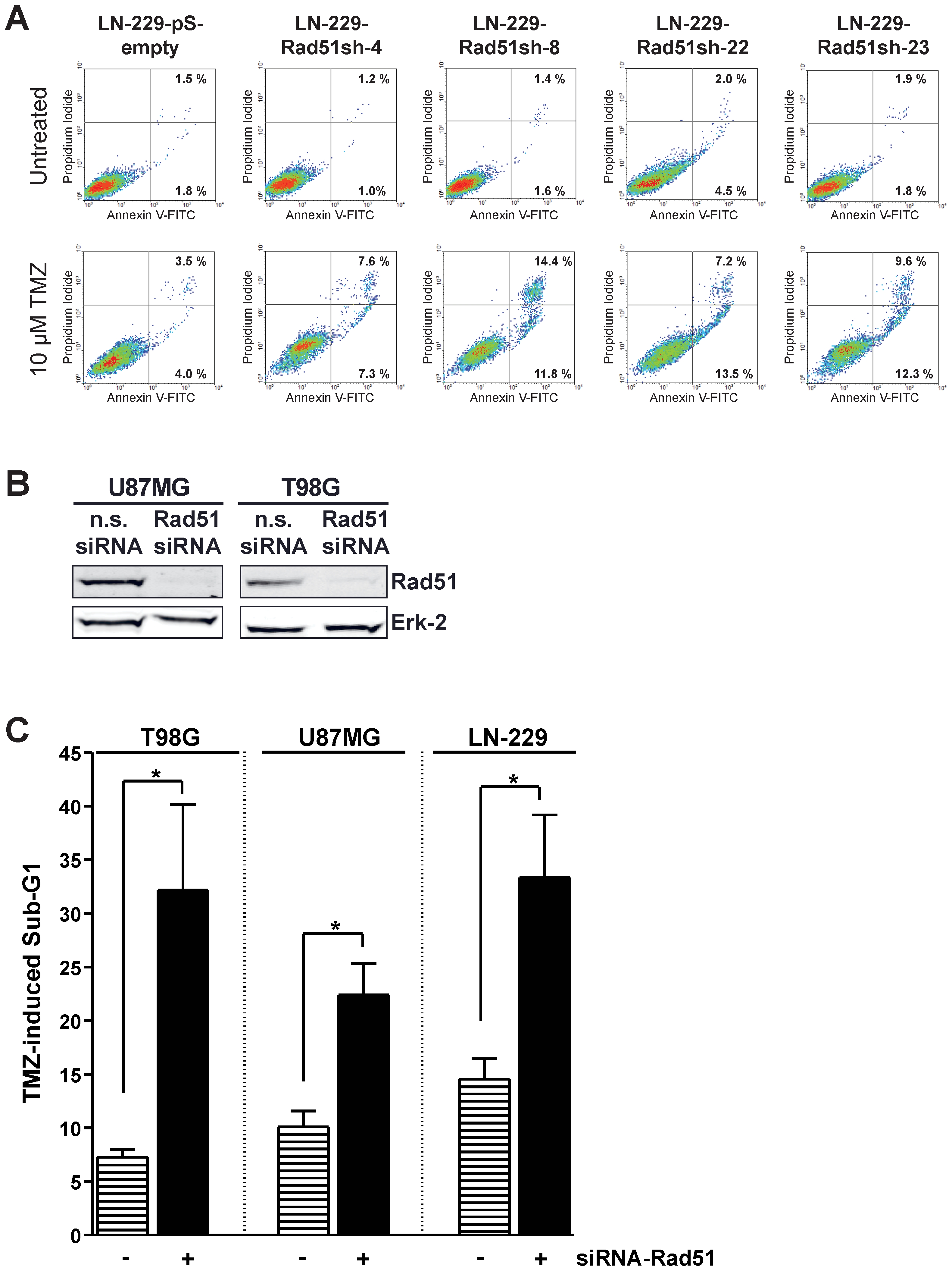
(A) Apoptosis determined by annexin V/PI double-staining 144 h after 10 µM TMZ in stable Rad51 knockdown clones of the cell line LN229. (B) Western blot analysis of transient Rad51 knockdown in U87MG and T98G cells, or transfected with non-sense siRNA. Erk-2 was used as loading control. (C) Apoptosis induction after TMZ treatment in Rad51 transient knocked-down glioma cells U87MG and T98G, as well as in the stable Rad51 knockdown clone LN-229-Rad51-sh8 and the empty vector control cell line LN-229-pS-empty. Apoptosis was assessed by Sub-G1 analysis performed 144 h after 10 µM TMZ treatment. For MGMT depletion 10 µM O6BG was added 1 h before TMZ. * p<0.05, significance level determined using the t-student test (n=
3).
