Abstract
Free full text

Recent advances on tea polyphenols
Abstract
Over the past decade many scientific and medical studies have focused on green tea for its long-purported health benefits. There is convincing evidence that tea is a cup of life. It has multiple preventive and therapeutic effects. This review thus focuses on the recent advances of tea polyphenols and their applications in the prevention and treatment of human cancers. Of the various polyphenols in tea, (−)-Epigallocatechin-3-gallate (EGCG) is the most abundant, and active compound studied in tea research. EGCG inhibits several molecular targets to inhibit cancer initiation and modulates several essential survival pathways to block cancer progression. Herein, we describe the various mechanisms of action of EGCG and also discuss previous and current ongoing clinical trials of EGCG and green tea polyphenols in different cancer types.
2. INTRODUCTION
Lifestyle choices are critical components of cancer prevention, but a most appropriate prototype is yet to be established. According to American Cancer Society there were 1,479,350 new cancer cases recorded with 562,340 deaths in 2009 (1). Despite the many existing treatment approaches, researchers have yet to find a curative strategy for this insidious disease. Interestingly, many epidemiological and experimental studies have demonstrated the protective effects of dietary factors towards human cancers. The plant derived natural polyphenolic compounds are an invaluable treasure from nature, found to block various stages of carcinogenesis including cancer initiation, promotion and progression (2). This observation bolstered chemoprevention as a valuable approach to arrest or delay the multistage process of carcinogenesis prior to the development of malignancy (3).
Tea represents the most widely consumed beverage in the world next to water, and is grown in at least 30 countries around the world. It is distinguished by the presence of catechins, a subgroup of polyphenols, known for their powerful antioxidant properties. Significant data amassed from many laboratories around the world provide convincing evidence that tea consumption has a preventive effect on carcinogenesis. A typical cup of brewed green tea contains in the extract 30-40% catechins. After consumption, tea polyphenols can be detected in both intact forms and as metabolites that could reach sub-micromolar concentrations in blood plasma (4). Absorption appears to take place in the small intestine, with intact metabolites reaching the colon, where they undergo extensive bacterial degradation (5).
Only a number of studies have examined the bioavailability and biotransformation of tea polyphenols and other catechins. In some cases, biotransformative pathways result in metabolites that are more active than the parent drugs (6). It is increasingly important to determine plasma and tissue levels of catechin metabolites and their biological significance to gain insight into therapeutic and preventative effects. Thus understanding the full array of mechanisms of tea polyphenols can facilitate the design of improved strategies for preventing and treating cancer.
In addition, a better understanding of the mechanisms of action will provide a rationale for the clinical development of tea polyphenol alone or in combination strategies. The initial mechanism-based studies using combinational and molecular cancer therapies focused on inhibition of mutagenesis (7), angiogenesis (8), and cell proliferation (9). It has been found that in human plasma the maximum concentration of the green tea supplement would reach upon 4,400 pmol/ml (10). This concentration of EGCG would be sufficient to exert antioxidant and other biological activities in the bloodstream. With this known many health products including beverages, foods, health care products, and cosmetics are now supplemented with the extracts of green tea.
In the present review, we will thus discuss the recent advances of tea polyphenols and their applications in the prevention and treatment of human cancers. Much emphasis is given to their defined molecular targets and their mechanisms of action. We also discuss previously conducted and ongoing clinical trials of polyphenolic compounds in different cancer types.
3. TEA POLYPHENOLS
3.1. History and tea ingredients
Green tea is the distinctive “liquor” from the evergreen plant Camellia sinensis and is the most ancient beverage in the world, widely known for its refreshing and stimulating effects. It has garnered significant attention both in the scientific world and in the cosmetic industry for health benefits and a wide range of applications. Of the tea produced worldwide, 78% is black tea mostly consumed in Western countries and some Asian countries, and 20% is green tea, commonly consumed in Asia, few parts of North America and Middle East. Remaining 2% is oolong tea, which is mainly produced in southern China. The production of green tea involves steaming of freshly harvested leaves in order to inactivate enzymes that preclude the fermentation product, thereby producing a dry stable product.
Tea is very rich in polyphenolic constituents which have high anti-inflammatory, antioxidant, and antimutagenic properties in various biological systems. Tea contains large amounts of various flavanoids, which are characterized by having the benzopyrane skeleton, with the pyrane ring bearing at least one aromatic ring. A major class of flavanoids is catechins, which include epicatechin (EC), epigallocaetchin (EGC), epicatechin-3-gallate (ECG), and epigallocatechin-3-gallate (EGCG) (11) (12) (Figure 1). EGCG is the most abundant of the catechins, and accounts for 50-75% of the total amount of catechins. Other catechins, such as catechin gallate, gallocatechin, gallocatechin galllate, epigallocatechin digallate, methylepicatechin and methyl EGC are present in smaller quantities. Flavanols, including quercetin, kaempferol, myricetin, and their glycosides are also present in tea. One bag of green tea contain between 80 and 100 mgs of the polyphenols with EGCG accounting for about 25 to 30 milligrams. Other ingredients in green tea include threonine, which constitute about 4-6% weight of dried tea and is responsible for its characteristics flavor. Catechins are present in higher quantities in green tea as compared to black and oolong tea, because of the different methods of leave processing after harvesting.
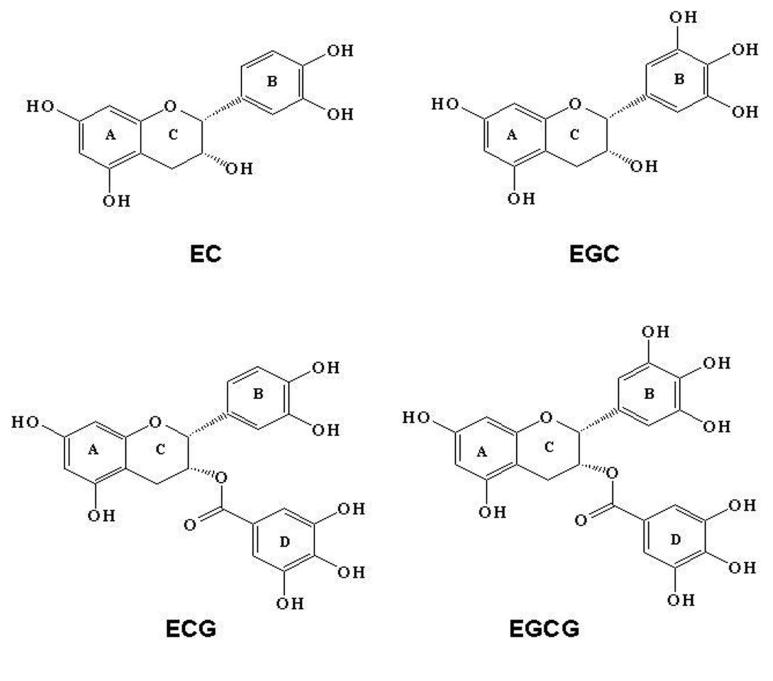
Chemical structures of major green tea polyphenols: The polyphenols that exist in green tea are mainly (+)-epicatechin (EC), (−)-epigallocatechin (EGC), (−)-epicatechin gallate (ECG), and (−)-epigallocatechin gallate (EGCG). EGCG is the most powerful and the most abundant of the major catechins found in green tea.
The manufacturing of black tea involves crushing of the leaves to promote oxidation and subsequent condensation of tea polyphenols in a process known as fermentation, which leads to the formation of theaflavins (TFs) and thearubigins. A benzotropolone ring structure is present in the flavins and is responsible for its unique black tea taste and bright red-orange color. Thearubigins, which have higher molecular weight, are poorly characterized chemically and biochemically.
3.2. Pharmacokinetics and bioavailability of green tea
The detailed pharmacokinetics of tea catechins in humans and rodents have been studied (13-14). The bioavailability of one of the most active polyphenolic constituents is poorly defined. Oral bioavailability in humans cannot be estimated due to the lack of an available IV formulation. Various processes such as gastrointestinal degradation/metabolism, poor membrane permeability, and transporter-mediated intestinal secretion/efflux may contribute to poor bioavailability of tea polyphenols. The plasma levels of EGCG, EGC and EC after intragastric administration of decaffeinated green tea is found to be 0.1, 14 and 31%, respectively (15). It has been shown that treatment of rats with green tea polyphenols in their drinking fluid results in increase of plasma levels of EGC and EC, which were higher than that of EGCG over a 14 day period (16). Whereas the tissue levels of green tea in male Sprague Dawley rats (300 grams), that were given 0.6% green tea in their drinking water for 14 days, had highest concentration of EGCG in large intestine (487.8 +/−121.5 ng/g), while the concentration of EGC was highest in bladder (810.4 +/−229.4) that approximated to 1.1 microM EGCG and 2.6 microM EGC (16). According to Zhang et al. non-gallate catechins are more susceptible to efflux as compared to gallate catechins. The possible mechanism of elimination of these green tea catechins is by transporter mediated intestinal efflux (17). The bioavailability of tea polyphenols with large molecular masses such as theaflavins (564-868 Da) is low, compared to EGCG (458 Da) that has limited bioavailability and smaller molecules, EGC and EC (306 Da and 290 Da, respectively), that have higher bioavailability. Tea polyphenols are rapidly methylated by S-adenosyl-methionine, catalyzed by the enzymes catechol-O-methyltransferase (COMT) inside the body. UDP-glucuronoryltransferase (UGT) and sulphotransferase (SULT) enzymes also catalyzed the tea polyphenols to form glucuronide and sulpahte conjugates of catechins (18).
