Abstract
Free full text

Interleukin-2 inhibits germinal center formation by limiting T follicular helper differentiation
SUMMARY
T follicular helper (Tfh) cells promote T-dependent humoral immune responses by providing T cell help to B cells and by promoting germinal center (GC) formation and long-lived antibody responses. However, the cellular and molecular mechanisms that control Tfh differentiation in vivo are incompletely understood. Here we show that interleukin-2 (IL-2) administration impaired influenza-specific GCs, long-lived IgG responses and Tfh cells. IL-2 did not directly inhibit GC formation, but instead suppressed the differentiation of Tfh cells, thereby hindering the maintenance of influenza-specific GC B cells. Our data demonstrate that IL-2 is a critical factor that regulates successful Tfh and B cell responses in vivo and regulates Tfh cell development.
INTRODUCTION
The generation of long-lived plasma cells that secrete high affinity antibodies is critical for the establishment of protective immunity to influenza and other pathogens. The selection of long-lived B cells with high affinity BCRs is dependent on events that occur in the germinal center (GC) (Allen et al., 2007; Cyster, 2010; Kelsoe, 1996). GCs are structures found in the B cell follicles of reactive lymphoid tissues and support intense B cell clonal expansion, somatic hypermutation of immunoglobulin genes, affinity maturation of the B cell response and ultimately, the differentiation of both memory B cells and long-lived plasma cells (Allen et al., 2007; Cyster, 2010; Vinuesa et al., 2010). Given that the goal of most vaccination strategies is the generation of long-lived antibody responses (Cox et al., 2004), it is essential that we understand the factors that regulate GC formation and maintenance.
GCs are dependent on help provided by CD4+ T follicular helper (Tfh) cells - a recently defined CD4+ T cell subset with a unique ability to help B cell responses (Crotty, 2011; Yu and Vinuesa, 2010). B cells and Tfh cells provide each other with reciprocal differentiation and survival signals (King, 2011). Tfh cells provide CD40 ligand (Goodnow et al., 2010; Han et al., 1995) and IL-21 to B cells (Bryant et al., 2007; Linterman et al., 2010; Vogelzang et al., 2008) and B cells present antigen and provide ICOS ligand to Tfh cells (Choi et al., 2011; Deenick et al., 2011; Johnston et al., 2009; Nurieva et al., 2009). As a result, all three of these molecules are essential for germinal center formation and long-lived antibody responses (Goodnow et al., 2010). Importantly, the differentiation of Tfh cells depends on the expression of the transcription factor Bcl6, which represses the expression of other lineage-specific transcription factors and promotes the expression of CXCR5 (Johnston et al., 2009; Nurieva et al., 2009; Yu et al., 2009). In turn, CXCR5 facilitates homing to the B cell follicle and germinal center (Hardtke et al., 2005; Haynes et al., 2007). As a result, Bcl6-deficient CD4+ T cells do not properly express CXCR5, fail to migrate into B cell follicles and do not to support GC reactions. Thus, Bcl6 is considered the master regulator of Tfh differentiation (Johnston et al., 2009; Nurieva et al., 2009; Yu et al., 2009). However, the molecular mechanisms that regulate Bcl6 expression and the differentiation of Tfh cells in vivo are incompletely defined (Crotty, 2011; Yu and Vinuesa, 2010).
Recent results demonstrate that CD4+ T cells responding to LCMV can be separated based on the expression of the interleukin-2 receptor α (CD25) into CD25hi and CD25lo subsets (Choi et al., 2011). Importantly, CD25hi T cells do not express Bcl6, suggesting a possible role for IL-2 in the segregation of effector and Tfh cells. IL-2 was originally defined as an essential T cell growth factor that promotes expansion of effector T cells and facilitates CD8+ memory T cell programming (Blattman et al., 2003; Malek, 2008; Malek and Castro, 2010; Williams et al., 2006). However, IL-2 signaling also controls the contraction of T cell responses by enhancing susceptibility of responding T cells to activation-induced cell death (Lenardo, 1991). IL-2 signaling through CD25 enhances CD25 expression and sensitivity to IL-2 and also controls the expression of key transcription factors, such as eomesodermin (Eomes), Blimp1 and Bcl6 in CD8 T cells (Pipkin et al., 2010).
The generation and maintenance of FoxP3-expressing CD4+ regulatory T cells (Tregs) are also dependent on IL-2 (Almeida et al., 2002; de la Rosa et al., 2004; Malek and Castro, 2010; Malek et al., 2002). Tregs suppress the activation of autoreactive T cells, prevent the development autoimmune disease (Campbell and Koch, 2011; Malek et al., 2002) and play an important role in the control of B cell responses (Alexander et al., 2011; Chung et al., 2011; Lim et al., 2005; Lim et al., 2004; Linterman et al., 2011). Given the essential role for IL-2 in the maintenance of Tregs, some investigators are using IL-2 therapy to treat autoimmune disease in mouse models (Grinberg-Bleyer et al., 2010; Humrich et al., 2010). In humans, IL-2 therapy has been used to enhance antitumor immunity (Kovacs et al., 2001; Rosenberg et al., 1985a; Rosenberg et al., 1985b), particularly in combination with B cell depletion in the treatment of non-Hodgkin lymphoma (Friedberg et al., 2002; Gillies et al., 2005; Gluck et al., 2004; Lopes de Menezes et al., 2007). Thus, IL-2 may be used to treat a variety of diseases.
Although IL-2 can modulate effector and Treg cell function by a variety of mechanisms, the role of IL-2 in the control of B cell and Tfh responses remain unclear. Here we tested the role of IL-2 in the GC B cell response to influenza. We found that IL-2 impaired the influenza-specific GC response, reduced the influenza-specific IgG response and prevented the accumulation of influenza-specific Tfh cells. We demonstrated that IL-2 did not directly inhibit GCs, but instead directly suppressed Tfh cell responses. We found that CD25 signaling triggered by IL-2 negatively modulated Bcl6 expression and Tfh cell development. These data demonstrate that the physiological availability of IL-2 is a critical factor that regulates successful Tfh and B cell responses in vivo.
RESULTS
IL-2 treatment impairs GC B cell formation and antibody responses following influenza infection
To determine the role of IL-2 in the B cell response to influenza, we infected C57BL/6 (B6) mice with influenza virus A/PR8/34 (PR8) and treated infected mice with 30,000 U of human recombinant IL-2 (rIL-2) or PBS twice a day starting three days after infection. On day 21 post-infection, we collected sera from rIL-2 treated and control mice and determined the titers of influenza-specific IgG by ELISA (Fig. 1A). We observed a substantial reduction in influenza-specific IgG1 titers in the rIL-2 treated group compared to control mice. To determine whether rIL-2 treatment specifically blocked the generation of influenza-specific IgG-secreting plasma cells that home to the bone marrow (BM) and provide long-lasting immunity to influenza, we enumerated influenza-specific IgG-secreting plasma cells in the BM on day 21 post-infection by ELISPOT. In agreement with the antibody titers, we found that the number of IgG-secreting influenza-specific plasma cells in the bone marrow was reduced in the BM of rIL-2-treated mice relative to control mice (Fig. 1B). Thus, our results indicate that rIL-2 treatment compromised influenza-specific humoral immune responses.
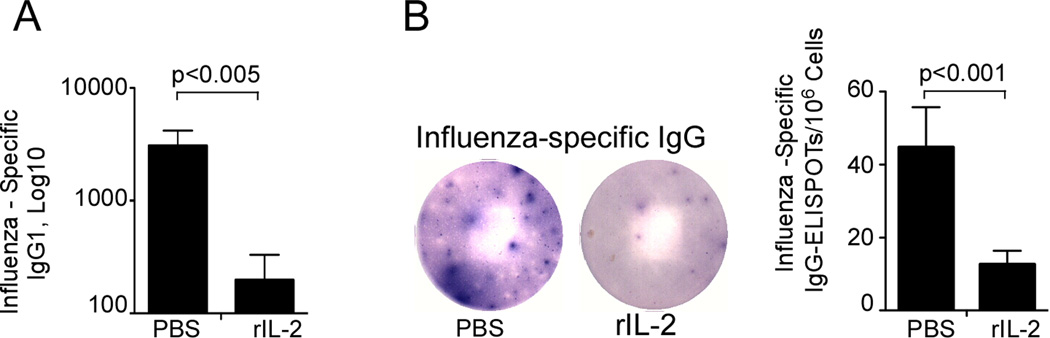
B6 mice were infected with PR8 and treated with 30,000 U of human rIL-2 or PBS daily starting 3 days after infection. (A) Serum was obtained on day 21 after infection and titers of influenza-specific IgG1 were determined by ELISA. (B) Influenza-specific IgG-secreting cells in the BM were enumerated by ELISPOT on day 21 after infection. Data are representative of two independent experiments (mean ± s.d of 5 mice per group).
