Abstract
Free full text

The Syrian hamster model of hantavirus pulmonary syndrome
Abstract
Hantavirus pulmonary syndrome (HPS) is a relatively rare, but frequently fatal disease associated with New World hantaviruses, most commonly Sin Nombre and Andes viruses in North and South America, respectively. It is characterized by fever and the sudden, rapid onset of severe respiratory distress and cardiogenic shock, which can be fatal in up to 50% of cases. Currently there are no approved antiviral therapies or vaccines for the treatment or prevention of HPS. A major obstacle in the development of effective medical countermeasures against highly pathogenic agents like the hantaviruses is recapitulating the human disease as closely as possible in an appropriate and reliable animal model. To date, the only animal model that resembles HPS in humans is the Syrian hamster model. Following infection with Andes virus, hamsters develop HPS-like disease which faithfully mimics the human condition with respect to incubation period and pathophysiology of disease. Perhaps most importantly, the sudden and rapid onset of severe respiratory distress observed in humans also occurs in hamsters. The last several years has seen an increase in studies utilizing the Andes virus hamster model which have provided unique insight into HPS pathogenesis as well as potential therapeutic and vaccine strategies to treat and prevent HPS. The purpose of this article is to review the current understanding of HPS disease progression in Syrian hamsters and discuss the suitability of utilizing this model to evaluate potential medical countermeasures against HPS.
1. Introduction
Hantaviruses (family Bunyaviridae, genus Hantavirus) are a large group of viruses which are associated with two clinical syndromes in humans: hemorrhagic fever with renal syndrome (HFRS), and hantavirus pulmonary syndrome (HPS). Both HFRS and HPS are associated with vascular leakage with HFRS mainly affecting the kidneys and HPS preferentially targeting the lungs. Case fatality rates for HFRS can reach as high as 15% and for HPS can exceed 40% (Jonsson et al., 2010). Currently, the ICTV recognizes 23 unique species of hantaviruses, at least half of which are medically important to humans (ICTV, 2009). In nature, pathogenic hantaviruses are primarily maintained in specific rodent hosts which dictate the geographic regions of endemicity for individual viruses. HFRS occurs throughout Europe and Asia and is caused by Old World hantaviruses including Hantaan and Puumala viruses (HTNV and PUUV), while HPS occurs in the Americas and is associated with infection of New World hantaviruses, primarily Sin Nombre and Andes viruses (SNV and ANDV). Humans are predominantly exposed to hantaviruses via inhalation or ingestion of contaminated rodent excreta or secreta, although direct transmission through rodent-bites and human-to-human transmission have also occasionally been documented (Martinez et al., 2005; Padula et al., 1998; St Jeor, 2004; Torres-Perez et al., 2010).
Based on the high mortality rates and the capacity to spread via aerosolization, hantaviruses are considered Category A priority pathogens by the National Institute of Allergy and Infectious Diseases. At present there are no FDA-approved medical countermeasures to treat or prevent HPS or HFRS, therefore the development of safe and effective vaccines and antivirals to reduce the threat of hantaviruses globally is imperative. A major impedance to developing appropriate interventions has been a lack of practical animal models of disease. To date, researchers have had to rely on testing therapeutics in suckling mice or natural rodent hosts, neither of which are models for HFRS or HPS. In 2001 a lethal model for HPS was described in Syrian hamsters (Hooper et al., 2001). The purpose of this article is to review the current literature on the ANDV hamster model and discuss its appropriateness for use in evaluating medical countermeasures for HPS.
2. Animal models of HFRS and HPS
The development of animal models of HFRS and HPS has been an intense area of research since their respective discoveries (Tables 1 and and2).2). Currently no animal model exists which reflects the disease manifestations of severe HFRS. Non-human primates often provide good models for studying hemorrhagic fever viruses, and therefore have been assessed as potential models for HFRS, though with limited success (Gowen and Holbrook, 2008; Yanagihara et al., 1988). The lone exception is Cynomolgus macaques which develop a mild disease (i.e. lethargy, proteinuria, and microhaematuria) suggestive of acute nephropathy following inoculation with wild-type PUUV (Klingstrom et al., 2002; Sironen et al., 2008). Several small laboratory animals have also been experimentally infected with numerous Old World hantavirus species resulting in the characterization of traditional infection and/or acute disease models (summarized in Table 1). Perhaps most successful of these are infection of newborn (suckling), juvenile and adult mice with HTNV which, depending on the virus strain and route of infection utilized results in lethal disease, albeit with primarily neurological or pulmonary manifestations (Ebihara et al., 2000; Kurata et al., 1983; McKee et al., 1985; Seto et al., 2012; Tamura et al., 1989; Wichmann et al., 2002). Although the disease characteristics of the HTNV mouse models differ from HFRS, the lethal outcome of infection has supported their use in the evaluation of antivirals for HFRS (Huggins et al., 1986; Murphy et al., 2001).