Fewer studies have been conducted on the biological activities of the metabolites of green tea polyphenols. Under in vitro conditions, EGCG is biologically more active in cancer cells towards growth inhibition as compared to their metabolites. The polyphenolic structure of EGCG also makes them good hydrogen donors, allowing EGCG to bind tightly to proteins and nucleic acids. Recently, EGCG has been shown to bind strongly to Bcl-2 protein, laminin receptor, vimentin and proteasome, contributing to its anticancer activities (19-20). These studies may provide important insights into the design of future strategies aiming towards the development of green tea extracts (GTE) or green tea polyphenols (GTP) as a better chemopreventive agent.
3.3. Chemical properties of tea constituents
3.3.1. Antioxidant nature of green tea polyphenols (GTPs)
Emerging evidence has placed considerable emphasis on the antioxidative properties of tea polyphenols. Tea polyphenols prevent the formation of reactive oxygen species (ROS) and are strong metal ion chelators. The strong antioxidant nature of tea polyphenols is attributed to their polyphenolic structure (21). The B ring consisting of vicinal dihydroxy or trihydroxy groups in GTPs is the preferred site for antioxidation. The free radical scavenging activities of catechins have been well studied. ROS such as superoxide radical, singlet oxygen, hydroxyl ROS, nitric oxide, nitrogen dioxide, and peroxynitrite are found to be precluded by the scavenging ability of tea polyphenols. EGCG induced inhibition of soybean lipoxygenase served as the earliest experimental evidence for its antioxidative properties (22). It was later reported that EGCG could inhibit 12-O-tetradecanoylphorbol-13-acetate (TPA)-induced oxidative damage in Hela cells, prevented Cu+2-mediated oxidation of low density lipoprotein (LDL), decreased tert-butyl hyperperoxide-induced lipid peroxidation, and blocked the generation of ROS from NADPH-cytochrome P450-mediated oxidation (23). Sato et al reported the structural characteristics for superoxide anion radical-scavenging and productive activities of green tea polyphenols. In the polyphenols with the pyrogallol-type B-ring and/or galloyl group, electron-withdrawing substituents (carbonyl and ketal carbons) and/or intramolecular hydrogen bonding constituted structural characteristics against the autoxidation reaction. Though the O2.-productive activity partially counteracted O2.-scavenging activity, such structural characteristics appeared to enhance the scavenging activity (24). Neshchadin et al used CIDNP spectroscopy (CIDNP=chemically induced dynamic nuclear polarization) to provide a deeper understanding of the antioxidant mechanism and model hydrogen abstraction reactions with four catechin-based polyphenols: catechin (C), gallocatechin (GC), epigallocatechin (EGC), and epigallocatechin gallate (EGCG). They reported that hydrogen abstraction is an essentially stochastic process with a slight preference for the B rings in the catechin-based polyphenols (25). Luczajet et al reported the action of polyphenols against oxidative stress formation in endothelial cells. Endothelial cells pretreated with 100 mM tert-butyl hydroperoxide (t-BHP) for oxidative stress formation, subjected to EGCG, TFs and black tea extract, were partially protected against oxidation of t-BHP causing statistically significant increase in GSH-Px activity, GSH and tryptophan level and a decrease in MDA and dityrosine levels in comparison with human vein endothelial cells (HUVEC) pretreated with t-BHP group (26). Supplementation of GTP and black tea polyphenols (BTP) as a sole source of drinking solution to Winstar rats leads to scavenging of ROS by 72% and 69%, respectively (27). The presence of the vicinyl hydroxy groups also makes them more susceptible to air oxidation under alkaline and neutral pH.
In addition to their reduction potential and antioxidant abilities, GTPs can actively trap the reactive carbonyl species (RCS) (28). Extremely reactive RCS such as glyoxal (GO), methylglyoxal (MG), and 3-deoxyglucosone (3-DG), generated from the Maillard reaction, readily modify lysine, arginine, and cysteine residues of proteins, forming advanced glycation end products (AGEs). AGEs are associated with chronic and age-related diseases such as diabetes and diabetes complications, confirmed by several epidemiological and large prospective clinical studies. The binding site for RCS trapping is the A ring of the catechins, whereas the preferred sites for antioxidant activity in the B ring. (29).
Polyphenolic structures in GTPs favors the formation of strong H-bonds. These H-bonds mediate the biochemical effects of GTPs by enabling them to bind strongly with proteins and nucleic acids. Recent studies using NMR spectroscopy revealed that GTPs could directly bind to the BH3 domain of anti-apoptotic BCl-2 family of proteins (30) and mediate their cancer preventive effects by induction of apoptosis. Molecular modeling studies showed that EGCG binds and inhibits the proteasome (31) and urokinase plasminogen activator (uPA), a matrix remodeling protein thereby preventing tumor metastasis (32). On the other hand the large hydration shell affects their absorbability.
3.3.2. EGCG structure and activity
EGCG is a major constituent of green tea and various studies have demonstrated that EGCG can inhibit carcinogenesis and prevent metastasis in established tumors. The polyphenolic structure of EGCG consists of 4 rings, A, B, C and D (Figure 1). A and C rings constitute the benzopyran ring. This benzopyran ring has a phenyl group at C2 and gallate group at C3 positions. The B ring of EGCG has vicinal 3,4,5-trihydroxy groups, and the D ring galloyl moiety in EGCG is in the form of an ester at C3. The presence of this ester carbon makes EGCG highly susceptible to nucleophilic attack (Figure 1).
The precise molecular targets and the exact anti-cancer mechanism of EGCG are not clearly defined. However, convincing evidence suggests the proteasome is one of the molecular targets for EGCG. Regulated proteolysis via the proteasome pathway is important for the integrity of intracellular biochemical events. Inhibition of the ubiquitin-proteasome pathway (UPP) has emerged as a promising strategy in the prevention and treatment of human cancer. It was found that EGCG could potently and specifically inhibit the chymotrypsin-like activity of the proteasome both in vitro and in cultured tumor cells (31). The A-ring of (−)-EGCG-containing two hydroxyl groups at C5 and C7 positions facilitates binding to Lys 32 and Ala 146 in the hydrophobic S1 pocket of the Beta5-subunit of proteasome. Irreversible transfer of the gallate moiety from EGCG to the hydroxy oxygen of Thr 1 results in the inhibition of proteasome chymortypsin-like activity. In silico docking studies predict that electrophilic carbonyl carbon of (−)-EGCG is oriented in a suitable position for nucleophillic attack by the hydroxyl group of N-terminal Thr 1 of the Beta5-subunit of the proteasome, thus inhibiting the proteasomal chymotrypsin-like activity (33). The hydroxyl groups on D-ring forms hydrogen bonds with Gly 47 and Ser 131 of the proteasome which further augments the binding stability of (−)-EGCG to the proteasome (34).
The number of hydroxyl groups on the B-ring and D-ring influences the potency of EGCG for proteasome inhibition. Thus analogs involving modifications of the A ring and B ring of EGCG as proteasome inhibitors has been examined (35). It was found that in the B ring, a decrease in the number of OH groups led to decreased potency. However, the addition of a hydrophobic benzyl group in the C8 position had no significant affect on the potency.
The D-ring (galloyl group) composed of one benzoyl and three phenoxy groups in EGCG shows significant antiproliferative activity in PC-9 human lung cancer cells (36). The hydroxyl groups of the galloyl moiety were also discovered to be essential for cell growth inhibition, indicating that the galloyl group greatly contributes to its activities. There was a strong antiproliferative effect by alkyl gallate and gallamide derivatives towards human leukemia HL-60 cells through inducing apoptosis (37). Various bisgallate and bisgallamide derivatives were synthesized and tested for antiproliferative activity towards HL-60 cells. In gallamide derivatives having a short alkyl chain, the additional galloyl group enhanced the antiproliferative activity but in gallate derivatives, the addition of a galloyl group had no effect on the antiproliferative activity.