Given that GC formation is required for the differentiation of long-lived plasma cells (Johnston et al., 2009; Nurieva et al., 2009; Vinuesa et al., 2010; Yu et al., 2009), we hypothesized that rIL-2 treatment may inhibit the formation of GCs following influenza infection. To test this hypothesis, we infected B6 mice with influenza and treated them with either rIL-2 or PBS. On day 10 post-infection, we probed cryosections of mediastinal lymph nodes (mLNs) with fluorochrome-labeled PNA and anti-B220 to detect GCs. As expected, PNA+ GC structures were easily detected in the B cell follicles of control mice. However, although B cell follicles were present in rIL-2-treated mice, PNA+ GC structures were virtually undetectable after rIL-2 treatment (Fig. 2A). Consistent with the histological results, the frequency (Fig. 2B) and number (Fig. 2C) of CD19+PNAhiFAS+ GC B cells were reduced in rIL-2-treated mice.
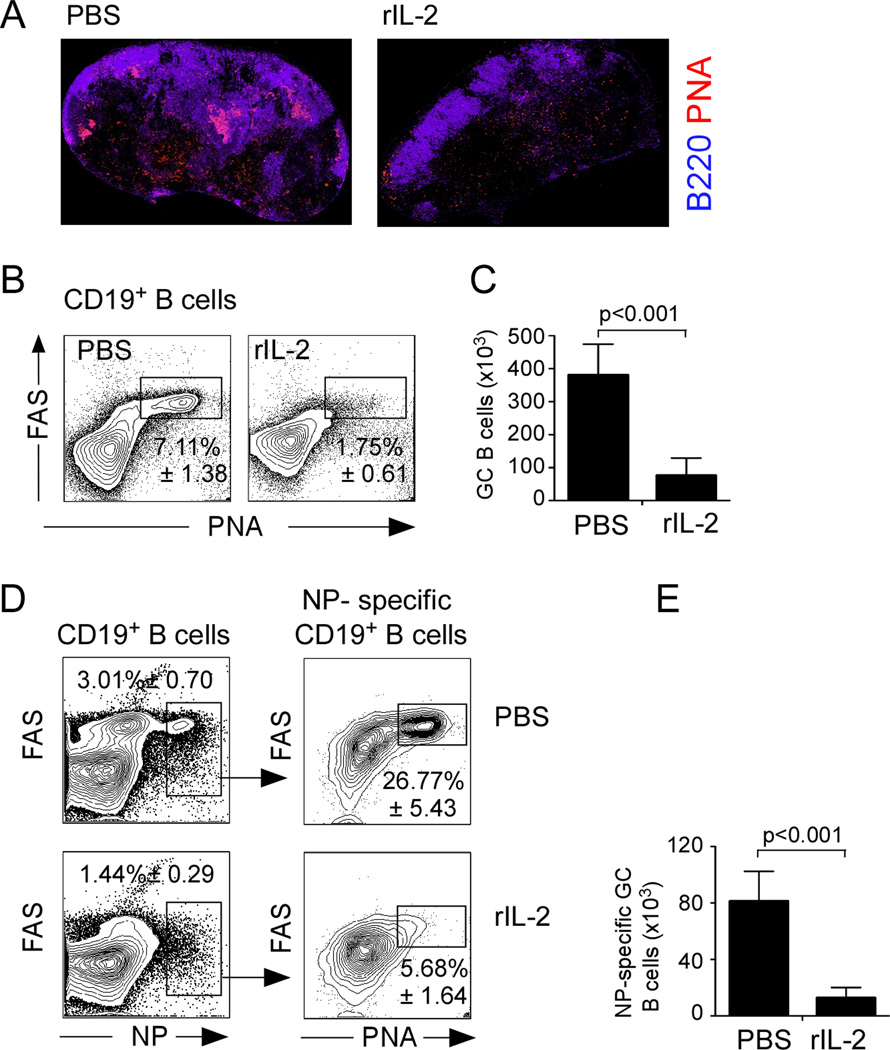
(A) B6 mice were infected with PR8 and treated with 30,000 U of human recombinant rIL-2 or PBS twice a day starting 3 days after infection. mLNs were obtained on day 10 after infection and cryosections were stained with anti-B220 (blue) and PNA (red) and analyzed by fluorescent microscopy. (B–C) Cells from the mLNs of mice treated with IL-2 or PBS were obtained on day 10 and the percentage of CD19+ B cells with a PNA+FAS+ GC phenotype was determined (B), and the number of GC B cells was calculated (C). Data are representative of four independent experiments (mean ± s.d of 5 mice per group). (D–E) Cells from mLN were obtained on day 10 after infection and GC B cells were identified by flow cytometry using an NP-tetramer. The percentage of NP-specific B cells with a PNA+FAS+ GC phenotype was determined (D) and the number of NP-specific PNA+FAS+ CD19+ B cells was calculated (E). Data are representative of three independent experiments (mean ± s.d of 5 mice per group). All P values were determined using a two-tailed Student´s t-test. See also Supp. Fig. 1.
To determine whether the IL-2 treatment impaired the development of influenza-specific GC B cell responses, we used fluorochrome-labeled recombinant influenza nucleoprotein (NP) tetramers to identify NP-specific PNAhiFAS+ GC B cells. We found that rIL-2 treatment reduced the frequency of NP-specific PNAhiFAS+ GC B cells by nearly 80% compared to control mice (Fig. 2D). In a control experiment, we demonstrated that the NP B cell tetramer only bound B cells from influenza-infected mice and not B cells from mice infected with other pathogens (Supp Fig. 1). Collectively, these data show that the influenza-specific GC B cell response was severely compromised in the presence of excess IL-2.
IL-2 indirectly suppresses the GC B cell response to influenza
To determine whether rIL-2 treatment acted directly on GC B cells, we generated 50:50 mixed BM chimeras using B6.CD45.1 bone marrow (BM) and Cd25−/− BM (CD45.2). As expected, the cells that repopulated the chimeric mice were derived equally from both donors (Fig. 3A). We subsequently infected the chimeric mice with influenza, administered either rIL-2 or PBS, and determined the frequency of PNAhiFAS+ GC cells within the B6 and Cd25−/− CD19+ B cells on day 10. We found that B6 (CD45.1) and Cd25−/− (CD45.2) B cells contained equal frequencies of GC B cells in both PBS-treated (Fig. 3B), and IL-2-treated mice (Fig. 3C). Although, the GC B cell response was diminished in the rIL-2 treated chimeras relative to that in the PBS-treated mice (Fig. 3B and C), the ratio of B6 to Cd25−/− GC B cells was close to 1.0 in both groups (Fig. 5D). We observed similar results when we examined NP-specific GC B cell response of B6 and Cd25−/− donors (Fig. 3E–G). These results suggest that IL-2 inhibited the development of GC B cells indirectly and not through a B cell intrinsic mechanism.
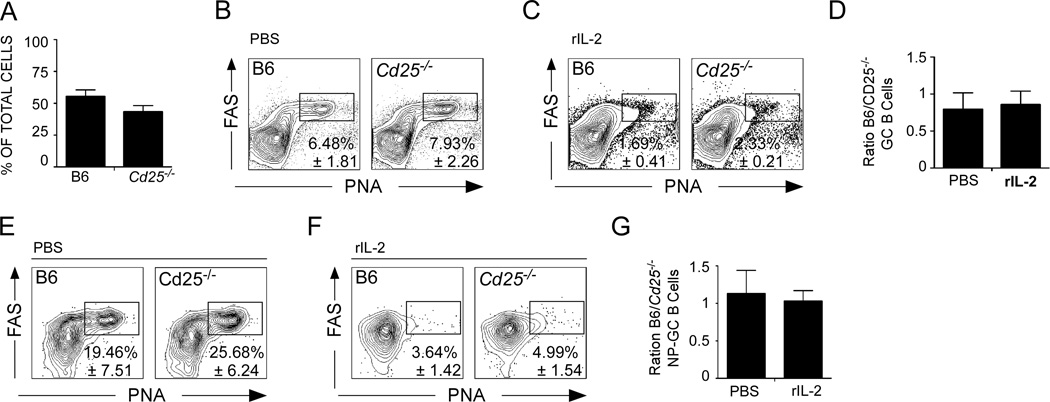
(A–H) B6 mice were irradiated and reconstituted with a 50:50 mix of BM from wild type CD45.1 donors and Cd25−/− donors (CD45.2) and the percentage of leukocytes that expressed either CD45.1 or CD45.2 was determined in the mLN 8 weeks after reconstitution (A). (B–D) Reconstituted mice were infected with PR8, treated daily with 30,000 U of PBS (B) or rIL-2 (C) starting 3 days after infection and the percentage of CD45.1+ or CD45.2+ CD19+ B cells with a PNA+FAS+ GC phenotype cells was determined on day 10. (D) The ratio of B6 to Cd25−/− PNA+FAS+ CD19+ B cells was calculated. (E–G) Reconstituted mice were infected with PR8, treated daily with 30,000 U of rIL-2 or PBS starting 3 days after infection and on day 10 the percentage of CD45.1+ or CD45.2+ NP-specific CD19+ B cells with a PNA+FAS+ GC phenotype was determined in the mLN of mice treated with PBS (E) or with rIL-2 (F). (G) The ratio of B6 to Cd25−/− PNA+FAS+ NP-specific CD19+ B cells was determined. Data are representative of three independent experiments (mean ± s.d of 3–5 mice five mice per group). P values were determined using a two-tailed Student´s t-test.
