Abstract
Free full text

Cultured Representatives of Two Major Phylogroups of Human Colonic Faecalibacterium prausnitzii Can Utilize Pectin, Uronic Acids, and Host-Derived Substrates for Growth
Abstract
Faecalibacterium prausnitzii is one of the most abundant commensal bacteria in the healthy human large intestine, but information on genetic diversity and substrate utilization is limited. Here, we examine the phylogeny, phenotypic characteristics, and influence of gut environmental factors on growth of F. prausnitzii strains isolated from healthy subjects. Phylogenetic analysis based on the 16S rRNA sequences indicated that the cultured strains were representative of F. prausnitzii sequences detected by direct analysis of fecal DNA and separated the available isolates into two phylogroups. Most F. prausnitzii strains tested grew well under anaerobic conditions on apple pectin. Furthermore, F. prausnitzii strains competed successfully in coculture with two other abundant pectin-utilizing species, Bacteroides thetaiotaomicron and Eubacterium eligens, with apple pectin as substrate, suggesting that this species makes a contribution to pectin fermentation in the colon. Many F. prausnitzii isolates were able to utilize uronic acids for growth, an ability previously thought to be confined to Bacteroides spp. among human colonic anaerobes. Most strains grew on N-acetylglucosamine, demonstrating an ability to utilize host-derived substrates. All strains tested were bile sensitive, showing at least 80% growth inhibition in the presence of 0.5 μg/ml bile salts, while inhibition at mildly acidic pH was strain dependent. These attributes help to explain the abundance of F. prausnitzii in the colonic community but also suggest factors in the gut environment that may limit its distribution.
INTRODUCTION
Faecalibacterium (formerly Fusobacterium) prausnitzii (11) is one of the three most abundant species detected in human feces by anaerobic cultivation (32) and by 16S rRNA-based molecular analyses (21, 51, 52, 57). Following its first isolation (4, 20), this species received little attention, partly because of its oxygen sensitivity (14), until new isolates became available from studies on the dominant butyrate-producing bacteria from the human colon (2) that allowed the definition of the new genus Faecalibacterium (11). Interest in this bacterium has increased recently with reports that the relative abundance of F. prausnitzii among the human colonic microbiota, as estimated by 16S rRNA-based culture-independent methods, is reduced in certain forms of inflammatory bowel disease. Crohn's disease (CD) patients, mainly those with ileal involvement, have been reported to exhibit diminished prevalence of Firmicutes, often with a concomitant increase in Proteobacteria (15, 30, 60). Molecular analysis of both fecal and biopsy samples has revealed that the depletion in the former is due in part to decreased abundance of the F. prausnitzii group (6, 45, 47, 50, 60). Reduced F. prausnitzii abundance has also been reported in colorectal cancer (1) and in the frail elderly (29, 56), leading to the suggestion that this bacterium could provide an indicator of a healthy gut microbiota. F. prausnitzii is one of the main sources of butyrate in the colon (27, 37), and the multiple effects of butyrate as the preferred energy source for the colonocytes and upon apoptosis, inflammation, and oxidative stress are generally considered to be beneficial to intestinal health (18, 37, 40). F. prausnitzii is also thought to have additional anti-inflammatory properties that are suggested by cellular studies and trinitrobenzene sulfonic acid colitis models in mice (49).
In view of the proposed role of F. prausnitzii in intestinal health, it is important to gain a better understanding of the microbial ecology of this species. It is currently unclear what major substrates, of dietary or host origin, are likely to support growth and what factors in the gut environment may influence its distribution in the intestine. It is also important to establish how much genetic and phenotypic variation occurs within this species and the extent to which available cultured strains represent the diversity present in vivo. This study addresses these questions by examining the characteristics of the available cultured strains, including new isolates from healthy humans.
MATERIALS AND METHODS
Bacterial strains and growth conditions.
The F. prausnitzii isolates listed in Table 1 were from stocks held by the authors (S. H. Duncan, Rowett Institute of Nutrition and Health, Aberdeen, United Kingdom, and H. J. M. Harmsen, Department of Medical Microbiology, University of Groningen, Groningen, The Netherlands), and all are of human fecal origin (Table 1). F. prausnitzii-related isolates were obtained from the highest countable dilution of human fecal samples in roll tubes of anaerobic M2GSC medium (31), as described previously (2). Anaerobic culture methods were those of Bryant (3) using Hungate culture tubes, sealed with butyl rubber septa (Bellco Glass). Additional F. prausnitzii strains designated HTF isolates were isolated from freshly voided human stools, by plating 1 μl of the fecal material with a loop as a lawn directly on YCFAG medium (see below). After 12 h to 16 h of incubation at 37°C in an anaerobic tent (80% N2, 12% CO2, and 8% H2), 500 translucent colonies per sample were selected and subcultured on fresh plates (50 per plate in a grid-like fashion). After growth, the colonies were presumptively identified based on morphology, eliminating 95% of the colonies. The remaining colonies were further purified and Gram stained. Up to 5 colonies per sample were finally identified by 16S rRNA gene sequencing. The isolates were routinely maintained by being grown for 16 to 18 h at 37°C in 7.5-ml aliquots of M2GSC medium (31) and maintained anaerobically using O2-free CO2. The low-percent G+C Gram-positive Firmicutes strains screened for pectin utilization in this study (see Table 3) were also from stocks held by the authors (Rowett Institute of Nutrition and Health, Aberdeen, United Kingdom), and several came from previous studies (2, 26). The strains included Roseburia intestinalis L1-82 (DSM 14610T), Roseburia hominis A2-183 (DSM 16839T), Roseburia inulinivorans strains A2-194 (DSM 16841T) and L1-83, Roseburia faecis M72/1 (DSM 16841T) and M88/1, and Eubacterium rectale A1-86 (DSM 17629), M104/1, and L2-21, with type strains deposited with the Deutsche Sammlung von Mikrooganismen und Zellkulturen (DSMZ). Other Firmicutes tested in the study included Butyrivibrio fibrisolvens 16/4, which was isolated as a butyrate-producing wheat bran degrader (41). Eubacterium siraeum 70/3 (8) and V10Sc8a are also isolates from human fecal samples. Eubacterium eligens DSM 3376 was from DSMZ, Bacteroides thetaiotaomicron B5482 was a gift from A. Salyers, and both strains were included in the coculture studies.