3.3.3. Stability
EGCG is less stable in neutral and alkaline medium because the hydroxyl groups on the phenyl ring are attacked by the basic medium leading to the formation of a more active phenoxide anion. Autooxidation of EGCG results in the generation of superoxide anion and hydrogen peroxide. This can be prevented in cell culture which can be stabilized by the addition of superoxide dismutase (38). Many of the reported activities could thus be attributed to the production of ROS. For example induction of apoptosis reported in H661 lung cancer cells by EGCG could be blocked by the presence of catalase (39). Recently Smith et al (40) investigated the ability of one preformulation method to improve the oral bioavailability of EGCG and found that forming nanolipidic EGCG particles improves oral bioavailability in vivo by more than two-fold over free EGCG. When EGCG was encapsulated in polylactic acid (PLA)-polyethylene glycol (PEG) nanoparticles, the resulting nano-EGCG was reported to retain its biological efficacy when compared to non-encapsulated EGCG, with over 10-fold dose advantage both in cell culture system and in vivo settings in athymic nude mice implanted with human prostate cancer cells (41).
3.3.4. Effect of substitutions on different rings
As mentioned, EGCG is very unstable in neutral or alkaline medium, and this instability leads to a low bioavailability. To enhance the stability of EGCG, peracetate protection groups were introduced on the reactive hydroxyls of EGCG. Analogs involving protected OH-groups called Pro-EGCG(1) (Figure 2) show a higher level of potency compared to natural –(−)EGCG. As expected, these prodrugs fail to show any inhibitory activity toward purified 20S proteasome, but show increased proteasome-inhibitory activity in intact leukemic cells compared to its parent compound. To improve the stability and potency of (−)-EGCG, OH groups from D-ring were replaced with one or two fluorines. The novel fluoro-substituted (−)-EGCG analogues were named F-EGCGs (42). Prodrug of fluoro-substituted (−)-EGCG at meta-position on the phenyl ring (Pro-F-EGCG2) or difluoro-substituted (−)-EGCG at both meta- and para-positions on the phenyl ring (Pro-F-EGCG4, Figure 2) had similar or even more inhibitory potency as Pro-EGCG (1) to induce apoptosis in cultured human breast cancer cells.
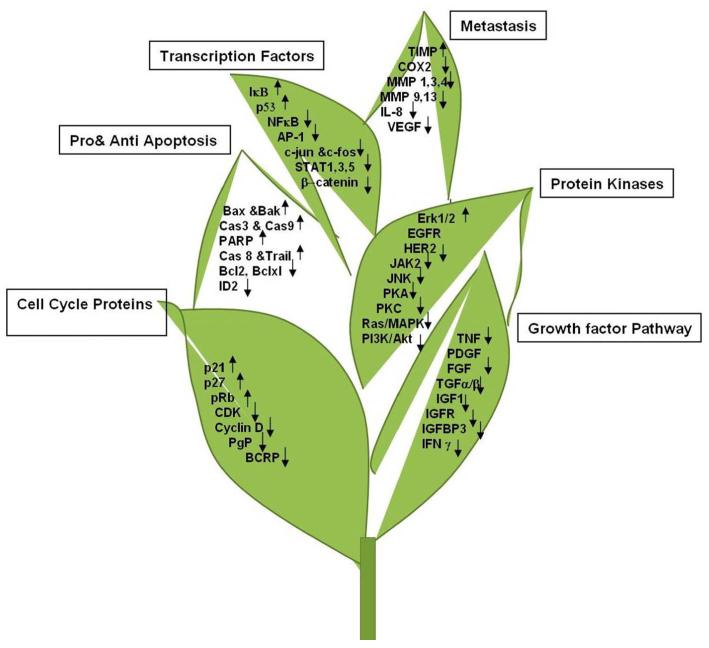
Chemical structures of EGCG analogues 5 and 7 differ in their number of hydroxyl groups; Peracetate-protected EGCG [Pro-EGCG (1)] is more stable and has improved potency over parental compound EGCG. EGCG analogs with fluoro or amino substitutions also inhibit tumor growth with similar potency to that of Pro-EGCG.
Analogs of (−)-EGCG containing a para-amino group on the D-ring (p-NH2EGCG, Figure 2) in place of the hydroxyl groups were tested for their proteasome-inhibitory activities (43). The O-acetylated (−)-EGCG analogs possessing a p-NH2 [or p-NHBoc (Boc; tert-butoxycarbonyl)] in the D-ring (9) act as novel tumor cellular proteasome inhibitors. Their potency was similar to natural (−)-EGCG and (−)-EGCG peracetate (1). Inactivating processes such as methylation render EGCG inactive. Methylation of (−)-EGCG occurs by catechol-O-methyltransferase (COMT), an enzyme widely distributed throughout the body. Synthetic analogues were synthesized with no hydroxyl groups at C5 & C7 of the A ring in EGCG (44). In MDA-MB-231 breast cancer cells with high COMT pro-drug 8 of synthetic analog 7 was more active than the prodrugs of EGCG and analog 5 because it is not a substrate of COMT (Figure 2).
4. EPIDEMIOLOGICAL STUDIES
4.1. Gastrointestinal cancer
The incidence of gastrointestinal cancers has risen rapidly over the past three decades in the United States and northern Europe. Early correlative and cohort studies indicated that tea consumption either negatively or positively associated with stomach cancer (45). In a population based case-control study conducted in Taixing, Jiangsu province of China, green tea reduced the risk of developing stomach cancer by 81% and esophageal cancer by 39% in alcoholics. It also reduced the incidence of stomach cancer by 16% and esophageal cancer by 31% among cigarette smokers (46). In a case-control study conducted in China, drinking green tea was found to reduce the risk of esophageal and gastric cancers (47).
4.2. Breast cancer
Worldwide, breast cancer is the most commonly diagnosed cancer in women representing 16% of all cancers and its incidences continues to increase. It has been suggested that tea consumption reduces the risk of mammary cancer. In a population-based case-control study of breast cancer in Chinese, Japanese and a Filipino-American woman, the risk of breast cancer was found to be considerably reduced by intake of green tea (48). A population-based cohort study of 74,942 Chinese women suggests that regular drinking of green tea may delay the onset of breast cancer. In terms of breast cancer prevention, the studies are inconclusive. In one large study, researchers found that drinking tea of any type was not associated with a reduced risk of breast cancer. On the other hand, when the researchers broke down the sample age of women under 50, those who consumed 3 or more cups of tea per day were 37% less likely to develop breast cancer compared to women who didn’t drink tea. Wu et al reported that women who possessed at least one low activity COMT allele have an inverse association between tea intake and breast cancer risk. In contrast the women who were homozygous for high activity COMT allele, a risk of breast cancer did not differ between tea drinkers and non tea drinkers (49).
4.3. Pancreatic cancer and ovarian cancer
Pancreatic cancer is one of the most aggressive and treatment-refractory malignancies in humans. In one clinical study researchers compared non-drinkers of green tea with drinkers and found that those who drink green tea regular were significantly less likely to develop pancreatic cancer. A population-based cohort study was conducted in Japan to investigate the substantial impact of green tea or coffee consumption on pancreatic cancer risk in general (50). However, it is not clear from this population-based study whether green tea is solely responsible for reducing pancreatic cancer risk.
Ovarian cancer is prevalent in industrialized nations, with the exception of Japan. In the United States, females have a 1.4% to 2.5% (1 out of 40-60 women) lifetime chance of developing ovarian cancer. A population-based Swedish Mammography Cohort study demonstrates that tea consumption is associated with a reduced risk of epithelial ovarian cancer in a dose-response manner (51). An interesting clinical study conducted in China found that women with ovarian cancer who drank at least one cup of green tea per day survived longer than those who were not green tea drinkers (52).
4.4. Prostate cancer
The second leading cause of cancer deaths among men in the Western countries is prostate cancer. Many factors, including genetics and diet, have been implicated in the development of prostate cancer. Over the last two decades various epidemiological studies have suggested that green tea consumption correlates with lower risk of prostate cancer. Similarly, the prostate cancer risk declined with increasing frequency, duration, and quantity of green tea consumption in case-control study conducted in southeast China (53). However, of two cohort studies investigating the risk of prostate cancer, one found no association between green tea consumption and prostate cancer risk (54) whereas the other found a positive association (55). Recently, a large cohort study was conducted in Japan found that drinking >5 cups of green tea per day showed reduced risk of developing advanced cancer compared to those having 1 cup per day. Three other cohort studies have been also performed by the same group and found no statistically significant effect in reducing the risk of prostate cancer (56). These studies suggest that a well-designed intervention trial may be able to provide a clear demonstration about the possible cancer-preventive effect of tea polyphenols (see section 7.1. Chemoprevention trials)
4.5. Lung cancer
Lung cancer is one of the deadliest forms of cancer in both men and women in terms of incidence. In vitro green tea polyphenols have been shown to inhibit the growth of human lung cancer cells. A few clinical studies have investigated the link between green tea consumption and lung cancer in people and these studies have been conflicting. On the association of tea and lung cancer, around 20 studies were published, including 6 cohort studies. Two of the cohort studies used data for cancer mortality instead of incidence. The overview of all studies on tea and lung cancer is fairly symmetrical, although it appears slightly skewed toward a protective association of tea intake. When tea intake and lung cancer were studied among never- or former smokers to eliminate the confounding effect of smoking, 4 of 7 reported associations were significantly protective. Well-designed cohort studies, in particular for catechins, are needed to strengthen the evidence on the effects of long term exposure to physiological doses of dietary flavanoids. Another population-based cohort study in Japan among 41,440 men and women, aged 40-79 years, found no evidence that green tea consumption is associated with lung cancer (57).