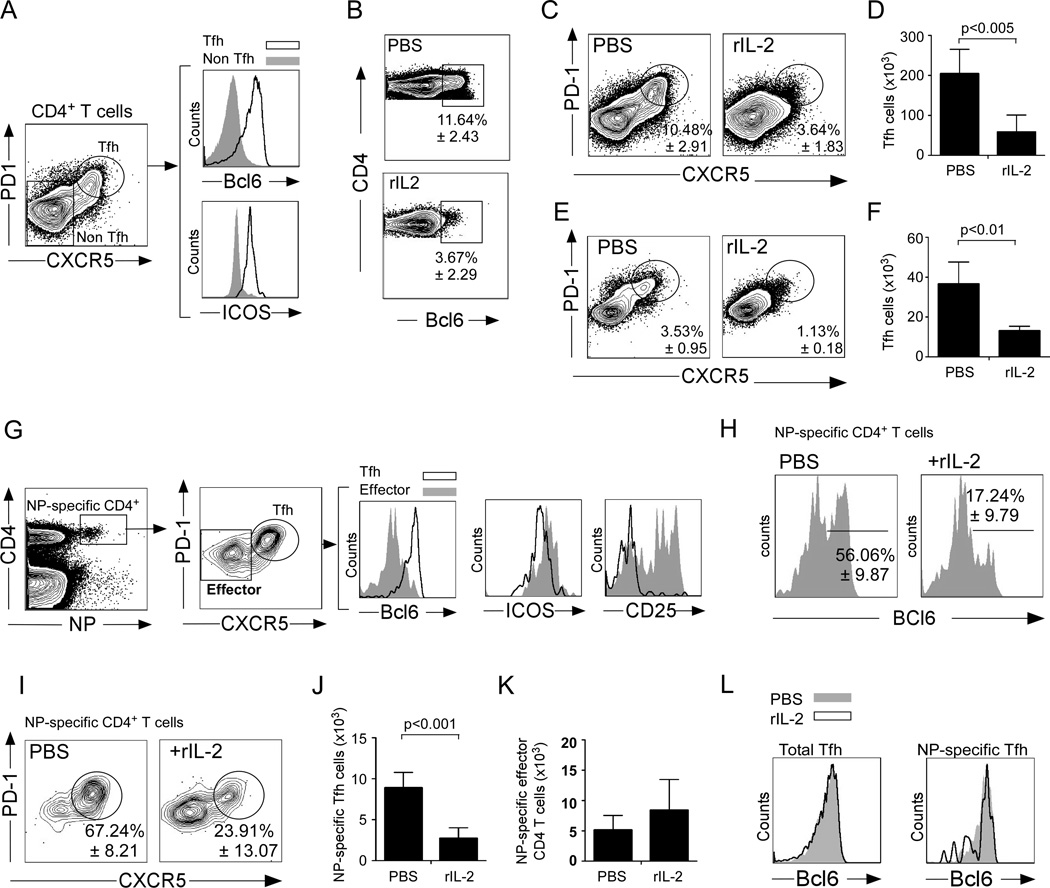
(A) B6 mice were infected with PR8 and the expression of Bcl6 and ICOS was evaluated on PD-1hiCXCR5hiCD4+ T cells and PD-1loCXCR5loCD4+ T cells on day 10. (B–D) B6 mice were infected with PR8, treated with 30,000 U of human rIL-2 or PBS twice a day starting 3 days after infection and cells from the mLNs were analyzed by flow cytometry on day 10. (B) The expression of Bcl6 in CD4+ T cells was evaluated. (C) The percentage of CD4+ T cells with a CXCR5hiPD-1hi Tfh phenotype was determined. (D) The number of CXCR5hiPD-1hi Tfh cells was calculated. (E–F) B6 mice were infected with PR8, treated with 30,000 U of human rIL-2 or PBS twice a day starting 3 days after infection and cells from the mLNs were analyzed by flow cytometry on day 6. (E) The percentage of CD4+ T cells with a CXCR5hiPD-1hi Tfh phenotype was determined. (F) The number of CXCR5hiPD-1hi Tfh cells was calculated. (G) Bcl6, ICOS and CD25 expression was evaluated on PD-1hiCXCR5hi NP-specific CD4+ T cells and PD-1loCXCR5lo NP-specific CD4+ T cells. (H) The expression of Bcl6 in NP-specific CD4+ T cells was evaluated. (I) The percentage of NP-specific CD4+ T cells with a CXCR5hiPD-1hi Tfh phenotype was determined. (J) The number of NP-specific CXCR5hiPD-1hi Tfh cells was calculated. (K) The number of NP-specific CXCR5loPD-1lo effector CD4+ T cells was calculated. (L) The expression of Bcl6 on CD4+CXCR5+PD-1hi cells and NP-specific CD4+CXCR5+PD-1hi cells from IL-2-treated and control mice was evaluated by flow cytometry. Data are representative of four independent experiments (mean ± s.d of 5 mice per group). P values were determined using a two-tailed Student´s t-test.
rIL-2 suppresses the GC B cell response to influenza by a Treg independent mechanism
IL-2 signaling is crucial for the generation and maintenance of FoxP3-expressing Tregs (Almeida et al., 2002; de la Rosa et al., 2004; Malek and Castro, 2010; Malek et al., 2002). Corresponding with this idea, we found more Tregs in influenza-infected mice that were treated with rIL-2 than in control mice (Fig. 4A). Since Tregs suppress B cell immune responses, we next tested whether the inhibitory effect of rIL-2 treatment on GC formation was mediated by enhanced Treg function. Therefore, we treated FoxP3-DTR mice (Kim et al., 2007) with diptheria toxin (DT), infected them with influenza virus and treated them with either rIL-2 or PBS. Treatment of Foxp3-DTR mice with DT efficiently depleted FoxP3 expressing cells even after rIL-2 administration (Fig. 4A). Infected mice were then administered rIL-2 on a daily basis, and we determined the frequency of PNAhiFAS+ GC cells within CD19+ B cells on day 10 after infection. As expected, FoxP3-DTR treated with rIL-2 alone had a decreased frequency (Fig. 4B), and number (Fig. 4C) of GC B cells compared to untreated FoxP3-DTR mice. Importantly, the frequency (Fig. 4B), and number (Fig. 4C) of GC B cells were even more reduced in Treg-depleted mice after rIL-2 treatment. As a control, DT administration to B6 mice did not affect the accumulation of GC B cell after influenza infection (Data not shown). Thus, these results indicate that rIL-2 inhibited GC B cell development by a Treg independent mechanism.
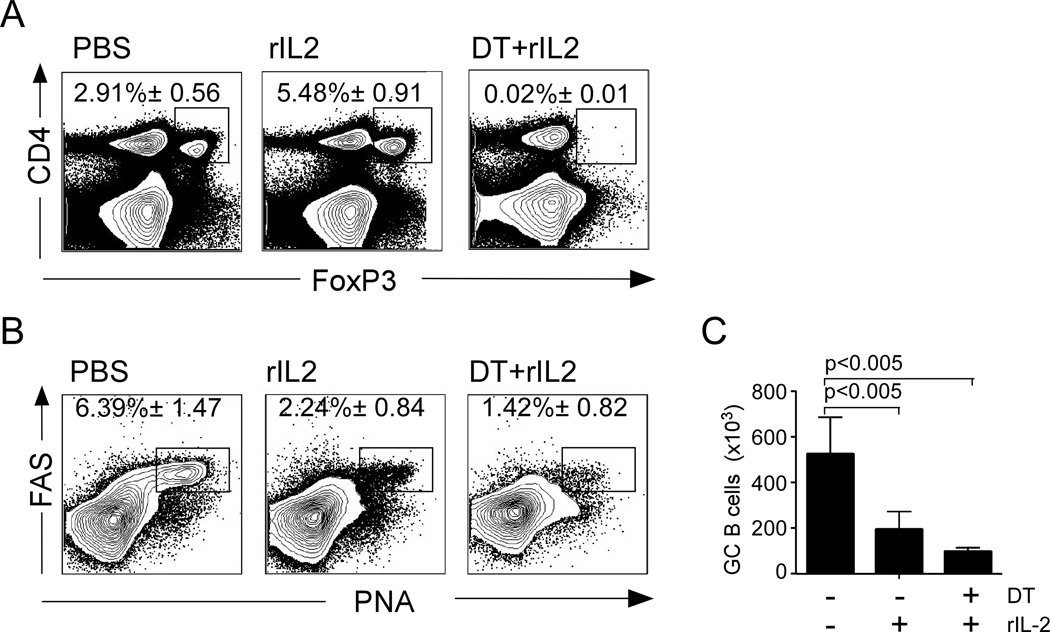
(A) FoxP3-DTR mice were infected with PR8, administered PBS, or DT on days 0, 4 and 7 after infection, or received DT on days 0, 4 and 7 after infection together with 30,000 U of human recombinant rIL-2 twice a day starting 3 days after infection. Cells from the mLN were analyzed on day 10. The percentage of CD4+ T cells that expressed FoxP3 (A), and the percentage of CD19+ B cells with a FAS+PNA+ GC phenotype (B) were determined by flow cytometry. (C) The number of FAS+PNA+ GC B cells was calculated. Data are representative of two independent experiments (mean ± s.d of 5 mice per time point). P values were determined using a two-tailed Student´s t-test.