Sensitization of Rad51 knockdown depends on O6-alkylguanine lesions
TMZ methylates DNA at 13 positions [18]. Prominent alkylation lesions are N7-methylguanine, N3-methyladenine, N3-methylguanine and O6-methylguanine. In rodent cells, HR has been reported to be the major downstream protection mechanism against both N-alkylations in purines [19] and O6-alkylations in guanine [17]. The TMZ-induced adduct O6MeG is repaired by MGMT [2]; consequently, over-expression of MGMT in HR knockdown glioma cells should render them resistant to TMZ if HR knockdown sensitizes towards the O6MeG adduct. LN-229 does not express MGMT detectably. Therefore, we transfected MGMT into these cells generating stable MGMT transfectants (Fig. 4A). As expected, these cells were highly protected against TMZ-induced cell death. Knockdown of Rad51 in these cells had no significant effect on TMZ-induced toxicity (Fig. 4B, compare with Fig. 1B). The data shows that blocking the HR repair pathway by Rad51 knockdown sensitizes to cell death triggered by the DNA adduct O6MeG.
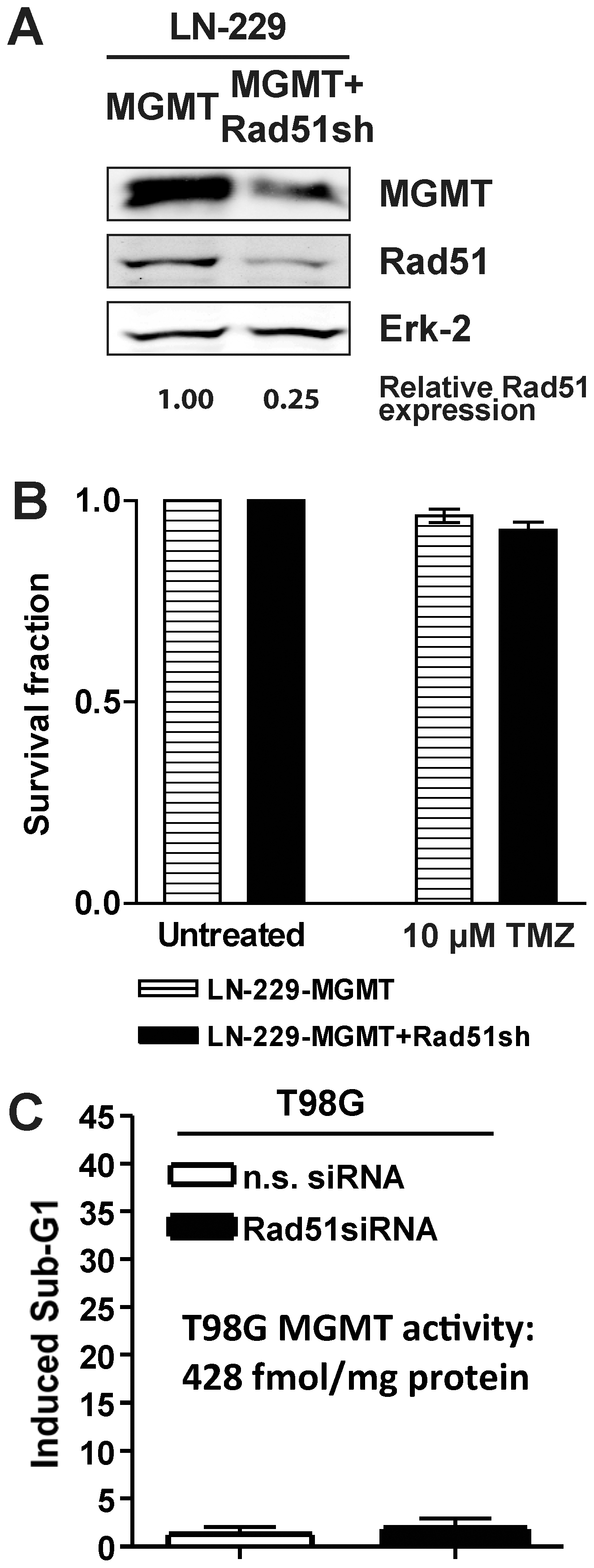
(A) Western blot analysis of MGMT and Rad51 protein levels of glioma cells stably transfected to express MGMT (LN-229-MGMT) and cells stably expressing MGMT and knockdown for Rad51 (LN-229-MGMT+Rad51sh). Erk-2 was used for loading control. (B) Clonogenic survival for cells stably transfected to express MGMT (LN-229-MGMT) and cells stably expressing MGMT and knockdown for Rad51 (LN-229-MGMT+Rad51sh) after TMZ treatment. (C) Apoptosis in T98G glioma cells that were transiently knocked-down for Rad51 (or transfected with non-sense siRNA) treated with TMZ. Apoptosis was determined by Sub-G1 flow cytometry 144 h after TMZ addition.
Although brain tumors usually express low levels of MGMT [20], a major fraction of these malignancies do indeed show clinically relevant expression levels of this repair enzyme, making them unsuitable for treatment with O6AA. To overcome this problem, MGMT inhibitors are being tested in trials for enhancing the effectiveness of TMZ and related