5. MECHANISM OF ACTION
Signal transduction pathways are critical for mediating biological processes and maintaining cellular homeostasis. Thus disruption of these complex pathways is associated with various diseases such as cancer. Green tea polyphenols (GTPs) mediate their cancer preventive effects through regulation of these multiple signaling pathways, resulting in altered expression of genes that are involved in cell proliferation, angiogenesis and apoptosis. Therefore it is important to delineate the molecular mechanisms by which GTPs, specifically EGCG, modulate signaling pathways and exert their cancer preventive effects (Figure 3).
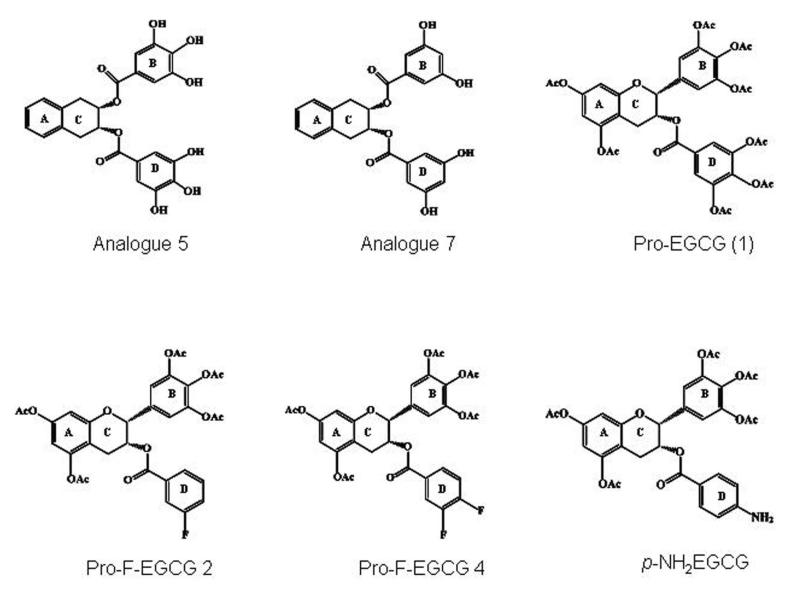
Mechanisms of action: EGCG modulates several key regulators of cell cycle progression, apoptosis, transcription factors, and various signaling pathways that are involved in tumor growth, angiogenesis and metastasis. Recent advances on tea polyphenols: chemistry, biology, molecular targets and application in cancer prevention and treatment
5.1. Cell cycle arrest & induction of apoptosis
Families of proteins known as cyclins and cyclin dependent kinases (cdks) are critical components for regulating the cell cycle in eukaryotes. Several investigators have shown that EGCG can affect the expression of these cell cycle regulators and inhibit the cell cycle (56). It has been shown that EGCG induces cell cycle arrest and apoptosis in several cancer cell lines including lung, colon, prostate and skin cancer with minimal effect toward normal cells (56, 58). EGCG induced cell cycle arrest at G2/M phase in both CaSki and HeLa cell lines. Treatment with 25 microM or 50 microM EGCG for 24 h led to significant G2/M arrest and sub-G1 population production. The EGCG was found to be increased sub-G1 from 2.24% to 23.54% in CaSki cells and 1.96% to 11.64% in HeLa cells, which indicates apoptosis occurred in human cervical cancer cells (59). In another finding, it was demonstrated that EGCG significantly increased the cell percentage in sub-G1 phase, which was (73.5−/+4.4)% after a 48-h EGCG treatment toward human oral epithelial cancer cell line KB cells. Studies by Liang et al have showed that EGCG decreases the expression of cyclin D1 and increases the expression of cdk inhibitors like p21 and p27 thereby inhibiting the activity of cdks, resulting in cell cycle arrest (60).
Apoptosis involves a series of biochemical events, leading to a variety of morphological changes, including changes in the cell membrane. As one of several mechanisms, EGCG has been shown to inhibit the expression of anti-apoptotic proteins Bcl-2 & Bcl-XL while increasing the expression of Bax and Bak pro-apoptotic proteins (61). It has been reported that EGCG effectively inhibited cellular proliferation and induced apoptosis of SW1353 and CRL-7891 chondrosarcoma cells. Expression levels of Bcl-2 were significantly decreased and the levels of Bax were significantly increased (62). It was also reported that EGCG-induced apoptosis of human laryngeal epidermoid carcinoma Hep2 cells, which proceeds through a caspase-independent, p53-mediated pathway (62). EGCG induced cell cycle arrest and apoptosis in Ewing family tumors (EFT) cells which correlated with altered expression of Bcl-2 family proteins, including increased expression of proapoptotic Bax and decreased expression of prosurvival Bcl2, Bcl-XL, and Mcl-1 proteins (63). Britschgi et al reported in acute promyelocytic leukaemia (APL), EGCG treatment resulted in a significant increase of death-associated protein kinase 2 (DAPK2) levels and 67 kDa laminin receptor expression, associated with increased cell death. All-trans retinoic acid (ATRA) and EGCG co-treatment significantly boosted neutrophil differentiation, and 67LR expression correlated with susceptibility of acute melocytic leukaemia (AML) cells to EGCG (64).
Human prostate cancer cell lines LNCaP and PC-3 express high levels of a hyperphosphorylated Bcl-XL protein in mitochondria. Using a combination of nuclear magnetic resonance binding assays, fluorescence polarization assay, and computational docking studies one research group investigated the interaction of tea polyphenols with Bcl-XL and Bcl-2 proteins. They found that certain green tea catechins such as (−)-EGCG and (−)-ECG were very potent inhibitors (Ki in the nanomolar range) of Bcl-XL and Bcl-2. This study revealed that tea polyphenols could recognize the BH3 domain on Bcl-2 family of proteins, suggesting that EGCG could inhibit the activity of anti-apoptotic Bcl-2 family of proteins by directly binding to their BH3 domain (30).
It was reported that EGCG triggers the intrinsic apoptosis pathway by regulating the mitochondrial functions, activating caspase-3 and caspase-9 and cleaving PARP (65). In gastrointestinal stromal tumor cell line GIST-T1 including imatinib-resistant cells, it was reported that EGCG inhibits cell growth and causes caspase-dependent cell death (66). In addition Hastak et al reported induction of apoptosis through modulation of p53 stabilization in LNCaP cells by EGCG, consequently activating the downstream targets p21/WAF1 and Bax (67). In human bladder cancer cells, EGCG suppressed cell growth and induced apoptosis in a dose-dependent manner (68). In androgen-independent DU-145 prostate cancer cells, the inhibitor of DNA binding 2 (ID2), known as a dominant anti-retinoblastoma (Rb) helix-loop-helix protein, was found to be down regulated 4-fold by EGCG treatment. Expression of ID2 resulted in increased survival and decreased apoptosis, indicating ID2 as a target in EGCG-mediated apoptosis (69). In cervical cancer cell lines Caski and Hela, EGCG also induced cell cycle arrest and apoptosis (59).
Another promising cancer target is Tumor necrosis factor-related apoptosis-inducing ligand (TRAIL). In human melanoma A375 cell line EGCG was reported to substantially enhance caspase-8 and TRAIL mediated apoptosis (70). EGCG was also reported to mediate its growth inhibitory effects via pRb-E2F/DP pathway, an important regulator of cell cycle arrest and apoptosis (71).
Additionally, EGCG exerts growth inhibitory effects and disruption of cell cycle regulation in androgen-dependent and androgen-independent prostate cancer cells (9). Recent finding demonstrates that addition of cupric acid to one of the catechins [(2R,3S)-3′,4′,5,7-Tetrahydroxyflavan-3-yl decanoate; catechin-C10] induces significant apoptosis in U937 cells. In addition to this C10 was found to induce apoptosis in human colon cancer (HCT116) cells while it showed resistance to human keratinocytes (HaCat) (72). In breast carcinoma cell line resistant to tamoxifen (MCF-7Tam cells), EGCG down-regulated the activity of two molecules Pg-p and BCRP that play a key role in drug metabolism and transport and that are highly expressed in MCF-7Tam cells (73).