Table 1
Details of F. prausnitzii strains included in this studya
Isolate code | Laboratory of isolation | Volunteer | Sex | Age (yr) | Culture collection | Reference(s) for original isolation |
---|---|---|---|---|---|---|
ATCC 27768 | 1 | Unknown | Unknown | ATCC 27768 | 4 | |
A2-165 | RINH | 2 | F | 34 | DSMZ 17677 | 2, 11 |
L2-15 | RINH | 3 | M | 2 | 2 | |
L2-39 | RINH | 3 | M | 2 | 2 | |
L2-6 | RINH | 3 | M | 2 | 2, 11 | |
L2-61 | RINH | 3 | M | 2 | 2 | |
M21/2 | RINH | 4 | F | 36 | 26 | |
S3L/3 | RINH | 5 | F | 46 | 26 | |
S4L/4 | RINH | 5 | F | 46 | 26 | |
HTF-A | GU | 6 | M | 31 | This study | |
HTF-B | GU | 6 | M | 31 | This study | |
HTF-C | GU | 6 | M | 31 | This study | |
HTF-E | GU | 7 | M | 44 | This study | |
HTF-F | GU | 7 | M | 44 | This study | |
HTF-I | GU | 8 | M | 28 | This study | |
HTF-60C | GU | 8 | M | 28 | This study | |
HTF-75H | GU | 9 | M | 65 | This study |
Table 3
Distribution of pectin-utilizing ability among cultured strains of human colonic anaerobes
Phylum and species | No. of strains testeda | No. of pectin utilizers |
---|---|---|
Bacteroidetes | ||
![]() ![]() ![]() ![]() | 22 | 22 |
![]() ![]() ![]() ![]() | 24 | 23 |
![]() ![]() ![]() ![]() | 22 | 7 |
![]() ![]() ![]() ![]() | 53 | 17 |
![]() ![]() ![]() ![]() | 67 | 19 |
Actinobacteria | ||
![]() ![]() ![]() ![]() | 41 | 0 |
![]() ![]() ![]() ![]() | 15 | 0 |
Firmicutes | ||
![]() ![]() ![]() ![]() | 20; 10b | 0 |
![]() ![]() ![]() ![]() | 5 | 3 |
![]() ![]() ![]() ![]() | 5 | 0 |
![]() ![]() ![]() ![]() | 16 | 0 |
![]() ![]() ![]() ![]() | 7 | 0 |
![]() ![]() ![]() ![]() | 8 | 0 |
![]() ![]() ![]() ![]() | 6 | 0 |
![]() ![]() ![]() ![]() | 10 | 0 |
![]() ![]() ![]() ![]() | 10b | 8b |
![]() ![]() ![]() ![]() | 14 | 0 |
![]() ![]() ![]() ![]() | 2b | 0 |
![]() ![]() ![]() ![]() | 7 | 0 |
Growth medium.
YCFA medium consists of (per 100 ml) Casitone (1.0 g), yeast extract (0.25 g), NaHCO3 (0.4 g), cysteine (0.1 g), K2HPO4 (0.045 g), KH2PO4 (0.045 g), NaCl (0.09 g), (NH4)2SO4 (0.09 g), MgSO4 · 7H2O (0.009 g), CaCl2 (0.009 g), resazurin (0.1 mg), hemin (1 mg), biotin (1 μg), cobalamin (1 μg), p-aminobenzoic acid (3 μg), folic acid (5 μg), and pyridoxamine (15 μg). In addition, the following short-chain fatty acids (SCFA) are included (final concentrations): acetate (33 mM); propionate (9 mM); isobutyrate, isovalerate, and valerate (1 mM each). Cysteine is added to the medium following boiling and dispensed into Hungate tubes while the tubes are flushed with CO2. After autoclaving, filter-sterilized solutions of thiamine and riboflavin are added to give final concentrations of 0.05 μg ml−1 of each. For some experiments, the Casitone content was decreased to 0.2%; this modified medium is referred to as YcFA. Carbohydrate or other energy sources were added as indicated, and the final pH of the medium was adjusted to 6.8 ± 0.1.
DNA extraction, PCR amplification, and DGGE fingerprinting.
DNA was extracted and purified from 18-h-old cultures of F. prausnitzii strains grown on M2GSC medium by using the Wizard genomic purification kit (Promega Corporation, Madison, WI). 16S rRNA sequences were amplified using universal bacterial primers GC-357F (33) and 907R (34) to give an approximately 580-bp product flanking variable regions V3 to V5. PCR and denaturing gradient gel electrophoresis (DGGE) were carried out as previously reported (30).