Treatment of mice with rIL-2 impairs Tfh response
Tfh cells are required for the development and maintenance of GCs (Johnston et al., 2009; Nurieva et al., 2009; Yu et al., 2009). Since GCs are dependent on Tfh cell help, we next considered the possibility that the excess IL-2 compromised GC B cell formation by inhibiting the development of Tfh cells. To test this possibility, we treated influenza-infected B6 mice with influenza, treated them with IL-2 or PBS and enumerated Tfh cells in the mLN on day 10 post-infection. We characterized Tfh cells as PD-1hiCXCR5hi CD4+ T cells (Fig. 5A left panel) that expressed high levels of the transcription factor Bcl6 and the costimulatory receptor ICOS compared to non-Tfh cells (Fig. 5A right panel). Importantly, we found fewer CD4+ T cells that expressed Bcl6 after rIL-2 treatment (Fig. 5B), and both the frequency (Fig. 5C) and number (Fig. 5D) of PD-1hiCXCR5hi Tfh cells were reduced relative to control mice.
To determine whether IL-2 was altering the maintenance of Tfh or preventing their development, we performed a similar experiment, but examined the Tfh response on day 6, which is as early as we can consistently detect Tfh by flow cytometry. We found that IL-2 treatment between days 3 and 6 after infection reduced both the frequency (Fig. 5 E) and number (Fig. 5F) of Tfh cells in the mLN. Thus, IL-2 acts early in the process of Tfh development and probably suppresses the commitment of primed CD4 T cells to the Tfh developmental pathway.
To test whether rIL-2 treatment disrupted Tfh by affecting the number of influenza-specific Tfh cells, we characterized the influenza nucleoprotein (NP)-specific CD4+ T cell response using a fluorochrome-labeled MHC Class II tetramer (Fig. 5G). We found that NP-specific CD4+ T cells could be divided into a PD-1hiCXCR5hi population, which expressed high levels of ICOS and Bcl6 (Tfh cells), and a PD-1loCXCR5lo population, that expressed low levels of ICOS and Bcl6 (CD4+ effectors) (Fig. 5G). Interestingly, whereas NP-specific effector CD4+ T cells were CD25hi, NP-specific Tfh cells were CD25low (Fig. 5G), suggesting that these populations received quantitatively different signals from the IL-2 receptor. We also found that IL-2 treatment reduced the frequency of NP-specific CD4+ T cells that expressed Bcl6 (Fig. 5H), and that the frequency (Fig. 5I), and number (Fig. 5J) of NP-specific Tfh cells were reduced in IL-2 treated mice compared to control. In contrast, rIL-2 treatment did not affect the accumulation of NP-specific effector CD4+ T cells (Fig. 5K). Given the reduced frequency of Bcl6-expressing Tfh cells in IL-2 treated mice, we next determined whether those Tfh remaining also expressed reduced amounts of Bcl6. We found that Bcl6 expression was identical in CD4+CXCR5+PD-1hi cells from IL-2 treated and control mice and in NP-specific CD4+CXCR5+PD-1hi cells from IL-2-treated and control mice (Fig. 5L). Taken together, these results suggest that rIL-2 administration compromised the early commitment to the Tfh lineage rather than affecting already differentiated Tfh cells.
IL-2 directly suppresses the Tfh response to influenza
Since rIL-2 compromised the accumulation of Tfh cells, we next considered the possibility that rIL-2 acted directly on CD4+ T cells and inhibited Tfh development. To test this possibility, we generated mixed BM chimeras that contained a 50:50 mix of B6 and Cd25−/− cells. Two months after reconstitution, we infected the chimeric mice with influenza, administered rIL-2 or PBS, and determined the frequency of Tfh cells within the B6 and Cd25−/− compartments on day 10 after infection. We found a higher frequency of Tfh cells in the Cd25−/− compartment than in the B6 compartment (Fig. 6A). Similar results were obtained when we analyzed the frequency of NP-specific Tfh cells (Fig. 6B). Thus, these results indicated that rIL-2 acts directly on T cells to inhibit Tfh development, and that the loss of Tfh cells is the likely cause of poor GC and antibody responses in rIL-2-treated mice.
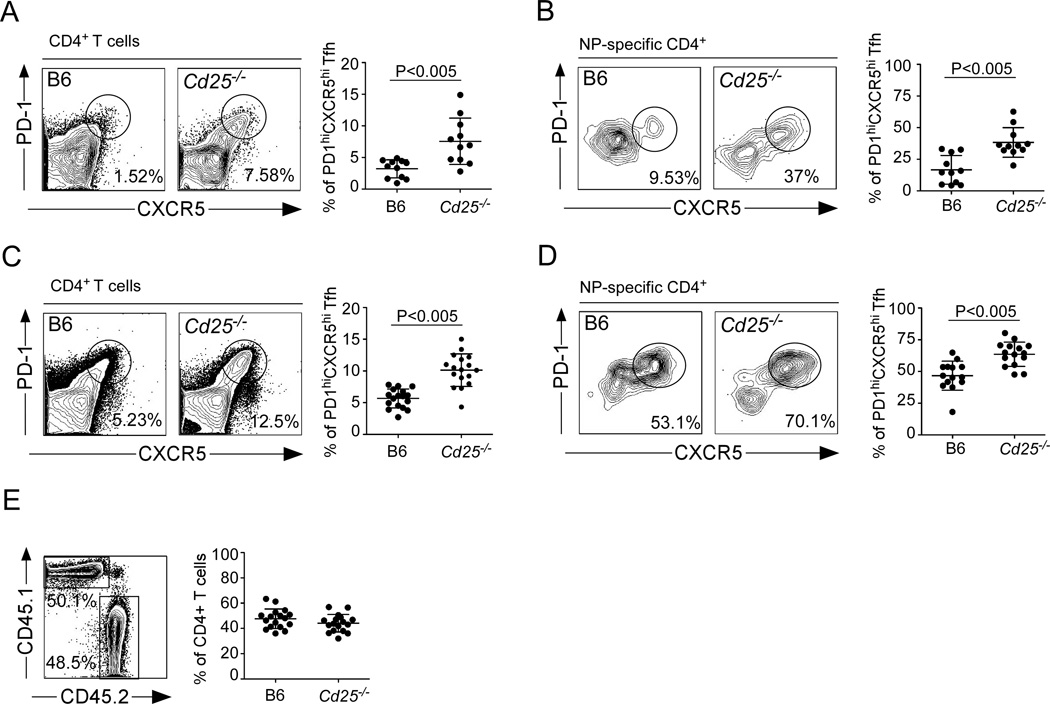
(A–B) B6 mice were irradiated and reconstituted with a 50:50 mix of BM from wild type CD45.1 donors and Cd25−/− donors (CD45.2). Reconstituted mice were infected with PR8, treated daily with 30,000 U of rIL-2 starting 3 days after infection and cells from the mLN were analyzed on day 10. The percentage of CD45.1+ or CD45.2+ CD4+CXCR5+PD-1hi Tfh cells (A) and NP-specific CD45.1+ or CD45.2+ CD4+CXCR5+PD-1hi Tfh cells (B) was determined. Data were pooled from three independent experiments (mean ± s.d ). Representative plots gated on CD4+ T cells are shown. (C–D) B6 mice were irradiated and reconstituted with a 50:50 mix of BM from wild type CD45.1 donors and Cd25−/− donors (CD45.2). Reconstituted mice were infected with PR8, and cells from the mLN were analyzed on day 10. The percentage of CD45.1+ or CD45.2+ CD4+CXCR5+PD-1hi Tfh cells (C) and NP-specific CD45.1+ or CD45.2+ CD4+CXCR5+PD-1hi Tfh cells (D) was determined. (E) The percentage of CD45.1+ or CD45.2+ total CD4+ T cells was determined. Plot is gated on CD4+ T cells. Data were pooled from three independent experiments (mean ± s.d ). Representative plots gated on CD4+ T cells are shown. P values were determined using a two-tailed Student´s t-test.
The previous results suggested that CD25 signaling negatively regulates Tfh development. If this was true, then we would predict a higher frequency of Tfh cells in the Cd25−/− CD4+ T cell population compared to the B6 counterparts - even in untreated mice. To test this hypothesis, we infected B6:Cd25−/− mixed BM chimeras with influenza and calculated the frequency of Tfh cells within the the B6 and Cd25−/− compartments 10 days post infection. We found more Cd25−/− Tfh cells than B6 Tfh cells when we looked at total Tfh cells (Fig. 6C) and NP-specific Tfh cells (Fig 6D). As a control, we also determined the relative frequencies of CD45.1 and CD45.2 cells in the total CD4+ T cell compartment. We found that the frequencies of B6 and Cd25−/− T cells in the 50:50 chimeras were essentially identical (Fig. 6E). Thus, our results indicated that lack of CD25 did not impair CD4+ T cell development, but did promote the differentiation of Tfh cells.