agents. Employing a model that resembles this situation, T98G glioma cells that express endogenous MGMT were knocked-down for Rad51. The MGMT expressing T98G cells were completely resistant to TMZ, irrespective of Rad51 knockdown (Fig. 4C). This data are similar to those obtained with LN-229 MGMT transfected cells, supporting the notion that HR knockdown sensitizes glioma cells to O6MeG adducts and that MGMT inhibition greatly improves on this.
Knockdown of Rad51 in glioma cells prevents the repair of DSBs formed during the processing of O6-methylguanine
Having shown that knockdown of HR proteins sensitizes glioma cells to O6AAs, the question of whether it would also have an effect on the formation and repair of DSBs following TMZ treatment was addressed. A reliable and robust marker for DSBs are phosphorylated histone 2AX (γH2AX) foci [21]. Glioma cells knocked-down in Rad51 were treated with TMZ and compared with non-knockdown cells as to DSB formation and repair (Fig. 5A for representative immunofluorescence and Fig. 5B for quantification). In control cells transfected with the empty vector, DSBs were formed 48 h after treatment and then repaired (Fig. 5B), which conforms to previous data [17]. Contrary to this, in Rad51 knockdown lines (clones 8 and 23) DSBs were formed and remained unrepaired up to 144 h after TMZ treatment (Fig. 5B). Clearly, knockdown of Rad51 in glioma cells caused a significant defect in the repair of DSBs induced by TMZ. The inhibition of DSB repair as determined by γH2AX showed an inverse and significant correlation with Rad51 expression in the knockdown cells (Fig. 6A). The γH2AX foci level that remained after 144 h was also related to cell survival following TMZ (Fig. 6B). To confirm that TMZ-induced γH2AX foci are “true” DSBs, a second DSB marker was used that is known to physically interact with γH2AX, namely 53BP1 [22]. Similar to γH2AX, TMZ also induced the formation of 53BP1 foci, which co-localized with γH2AX (Fig. 7). The data support the notion that Rad51 dependent HR is required for the repair of TMZ-induced DSBs.