16S rRNA gene amplification and sequencing.
16S rRNA genes were amplified using the universal bacterial primers 7F and 1510R (23) as described previously (12). PCR products were cleaned with the Wizard PCR product purification kit (Promega, Southampton, United Kingdom) and used to obtain bidirectional partial 16S rRNA gene sequences by using primers 7F, 519F, 519R, 916F, 916R, and 1510R (16, 23) on a Beckman capillary sequencer. All primers were obtained from Eurofins MWG.
16S rRNA gene sequence full-length construction and phylogenetic analysis.
Sequences from cultured isolates were manually inspected in order to assess quality. Sequence editing and assembling were carried out using the BioEdit sequence alignment editor, version 7.0.9.0 (17). Sequences were then aligned in Mothur (http://www.mothur.org) (46) using the SILVA bacterial database as a reference alignment, available at the same source. Alignment was then imported into the ARB software package (28) loaded with the SILVA 16S rRNA-ARB-compatible database (SSURef-100, August 2009, available through the SILVA rRNA database project at http://www.arb-silva.de/) (36). For the detection of chimeric sequences, each sequence was checked manually in the alignment, and phylogenetic trees were screened for sequences with unrealistically long branches or unique branching sites. Cultured representatives from the Ruminococcaceae were included as reference, and Eubacterium desmolans was used to root the tree. Phylogenetic analyses of the 16S rRNA gene sequences were conducted using the ARB software package, using the neighbor-joining (NJ) method (42) and the Jukes-Cantor (JC) algorithm for distance analysis. Tree topologies were evaluated using maximum parsimony and maximum likelihood methods. No filters or masks were used when constructing the trees. Bootstrapping analysis (1,000 replicates) was done to test the robustness of the NJ-JC tree using PHYLIP (13).
To assess which F. prausnitzii phylogroups were represented by the isolates, representative sequences of 16S rRNA genes directly amplified from fecal DNA were included (boldfaced in Fig. 2). These uncultured sequences were aligned and processed as described above and then added to the isolate-based tree using the Parsimony Quick Add Marked Tool already implemented in the ARB software package, thereby maintaining the overall tree topology.
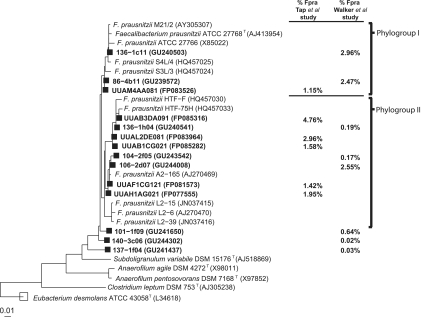
Neighbor-joining phylogenetic tree showing the relationship between cultured F. prausnitzii strains and directly amplified partial 16S rRNA gene sequences from human fecal samples. 16S rRNA sequence accession numbers are given in parentheses. Squares indicate OTU representative sequences from two recent studies on gut microbiota of healthy subjects (shown in boldface): the Tap et al. (53) study (1,443 F. prausnitzii sequences out of 10,456 clones from 17 healthy adults of both sexes) and the Walker et al. (57) study (534 F. prausnitzii sequences out of 5,915 total sequences from six obese males). The percentage of all clones represented by each OTU in each of these studies is shown on the right.
RAPD-PCR.
Isolates were screened by random amplified polymorphic DNA PCR (RAPD-PCR) using the primer 1254, according to a previously described method (59). RAPD-PCR profiles were compared using the GelComparII software (Applied Maths, Belgium). The UPGMA (unweighted-pair group method using average linkages) method was used to build the dendrogram (see Fig. S1 in the supplemental material), and clusters were defined at a similarity score of >93.5%.
Carbohydrate utilization and assessment of bacterial growth.
Substrate utilization was determined by adding a final concentration of 0.5% (wt/vol) sugar to YCFA medium. Where possible, growth was measured spectrophotometrically as optical density at 650 nm (OD650) for triplicate cultures at regular intervals up to stationary phase. For insoluble xylan, however, fermentation was monitored by final pH measurement. To study competition for pectin, F. prausnitzii strains S3L/3 and A2-165 were inoculated individually and together with the known pectin-utilizing species B. thetaiotaomicron B5482 and E. eligens 3376 in cocultures and tricultures (see Table S2 in the supplemental material). These experiments used YcFA medium supplemented with 0.5% apple pectin (BDH Chemicals) that had been preadjusted to three different initial pH values (6.12, 6.45, and 6.79). Samples were collected at 0 h and 24 h to estimate bacterial numbers by fluorescent in situ hybridization (FISH), total sugar analysis, and SCFA concentrations. SCFA were analyzed by gas chromatography following conversion to t-butyldimethylsilyl derivatives (39). Total sugars were determined using the colorimetric phenol sulfuric assay (10).
Influence of initial pH and bile salts on bacterial growth.