DISCUSSION
Our data shows that the availability of IL-2 regulates the differentiation of Tfh cells and thus controls GC formation and long-lived antibody responses. In conditions when excess IL-2 is available, Tfh cells are suppressed, GCs do not form and influenza-specific IgG responses are reduced. Importantly, the lack of germinal center B cells in IL-2-treated mice is not a consequence of IL-2 signaling in B cells, but is the result of IL-2 signaling in T cells, which impairs Tfh differentiation. Moreover, although IL-2 signaling promotes the accumulation of Tregs, which could suppress Tfh and GCs, Tregs are not required for the ability of IL-2 to repress Tfh differentiation. Thus, our results demonstrate that IL-2 fine-tunes the balance of T effector and B cell helper aspects of the immune response to influenza.
An important functional consequence of impaired Tfh differentiation is the reduced number of GC B cells and influenza-specific IgG-secreting cells. Although we cannot easily measure the affinity of the influenza-specific antibody produced in IL-2-treated mice, we anticipate that the antibodies that are produced would be of low affinity due to lack of selection in the GC. Moreover, in addition to reductions in long-lived, influenza-specific IgG-secreting cells in the BM, we would also anticipate that there would be defects in the generation of influenza-specific memory B cells, as both these cell types are also thought to depend on events that occur in the GC. Thus, the ability of IL-2 to repress Tfh differentiation has multiple effects on the humoral immune response to influenza.
IL-2 is often perceived as a T cell growth factor for both CD4+ and CD8+ T cells (Malek, 2008). However, both CD4+ and CD8+ T cells respond to antigen and proliferate in mice lacking IL-2 or its receptor (Bachmann et al., 1995; Malek and Bayer, 2004; Williams et al., 2006). In fact, IL-2-deficient mice develop autoimmunity due to excessive effector T cell responses (Sadlack et al., 1995; Sadlack et al., 1993; Suzuki et al., 1995). This phenomenon can be attributed, in part, to poor Treg survival in the absence of IL-2 signaling (de la Rosa et al., 2004; Malek et al., 2002). Although Tregs clearly have the ability to impair CD4 T cell responses and even B cell responses (Alexander et al., 2011; Chung et al., 2011; Linterman et al., 2011), the role of IL-2 in preventing Tfh differentiation and GC formation does not appear to be dependent on Treg. Despite the presence of higher numbers of Tregs in IL-2-treated mice, IL-2 still suppresses GC formation when Tregs are eliminated. Moreover, only Cd25+/+ Tfh are suppressed by the addition of IL-2 in B6:Cd25−/− chimeric mice, despite the equal opportunity of Tregs to interact with both B6 and Cd25−/− T cells. Thus, IL-2 acts directly on differentiating Tfh precursor cells, rather than indirectly via Treg-mediated suppression.
The transcription factor Blimp1 controls the terminal differentiation of B cells into antibody secreting plasma cells. As part of this pathway, Blimp1 represses the expression of Bcl6 (Johnston et al., 2009). Similarly, Blimp1 represses Bcl6 expression in T cells and promotes their terminal differentiation into effector cells (Crotty, 2011; Fazilleau et al., 2009; Poholek et al., 2010). Of course, impaired Bcl6 expression in T cells would also preclude the differentiation of Tfh cells (Johnston et al., 2009; Nurieva et al., 2009; Yu et al., 2009). Conversely, Bcl6 over-expression represses Blimp1 and favors Tfh cell development (Johnston et al., 2009). Thus, the balance between Blimp1 and Bcl6 expression is thought to control the relative commitment of CD4 T cells to effector or Tfh pathways (Crotty, 2011) (Choi et al., 2011).
Interestingly, Blimp1 expression is increased in response to IL-2 (Gong and Malek, 2007). Thus, we speculate that one way in which IL-2 signaling impairs Tfh responses to influenza is by altering the Blimp1:Bcl6 ratio. This view is in agreement our results, and previous results demonstrating that Blimp1 and Bcl6 are reciprocally expressed in CD25hi and CD25lo CD4+ T cells responding to LCMV (Choi et al., 2011). Therefore, we suggest that prolonged IL-2 signaling leads to increased Blimp1 expression and represses Bcl6 expression and thus impairs the differentiation or maintenance of Tfh cells. Interestingly, our data show that although excess IL-2 suppresses Tfh cells, there is not a corresponding increase in the number of effector CD4+ T cells. These results suggest that IL-2 signaling compromises the maintenance or survival of T cells committed to the Tfh pathway, rather than diverting influenza-specific CD4+ T cells from the Tfh pathway into the Th1 effector pathway.
Published results show that IL-2 controls the differentiation of T cells by regulating the expression of a variety of transcription factors (Pipkin et al., 2010). For example, IL-2 represses RORγt in CD4 T cells and prevents their differentiation into Th17 cells (Chen et al., 2011; Laurence et al., 2007; Pandiyan et al., 2011). IL-2 and STAT5 are also negative regulators of BCL6 in CD8+ T cells and consequently, IL-2 signaling promotes the expansion of effector CD8+ T cells at the expense of memory CD8+ T cells (Kalia et al., 2010; Pipkin et al., 2010). Similarly, STAT5 inhibits the differentiation of Tfh cells via the BLIMP-1-dependent repression of BCL6 (Johnston et al., 2012). Consistent with this idea, we suggest that IL-2 signaling impairs or prevents Bcl6 expression in primed CD4+ T cells, possibly via a STAT5-dependent mechanism (Johnston et al., 2012), and prevents their subsequent differentiation into Tfh cells. In the absence of Bcl6, Tfh precursors do not express CXCR5, do not home to the T cell area and do not receive the necessary signals from B cells to be maintained as Tfh cells (Choi et al., 2011; Deenick et al., 2011; Good-Jacobson et al., 2010). In contrast, once CD4+ T cells have differentiated into Tfh cells, they lose CD25 expression and the addition of IL-2 does not affect Bcl6 or CXCR5 expression. Thus, we believe that IL-2 acts early in the Tfh differentiation pathway.
One of the hallmarks of Tfh cells is the expression of high levels of PD-1 (Yu and Vinuesa, 2010). PD-1 is an inhibitory receptor that is often associated with T cell exhaustion (Sharpe et al., 2007). However, PD-1 is also required for Tfh cell function and for germinal center formation (Good-Jacobson et al., 2010). However, it is not clear how PD-1 contributes to Tfh differentiation or maintenance. Interestingly, PD-1 signaling inhibits IL-2 production (Chikuma et al., 2009). Based on our observations showing that IL-2 signaling is deleterious to Tfh responses, we speculate that PD-1 signaling may promote the maintenance of Tfh cells by blocking autocrine IL-2 production.
Collectively, our results demonstrate that IL-2 plays a central role in the control of the Tfh cell response to influenza. Our findings also offer a new perspective for how Tfh cell development is regulated, and demonstrate that the physiological availability of IL-2 is a critical factor that regulates successful B cell responses in vivo.
EXPERIMENTAL PROCEDURES
Mice, infections and in vivo treatments
C57BL/6 (B6), B6.129S4-Il2ratm1Dw/J(Cd25−/−) and B6.IgHa.Thy-1a.Ptrpca (CD45.1) mice were originally obtained from Jackson Laboratories and were bred in the University of Rochester animal facility. B6.129S6-Foxp3tm1DTR (FoxP3-DTR) mice were obtained from Dr. Alexander Rudensky (Memorial Sloan-Kettering Cancer Center). Influenza virus infections were performed intranasally with 500 egg infectious units of A/PR8/34 (PR8) in 100 µl of PBS. Heligmosomoides polygyrus (Hp) infections were performed by gavage with 200 L3 Hp larvae as described (Wojciechowski et al., 2009). BM chimeric mice were generated by lethally irradiating CD45.1 mice with 950 Rads from a 137Cs source delivered in 2 equal doses 4–5 hours apart. After irradiation, mice were intravenously injected with 5 × 106 total BM cells and allowed to reconstitute for 8–12 weeks before influenza infection. In indicated experiments, WT and FoxP3-DTR received an intraperitoneal injection of 50 µg/kg of DT (Sigma) on days 0, 4 and 7 after infection. For IL-2 treatment, mice were intraperitoneally administered 30,000 U of recombinant human IL-2 (National Cancer Institute), twice a day starting 3 days after infection. Control mice received injections of PBS. All experimental procedures involving animals were approved by the University of Rochester University Committee on Animal Resources and were performed according to guidelines outlined by the National Research Council.