Table 1
Summary of animal models for Old World hantaviruses
Virus | Brief description | |
---|---|---|
Natural hosts | ||
Striped field mouse | Hantaan | Subclinical, persistent infection with transient viremia between days 7 and 12 p.i. and infectious virus and/or antigen detected in organs between days 10 and 360 p.i. Horizontal transmission, primarily associated with infectious virus in urine, was observed for 360 days p.i. a |
Bank Vole | Puumala | Subclinical, persistent infection with transient viremia between days 10 and 14 p.i. and infectious virus/antigen detected in organs between days 14 and 270 p.i. Horizontal transmission observed between days 14 and 42 p.i. b |
Norway (brown) rat | Seoul | Subclinical, most likely persistent, infection with viral RNA detected in organs for 40 days p.i. c,d |
| ||
Laboratory animals | ||
Juvenile rat | Hantaan | Subclinical infection with transient viremia between days 10 and 13 p.i. and persistent detection of virus/antigen in organs. Horizontal transmission was observed for up to 63 days p.i. e |
Suckling mouse | Hantaan | Acute, disseminated infection with neurological signs of disease and lethality rates between 88 and 100% at 13–14 days p.i. f,g,h |
Suckling mouse | Seoul | Disseminated infection with 100% lethality between days 18–24 p.i.i |
Suckling mouse | Dobrava | Disseminated infection associated with increased levels of nitric oxide that is fatal in up to 88% of mice between days 18–26 p.i. j |
Juvenile mouse | Hantaan | Acute, disseminated infection with primarily pulmonary disease manifestations which are fatal in approximately 25% of mice k |
Adult mouse | Hantaan | Acute, disseminated infection resulting in lethal encephalitis in 100% of infected mice l |
Mongolian Gerbil | Seoul | Subclinical, disseminated infection of unknown duration m |
Hantaan | Subclinical, disseminated infection of unknown duration m | |
Syrian hamster | Puumala | Subclinical, disseminated infection with viral antigen detected for at least 56 days p.i. n,o,p,q |
Syrian hamster | Hantaan | Subclinical, disseminated infection with viral antigen/RNA detected for > 30 days p.i. o,p,q |
Syrian hamster | Seoul | Subclinical, disseminated infection with viral antigen/RNA detected for > 30 days p.i. o,q,r |
Syrian hamster | Dobrava | Subclinical, disseminated infection with viral antigen/RNA detected for > 30 days p.i. o |
Cynomolgus macaque | Puumala | Self-limiting infection resulting in low grade fever, serum biochemical changes, mild proteinuria and microhematuria as well as transient viremia and renal inflammatory infiltrates similar to a mild form of HFRS known as Nephropathia epidemica s,t |
Table 2
Summary of animal models for New World hantaviruses
Virus | Brief description | |
---|---|---|
Natural hosts | ||
Deer mouse | Sin Nombre | Persistent, disseminated infection with transient viremia and viral antigen detected in organs for at least 90 days p.i. a,b |
Cotton rat | Black Creek Canal | Persistent, disseminated infection with infectious viruses detected in organs for at least 150 days p.i. Animals did not demonstrated any clinical signs of infection, though subacute pneumonitis was noted in the lungs of some animals, the severity of which was dependent on inoculum dose c,d |
Alston’s cotton rat | Cano Delgadito | Persistent, disseminated infection with transient viremia and isolation of infectious virus from solid organs for at least 56 days p.i. Virus was also detected in excreta/secreta suggesting the capability for transmission e |
| ||
Laboratory animals | ||
Syrian hamster | Andes | Systemic infection resulting in lethal HPS like disease in 100% of animals f,g,h,i |
Syrian hamster | Maporal | Systemic infection leading to lethal HPS like disease in approximately 30% of animals j |
Syrian hamster | Sin Nombre | Self-limited infection with little dissemination and no detectable viremia f,h |
Syrian hamster | Choclo | Systemic infection with notable viral replication in the lung endothelium, though animals do not demonstrate any signs of illness k |
Cynomolgus macaque | Andes | Self-limiting infection with no signs of illness l |