Each strain was inoculated into YCFA medium supplemented with 10 mM glucose (YCFAG) that had been adjusted to the three different initial pH values (6.7, 6.2, and 5.75) as described previously (12). Growth was followed for 24 h by measuring absorbance at 650 nm for triplicate cultures, and specific growth rates (h−1) were calculated in exponential phase. The influence of bile salts (Sigma B8631) was assessed by inoculating culture into YCFAG medium containing 0% (control), 0.1%, 0.25%, or 0.5% bile salts (all percentages in wt/vol), in triplicate. Growth was measured spectrophotometrically up to 24 h using absorbance at the 650-nm wavelength. The pH of the medium was also monitored at the beginning and at the end of each experiment.
Enumeration of F. prausnitzii bacteria by FISH analysis.
Cultures were prepared for analysis as described previously (19). Cell suspensions were applied to gelatin-coated slides. Dried slides were hybridized with 10 μl of the Fprau645 oligonucleotide probe (52) (50-ng/μl stock solution) and washed. Between 25 and 30 fields were counted per well using an epifluorescence microscope (Olympus) and image analysis software (Olympus Cell F digital imaging software) or manual counting for numbers of less than 10 fluorescent cells per field.
Statistical analysis.
Quantitative parameters, such as growth rates and relative OD650, were compared by one-way analysis of variance (ANOVA). The Bonferroni post hoc test was applied for multicomparisons of those variables with more than two subgroups of samples. Previously, data normality was assessed by the Shapiro-Wilks test and the Leven test was conducted to assess for homoscedasticity. The Kruskal-Wallis nonparametric test was performed when required. All statistical analyses were conducted via SPSS 15.0 (SPSS Inc., Chicago, IL).
Nucleotide sequence accession numbers.
The 16S rRNA gene full-length sequences of isolates S3L/3, S4L/4, HTF-A, HTF-B, HTF-C, HTF-E, HTF-F, HTF-I, HTF-60C, HTF-75H, L2-15, L2-39, and L2-61 were deposited in the GenBank/EMBL/DDBJ database under the accession numbers HQ457025 to HQ457033 and JN037415 to JN037417, respectively.
RESULTS AND DISCUSSION
Phylogenetic diversity of Faecalibacterium prausnitzii.
Nearly full-length 16S rRNA gene sequences were determined for the first time here for 13 recent isolates of Faecalibacterium prausnitzii (Table 1; Fig. 1). The 16S rRNA sequences define two branches within the Ruminococcaceae, within which sequences share >97% sequence identity; these also include five sequences reported previously for the isolates M21/2, ATCC 27766, and ATCC 27768T (phylogroup I) and A2-165 and L2-6 (phylogroup II). The 18 isolates shown in Fig. 1 originated from 10 healthy individuals. Each of these 16S rRNA sequences is unique and came from a different colony, although there was a tendency for sequences to group by isolation and individual. This was also suggested by RAPD-PCR profiles for these strains (see Fig. S1 in the supplemental material). Comparison was also made with F. prausnitzii-related operational taxonomic units (OTUs) defined by partial 16S rRNA gene sequences obtained in two recent human studies by direct amplification from fecal DNA (53, 57) (Fig. 2). These represent an additional 23 individuals. Phylogroups I and II together account for 97.9% of these directly amplified F. prausnitzii-related sequences, with phylogroup I more abundant in the six subjects examined by Walker et al. (57) (62%) than in the 17 subjects examined by Tap et al. (53) (8.3%).
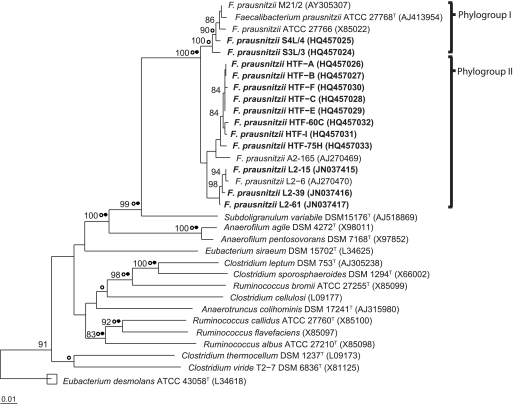
Phylogenetic relationship of F. prausnitzii isolates to other members of Clostridium cluster IV (Ruminococcaceae) based on 16S rRNA gene sequences. The tree was constructed using the ARB software package using the neighbor-joining method for distance analysis (Jukes-Cantor algorithm) with 1,533 informative positions considered (61 to 1,442 by E. coli 16S rRNA gene numbering). Bootstrap values above 80% (expressed as a percentage of 1,000 replications) are shown at branching points. Solid circles indicate branches that were consistent with calculations obtained by maximum-parsimony method. Empty circles represent those branches consistent with the maximum likelihood. The scale bar indicates the number of substitutions per site. F. prausnitzii isolates incorporated in this study are highlighted in bold. Sequence accession numbers are shown in parentheses. The database sequence for ATCC 27766 was included, but this strain was not studied here and it is not listed in Table 1.
DGGE analysis of PCR products amplified from phylogroup I isolates showed a distinct band position compared with phylogroup II isolates (Fig. 3). These band positions correspond to two dominant bands that have previously been associated with F. prausnitzii in DGGE analyses of 16S rRNA sequences amplified from human fecal and biopsy samples (22, 30). This previous work also suggested that there is a differential reduction in phylotypes related to M21/2 (phylogroup I) compared with A2-165 relatives (phylogroup II) in biopsy specimens (30) and fecal samples (22) from CD patients.