Cell preparation and flow cytometry
Cell suspensions from mLNs were centrifuged, resuspended in 150 mM NH4Cl, 10 mM KHCO3 0.1 mM EDTA for 10 min to lyse red cells and filtered through a 70 µm nylon cell strainer (BD Biosciences). Cells were washed and resuspended in PBS with 5% donor calf serum and 10 µg/ml FcBlock (2.4G2 - Trudeau Institute) for 10 min on ice followed by staining with fluorochrome-conjugated antibodies or tetramer reagents. The IAbNP311–325 MHC class II tetramer was obtained from the NIH Tetramer Core Facility. Fluorochrome-labeled anti-CD45.1 (A20), anti-CD45.2 (104), anti-PD-1 (J43), anti-FoxP3 (FJK-16s), anti-CD19 (1D3), anti-B220 (RA3-6B2), anti-CD138 (281.2), were from eBioscience. Anti-Bcl6 (K112.91), anti-CXCR5 (2G-8), anti-ICOS (7E.17G9), anti-CD4 (RM4-5), anti-CD95 (RM4-5), were from BD Biosciences. Peanut agglutinin (PNA) was obtained from Sigma (L7381; Sigma) and conjugated with FITC. Intracellular staining was performed using the mouse regulatory T cell staining kit (eBioscience) following manufacturer’s instructions. Flow cytometry was performed using a FACSCanto II (BD Biosciences), or a C6 Flow Cytometer (Accuri), available through the Flow Cytometry Core Facility at the University of Rochester.
Immunofluorescence
Lymph nodes were frozen in OCT (Tissue-Tek; Sakura) and 7 µm frozen sections were prepared and stained as described (Rangel-Moreno et al., 2011). Slides were probed for 30 min at 25 °C with anti-B220 (RA3-6B2), anti-IgDb (2170-170) obtained from BD Biosciences, anti-CD21 (7E9, BioLegend) and PNA (L7381; Sigma). Biotin-conjugated primary antibodies were detected with streptavidin–Alexa Fluor 555 (S21381; Invitrogen Life Sciences). Images were obtained using a Zeiss Axioplan 2 microscope with a Zeiss Axiocam digital camera (Zeiss) and saved as TIFF files.
ELISAS and ELISPOT
For ELISAS, 96-well plates were coated with purified viral proteins (Lee et al., 2005) at 1 µg/ml in 0.05 M Na2CO3 pH 9.6 overnight and then blocked for 1 hour with 1% BSA in PBS. Serum samples were serially diluted (3-fold) in PBS with 10 mg/ml BSA and 0.1% Tween 20 before incubation on coated plates. After washing, bound antibody was detected with HRP-conjugated goat anti-mouse IgG1 (Southern Biotechnology Associates). For ELISPOT, Multiscreen cellulose filter plates (Millipore) were coated overnight with 1 µg/ml purified viral proteins. Cells were collected from bone marrow and plated in triplicate starting at 1 × 106/well and in 3-fold serial dilutions in complete RPMI 1640 containing 10% FBS. After 5 hours, the wells were washed with PBS containing 0.5% BSA and 0.05% Tween 20, and IgG was detected using alkaline phosphatase-conjugated goat anti-mouse IgG (Southern Biotechnology Associates). Spots were automatically counted in a CTL-immunoSpot S5 analyzer.
NP-tetramer preparation for detection of influenza-specific B cells
The coding sequence of NP from the A/PR8/34 virus was synthesized in frame with the coding sequence for a 15 amino acid biotinylation consensus site (Beckett et al., 1999) on the 3’ end (GeneArt, Regensburg, Germany). The modified NP sequence was cloned in frame to the 6X His tag in the pTRC-His2c expression vector (Invitrogen, Carlsbad, CA). NP protein was expressed in E. coli strain Ultra BL21 (DE3) (EdgeBio, Gaithersburg, MD) and purified by FPLC using a HisTrap HP Column (GE Healthcare). Purified protein was biotinylated by addition of Biotin-protein ligase (Avidity, Aurora, CO). The biotinylated NP protein was then tetramerized with PE-labeled streptavidin (Prozyme, Hayward, CA). Labeled tetramers were purified by size exclusion on a HiPrep 16/60 Sephacryl S-300 column (GE Healthcare, Piscataway, NJ).
Statistical analysis
The statistical significance of differences in mean values was analyzed using a two-tailed Student's t-test. P values of less than 0.05 were considered statistically significant.
ACKNOWLEDGEMENTS
The authors would like to thank L. LaMere and A. Boucher for animal husbandry. This work was supported by the University of Rochester and by NIH grants HL069409, AI072689 and AI061511 to T.D.R. and NIH grants AI078907 and AI068056 to F.E.L.
Footnotes
Publisher's Disclaimer: This is a PDF file of an unedited manuscript that has been accepted for publication. As a service to our customers we are providing this early version of the manuscript. The manuscript will undergo copyediting, typesetting, and review of the resulting proof before it is published in its final citable form. Please note that during the production process errors may be discovered which could affect the content, and all legal disclaimers that apply to the journal pertain.
REFERENCES
- Alexander CM, Tygrett LT, Boyden AW, Wolniak KL, Legge KL, Waldschmidt TJ. T regulatory cells participate in the control of germinal centre reactions. Immunology. 2011;133:452–468. [Abstract] [Google Scholar]
- Allen CD, Okada T, Cyster JG. Germinal-center organization and cellular dynamics. Immunity. 2007;27:190–202. [Europe PMC free article] [Abstract] [Google Scholar]
- Almeida AR, Legrand N, Papiernik M, Freitas AA. Homeostasis of peripheral CD4+ T cells: IL-2R alpha and IL-2 shape a population of regulatory cells that controls CD4+ T cell numbers. J Immunol. 2002;169:4850–4860. [Abstract] [Google Scholar]
- Bachmann MF, Schorle H, Kuhn R, Muller W, Hengartner H, Zinkernagel RM, Horak I. Antiviral immune responses in mice deficient for both interleukin-2 and interleukin-4. J Virol. 1995;69:4842–4846. [Europe PMC free article] [Abstract] [Google Scholar]
- Beckett D, Kovaleva E, Schatz PJ. A minimal peptide substrate in biotin holoenzyme synthetase-catalyzed biotinylation. Protein science : a publication of the Protein Society. 1999;8:921–929. [Europe PMC free article] [Abstract] [Google Scholar]
- Blattman JN, Grayson JM, Wherry EJ, Kaech SM, Smith KA, Ahmed R. Therapeutic use of IL-2 to enhance antiviral T-cell responses in vivo. Nat Med. 2003;9:540–547. [Abstract] [Google Scholar]
- Bryant VL, Ma CS, Avery DT, Li Y, Good KL, Corcoran LM, de Waal Malefyt R, Tangye SG. Cytokine-mediated regulation of human B cell differentiation into Ig-secreting cells: predominant role of IL-21 produced by CXCR5+ T follicular helper cells. J Immunol. 2007;179:8180–8190. [Abstract] [Google Scholar]
- Campbell DJ, Koch MA. Phenotypical and functional specialization of FOXP3+ regulatory T cells. Nat Rev Immunol. 2011;11:119–130. [Europe PMC free article] [Abstract] [Google Scholar]
- Chen Y, Haines CJ, Gutcher I, Hochweller K, Blumenschein WM, McClanahan T, Hammerling G, Li MO, Cua DJ, McGeachy MJ. Foxp3(+) regulatory T cells promote T helper 17 cell development in vivo through regulation of interleukin-2. Immunity. 2011;34:409–421. [Abstract] [Google Scholar]
- Chikuma S, Terawaki S, Hayashi T, Nabeshima R, Yoshida T, Shibayama S, Okazaki T, Honjo T. PD-1-mediated suppression of IL-2 production induces CD8+ T cell anergy in vivo. J Immunol. 2009;182:6682–6689. [Abstract] [Google Scholar]
- Choi YS, Kageyama R, Eto D, Escobar TC, Johnston RJ, Monticelli L, Lao C, Crotty S. ICOS Receptor Instructs T Follicular Helper Cell versus Effector Cell Differentiation via Induction of the Transcriptional Repressor Bcl6. Immunity. 2011;34:932–946. [Europe PMC free article] [Abstract] [Google Scholar]
- Chung Y, Tanaka S, Chu F, Nurieva RI, Martinez GJ, Rawal S, Wang YH, Lim H, Reynolds JM, Zhou XH, et al. Follicular regulatory T cells expressing Foxp3 and Bcl-6 suppress germinal center reactions. Nat Med. 2011;17:983–988. [Europe PMC free article] [Abstract] [Google Scholar]
- Cox RJ, Brokstad KA, Ogra P. Influenza virus: immunity and vaccination strategies. Comparison of the immune response to inactivated and live, attenuated influenza vaccines. Scandinavian journal of immunology. 2004;59:1–15. [Abstract] [Google Scholar]