5.2. EGCG affects Ras/MAPK pathway
Ras is a small GTPase associated with multiple signaling pathways (74). Mutations in the ras gene and constitutively active ras lead to cellular transformations by activating the growth signal transduction pathways (75). Oncogenic ras is observed frequently in many cancers. Mitogen activated protein kinases (MAPK) are a group of serine/threonine kinases that act as signal transducers of cell proliferation that are activated by various extra cellular stimuli. Three well known MAPKs are extracellular signal regulated kinases (ERKs), stress activated or c-Jun N-terminak kinase (JNKs/SAPKs) and p38 kinases (76-77). EGCG has been reported to regulate various molecules in the MAPK pathway thereby inhibiting cancer cell survival. Chung et al in 2001 (78) reported that EGCG inhibited Ras/MAPK pathway. In HT1080 cells EGCG inhibited the phosphorylation of extracellular regulated kinases 1 and 2 (ERK1/2) (79). In human epidermal keratinocytes pretreatment with EGCG inhibited the phosphorylation of ERK1/2, JNK and p38 induced by H2O2 (80). EGCG inhibits UVB-induced c-fos expression in a human keratinocyte cell line HaCaT by significantly inhibiting the activation of p38 MAPK (81). EGCG also triggers JNK mediated apoptosis in p57 negative oral carcinoma cells (82). It was also reported that EGCG inhibited the activation of beta1 integrin-downstream molecules such as FAK, AKT and ERK as well as the expression of beta1 integrin itself (83). Shim et al. found that EGCG interacted with the Ras-GTPase-activating protein SH3 domain-binding protein 1 (G3BP1) with high binding affinity (Kd = 0.4 micromol/L) and interfered with the interaction of G3BP1 and the Ras-GTPase-activating protein and further suppressed the activation of Ras. Their results revealed that EGCG suppresses lung tumorigenesis by effectively attenuating G3BP1 downstream signaling, including ERK and MAPK/ERK kinase, in wild-type H1299 and shMock H1299 cells which contain an abundance of the G3BP1 protein but had little effect on knockdown shG3BP1-transfected H1299 cells (84).
5.3. Phosphoinositide 3 kinase (PI3 kinase)/Akt pathway
PI3kinase/Akt is a pro-survival signaling pathway. Three different isoforms of Akt are present in mammalian cells. Growth factor receptors activate PI3 kinase which phosphorylates the inositol ring of phosphoinositol to give PIP3. PIP3 binding to Akt and subsequent translocation to plasma membrane assists in phosphorylation of Akt by PI3 dependent kinases (PDK) 1 and 2. Active Akt inhibits apoptosis by phosphorylation of apoptotic proteins like Bad and caspase-9 (85-86).
Akt is frequently found activated in many human cancers (87). Activation of Akt is associated with increased proliferation and resistance to apoptosis. EGCG has been shown to inhibit PI3K/Akt pathway in many cancers like breast, prostate and cervical cancers. In the benign skin tumor keloid fibroblasts, EGCG inhibited phosphorylation of PI3K, ERK1/2, and STAT3 (Tyr705 and Ser727). This study demonstrated that EGCG suppresses the pathological characteristics of keloids through inhibition of the STAT3-signaling pathway and proposed the potential of EGCG in the treatment and prevention of keloids (88). Cheng et al reported that EGCG inhibits both tyrosine and serine phosphorylation of STAT1 and its translocation into the nucleus in IFN-gamma-stimulated human oral cancer cells. Phosphorylation of PKC-delta, JAK-1, and JAK-2, which are the upstream events for the activation of STAT1, were also inhibited by EGCG in IFN-gamma-stimulated human oral cancer cells (89). In pancreatic cancer EGCG has been reported to inhibit downstream signaling and activation of PI3K/Akt pathway by inhibiting tyrosine kinase phosphorylation of PDGF receptor (90). In MDA-MB-231 breast cancer cell line and YCU-H891 head and neck squamous cell carcinoma cell line, EGCG could inhibit the constitutive activation of EGFR, Stat3 and Akt (91). In T24 bladder cancer cell line, EGCG treatments lead to enhanced apoptosis by modulation of Bcl-2 family and inhibiting PI3K/Akt pathway (61). In prostate cancer DU154 and LNCaP cells, both EGCG and TF treatment were found to (i) decrease the levels of PI3K and phospho-Akt and (ii) increase Erk1/2 in both cells. Modulation of the constitutive activation of PI3K/Akt and Erk1/2 pathways by EGCG as well as TF could be one of the anti-cancer effects of EGCG (92). EGCG inhibited mouse mammary tumor virus (MMTV)-Her-2/neu NF639 cell growth by reducing the signaling via PI3K/Akt to NF-kappa B pathway because of inhibition of basal Her-2/neu receptor tyrosine phosphorylation (93).
5.4. Inhibition of insulin like growth factor (IGF)-1 pathway
IGF pathway operates via peptide hormones, their cell surface receptors, and a family of six distinct IGF binding proteins (IGFBPs) that determine the activity by modulating the bioavailability of IGFs in circulation. Heterotetrameric IGF-R1 transmembrane glycoprotein has an intracellular tyrosine kinase domain that phosphorylates various targets within the cell. IGF-R1 initiates a signaling cascade in response to the mitogens IGF-1 and IGF-2 that regulate cell proliferation, differentiation and apoptosis. IGFBPs and IGF-R2 neutralize IGF action by regulating the internalization and degradation of IGF-2. Increased expression of IGF-R1 and low levels of IGFBPs are associated with an increased risk of cancer and are thus considered as potential therapeutic targets (94).
Several investigators thus focused on the effects of GTPs on IGF signaling pathway. Effects of GTPs, specifically EGCG, on IGF-R1 tyrosine kinase activity in malignant cell growth were found to be highly inhibitory (95). In TRAMP mice there was a significant increase in IGFBP-3 while decreased levels of IGF-1 were observed in response to GTP (96). It was also reported that EGCG caused a transient increase in TGF-beta, an inducer of IGFBP-3, and decreased the mRNA levels of proteins that proteolyses IGFBP-3 in human colon cancer HT29 cells (97). Kang et al showed that treatment of Ewing family tumors (EFT) cell lines with EGCG blocked the autophosphorylation of IGFIR tyrosine residues and inhibited its downstream pathways including phosphoinositol 3-kinase-Akt, Ras-Erk, and Jak-Stat cascades. Effects of EGCG were associated with dose- and time-dependent inhibition of cellular proliferation, viability, and anchorage-independent growth, as well as with the induction of cell cycle arrest and apoptosis (63). Thus EGCG has been reported to exert its anticancer effects by inhibiting IGF signaling pathway.
5.5. Inhibition of metastasis
Degradation of the extracellular matrix by proteolytic enzymes is crucial for tumor invasion and metastasis. Urokinase Plasminogen activator (uPA) catalyzes the cleavage of plasminogen to plasmin, which in turn facilitates the release of several proteolytic enzymes (98). It has been shown that GTPs could modulate the secretion of uPA and inhibit the invasive behaviour of breast cancer cells by suppressing the constitutively active transcription factors AP-1 and NF-kappa B (99).
Further to uPA, matrix metalloproteinases (MMP’s) are structurally related zinc-dependent endopeptidases that are involved in ECM degradation and remodeling. Several investigations have reported that MMPs have anti-apoptotic properties, and aid in tumor progression and metastasis by promoting angiogenesis, tumor cell proliferation and differentiation. Thus MMPs and MAPK pathway that regulates the expression of MMPs are considered as a potential targets for chemotherapy (100). Not surprisingly cancer-preventive effects of EGCG have been reported in human salivary gland adenocarcinoma cells. EGCG inhibits the invasion and migration by inhibiting MMP-2 and MMP-9 expression and enzymatic activity in a dose-dependent manner (96). It is also reported to inhibit MMP-9, thereby preventing the metastasis of lung cancer cells (101). In rat hepatic stellate cells EGCG was reported to cause a decrease in MMP-2 mRNA and protein levels (102). In human tendon derived fibroblasts, IL-1B induced expression of collagenases, MMP-1, MMP-3, MMP-13 and stomelysin was inhibited by EGCG and EGC (103). Activities of secreted MMP-2 and MMP-9 were also inhibited by EGCG (104). In human gastric cancer AGS cells, activation of phorbol myristate 13-acetate (PMA) induced upstream regulators of AP-1, ERK and JNK was abrogated by EGCG thus inhibiting the invasiveness and MMP-9 expression of these cells (105). In the prostate of TRAMP mice oral administration of GTP significantly reduced the expression of MMP-2 and MMP-9 (106). MMP suppression mechanisms of EGCG also include increasing the expression of tissue inhibitors of MMPs (TIMPs) (107). Jackson et al reported that tannic acid, EGCG and ECG (but not GA) strongly inhibited collagen degradation at low micromolar concentrations by strongly binding and stabilizing collagen via extensive hydrogen bonding, augmented by some hydrophobic interactions and preventing the free access of collagenase to active sites on the collagen chains (108). Thus regulation of AP-1, MAPK pathway and inhibition of MMPs in several cancers by EGCG contributes to its overall cancer preventive effects.