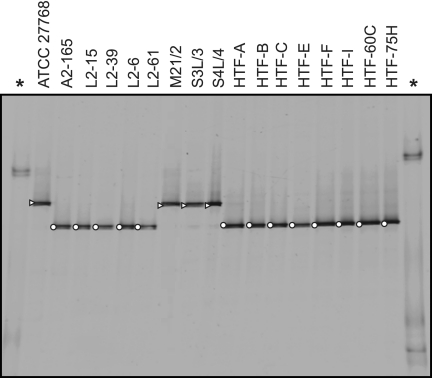
PCR-DGGE fingerprints from F. prausnitzii isolates. Isolates are distributed in two separate bands that correlate with phylogroup designation (, phylogroup I; ○, phylogroup II). Asterisks indicate the ladder lanes (made by 16S rRNA gene fragments of Mucor sp., Pseudomonas fluorescens, and Micrococcus luteus, respectively, from the top to the bottom).
Substrate utilization by Faecalibacterium prausnitzii isolates.
Growth on carbohydrates of dietary and host origin by four phylogroup I and six phylogroup II isolates is shown in Table 2. The basal YCFA medium (described in Materials and Methods) contained 33 mM acetate, which is known to stimulate the growth of F. prausnitzii strains (11). Growth was assessed where possible by the change in OD650, but for insoluble substrates such as xylan, it was necessary to rely on change in medium pH as an indicator of substrate fermentation. The ability of F. prausnitzii to utilize dietary polysaccharides was somewhat limited with no growth on arabinogalactan, no fermentation of xylan, and little or no growth on soluble starch. While two strains grew well on inulin, the remainder grew poorly. Stimulation of F. prausnitzii 16S rRNA sequences by inulin has been reported in vivo in healthy human volunteers (38), but it appears likely from the present work that this stimulation may favor certain strains. Interestingly, most isolates grew on apple pectin, although not on citrus pectin. Salyers et al. (43, 44) noted that the utilization of uronic acids was unusual in genera from the human colon other than Bacteroides species. In the present study, several F. prausnitzii strains were able to utilize galacturonic acid, which is an important constituent of pectin.
Table 2
Growth of F. prausnitzii strains on a range of carbohydrate substrates
Substratea | Supplier and catalog no. | OD650 (mean ± SD) after 24 h | |||||||||
---|---|---|---|---|---|---|---|---|---|---|---|
Phylogroup I strains | Phylogroup II strains | ||||||||||
ATCC 27768 | M21/2 | S3L/3 | S4L/4 | A2-165 | L2-15 | L2-39 | L2-6 | HTF-75H | HTF-F | ||
Glucose | BDH 10117 | 0.29 ± 0.02 | 0.96 ± 0.02 | 0.92 ± 0.18 | 0.83 ± 0.43 | 0.53 ± 0.13 | 0.29 ± 0.01 | 0.26 ± 0.05 | 0.32 ± 0.21 | 0.32 ± 0.02 | 0.85 ± 0.07 |
Cellobiose | Sigma C7252 | 0.26 ± 0.02 | 0.87 ± 0.33 | 0.81 ± 0.11 | 0.72 ± 0.21 | 0.63 ± 0.10 | 0.28 ± 0.01 | 0.18 ± 0.01 | 0.02 ± 0.07 | 0.32 ± 0.05 | 0.87 ± 0.01 |
Maltose | Sigma M5885 | 0.32 ± 0.35 | 0.85 ± 0.15 | 0.75 ± 0.07 | 0.82 ± 0.12 | 0.62 ± 0.07 | 0.44 ± 0.11 | 0.78 ± 0.05 | 0.22 ± 0.21 | 0.55 ± 0.10 | 1.01 ± 0.04 |
Rhamnose | Sigma R3875 | —b | — | — | 0.12 ± 0.03 | — | — | — | — | — | — |
Galacturonic acid | BDH 571670 | 0.12 ± 0.00 | 0.31 ± 0.04 | 0.45 ± 0.04 | 0.61 ± 0.06 | 0.21 ± 0.04 | 0.12 ± 0.01 | 0.07 ± 0.02 | — | — | 0.26 ± 0.02 |
Galactose | BDH G0750 | 0.24 ± 0.05 | 0.95 ± 0.03 | 0.44 ± 0.02 | 0.11 ± 0.09 | 0.80 ± 0.08 | 0.75 ± 0.28 | — | 0.61 ± 0.12 | 0.33 ± 0.28 | 0.66 ± 0.25 |
Pectin, apple | BDH 38052 | 0.31 ± 0.09 | 0.40 ± 0.04 | 0.36 ± 0.03 | 0.56 ± 0.02 | 0.66 ± 0.01 | 0.08 ± 0.00 | 0.07 ± 0.00 | 0.24 ± 0.02 | 0.18 ± 0.07 | 0.39 ± 0.07 |
Starch, potato | BDH 102713 | — | 0.06 ± 0.01 | 0.09 ± 0.02 | — | 0.07 ± 0.03 | — | 0.06 ± 0.01 | 0.07 ± 0.02 | 0.08 ± 0.06 | 0.05 ± 0.02 |
Inulin, chicory | Sigma I2255 | 0.21 ± 0.27 | 0.10 ± 0.00 | 0.08 ± 0.01 | 0.07 ± 0.01 | 0.80 ± 0.05 | — | — | 0.09 ± 0.18 | 0.18 ± 0.07 | 0.97 ± 0.26 |
Glucuronic acid | Fluka 71560 | 0.09 ± 0.00 | — | 0.28 ± 0.05 | 0.08 ± 0.01 | 0.83 ± 0.02 | — | — | — | 0.08 ± 0.03 | 0.17 ± 0.03 |
N-Acetylglucosamine | Sigma A8625 | 0.34 ± 0.03 | 0.88 ± 0.04 | 0.67 ± 0.00 | 0.57 ± 0.03 | 0.98 ± 0.01 | 0.18 ± 0.06 | 0.07 ± 0.00 | — | 0.20 ± 0.02 | 0.51 ± 0.24 |
Glucosamine HCl | BDH 962240 | 0.15 ± 0.01 | 0.31 ± 0.01 | 0.58 ± 0.01 | 0.34 ± 0.11 | 0.95 ± 0.15 | 0.13 ± 0.03 | 0.08 ± 0.02 | 0.14 ± 0.03 | 0.14 ± 0.03 | 0.16 ± 0.07 |
Growth was also detected for most F. prausnitzii strains on the host-derived sugar N-acetylglucosamine and for some strains on d-glucosamine and d-glucuronic acid, while β-glucuronidase activity has been reported previously in some F. prausnitzii isolates (8). This suggests that F. prausnitzii has the ability to switch between diet- and host-derived substrates, in common with several other dominant human colonic species (48). None of the carbohydrates tested allowed differentiation between the two phylogroups.