- Crotty S. Follicular helper CD4 T cells (TFH) Annu Rev Immunol. 2011;29:621–663. [Abstract] [Google Scholar]
- Cyster JG. B cell follicles and antigen encounters of the third kind. Nat Immunol. 2010;11:989–996. [Abstract] [Google Scholar]
- de la Rosa M, Rutz S, Dorninger H, Scheffold A. Interleukin-2 is essential for CD4+CD25+ regulatory T cell function. Eur J Immunol. 2004;34:2480–2488. [Abstract] [Google Scholar]
- Deenick EK, Ma CS, Brink R, Tangye SG. Regulation of T follicular helper cell formation and function by antigen presenting cells. Curr Opin Immunol. 2011;23:111–118. [Abstract] [Google Scholar]
- Fazilleau N, McHeyzer-Williams LJ, Rosen H, McHeyzer-Williams MG. The function of follicular helper T cells is regulated by the strength of T cell antigen receptor binding. Nat Immunol. 2009;10:375–384. [Europe PMC free article] [Abstract] [Google Scholar]
- Friedberg JW, Neuberg D, Gribben JG, Fisher DC, Canning C, Koval M, Poor CM, Green LM, Daley J, Soiffer R, et al. Combination immunotherapy with rituximab and interleukin 2 in patients with relapsed or refractory follicular non-Hodgkin's lymphoma. Br J Haematol. 2002;117:828–834. [Abstract] [Google Scholar]
- Gillies SD, Lan Y, Williams S, Carr F, Forman S, Raubitschek A, Lo KM. An anti-CD20-IL-2 immunocytokine is highly efficacious in a SCID mouse model of established human B lymphoma. Blood. 2005;105:3972–3978. [Abstract] [Google Scholar]
- Gluck WL, Hurst D, Yuen A, Levine AM, Dayton MA, Gockerman JP, Lucas J, Denis-Mize K, Tong B, Navis D, et al. Phase I studies of interleukin (IL)-2 and rituximab in B-cell non-hodgkin's lymphoma: IL-2 mediated natural killer cell expansion correlations with clinical response. Clin Cancer Res. 2004;10:2253–2264. [Abstract] [Google Scholar]
- Gong D, Malek TR. Cytokine-dependent Blimp-1 expression in activated T cells inhibits IL-2 production. J Immunol. 2007;178:242–252. [Abstract] [Google Scholar]
- Good-Jacobson KL, Szumilas CG, Chen L, Sharpe AH, Tomayko MM, Shlomchik MJ. PD-1 regulates germinal center B cell survival and the formation and affinity of long-lived plasma cells. Nat Immunol. 2010;11:535–542. [Europe PMC free article] [Abstract] [Google Scholar]
- Goodnow CC, Vinuesa CG, Randall KL, Mackay F, Brink R. Control systems and decision making for antibody production. Nat Immunol. 2010;11:681–688. [Abstract] [Google Scholar]
- Grinberg-Bleyer Y, Baeyens A, You S, Elhage R, Fourcade G, Gregoire S, Cagnard N, Carpentier W, Tang Q, Bluestone J, et al. IL-2 reverses established type 1 diabetes in NOD mice by a local effect on pancreatic regulatory T cells. J Exp Med. 2010;207:1871–1878. [Europe PMC free article] [Abstract] [Google Scholar]
- Han S, Hathcock K, Zheng B, Kepler TB, Hodes R, Kelsoe G. Cellular interaction in germinal centers. Roles of CD40 ligand and B7-2 in established germinal centers. Journal of immunology. 1995;155:556–567. [Abstract] [Google Scholar]
- Hardtke S, Ohl L, Forster R. Balanced expression of CXCR5 and CCR7 on follicular T helper cells determines their transient positioning to lymph node follicles and is essential for efficient B-cell help. Blood. 2005;106:1924–1931. [Abstract] [Google Scholar]
- Haynes NM, Allen CD, Lesley R, Ansel KM, Killeen N, Cyster JG. Role of CXCR5 and CCR7 in follicular Th cell positioning and appearance of a programmed cell death gene-1high germinal center-associated subpopulation. J Immunol. 2007;179:5099–5108. [Abstract] [Google Scholar]
- Humrich JY, Morbach H, Undeutsch R, Enghard P, Rosenberger S, Weigert O, Kloke L, Heimann J, Gaber T, Brandenburg S, et al. Homeostatic imbalance of regulatory and effector T cells due to IL-2 deprivation amplifies murine lupus. Proc Natl Acad Sci U S A. 2010;107:204–209. [Europe PMC free article] [Abstract] [Google Scholar]
- Johnston RJ, Choi YS, Diamond JA, Yang JA, Crotty S. STAT5 is a potent negative regulator of TFH cell differentiation. J Exp Med. 2012;209:243–250. [Europe PMC free article] [Abstract] [Google Scholar]
- Johnston RJ, Poholek AC, DiToro D, Yusuf I, Eto D, Barnett B, Dent AL, Craft J, Crotty S. Bcl6 and Blimp-1 are reciprocal and antagonistic regulators of T follicular helper cell differentiation. Science. 2009;325:1006–1010. [Europe PMC free article] [Abstract] [Google Scholar]
- Kalia V, Sarkar S, Subramaniam S, Haining WN, Smith KA, Ahmed R. Prolonged interleukin-2Ralpha expression on virus-specific CD8+ T cells favors terminal-effector differentiation in vivo. Immunity. 2010;32:91–103. [Abstract] [Google Scholar]
- Kelsoe G. The germinal center: a crucible for lymphocyte selection. Semin Immunol. 1996;8:179–184. [Abstract] [Google Scholar]
- Kim JM, Rasmussen JP, Rudensky AY. Regulatory T cells prevent catastrophic autoimmunity throughout the lifespan of mice. Nat Immunol. 2007;8:191–197. [Abstract] [Google Scholar]
- King C. A fine romance: T follicular helper cells and B cells. Immunity. 2011;34:827–829. [Abstract] [Google Scholar]
- Kovacs JA, Vogel S, Metcalf JA, Baseler M, Stevens R, Adelsberger J, Lempicki R, Hengel RL, Sereti I, Lambert L, et al. Interleukin-2 induced immune effects in human immunodeficiency virus-infected patients receiving intermittent interleukin-2 immunotherapy. Eur J Immunol. 2001;31:1351–1360. [Abstract] [Google Scholar]
- Laurence A, Tato CM, Davidson TS, Kanno Y, Chen Z, Yao Z, Blank RB, Meylan F, Siegel R, Hennighausen L, et al. Interleukin-2 signaling via STAT5 constrains T helper 17 cell generation. Immunity. 2007;26:371–381. [Abstract] [Google Scholar]
- Lee BO, Rangel-Moreno J, Moyron-Quiroz JE, Hartson L, Makris M, Sprague F, Lund FE, Randall TD. CD4 T cell-independent antibody response promotes resolution of primary influenza infection and helps to prevent reinfection. Journal of immunology. 2005;175:5827–5838. [Abstract] [Google Scholar]
- Lenardo MJ. Interleukin-2 programs mouse alpha beta T lymphocytes for apoptosis. Nature. 1991;353:858–861. [Abstract] [Google Scholar]
- Lim HW, Hillsamer P, Banham AH, Kim CH. Cutting edge: direct suppression of B cells by CD4+ CD25+ regulatory T cells. J Immunol. 2005;175:4180–4183. [Abstract] [Google Scholar]
- Lim HW, Hillsamer P, Kim CH. Regulatory T cells can migrate to follicles upon T cell activation and suppress GC-Th cells and GC-Th cell-driven B cell responses. J Clin Invest. 2004;114:1640–1649. [Europe PMC free article] [Abstract] [Google Scholar]
- Linterman MA, Beaton L, Yu D, Ramiscal RR, Srivastava M, Hogan JJ, Verma NK, Smyth MJ, Rigby RJ, Vinuesa CG. IL-21 acts directly on B cells to regulate Bcl-6 expression and germinal center responses. J Exp Med. 2010;207:353–363. [Europe PMC free article] [Abstract] [Google Scholar]
- Linterman MA, Pierson W, Lee SK, Kallies A, Kawamoto S, Rayner TF, Srivastava M, Divekar DP, Beaton L, Hogan JJ, et al. Foxp3+ follicular regulatory T cells control the germinal center response. Nat Med. 2011;17:975–982. [Europe PMC free article] [Abstract] [Google Scholar]
- Lopes de Menezes DE, Denis-Mize K, Tang Y, Ye H, Kunich JC, Garrett EN, Peng J, Cousens LS, Gelb AB, Heise C, et al. Recombinant interleukin-2 significantly augments activity of rituximab in human tumor xenograft models of B-cell non-Hodgkin lymphoma. J Immunother. 2007;30:64–74. [Abstract] [Google Scholar]
- Malek TR. The biology of interleukin-2. Annu Rev Immunol. 2008;26:453–479. [Abstract] [Google Scholar]
- Malek TR, Bayer AL. Tolerance, not immunity, crucially depends on IL-2. Nat Rev Immunol. 2004;4:665–674. [Abstract] [Google Scholar]
- Malek TR, Castro I. Interleukin-2 receptor signaling: at the interface between tolerance and immunity. Immunity. 2010;33:153–165. [Europe PMC free article] [Abstract] [Google Scholar]