Cynomolgus macaque | Prospect Hill | Self-limiting infection with mild proteinuria as the only clinical sign of illness and viremia detected between days 5 and 7 p.i. m |
Chimpanzee | Prospect Hill | Self-limiting infection with mild proteinuria as the only clinical sign of illness and viremia detected between days 5 and 7 p.i. m |
Over the last several decades, Syrian hamsters (Mesocricetus auratus) have become commonly used laboratory animal for the study of parasites, bacteria, prions and viruses, including hantaviruses (Zivcec et al., 2011). Several studies have demonstrated the Syrian hamster as a useful infection model for numerous Old World hantaviruses including HTNV and PUUV as well as Dobrava and Seoul viruses (Kamrud et al., 1999; Sanada et al., 2011; Schmaljohn et al., 1990). Thus, it has been possible to perform vaccine studies aimed at evaluating cross-protective immune responses where the read-out is protection from infection (see Table 1).
Efforts aimed at developing animal models for HPS are limited compared to those for HFRS, due in part to the higher mortality rates of HPS in humans which generally restrict in vivo experiments to maximum containment (i.e., biosafety level 4) facilities (Table 2). Cynomolgus monkeys have been evaluated as a potential NHP model and although they are susceptible to infection with ANDV, clinical signs of illness were not observed (McElroy et al., 2002). In 2001, a lethal disease model was described whereby Syrian hamsters infected with ANDV develop pulmonary deficiencies similar to HPS in humans (Hooper et al., 2001). Interestingly, hamsters are not equally susceptible to lethal disease following infection with other etiological agents of HPS. To date, only Maporal virus (MAPV), a hantavirus which is believed to be non-pathogenic to humans, causes HPS-like disease in hamsters, though with a lower mortality rate than ANDV (Milazzo et al., 2002). Other New World hantaviruses, including SNV and Choclo virus can readily infect hamsters, but similar to HFRS-causing viruses, show no signs of illness (Eyzaguirre et al., 2008; Hooper et al., 2001; Wahl-Jensen et al., 2007).
It should also be noted that for both Old and New World hantaviruses, considerable efforts have been made at modeling the natural infection in rodent hosts in attempt to glean information on persistence, transmission and maintenance in nature (Botten et al., 2000; Easterbrook et al., 2007; Fulhorst et al., 2002; Hutchinson et al., 1998; Lee et al., 1981; Schountz et al., 2007; Yanagihara et al., 1985). Although these models have also been employed to evaluate potential therapies and vaccines (Lundkvist et al., 1996; Medina et al., 2007), they are not ideal for these purposes since the infection dynamics and immunological responses in the natural hosts, which are generally persistently infected, are significantly different from acute infections in humans.
3. HPS in humans and hamsters
HPS is a febrile illness characterized by diffuse bilateral interstitial pulmonary infiltrates and compromised respiratory function which often requires supplemental oxygen (CDC, 2010). On average, the incubation period from exposure to symptom onset is less than 21 days with ranges of 11–32 days for ANDV and 9–33 days for SNV (Vial et al., 2006; Young et al., 2000). Clinically, HPS in humans is characterized by four phases (febrile/prodrome, cardiopulmonary, diuretic and convalescent). Disease typically commences with a prodrome characterized by nondescript symptoms, including fever. The cardiopulmonary phase is distinguished by sudden onset of severe respiratory symptoms. Typically patients rapidly progress from coughing and shortness of breath to severe respiratory distress, hypoxemia, shock and cardiac failure. The majority of HPS-related fatalities occur during this phase, often within 48 hours. Pathophysiologically, this phase is characterized by vascular leakage leading to interstitial pneumonitis with congestion, edema, mononuclear cell infiltration, fibrin deposition and focal hyaline membranes in the respiratory tissue (Zaki et al., 1995). Resolution of pulmonary symptoms in nonfatal cases is rapid and patients who progress to the third and fourth (diuretic and convalescent) stages of disease have a good prognosis.