Very little growth was observed when carbohydrates were omitted from the medium, although the basal YCFA medium contains 1% Casitone. This indicates that F. prausnitzii strains have little or no ability to grow with peptides as their sole energy source. No evidence was found for fermentation of porcine gastric mucin.
Tolerance of Faecalibacterium prausnitzii isolates to the gut environment.
Previous studies have reported that F. prausnitzii growth is inhibited by slightly acidic pH (12). The eight isolates tested showed growth rates at pH 5.75 ranging between 20% (for A2-165) and 80% (for HTF-F) of those at pH 6.7 (Fig. 4A). On average, there was a 14% decrease at pH 6.2, but a 60% decrease at pH 5.75, compared with pH 6.7. Tolerance of bile salts, whose concentrations have been reported to increase in certain gut disorders (24, 35), is also considered to be an important factor for survival in the intestine. Bile salt tolerance differed among isolates, particularly at the lowest concentration tested (0.1%), but all the strains tested were bile salt sensitive, showing on average 76%, 95%, and 97% inhibition at 0.1%, 0.25%, and 0.5% bile salts, respectively (Fig. 4B). In contrast, other species of intestinal bacteria such as Bacteroides spp. and Enterococcus faecium have been reported to be resistant to up to 20% and 40% bile salt concentrations, respectively (5). Bile acids are synthesized in the liver and released into the small intestine, where it is estimated that 90 to 95% of secreted bile is absorbed. The concentration of bile in the healthy large intestine is approximately 0.05 to 0.3%. The sensitivity of all the F. prausnitzii isolates tested to bile salts suggests that this is a factor that may restrict populations of this species in regions of high bile concentration, e.g., within the small intestine.
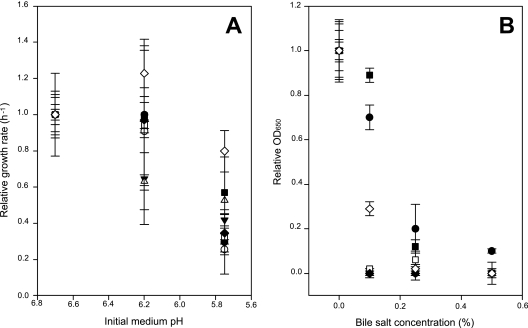
Tolerance of F. prausnitzii isolates to changes in initial medium pH values and bile salt concentrations. (A) Relative growth rates (h−1) of F. prausnitzii strains on YCFAG medium at three initial pH values (6.7, 6.2, and 5.75) have been represented. For comparison, the growth rate determined for each strain at pH 6.7 is taken as 1.0. (B) Relative OD650 after 24 h of F. prausnitzii isolates at four bile salt concentrations (0%, 0.1%, 0.25%, and 0.5%) on YCFAG medium. For comparison, the OD650 after 24 h of incubation determined for each isolate in medium without bile salt has been taken as 1.0. Mean growth rates at pH 6.7 and mean OD650s in the absence of bile salts for each strain (±standard deviation) were as follows: ●, ATCC 27768 (0.17 ± 0.02 and 0.33 ± 0.05, respectively); , M21/2 (0.32 ± 0.07 and 1.39 ± 0.05, respectively); ■, S3L/3 (0.16 ± 0.02 and 0.52 ± 0.07, respectively); ♦, S4L/4 (0.20 ± 0.02 and 0.63 ± 0.06, respectively); ○, A2-165 (0.55 ± 0.04 and 0.77 ± 0.02, respectively); Δ, L2-6 (0.19 ± 0.01 and 0.47 ± 0.02, respectively); □, HTF-75H (0.15 ± 0.01 and 0.386 ± 0.046, respectively);
, HTF-F (0.18 ± 0.01 and 0.826 ± 0.089, respectively). Phylogroup I isolates have been represented in black while phylogroup II isolates are shown in white.
While these differences in sensitivity to bile salts and pH seem likely to influence the distribution of individual strains, there was no statistically significant evidence for consistent differences between phylogroups.