- Malek TR, Yu A, Vincek V, Scibelli P, Kong L. CD4 regulatory T cells prevent lethal autoimmunity in IL-2Rbeta-deficient mice. Implications for the nonredundant function of IL-2. Immunity. 2002;17:167–178. [Abstract] [Google Scholar]
- Nurieva RI, Chung Y, Martinez GJ, Yang XO, Tanaka S, Matskevitch TD, Wang YH, Dong C. Bcl6 mediates the development of T follicular helper cells. Science. 2009;325:1001–1005. [Europe PMC free article] [Abstract] [Google Scholar]
- Pandiyan P, Conti HR, Zheng L, Peterson AC, Mathern DR, Hernandez-Santos N, Edgerton M, Gaffen SL, Lenardo MJ. CD4(+)CD25(+)Foxp3(+) regulatory T cells promote Th17 cells in vitro and enhance host resistance in mouse Candida albicans Th17 cell infection model. Immunity. 2011;34:422–434. [Europe PMC free article] [Abstract] [Google Scholar]
- Pipkin ME, Sacks JA, Cruz-Guilloty F, Lichtenheld MG, Bevan MJ, Rao A. Interleukin-2 and inflammation induce distinct transcriptional programs that promote the differentiation of effector cytolytic T cells. Immunity. 2010;32:79–90. [Europe PMC free article] [Abstract] [Google Scholar]
- Poholek AC, Hansen K, Hernandez SG, Eto D, Chandele A, Weinstein JS, Dong X, Odegard JM, Kaech SM, Dent AL, et al. In vivo regulation of Bcl6 and T follicular helper cell development. J Immunol. 2010;185:313–326. [Europe PMC free article] [Abstract] [Google Scholar]
- Rangel-Moreno J, Carragher DM, de la Luz Garcia-Hernandez M, Hwang JY, Kusser K, Hartson L, Kolls JK, Khader SA, Randall TD. The development of inducible bronchus-associated lymphoid tissue depends on IL-17. Nature immunology. 2011;12:639–646. [Europe PMC free article] [Abstract] [Google Scholar]
- Rosenberg SA, Lotze MT, Muul LM, Leitman S, Chang AE, Ettinghausen SE, Matory YL, Skibber JM, Shiloni E, Vetto JT, et al. Observations on the systemic administration of autologous lymphokine-activated killer cells and recombinant interleukin-2 to patients with metastatic cancer. N Engl J Med. 1985a;313:1485–1492. [Abstract] [Google Scholar]
- Rosenberg SA, Mule JJ, Spiess PJ, Reichert CM, Schwarz SL. Regression of established pulmonary metastases and subcutaneous tumor mediated by the systemic administration of high-dose recombinant interleukin 2. J Exp Med. 1985b;161:1169–1188. [Europe PMC free article] [Abstract] [Google Scholar]
- Sadlack B, Lohler J, Schorle H, Klebb G, Haber H, Sickel E, Noelle RJ, Horak I. Generalized autoimmune disease in interleukin-2-deficient mice is triggered by an uncontrolled activation and proliferation of CD4+ T cells. Eur J Immunol. 1995;25:3053–3059. [Abstract] [Google Scholar]
- Sadlack B, Merz H, Schorle H, Schimpl A, Feller AC, Horak I. Ulcerative colitis-like disease in mice with a disrupted interleukin-2 gene. Cell. 1993;75:253–261. [Abstract] [Google Scholar]
- Sharpe AH, Wherry EJ, Ahmed R, Freeman GJ. The function of programmed cell death 1 and its ligands in regulating autoimmunity and infection. Nat Immunol. 2007;8:239–245. [Abstract] [Google Scholar]
- Suzuki H, Kundig TM, Furlonger C, Wakeham A, Timms E, Matsuyama T, Schmits R, Simard JJ, Ohashi PS, Griesser H, et al. Deregulated T cell activation and autoimmunity in mice lacking interleukin-2 receptor beta. Science. 1995;268:1472–1476. [Abstract] [Google Scholar]
- Vinuesa CG, Linterman MA, Goodnow CC, Randall KL. T cells and follicular dendritic cells in germinal center B-cell formation and selection. Immunol Rev. 2010;237:72–89. [Abstract] [Google Scholar]
- Vogelzang A, McGuire HM, Yu D, Sprent J, Mackay CR, King C. A fundamental role for interleukin-21 in the generation of T follicular helper cells. Immunity. 2008;29:127–137. [Abstract] [Google Scholar]
- Williams MA, Tyznik AJ, Bevan MJ. Interleukin-2 signals during priming are required for secondary expansion of CD8+ memory T cells. Nature. 2006;441:890–893. [Europe PMC free article] [Abstract] [Google Scholar]
- Wojciechowski W, Harris DP, Sprague F, Mousseau B, Makris M, Kusser K, Honjo T, Mohrs K, Mohrs M, Randall T, Lund FE. Cytokine-producing effector B cells regulate type 2 immunity to H. polygyrus. Immunity. 2009;30:421–433. [Europe PMC free article] [Abstract] [Google Scholar]
- Yu D, Rao S, Tsai LM, Lee SK, He Y, Sutcliffe EL, Srivastava M, Linterman M, Zheng L, Simpson N, et al. The transcriptional repressor Bcl-6 directs T follicular helper cell lineage commitment. Immunity. 2009;31:457–468. [Abstract] [Google Scholar]
- Yu D, Vinuesa CG. The elusive identity of T follicular helper cells. Trends Immunol. 2010;31:377–383. [Abstract] [Google Scholar]
Full text links
Read article at publisher's site: https://doi.org/10.1016/j.immuni.2012.02.012
Read article for free, from open access legal sources, via Unpaywall:
http://www.cell.com/article/S1074761312001215/pdf
Citations & impact
Impact metrics
Citations of article over time
Alternative metrics
Article citations
Spatial microniches of IL-2 combine with IL-10 to drive lung migratory T<sub>H</sub>2 cells in response to inhaled allergen.
Nat Immunol, 25(11):2124-2139, 11 Oct 2024
Cited by: 0 articles | PMID: 39394532
Precursor central memory versus effector cell fate and naïve CD4+ T cell heterogeneity.
J Exp Med, 221(10):e20231193, 25 Sep 2024
Cited by: 0 articles | PMID: 39321257 | PMCID: PMC11448869
Regulation of the Function of T Follicular Helper Cells and B Cells in Type 1 Diabetes Mellitus by the OX40/OX40L Axis.
J Clin Endocrinol Metab, 109(11):2823-2830, 01 Oct 2024
Cited by: 0 articles | PMID: 38625053 | PMCID: PMC11479688
Role of cellular effectors in the induction and maintenance of IgA responses leading to protective immunity against enteric bacterial pathogens.
Front Immunol, 15:1446072, 11 Sep 2024
Cited by: 0 articles | PMID: 39324143 | PMCID: PMC11422102
Review Free full text in Europe PMC
Psoriasis and Lupus Erythematosus-Similarities and Differences between Two Autoimmune Diseases.
J Clin Med, 13(15):4361, 25 Jul 2024
Cited by: 0 articles | PMID: 39124628 | PMCID: PMC11312967
Review Free full text in Europe PMC
Go to all (342) article citations
Data
Data behind the article
This data has been text mined from the article, or deposited into data resources.
BioStudies: supplemental material and supporting data
Similar Articles
To arrive at the top five similar articles we use a word-weighted algorithm to compare words from the Title and Abstract of each citation.
Context-Dependent Role for T-bet in T Follicular Helper Differentiation and Germinal Center Function following Viral Infection.
Cell Rep, 28(7):1758-1772.e4, 01 Aug 2019
Cited by: 26 articles | PMID: 31412245 | PMCID: PMC6711398
Crucial roles of interleukin-7 in the development of T follicular helper cells and in the induction of humoral immunity.
J Virol, 88(16):8998-9009, 04 Jun 2014
Cited by: 53 articles | PMID: 24899182 | PMCID: PMC4136280
Interleukin-21 promotes germinal center reaction by skewing the follicular regulatory T cell to follicular helper T cell balance in autoimmune BXD2 mice.
Arthritis Rheumatol, 66(9):2601-2612, 01 Sep 2014
Cited by: 71 articles | PMID: 24909430 | PMCID: PMC4146687
Role of TRAFs in Signaling Pathways Controlling T Follicular Helper Cell Differentiation and T Cell-Dependent Antibody Responses.
Front Immunol, 9:2412, 22 Oct 2018
Cited by: 12 articles | PMID: 30405612 | PMCID: PMC6204373
Review Free full text in Europe PMC
Funding
Funders who supported this work.
NHLBI NIH HHS (3)
Grant ID: R01 HL069409
Grant ID: R01 HL069409-10
Grant ID: HL069409
NIAID NIH HHS (10)
Grant ID: AI072689
Grant ID: AI061511
Grant ID: R01 AI061511-05
Grant ID: R01 AI072689
Grant ID: AI068056
Grant ID: AI078907
Grant ID: R01 AI072689-05
Grant ID: P01 AI078907
Grant ID: R01 AI061511
Grant ID: R01 AI068056
National Institutes of Health (5)
Grant ID: HL069409
Grant ID: AI078907
Grant ID: AI068056
Grant ID: AI061511
Grant ID: AI072689