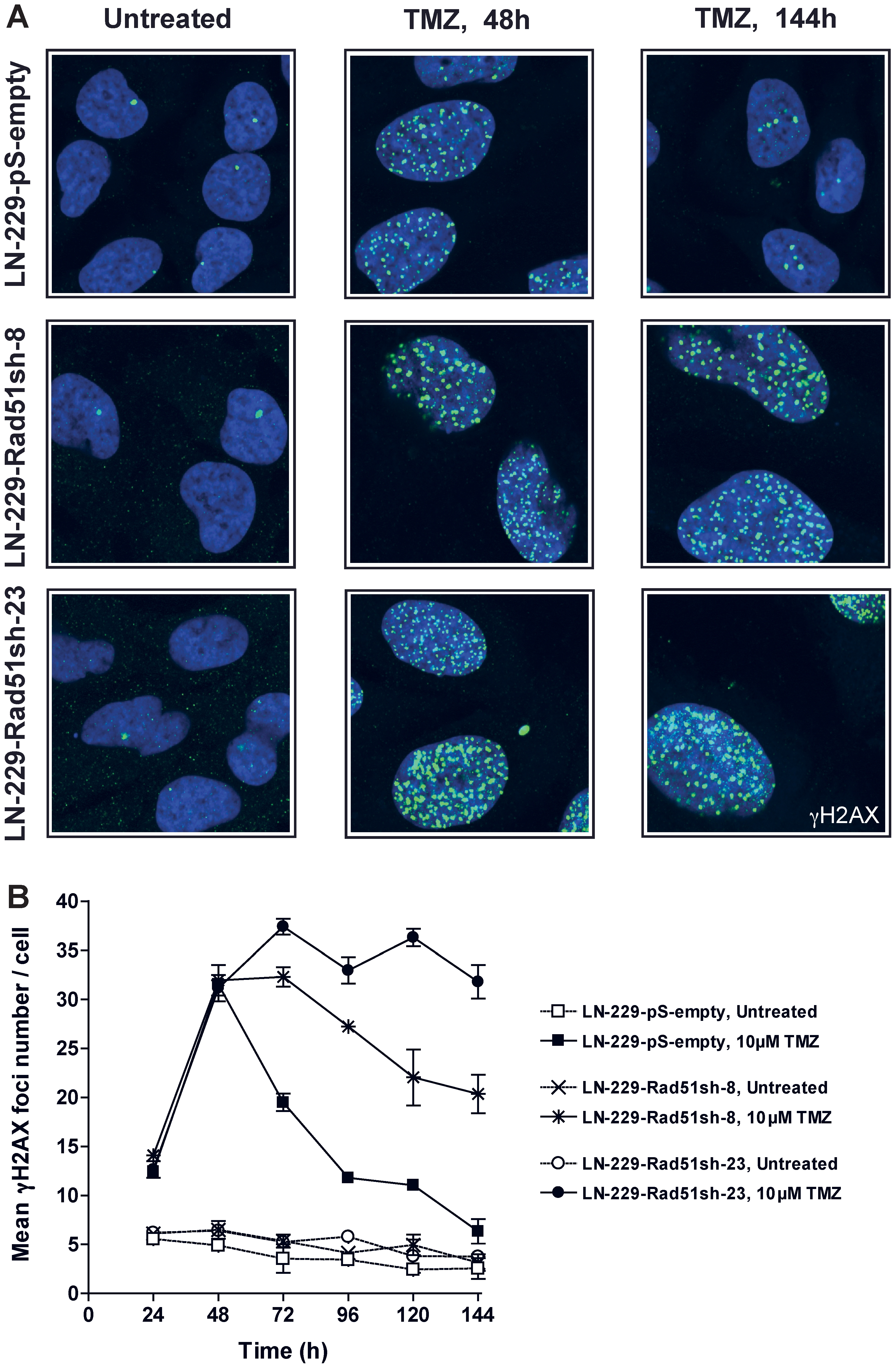
Kinetics of TMZ-induced γH2AX foci formation and disappearance in Rad51 knockdown glioma cells treated with 10 µM TMZ. See (A) for representative micrographs and (B) for quantification. Each measure point represents the mean of 200 cells per experiment. Experiments were repeated twice and mean values +/− SD are shown.