Potential role of Faecalibacterium prausnitzii in the fermentation of pectin in the colon.
Pectin is extensively fermented in the human colon (7, 55), but the ability to utilize pectin for growth has been reported for relatively few groups of human colonic bacteria. Salyers et al. (43, 44) showed that pectin utilization was relatively common among Bacteroides spp., occurring in 47% of 188 isolates surveyed and prompting subsequent studies on B. thetaiotaomicron (9, 54). In contrast, of the 154 strains of Gram-positive anaerobes tested, which included five strains reported as Fusobacterium prausnitzii, only Eubacterium eligens was previously found to utilize pectin or polygalacturonic acid (43) (Table 3). The present data, however, indicate that F. prausnitzii could have a major role in pectin utilization (Table 3).
In order to test this hypothesis further, we examined the ability of two F. prausnitzii strains (S3L/3 and A2-165) to compete for apple pectin with representatives of the two other known groups of pectin-utilizing bacteria, B. thetaiotaomicron and E. eligens. As previous studies have shown that pH plays a critical role in determining the outcome of competition between Bacteroides spp. and Firmicutes (12, 58), incubations were performed at three initial pH values typical of the range seen in the distal colon (Fig. 5; see also Tables S1 and S2 in the supplemental material). In pure cultures, the major fermentation products produced from pectin were butyrate for F. prausnitzii, acetate and succinate for B. thetaiotaomicron, and formate and acetate for E. eligens (Fig. 5A). As previously observed for growth on starch and glucose (12), the lowest pH (6.12) curtailed fermentation of pectin by B. thetaiotaomicron. As expected (Fig. 4A), both F. prausnitzii strains grew well at the lowest pH (Fig. 5). Tricultures including all three species showed large amounts of butyrate at all three pH values, thus confirming the ability of F. prausnitzii to compete for this substrate with the other two pectin-utilizing species (Fig. 5B). Counts estimated by FISH for F. prausnitzii after 24 h of incubation indicated greater numbers in the triculture at the lowest pH than at the highest pH (Fig. 5C). Butyrate concentration was less affected by pH, indicating continued fermentative activity by F. prausnitzii in spite of decreased cell growth at the highest pH. Data for two-membered cocultures from this experiment are shown in Tables S1 and S2 in the supplemental material. Pectin utilization (measured by decrease in total sugar) was highest for cultures including B. thetaiotaomicron at pH 6.79 (see Table S2 in the supplemental material).

Competition for apple pectin. (A) Change in acidic product concentrations in the growth medium after 24-h fermentation of 0.5% apple pectin by monocultures and cocultures of isolated pectin-utilizing bacteria. F1, F. prausnitzii SL3/3; F2, F. prausnitzii A2-165; E, Eubacterium eligens 3376; B, B. thetaiotaomicron 5482 (monocultures). (B) F1+E+B and F2+E+B were tricultures of the three strains indicated. Negative values for acetate reflect the net consumption of acetate initially present in the medium by F. prausnitzii strains. Each strain or strain combination was inoculated into YcFA medium adjusted to three different initial pH values (6.12, 6.45, and 6.79). Final medium pH (measured in all cases and detailed in Table S2 in the supplemental material) had decreased after 24 h by up to 0.3 unit for F. prausnitzii monocultures, up to 0.7 unit for B. thetaiotaomicron, and up to 0.9 unit for E. eligens. The final pHs in the tricultures were 6.16 (F1+E+B) and 6.07 (F2+E+B) from initial pH 6.79, 5.79 (F1+E+B) and 5.64 (F2+E+B) from initial pH 6.45, and 5.47 (F1+E+B) and 5.33 (F2+E+B) from initial pH 6.12. Data for two-membered cocultures and on overall sugar utilization from the same experiment are given in Tables S1 and S2 in the supplemental material. (C) Numbers of F. prausnitzii cells detected by fluorescent in situ hybridization in cultures and cocultures. Counts/ml immediately after inoculation (t = 0) were as follows: S3L/3, 0.91 × 107 ± 0.05 × 107, and A2-165, 1.31 × 107 ± 0.01 × 107.
Conclusions.
F. prausnitzii is one of the three most abundant bacterial species found in the healthy adult human large intestine, but its ecology has remained largely unknown. This study has substantially increased the number of cultured, characterized F. prausnitzii isolates of human origin and has begun to provide a better understanding of the diversity and microbial ecology of this species in the colon. Based on their 16S rRNA sequences, the available cultured isolates define two broad phylogroups that also include 97% of F. prausnitzii 16S rRNA sequences that are detected by direct amplification from human fecal DNA. Our analysis of phylogroup I and II strains from healthy individuals did not reveal systematic differences between the phylogroups with respect to substrate utilization, pH tolerance, or bile sensitivity. Nevertheless, molecular surveys indicate that representatives of the two phylogroups often coexist among the dominant microbiota of individuals (53, 57). There is evidence for reduced representation of F. prausnitzii in active ileal Crohn's disease (50), and it would be of interest in the future to compare the characteristics, including potential interactions with the immune system, of F. prausnitzii strains isolated from CD patients with those from healthy subjects.