5.6. EGCG affects transcription factors
5.6.1. Nuclear factor kappa B (NF-kappa B)
NF-kappa B is a sequence-specific transcription factor sensitive to oxidative stress. Under resting conditions I-kappa B binds NF-kappa B and localizes it in the cytoplasm of the cell. NF-kappa B inducing kinase/I-kappa B kinase regulates I-kappa B phosphorylation. Phosphorylation of I-kappa B releases active NF-kappa B that translocates to the nucleus and induces the expression of over 200 genes. Many of these genes suppress apoptosis and can induce proliferation. Deregulation of the pathway and aberrant activation of NF-kappa B or constitutively active NF-kappa B is frequently found in many human cancers (109). Hence inhibiting NF-kappa B has potential for cancer prevention. EGCG inhibited NF-kappa B activation in UV induced normal human epidermal keratinocytes (110). In A431 epidermoid cancer cells I-kappa B levels were increased in a dose/time dependent manner of EGCG while inhibiting the nuclear translocation of NF-kappa B (111). In human bronchial epithelial cells EGCG could suppress NF-kappa B activation along with other pro-survival pathways (112).
5.6.2. Activator protein 1 (AP-1)
c-Jun and c-fos are oncogenes that encode for AP-1, a transcription factor, whose activity has been associated with invasive and metastatic characteristics of cancer cells. They are immediate-early genes, whose transcription is induced rapidly in response to external stimuli. These oncogenes are components of signal transduction pathways that function to stimulate cell proliferation. Researchers have shown that EGCG inhibits the activity of AP-1 through the inhibition of mitogen-activated protein kinase (MAPK), specifically, through inhibition of c-Jun NH2-terminal kinase (JNK) dependent activity in JB6 cells and H-ras transformed JB6 cells (113). In mouse epidermal JB6 cell line with mutant H-ras gene, both the galloyl structure on the B ring and the gallate moiety of catechins inhibited cell growth by exerting inhibitory effects on AP-1 activity (114). In Ha-ras-transformed (21BES) human bronchial epithelial cells EGCG and theaflavin digallate (TFdiG) are reported to induce rapid apoptosis by decreasing c-jun protein phosphorylation resulting in lowered AP-1 activity, which may contribute to the growth inhibitory activity of tea polyphenols (115). Chen et al (81) reported that in human keratinocyte cell line HaCaT cells, EGCG could inhibit UVB-induced c-fos expression, a major component of AP-1, and prevent AP-1 activation which is important for tumor promotion.
6. MOLECULAR TARGETS OF EGCG
6.1. Cyclooxygenase
One of the mediators of inflammation is prostaglandins. Cyclooxygenases (COXs) are enzymes that catalyze the rate limiting step during prostaglandin biosynthesis from arachidonic acid. COXs family consists of three enzymes COX-1, COX-2 and COX-3. COX-1 is ubiquitously expressed in mammalian tissues and is involved in homeostasis. COX-3 functions are unknown but it is found in the brain and spinal cord. More recent emphasis has been laid on COX-2 as its expression is found to be up regulated in inflammation and in cancer. In many tumors it has been reported that COX-2 plays a role in tumorigenesis by modulating cell proliferation and apoptosis (116). EGCG inhibited COX-2 expression in colon cancer cells by activating AMPK pathway (117). In androgen-dependent LNCaP cells and androgen-independent PC-3 cells of human prostate cancer, both mRNA and protein levels of COX-2 were inhibited by EGCG (118). Supplementation of GTP and BTP as the sole source of drinking solution to Winstar rats lead to scavenging of ROS by inhibition of COX-2 and inactivation of phosphorylated NF-kappa B and Akt (27). Thus EGCG exerts its antiproliferative effects via COX-2 inhibition.
6.2. Telomerase
A number of different approaches have been developed to inhibit telomerase activity in human cancer cells. It has been shown that telomerase is over expressed in many human cancers and is important for maintaining the telomere nuclear protein endcaps of the chromosome. Telomerase inhibition was seen both in a cell-free system (cell extract) as well as in living cells with a concentration of 1 microM (IC50) and 15 microM of EGCG. In cell-free systems at neutral pH, high nanomolar to low micromolar concentrations of EGCG inhibited telomerase activity. EGCG (5–10 microM) was reported to inhibit telomerase and induce cell senescence after long-term treatment (119). The possible implication was that EGCG decomposes to form a galloyl radical, which can covalently modify telomerase. The effect of EGCG on telomerase activity and growth rate was determined by using two different types of tumor U937 monoblastoid leukemia cells and HT29 colon adenocarcinoma cells. Interestingly, treatment of mice with 1.2 mg of EGCG per day for 80 days bearing telomerase-positive colon cancer xenografts (HCT-L2) resulted in a 50% inhibition in tumor size whereas mice bearing telomerase-negative tumors of the same parent cell line (HCT-S2R) shows no response to EGCG treatment (119).
The inhibition of the cancer-associated enzyme telomerase is a key mechanism involved in cancer inhibition by epigallocatechin gallate (EGCG), a major tea polyphenol. In healthy cells telomeres lose up to 300 bp of DNA per cell division that ultimately leads to senescence; though, most cancer cells bypass this lifespan restriction through the expression of telomerase. The telomerase has catalytic subunit, hTERT essential for its proper functioning and has been shown to be expressed in approximately 90% of all cancers. EGCG-treatment repressed telomerase activity and induced apoptosis in laryngeal squamous cell carcinoma Hep 2 cells (120). EGCG can inhibit cell growth by inducing apoptosis in MCF-7 breast cancer cells and is due to decreased hTERT transcription. These conclude that EGCG leads to progressive demethylation of the hTERT promoter including E2F-1 binding sites resulting in an increase in binding of this repressor. While HL60 promyelocytic leukemia cells when exposed to EGCG reduced cellular proliferation and induced apoptosis but no decrease in hTERT mRNA expression (120). The prolonged oral administration of EGCG to nude mice models bearing both telomerase-dependent and -independent xenograft tumors cloned from a single human cancer progeny shows that only the telomerase dependent tumors respond to the treatment (121).
6.3. Tumor suppressor p53
Tumor suppressor genes generally follow the two-hit hypothesis that if only one allele for the gene is damaged, the second can still produce the correct protein. p53 is a tumor suppressor protein that in humans is encoded by the TP53 gene. p53 mutations are found in almost all tumor types, where they contribute to the complex network of molecular events leading to tumor formation. (−)-EGCG in particular, can stabilize p53, inducing G1 phase cell cycle arrest and apoptosis (122-123). In another similar study 80 mg/ml of -(−) EGCG treatment for 8 hours in bovine aortic smooth muscle cells showed increased nuclear p53 protein levels for up to 9 folds (124). EGCG induced stabilization of p53, which caused an up-regulation in its transcriptional activity, thereby resulting in the activation of its downstream targets such as p21WAF1 and Bax and the induction of apoptosis in prostate carcinoma LNCaP cells. The erlotinib treatment activates p53, which plays a critical role in synergistic growth inhibition by erlotinib and EGCG via inhibiting NF~B signaling pathway in squamous cell carcinoma of the head and neck (125).
6.4. Vascular endothelial growth factor (VEGF)
One of the important factors for angiogenesis is a vascular endothelial growth factor (VEGF). Overexpression of VEGF can be seen in cancers and enables tumor to grow and metastasize. Neo-vascularization process required for the growth of tumors beyond a few millimeters in diameter, and also for tumor invasion and metastasis (126-127). Jung et al showed that angiogenesis can be inhibited by EGCG through blocking the induction of VEGF in human carcinoma cells. These results showed that (−)-EGCG inhibited tumor volume by 61% compared with (−)-EC treatment, as well as reduction in tumor vessel counts and increase in apoptosis after treatment with (−)-EGCG (128). Tea polyphenols decrease serum levels of prostate-specific antigen, hepatocyte growth factor, and vascular endothelial growth factor in prostate cancer patients. In vitro it also inhibits the production of hepatocyte growth factor and vascular endothelial growth factor. Another study shows that EGCG inhibits the expression of HIF-1-alpha, which strongly activates VEGF expression (129) at both the mRNA and protein levels in colorectal cancer cells. The inhibition of HIF-1-alpha is associated with the inhibition of ERK and Akt phosphorylation, because HIF-1-alpha protein synthesis is regulated by PI3K/Akt and MAPK/ERK pathway activation. Because the noticeable decrease in cellular HIF-1-alpha levels occurred within 3 hours after the addition of EGCG and preceded the inhibition of VEGFR-2 phosphorylation. The author further explained that EGCG inhibits activation of the VEGF/VEGFR axis, by suppressing the expression of HIF-1-alpha and several major growth factors in five different cell lines of colorectal cancer (130).