Influence of inhibiting NHEJ or poly(ADP-ribose)polymerase on TMZ treated glioma cells
As NHEJ may theoretically also play a protective role for methylating agents, independent of HR, its effect was investigated in TMZ-treated glioma cells. A main player in NHEJ is DNA-PKcs, which is a PI3-kinase that binds, together with Ku70 and Ku80, to free DSB ends and stimulates their rejoining and ligation [11], [12]. For inhibiting DNA-PK we used the small molecule inhibitor NU7026 [23], which strongly inhibited DNA-PK activity upon treatment of both LN-229 control (transfected with the empty vector) and LN-229 Rad51 transfected cells (Fig. 8A). Inhibition of DNA-PK with NU7026 in LN-229 cells treated with TMZ, irrespective of Rad51 knockdown, had no significant effect on survival (Fig. 8B), indicating that NHEJ does not protect significantly against TMZ. This is in line with our previous data obtained with rodent mutant cells, which showed that NHEJ only contribute marginally to the protection against O6-methylating agents [17]. Interestingly, DNA-PK inhibition had a significant sensitization effect on LN-229 glioma cells treated with γ-rays (Fig. 8B), which supports the observation that NHEJ was indeed inhibited in these cells. The results suggest a divergent role for this pathway in the repair of TMZ and ionizing radiation-induced DNA damage.
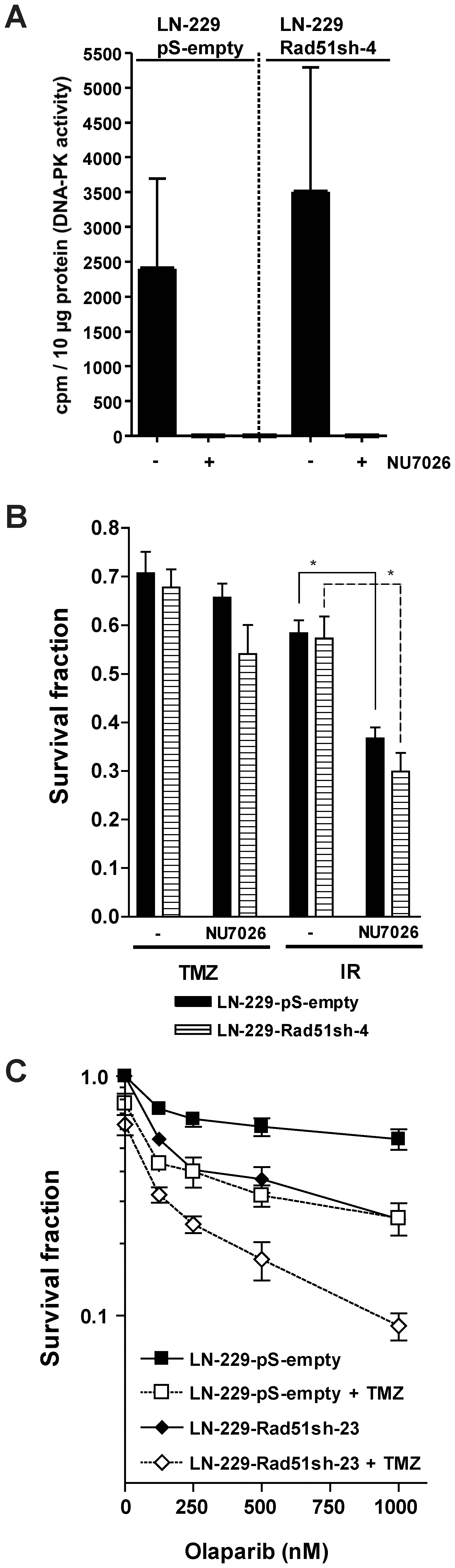
(A) Inhibition of DNA-PK activity with NU7026. DNA-PK activity was determined in cell extracts in the presence and absence of NU7026. (B) Clonogenic survival of stable Rad51 knocked-down glioma cells (LN-229-Rad51sh-4) compared to empty vector-transfected cells (LN-229-pS-empty) after TMZ or ionizing radiation (IR) treatment in the presence or absence of the DNA-PKcs inhibitor NU7026. Equitoxic TMZ and ionizing radiation (IR) doses producing about 30 to 40% toxicity in the absence of DNA-PK inhibition were used [TMZ/IR doses: LN-229-pS-empty: 2.7 µM/2.7Gy; LN-229-Rad51sh-4: 1.5 µM/1.9 Gy]. *, p<0.05, significance levels were calculated using the Mann-Whitney U test (n=
5). (C) Clonogenic survival of control (LN-229-pS-empty-2) and Rad51 knockdown (LN-229-Rad51sh-23) glioma cells as a function of olaparib concentration. Cells were co-treated with TMZ or not. TMZ doses producing 30 to 40% toxicity in the absence of PARP inhibition were selected for LN-229-Rad51sh-23 (0.8 µM) and control cells (2.5 µM).