Based on our analysis of substrate utilization in 10 cultured strains from seven healthy individuals, most F. prausnitzii strains have the ability to utilize apple pectin for growth. The previous report that F. prausnitzii strains failed to use pectin is most likely to reflect the use of citrus pectin in that study (43). We have shown that F. prausnitzii strains are able to compete for apple pectin as a substrate in the presence of two other known pectin-utilizing species, B. thetaiotaomicron and E. eligens, suggesting that they make a contribution to pectin fermentation in the colon. Our results suggest that this may apply especially at mildly acidic pH values when competition from Bacteroides spp. is reduced (12, 58). The possibility is also raised that certain pectin-rich substrates might be used to develop prebiotic approaches for stimulating F. prausnitzii numbers; interestingly, apple pectin has been shown to promote certain Firmicutes in a recent study with rats (25). Another notable attribute of some F. prausnitzii strains is the utilization of uronic acids for growth, an ability previously thought to be limited to Bacteroides spp. among human gut anaerobes. Further analysis of substrate utilization in this species will undoubtedly be aided by the availability of draft genomes for several of the F. prausnitzii strains studied here. In conclusion, the present findings demonstrate a broad capacity to utilize both diet- and host-derived growth substrates that helps to explain the remarkable abundance of this species within the human colonic microbiota.
ACKNOWLEDGMENTS
We acknowledge the Scottish Government Rural Environment Research and Analysis Directorate for support. Mireia Lopez-Siles was awarded a fellowship from the Spanish Ministerio de Educación to support a research stay at the RINH and is also the recipient of an FI grant from the Generalitat de Catalunya (2010FI_B2 00135), which receives support from the European Union Commissionate. This work was partially funded by the Spanish Ministry of Education and Science through project SAF2006-0041).
We thank Isabelle Laugaudin for contributing to growth tests, Donna Henderson for SCFA analysis, and Pauline Young for bacterial 16S rRNA sequencing. We also thank Anna Plasencia, Corran Musk, and Carles Borrego for their useful suggestions and technical assistance with phylogenetic analysis and Julien Tap, Petra Louis, and Alan Walker for help in identifying representative sequences for F. prausnitzii OTUs from published studies (53, 57) examining 16S rRNA clone libraries.
Footnotes
Published ahead of print 18 November 2011
Supplemental material for this article may be found at http://aem.asm.org/.
REFERENCES
Articles from Applied and Environmental Microbiology are provided here courtesy of American Society for Microbiology (ASM)
Full text links
Read article at publisher's site: https://doi.org/10.1128/aem.06858-11
Read article for free, from open access legal sources, via Unpaywall:
https://aem.asm.org/content/aem/78/2/420.full.pdf
Citations & impact
Impact metrics
Citations of article over time
Alternative metrics
Article citations
The complex link between the gut microbiome and obesity-associated metabolic disorders: Mechanisms and therapeutic opportunities.
Heliyon, 10(17):e37609, 07 Sep 2024
Cited by: 0 articles | PMID: 39290267 | PMCID: PMC11407058
Review Free full text in Europe PMC
Natural Foraging Selection and Gut Microecology of Two Subterranean Rodents from the Eurasian Steppe in China.
Animals (Basel), 14(16):2334, 13 Aug 2024
Cited by: 0 articles | PMID: 39199868 | PMCID: PMC11350848
Investigating the response of the butyrate production potential to major fibers in dietary intervention studies.
NPJ Biofilms Microbiomes, 10(1):63, 30 Jul 2024
Cited by: 1 article | PMID: 39080292 | PMCID: PMC11289085
Next generation probiotics for human health: An emerging perspective.
Heliyon, 10(16):e35980, 12 Aug 2024
Cited by: 0 articles | PMID: 39229543 | PMCID: PMC11369468
Review Free full text in Europe PMC
Exploring the resilience and stability of a defined human gut microbiota consortium: An isothermal microcalorimetric study.
Microbiologyopen, 13(4):e1430, 01 Aug 2024
Cited by: 1 article | PMID: 39115291 | PMCID: PMC11307317
Go to all (213) article citations
Other citations
Data
Data behind the article
This data has been text mined from the article, or deposited into data resources.
BioStudies: supplemental material and supporting data
Nucleotide Sequences (4)
- (1 citation) ENA - JN037417
- (1 citation) ENA - JN037415
- (1 citation) ENA - HQ457025
- (1 citation) ENA - HQ457033
Similar Articles
To arrive at the top five similar articles we use a word-weighted algorithm to compare words from the Title and Abstract of each citation.
Prebiotic potential of pectin and pectic oligosaccharides to promote anti-inflammatory commensal bacteria in the human colon.
FEMS Microbiol Ecol, 93(11), 01 Nov 2017
Cited by: 105 articles | PMID: 29029078
Changes in the Abundance of Faecalibacterium prausnitzii Phylogroups I and II in the Intestinal Mucosa of Inflammatory Bowel Disease and Patients with Colorectal Cancer.
Inflamm Bowel Dis, 22(1):28-41, 01 Jan 2016
Cited by: 68 articles | PMID: 26595550
Mucosa-associated Faecalibacterium prausnitzii phylotype richness is reduced in patients with inflammatory bowel disease.
Appl Environ Microbiol, 81(21):7582-7592, 21 Aug 2015
Cited by: 57 articles | PMID: 26296733 | PMCID: PMC4592880
Diversity, metabolism and microbial ecology of butyrate-producing bacteria from the human large intestine.
FEMS Microbiol Lett, 294(1):1-8, 13 Feb 2009
Cited by: 1046 articles | PMID: 19222573
Review