Following infection with ANDV, Syrian hamsters develop HPS-like disease which closely resembles the human condition with respect to incubation period, rapidity of disease progression and disease manifestations (Table 3). The strength of the model is the ability to recapitulate the cardiopulmonary phase of disease, which is the hallmark of HPS and similar to humans presents as a rapid progression from shortness of breath to severe dyspnea. Pathophysiologically, this phase is characterized by vascular leakage leading to pulmonary edema and pleural effusion. Recently, digital radiography was utilized to monitor disease progression in individual hamsters and demonstrated the presence of moderate to severe pleural effusion and diffuse pulmonary infiltrates that were consistent with pulmonary leak and fulminant HPS in humans (Safronetz et al., 2011b) (Figure 1). Consistent with the clinical presentation and radiographic findings, histological changes are most pronounced in the lungs of hamsters with varying degrees of interstitial pneumonitis and perivascular pulmonary inflammation, increased numbers of macrophages and neutrophils, expanding interstitial spaces, alveolar and perivascular edema, hemorrhage and fibrin deposition frequently observed. Death typically occurs rapidly (within 1–2 days) following onset of pulmonary manifestations in hamsters and in addition to respiratory failure is associated with a precipitous fall in blood pressure and rapid increase in heart rate indicative of cardiogenic shock (Campen et al., 2006).
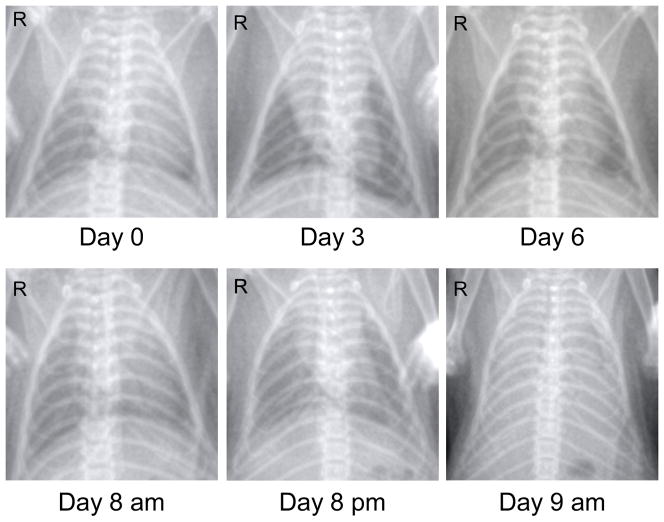
Digital radiography (x-ray) is an important, non-invasive technique which can be utilized to monitor progression of lung infiltrates in Syrian hamsters. Shown are the ventral-dorsal chest radiographs from a single hamster infected with Andes virus (200 focus-forming units, intraperitoneal route). Note the rapidity of lung infiltration which coincides with the onset of severe breathing insufficiences in hamsters (24–36 hours prior to death). The final x-ray taken at the time of euthanasia demonstrates diffuse pulmonary infiltrates with moderate to severe pleural effusion.