Synthetic lethality caused by PARP inhibitors in HR-deficient cells [24] has paved the way for several clinical trials for the use of these inhibitors in HR-deficient tumors. This prompted us to study the effect of PARP inhibition on Rad51 knockdown cells. As shown in Fig. 8C, PARP inhibition with olaparib resulted in increased killing in glioma cells knocked-down for Rad51 compared to controls. Co-treatment with TMZ and olaparib increased cell kill in both control and knocked-down cells; the most drastic decrease in survival was observed in the latter. The data indicates that glioma cells are unable to deal with TMZ-induced DNA damage when PARP is inhibited, which is exacerbated when HR is down-regulated by Rad51 knockdown.
Outlook
Collectively, the data shows a dramatic increase in the sensitivity of glioma cells treated with O6-methylating (TMZ) and O6-chlorethylating (ACNU) anticancer drugs once HR was impaired by knockdown of its major players Rad51 and BRCA2. This was demonstrated in glioma cell lines proficient (LN-229 and U87MG) and deficient (T98G) for p53. Although MGMT completely abolished the glioma cell sensitization achieved by HR knockdown, its pharmacological inhibition by the pseudosubstrate O6BG restored it. The data also corroborates previous observations indicating a minor role of NHEJ in the repair of O6MeG triggered DSBs [17], and indicates a potential use of PARP inhibitors to further enhance TMZ-induced toxicity in glioma cells. As O6AA are employed so widely as part of the treatment of different tumors, especially in the metastatic state and when complete surgical removal of the neoplasm is not possible, enhancement of chemotherapy efficacy is highly desired.
While we demonstrated that down-regulation of Rad51 and BRCA2 greatly sensitizes glioma cells to TMZ and ACNU and, therefore, are potential new targets in glioma therapy, the limitation of this approach should also be pondered. High MGMT levels still provoke resistance and therefore tumors with an unfavorable MGMT status will still represent a major hurdle. We should note, however, that HR also protects against N-alkylations (Nikolova et al., in preparation), which are formed by TMZ and CNUs at high amounts and are repaired by base excision repair. It is thus conceivable that inhibition of repair of N-alkylations together with knockdown of HR might be a strategy for targeting tumors that express high MGMT levels.
Another important issue pertains to the tumor targeting for HR knockdown strategies, in order to prevent unwanted side effects due to enhanced normal tissue toxicity. Indeed, systemic down-regulation of HR might exacerbate the side effects related to chemotherapeutics. Therefore, either local administration directly to the tumor or a proper tumor targeting strategy for iRNA [25], [26] and MGMT inhibitors [5], [27] might be fundamental for optimizing the therapeutic index of this approach. In summary, the study provides proof of principle evidence that siRNA targeting the HR pathway is a reasonable strategy for increasing O6AA efficacy, which may prove beneficial for patients treated with these anticancer drugs.
Materials and Methods
Cell lines and culture conditions
LN-229, U87MG and T98G cells were provided by Dr. M. Weller (Department of Oncology, University Hospital Zurich, Switzerland) [28] and were checked before experimental use for mycoplasma contamination. Cells were cultured in Dulbecco's modified Eagle's medium (DMEM) containing 10% fetal bovine serum as previously described [29], [30]. Cells were cultured in a humidified atmosphere with 7% CO2 at 37°C.