6.5. Proteasome
The proteasome is a multicatalytic protease responsible for the degradation of most cellular proteins and now is recognized as a novel and promising target for chemotherapy. The proteasome regulates the cell growth, survival, and metastasis of cancer cells making it an attractive target for new drugs. The eukaryotic proteasome contains three catalytic beta subunits responsible for the chymotrypsin (CT)-like (beta-5), trypsin (T)-like (beta-1), and peptidyl-glutamyl peptide-hydrolyzing (PHGH)-like (beta-2) (131). We and others have reported that inhibition of CT–like activity is associated with induction of apoptosis in various tumors (132-133).
We reported that (−)-EGCG potently and specifically inhibited the chymotrypsin-like activity of the proteasome in vitro (IC50 = 86-194 nM) and in vivo (1-10 microM) at the concentrations found in the serum of green tea drinkers and induced tumor cell growth arrest in G1 phase of the cell cycle while having little or no effect on normal, nontransformed cells. We also reported for the first time by using in silico docking methods, that ester bond within (−)-EGCG played a critical role in its inhibitory activity of the proteasome. We found that synthetic (−)-EGCG amides and (−)-EGCG analogs, with modifications in the A-ring, C-ring or ester bond, inhibited the chymotrypsin-like activity of purified 20S proteasome with altered potencies, induced growth arrest in the G1 phase of the cell cycle in leukemia Jurkat T cells, and suppressed colony formation of human prostate cancer LNCaP cells (134). In an effort to discover more stable and potent analogs, we synthesized several (−)-EGCG analogs with −OH groups eliminated from the B- and/or D-rings. We also synthesized the putative prodrugs with −OH groups protected by acetate that can be removed by cellular cytosolic esterases. We then examined the structural activity relationship between the compounds that are protected and those that are not protected. We found that decreasing −OH groups from either the B- or D-ring leads to diminish proteasome-inhibitory activity in vitro. The protected analogs were able to inhibit the proteasomal chymotrypsin-like activity by 97% in cultured tumor cells.
In vivo EGCG is subjected to rapid biotransformation, such as methylation by catechol-O-methyltransferase (COMT) that limits its action. The challenge in developing (−)-EGCG for cancer prevention and therapy is its low bioavailability, partly due to its poor absorption and its instability under neutral or alkaline conditions (i.e., physiologic pH) and partly due to biologically inactivating processes such as methylation. We have shown that Pro-EGCG (1), as a novel prodrug, converts to a cellular proteasome inhibitor and anticancer agent in nude mice after treatment for 31 days. Our recent study demonstrate that a pro-drug of a simple synthetic analog 5, the acetate 6, enhanced the cytotoxic activity of 5 just as pro-EGCG (1) enhanced the growth inhibitory activity relative to EGCG. An even simpler analog 7, though not as potent an inhibitor of purified proteasome as EGCG or 5, was found to be a better proteasome inhibitor when incubated with MDA-MB-231 cell extracts (44) (Figure 2). These results also suggest that COMT does play a significant role in affecting the anti proliferative activities of EGCG and its analogs.
7. CLINICAL TRIALS OF GREEN TEA
The effects of tea polyphenols are widely studied for cancer chemoprevention in many different parts of the world. Several excellent reviews compiling results of such studies are available (135). Unfortunately, the lack of a firm conclusion still exists in spite of bundle of reviews and papers that have been written on green tea. One apparent reason is etiological factors for different types of cancers are not the same and the populations studied are different.
From the available data of epidemiologic studies and research findings in laboratory, clinical trials are now warranted to evaluate the usefulness of green tea and polyphenols present therein. The first such trial was conducted by the MD Anderson Cancer Center in collaboration with the Memorial Sloan-Kettering Cancer Center. Research suggests that green tea consumption has promising effects against various types of cancers without inducing major toxicities. Studies using green tea in the treatment of cancers have focused on breast, prostate and lung cancers as well as recently on chronic lymphocytic leukemia (CLL) (136).
7.1. Chemoprevention trials
Cancer chemoprevention, as first defined by Sporn in 1976, uses natural, synthetic, or biological chemical agents to reverse, suppress, or prevent carcinogenic progression (137). Chemoprevention trials are based on the hypothesis that interruption of the biological processes involved in carcinogenesis will inhibit this process and, in turn, reduce cancer incidence (138).
7.1.1. Breast cancer
A randomized phase I clinical trial was conducted by M.D. Anderson Cancer Center studying the side effects and best dose of green tea extract in treating women with hormone receptor-negative stage I, stage II, or stage III breast cancer (NCT00516243). Patients were randomized to 1 of 2 treatment arms. Arm I: Patients received 1 of 3 dosages of oral green tea extract (Polyphenon E) twice daily for 6 months in the absence of disease progression or unacceptable toxicity. Arm II: Patients received oral placebo twice daily for 6 months in the absence of disease progression or unacceptable toxicity. More clinical trials are needed to evaluate its biological activities and molecular targets in patients.
Another phase II trial (NCT00917735) conducted by clinical and Translational Science Institute of University of Minnesota studying the efficacy of green tea extracts in reducing the risk of breast cancer. In this ongoing clinical trial, patients are given two capsules of green tea extracts twice daily. The investigators are evaluating the efficacy of green tea extracts by analyzing the different breast cancer markers such as plasma insulin-like growth factor 1 (IGF-1), IGF binding protein 3 (IGFBP-3), estrone, estradiol, androstenedione, sex hormone binding globulin (SHBG), urinary estrogen metabolites and plasma F2-isoprostanes.
7.1.2. Prostate cancer
In a very interesting study, the effects of green tea on premalignant lesions with a daily treatment of 600 mg green tea capsules in 60 patients with high grade prostate cancer shows 30% incidence among the 30 placebo treated subjects and only 3% incidence among green tea treated subjects. No significant side effects or adverse effects were documented (139).
Another phase II clinical trial conducted by multiple institutions, evaluated the effect of green tea on patients without symptoms of prostate cancer but with biopsy proven malignancy and clinical evidence of androgen independent disease. Patients were given the dose of 6 g/day for 6 months and each dose of green tea powder contains 100 calorie and 46 mg of caffeine. Among 42 patients one patient had shown tumor response defined by 50% decrease in PSA from baseline (229 ng/dl to 105 ng/dl), however this effect was not sustained beyond 2 months (140). The overall response rate was only 2% and after one month the median change in PSA from baseline was found to be increased. Even the radiographic and physical examination shows no biochemical tumor response (141).
A single institutional prospective clinical trial, evaluated the effect of standardized green tea extract (GTE) on 19 patients having progressive hormone-refractory prostate cancer. The median age of the patients in this study was 76 years old and was administrated 250 mg of green tea extract twice daily. Each capsule contains 30% of EGCG and less than 2% caffeine. Out of 19 patients, 15 took GTE for 2 months and remaining was found to have progressive disease. Only one patient demonstrated a modest decrease of PSA but that lasted for only two months. An issue related with study was lower dose of GTE. According to pharmacokinetics studies of Polyphenon E 400 to 1200 mg of EGCG should be given and thus it is 2 to 8 fold less than the actual dose 500mg/day. In summary this study provides some valuable insights that patients with late stage of hormone refractory prostate cancer are not the ideal population for the treatment of green tea.
7.1.3. Lung cancer
While green tea polyphenols have been shown to inhibit the growth of human lung cancer cells in test tubes, few clinical studies have investigated the link between green tea consumption and lung cancer in people and even these studies have been conflicting. A pilot study was designed to determine the maximum tolerated dose (MTD) of green tea extract (GTE) in patients with advanced lung cancer. 17 patients with advanced lung cancer were registered to receive once-daily oral dosing of GTE at a starting dose of 0.5 g/m2 per day. The GTE was administered daily once by mouth for 4 weeks. The drug was provided by Ito En (Tokyo, Japan) in 110 and 270 mg soft gelatin capsules. The GTE capsules contain 13.9% of EGCG and 6.8% caffeine. No objective responses were seen in this trial. Seven patients had stable disease ranging from 4 to 16 weeks (142). All other patients had no change. In summary, the study of GTE in combination with other chemotherapy drug may show better results.
Data available from various clinical trials on different types of cancer suggests that green tea polyphenol EGCG provides a rationale for exploring this compound in chemoprevention and as hematologic malignancies in prostate cancer.