Drugs, treatments and irradiation of cells
TMZ (a generous gift from Schering-Plough) stocks were prepared by dissolving it in DMSO and sterile dH2O (12) to a concentration of 35 mM. ACNU (Sigma) stocks were prepared by dissolving it in sterile dH2O to 10 mM. O6-benzylguanine (O6BG, Sigma), NU7026 (Sigma) and Olaparib (AZD2281, Selleck Chemicals) stocks were prepared by dissolving them in DMSO to 10 mM, 5 mM and 10 mM, respectively. TMZ stocks were stored at −80°C while the other stocks were stored at −20°C. For MGMT depletion or PARP inhibition, O6BG (10 µM) or Olaparib was added 1 h prior to drug treatments. NU7026 (10 µM) was added to cells 6h prior to treatments. Radiation was performed with a 137Cs source (Gammacell 2000, Molsgaard Medical).
Plasmids and stable transfections
A pSuper (OligoEngine) construct was generated to express shRNA targeting Rad51 mRNA using the previously described sequence (5′- GAAGAAAUUGGAAGAAGCU-3′) [31]. The pSV2MGMT vector has been described previously [32]. Plasmid DNA were transfected using Effectene (Qiagen). Transfected cells were selected with 0.75 mg/ml G418 or 0.44 µg/ml puromycin (both from Invitrogen) until clones formed.
Transient transfection
BRCA2 siRNA (5′-CUGAGCAAGCCUCAGUCAAtt-3′), Rad51 siRNA (-GAAGAAAUUGGAAGAAGCUtt-3′), (target sequences previously reported in [33], and [31], respectively) or non-sense siRNA (AllStars Negative Control siRNA, Qiagen) were transfected with Lipofectamine RNAiMAX (Invitrogen).
Flow cytometric analysis
For Sub-G1 analysis, harvested cells were fixed in 70% ethanol at -20°C. RNA digested-cells (30 µg/ml RNase A for 1 h in PBS) were stained with 16.7 µg/ml propidium iodide (PI) prior to FACS analysis. Annexin V-FITC/ PI double-staining was performed following the manufacturer specifications (BD Pharmingen). Flow cytometry analysis was performed using a FACSCalibur (Becton Dickinson).
Colony Formation Assay
Cells in logarithmic growth were seeded at appropriate numbers on 60 mm dishes. Cells were allowed to attach for 6 h before irradiation or drug treatments. Colonies were fixed in acetic acidmethanol
H2O (1
1
8), and stained with 1.25% Giemsa and 0.125% violet crystal. Transient transfected cells were seeded on dishes 18 h after transfection.
Immunofluorescence microscopy
Cells were sequentially fixed with 4% formaldehyde and 100% methanol. Primary antibodies (anti-phospho-S139-H2AX, 11000, Upstate; anti-53BP1, 1
400, Cell Signaling) were incubated overnight at 4°C followed by 2 h incubation with the secondary antibody (anti mouse- Alexa Fluor 488, 1
500, Invitrogen; anti-rabbit-Cy™3, 1
500, Jackson ImmunoResearch Laboratories). DNA was counterstained either with 100 nM DAPI or 1 µM TO-PRO-3 (Invitrogen). Slides were mounted in 1% DABCO- 50% glycerol- PBS. Foci were scored automatically with the Metafer Finder System v.3.1 (MetaSystems). Microphotographs were acquired by laser scanning microscopy (LSM710, Carl Zeiss MicroImaging).
DNA-PK activity assay
Total cell extracts were prepared as described [34]. Briefly, cells were lysed using three cycles of freeze (liquid nitrogen)/thaw (30°C) in extraction buffer (50 mM NaF, 20 mM HEPES (pH 7.8), 450 mM NaCl, 25% glycerol, 0.2 mM EDTA, 0.5 mM dithiothreitol, in the presence of protease inhibitors (Complete. EDTA-free. Roche)). Extracts were centrifuged (12 000 rpm for 30 min at 4°C), and supernatants were shock frozen and stored at −80°C. Endogenous DNA was removed by DEAE Sepharose Fast Flow (GE Healthcare). DNA-PK activity was determined by liquid scintillation counting using the SignaTECT DNA-Dependent Protein Kinase Assay System (Promega). The assay was performed on three independent cell extracts for each cell line. Each sample was analyzed in the presence or absence of 10 µM of the DNA-PKcs inhibitor NU7026. For background control, reactions were performed in the absence of activator, as suggested by the assay manufacturer.
Western blot analysis
Samples were resolved by SDS-PAGE, and blotted onto a nitrocellulose membrane. Primary antibodies [BRCA2 (Cell Signaling), Erk-2 (Santa Cruz), MGMT (Millipore), Rad51 (Calbiochem)] were used and detected by the Odyssey® Infrared Imaging System (LI-COR Biotechnology).
Acknowledgments
We thank Teodora Nikolova for help with the LSM and Markus Christmann for DNA-PK assay.
Footnotes
Competing Interests: The authors have declared that no competing interests exist.
Funding: The Deutsche Forschungsgemeinschaft and German Cancer Research Fund supported this work. SQ was supported by the Deutscher Akademischer Austauschdienst (grant A/06/05736). The funders had no role in study design, data collection and analysis, decision to publish, or preparation of the manuscript.
References
Articles from PLOS ONE are provided here courtesy of PLOS
Full text links
Read article at publisher's site: https://doi.org/10.1371/journal.pone.0027183
Read article for free, from open access legal sources, via Unpaywall:
https://journals.plos.org/plosone/article/file?id=10.1371/journal.pone.0027183&type=printable
Citations & impact
Impact metrics
Article citations
MGMT function determines the differential response of ATR inhibitors with DNA-damaging agents in glioma stem cells for GBM therapy.
Neurooncol Adv, 6(1):vdad165, 19 Dec 2023
Cited by: 0 articles | PMID: 38213834 | PMCID: PMC10783493
Temozolomide, Procarbazine and Nitrosoureas in the Therapy of Malignant Gliomas: Update of Mechanisms, Drug Resistance and Therapeutic Implications.
J Clin Med, 12(23):7442, 30 Nov 2023
Cited by: 7 articles | PMID: 38068493 | PMCID: PMC10707404
Review Free full text in Europe PMC
Lysine-specific histone demethylase 1A (KDM1A/LSD1) inhibition attenuates DNA double-strand break repair and augments the efficacy of temozolomide in glioblastoma.
Neuro Oncol, 25(7):1249-1261, 01 Jul 2023
Cited by: 5 articles | PMID: 36652263 | PMCID: PMC10326496
Ubiquitin-Specific Protease 6 n-Terminal-like Protein (USP6NL) and the Epidermal Growth Factor Receptor (EGFR) Signaling Axis Regulates Ubiquitin-Mediated DNA Repair and Temozolomide-Resistance in Glioblastoma.
Biomedicines, 10(7):1531, 28 Jun 2022
Cited by: 3 articles | PMID: 35884836 | PMCID: PMC9312792
The DNA Double-Strand Break Repair in Glioma: Molecular Players and Therapeutic Strategies.
Mol Neurobiol, 59(9):5326-5365, 13 Jun 2022
Cited by: 16 articles | PMID: 35696013
Review
Go to all (51) article citations
Similar Articles
To arrive at the top five similar articles we use a word-weighted algorithm to compare words from the Title and Abstract of each citation.
Integrin αVβ3 silencing sensitizes malignant glioma cells to temozolomide by suppression of homologous recombination repair.
Oncotarget, 8(17):27754-27771, 01 Apr 2017
Cited by: 22 articles | PMID: 27487141 | PMCID: PMC5438606
Apoptosis in malignant glioma cells triggered by the temozolomide-induced DNA lesion O6-methylguanine.
Oncogene, 26(2):186-197, 03 Jul 2006
Cited by: 315 articles | PMID: 16819506
Targeting Homologous Recombination by Pharmacological Inhibitors Enhances the Killing Response of Glioblastoma Cells Treated with Alkylating Drugs.
Mol Cancer Ther, 15(11):2665-2678, 29 Jul 2016
Cited by: 29 articles | PMID: 27474153
DNA repair in personalized brain cancer therapy with temozolomide and nitrosoureas.
DNA Repair (Amst), 78:128-141, 15 Apr 2019
Cited by: 67 articles | PMID: 31039537
Review