7.1.4. Chronic lymphocytic leukemia (CLL)
In vitro studies have shown that EGCG can induce apoptotic cell death in leukemic B-cells from a majority of patients with chronic lymphocytic leukemia (CLL). Mayo clinic reported an interesting observation in patients with CLL and low grade lymphoma who self administered over-the-counter green tea products. Patients with steady clinical, laboratory, and/or radiographic evidence of progression prior to initiation of over-the-counter green tea products had developed an objective response after this therapy (143).
Another study was conducted to investigate the optimal dose and tolerability of patients with chronic lymphocytic leukemia (CLL) to Polyphenone E. Three to six patients were given 400 to 2000 mg capsules (twice a day) of Polyphenon E with a standardized dose of epigallocatechin-3-gallate (EGCG) with asymptomatic Rai stage 0 to II CLL. Majority of patients showed decreased levels in absolute lymphocyte count (ALC) and /or lymphoadenopathy in phase I trial. This treatment was very well tolerated and suggests that Polyphenon E merits additional evaluation as a disease-stabilizing agent (136).
7.2. Combinational treatments
Studies using a human carcinoma xenograft model established with resistant human oral epidermoid carcinoma cells indicated that the combination of doxorubicin with EGCG increased the doxorubicin concentration in the tumors by 51% and increased apoptosis in the tumors compared with doxorubicin alone (144). Moreover, the combination of EGCG and tamoxifen has been shown to synergistically induce apoptosis and growth inhibition in MDA-MB-231 human breast cancer cells (145). A randomized phase II trial is studying how well giving erlotinib together with GTE works in preventing cancer recurrence in former smokers who have undergone surgery for bladder cancer.
As a chemosensitizer, EGCG has been shown to increase cisplatin potency by three to six fold in ovarian cancer cells (SKOV3, CAOV3, and C200 cells), including one cell line that exhibits several hundredfold resistance to cisplatin over the other cells. The addition of EGCG amplified the toxicity of cisplatin. The finding suggests that ovarian cancer cells are susceptible to the pro-oxidant activity of EGCG is very important (146). Its capacity to differentially influence the cancerous versus and normal cells may ease the problem of cisplatin toxicity and resistance, a key obstacle in chemotherapy for ovarian cancer.
7.3. Issues related to cancer therapy with green tea
Moderate intake of green tea is generally considered safe. Asians have consumed tea for thousands of years with few dangerous side effects. Drinking large amounts of tea may cause nutritional and other problems because of the caffeine content and the strong binding activities of the polyphenols, which can make it harder for the body to absorb certain medicines and iron supplements. Possible effects from too much caffeine are the major concern with green tea. In mice, it has been found that long-term consumption of low dose caffeine slowed the hippocampus-dependent learning and impaired long-term memory (147).
Another possible reason for potential adverse effects of green tea is the drug and green tea interactions. The combination of green tea and chemotherapy medications, specifically bortezomib (BZM; Velcade) interacts with each other thus preventing apoptosis. Recent studies show that various green tea components, in particularly EGCG and other polyphenols with 1, 2-benzenediol moieties, effectively prevented tumor cell death induced by BZM. Taken together, results indicates that green tea polyphenols may have the potential to reverse the therapeutic efficacy of BZM, namely via direct chemical inactivation of BZM. And thereby preventing its biological activity, that one would expect that not only the therapeutic efficacy but it also emergence some side effects that usually accompany BZM therapy (148). Authors strongly suggest that while investigating on the combinational therapies with natural compounds, potential nutrition-drug interactions should also be kept in mind.
However, these results have not yet been demonstrated sufficiently in studies on humans. On the other hand, there have been reports of both green and black tea extracts stimulating a gene in prostate cancer cells that may cause them to be less sensitive to chemotherapy drugs. Given this potential interaction, people might not drink black and green tea (as well as extracts of these teas) while receiving chemotherapy for prostate cancer in particular.
The adverse effect of green tea consumption has also been studied. One such evidence suggests that green tea has adverse effects on central nervous system (CNS) and gastrointestinal system (GI). The present study investigates the green tea extract on human s with HTLV-1 reported patients. Thirty-seven subjects were followed up for 5 months by measuring HTLV-1 provirus load after daily intake of 9 capsules of green tea extract powder per day. 12 patients were reported difficulties with monthly donation of blood samples and low compliance with the daily intake of GTE. Two clinical trials investigated the efficacy of green tea on different stages of prostate cancer. The aggressive and advanced stage of prostate cancer shows mild, moderate and severe toxicities in GI and CNS (149). According to Jatoi et al (140), 69% of patients reported toxicity but only one patient had experienced the severe confusion and grade IV toxicity.
8. CONCLUSION AND FUTURE DIRECTION
Epidemiologic observations have indicated that tea consumption has beneficial effects in reducing the risk of cancer in certain populations. Although a considerable body of information supports the preventive potential of tea, a proper understanding of mechanisms that reduce risk of disease remains elusive. Based on our understanding of the mechanisms for EGCG as a model compound, we conclude that EGCG is unlikely to have a single target or receptor that is responsible for all the observed activities. As information on the bioavailability of green tea after consumption is limited in humans, studies on absorption, distribution, metabolism of tea polyphenols in animals and humans are needed. Future studies should not only focus on understanding the mechanisms, but also design strategies for the development of green tea extracts as better chemopreventive agents.
ACKNOWLEDGEMENTS
This work is supported by grants from the National Cancer Institute (1R01CA120009, 3R01CA120009-04S1, 1R21CA139386-01, to Qing Ping Dou). We thank Miachel Frezza and Pratik Patel for critical reading of the manuscript.
10. REFERENCES
Full text links
Read article at publisher's site: https://doi.org/10.2741/363
Read article for free, from open access legal sources, via Unpaywall:
https://europepmc.org/articles/pmc3303150?pdf=render
Citations & impact
Impact metrics
Citations of article over time
Alternative metrics
Smart citations by scite.ai
Explore citation contexts and check if this article has been
supported or disputed.
https://scite.ai/reports/10.2741/363
Article citations
Nutrition Intervention and Microbiome Modulation in the Management of Breast Cancer.
Nutrients, 16(16):2644, 10 Aug 2024
Cited by: 1 article | PMID: 39203781 | PMCID: PMC11356826
Review Free full text in Europe PMC
Unveiling the Mechanisms of EGCG-p53 Interactions through Molecular Dynamics Simulations.
ACS Omega, 9(18):20066-20085, 23 Apr 2024
Cited by: 0 articles | PMID: 38737068 | PMCID: PMC11080030
Development of Genome-Wide Intron Length Polymorphism (ILP) Markers in Tea Plant (Camellia sinensis) and Related Applications for Genetics Research.
Int J Mol Sci, 25(6):3241, 13 Mar 2024
Cited by: 0 articles | PMID: 38542215 | PMCID: PMC10969888
Effect of tea polyphenols on intestinal barrier and immune function in weaned lambs.
Front Vet Sci, 11:1361507, 16 Feb 2024
Cited by: 2 articles | PMID: 38435366 | PMCID: PMC10904598
Antimicrobial Potential of Polyphenols: An Update on Alternative for Combating Antimicrobial Resistance.
Med Chem, 20(6):576-596, 01 Jan 2024
Cited by: 2 articles | PMID: 38584534
Review
Go to all (85) article citations
Data
Data behind the article
This data has been text mined from the article, or deposited into data resources.
BioStudies: supplemental material and supporting data
Clinical Trials
- (1 citation) ClinicalTrials.gov - NCT00917735
Similar Articles
To arrive at the top five similar articles we use a word-weighted algorithm to compare words from the Title and Abstract of each citation.
EGCG, green tea polyphenols and their synthetic analogs and prodrugs for human cancer prevention and treatment.
Adv Clin Chem, 53:155-177, 01 Jan 2011
Cited by: 109 articles | PMID: 21404918 | PMCID: PMC3304302
Review Free full text in Europe PMC
Molecular targets for the cancer preventive activity of tea polyphenols.
Mol Carcinog, 45(6):431-435, 01 Jun 2006
Cited by: 79 articles | PMID: 16652355
Review
Natural Polyphenols and their Synthetic Analogs as Emerging Anticancer Agents.
Curr Drug Targets, 18(2):147-159, 01 Jan 2017
Cited by: 25 articles | PMID: 26758667
Review
Molecular mechanisms of green tea polyphenols.
Nutr Cancer, 61(6):827-835, 01 Jan 2009
Cited by: 29 articles | PMID: 20155623 | PMCID: PMC3769684
Funding
Funders who supported this work.
NCI NIH HHS (7)
Grant ID: 1R01CA120009
Grant ID: 3R01CA120009-04S1
Grant ID: R01 CA120009-04S1
Grant ID: R01 CA120009-05
Grant ID: R01 CA120009
Grant ID: 1R21CA139386-01
Grant ID: R21 CA139386