Table 3
Comparison of hantavirus pulmonary syndrome in humans and hamsters
Humans | Hamsters | |
---|---|---|
Prodrome: | ||
Incubation period | 11–32 days a | 7–29 days b, c,* |
Time from symptom onset to severe/lethal disease | 2–4 days d, e | 1–2 days b, c |
| ||
Clinical presentation: | ||
Dyspnea | Mild to severe d, e | Severe b,c |
Hypotension/Shock | Yes d | Yes f |
Radiographic changes | Limited to fulminant, diffuse alveolar edema g | Fulminant, diffuse pulmonary infiltrates c |
Pleural effusion | Bilateral h | Bilateral; moderate to severe c, i |
Epistasis | Occasional e | Occasional c |
| ||
Hematology: | ||
Neutrophilic leukocytosis | Yes h | Yes c, i |
Thrombocytopenia | Moderate to severe h | Mild to moderate i |
Coagulopathy | Moderate d, e | Moderate c |
| ||
Pathology/Histopathology: | ||
Lungs | Interstitial pneumonitis, congestion, edema, mononuclear cell infiltration, fibrin deposition, focal hyaline membranes h | Interstitial pneumonitis, edema, inflammation, mononuclear cell infiltration, hemorrhage, fibrin deposition c, i |
Heart | None reported h | None observed c, i |
Spleen | Mononuclear infiltration h | Mononuclear infiltration i |
Liver | None reported h | Mild to moderate hepatitis, hepatocellular necrosis i |
Kidney | None reported h | None observed c, i |
| ||
Antigen distribution: | ||
Lung | Intense and extensive staining of endothelial cells, especially the pulmonary microvasculature h | Intense and extensive staining of the pulmonary endothelium and alveolar septal lining cells b,c, i |
Peripheral organs | Endothelial cells of capillaries and small vessels; dendritic cells, macrophages and lymphocytes h | Endothelium, macrophages, hepatocytes c, i |
Pathogenic hantaviruses establish a systemic infection in both humans and hamsters with viral antigen readily detectable in the endothelium of most tissues analyzed. Despite widespread replication, pathological abnormalities are generally limited to the lungs (as noted above) and spleen. In contrast to humans, the liver might be involved in ANDV disease progression in hamsters. High levels of viral replication (similar to that observed in lungs), leading to mild-to-moderate subacute hepatitis and hepatocellular necrosis has been noted in hamsters following intramuscular or intraperitoneal challenge (Hooper et al., 2001; Wahl-Jensen et al., 2007) (Safronetz unpublished observations). The involvement of the liver as a primary target organ in hamsters remains to be explained since HPS in humans is rarely reported to have liver involvement, although it should be noted that the detection of hantavirus antigen in hepatic cells appears to be more prominent in South American cases of HPS (Enria et al., 2001; Toro et al., 1998; Zaki et al., 1995). Despite the high level of infectious virus in the livers of ANDV-infected hamsters, the antigen positive endothelial cells and hepatocytes appeared undamaged and the blood chemistries indicated normal liver function (Wahl-Jensen et al., 2007).
Of particular importance, especially for therapeutic studies, the hamster model of HPS is extremely robust with minimal variation in disease progression and lethal outcome associated with infection of Syrian hamsters of different sex or age, or from different suppliers (Campen et al., 2006; Hammerbeck and Hooper, 2011; Hooper et al., 2008; Hooper et al., 2001; Safronetz et al., 2011a; Safronetz et al., 2009). Furthermore, the model is highly reproducible with lethal disease observed even at low infectious doses via multiple routes of exposure including intramuscular (50% lethal dose (LD50) = 8 plaque forming units (pfu)), intraperitoneal (LD50 = 1.5 focus forming units), intragastric (LD50 = 225 pfu), intranasal (LD50 =95 pfu) and subcutaneous injections (LD50 > 2,000 pfu) (Hooper et al., 2008; Hooper et al., 2001; Safronetz et al., 2009). Although there are currently no reports of aerosol challenge (the primary route of human exposure) it should be expected that hamsters will develop disease following this route as well.
To date, two isolates of ANDV have been shown to cause HPS disease in hamsters without prior host adaptation (Hooper et al., 2001; Martinez and Padula, 2012). Besides ANDV, only MAPV has been shown to cause disease in hamsters (Milazzo et al., 2002). Although the MAPV model has been less studied, the disease progression and pathophysiology appears to be similar to that described for ANDV infected hamsters making it an attractive alternative which can be utilized in BSL-3 facilities, rather than in BSL-4 facilities where ANDV animal studies are performed. One disadvantage of the MAPV model is the reduced lethality rate (approximately 30%), which would necessitate the use of increased numbers of animals in studies aimed at evaluating medical countermeasures against HPS, although this could potentially be negated by serially passaging the virus in hamsters. Another disadvantage is the Animal Rule requirement that the agent be a wild-type (not serial passaged to alter virulence) of an etiologic agent of human disease.
Despite the success of the Syrian hamster model, studies aimed at evaluating other species of hamsters as potential models for other hantaviruses have not been reported. There are three distinct phylogenetic lineages (Phodopus, Mesocricetus, Cricetus-related) representing at least six genera within the subfamily Cricetinae (Neumann et al., 2006). Although studies should be conducted to assess different species of hamsters as potential disease models for HPS or HFRS, based on the genetic similarities to Syrian hamsters it would be of particular interest to assess the susceptibility of M. radei, M. brandt, and M. newtoni (Ciscaucasian, Turkish and Romanian hamsters, respectively) to various HPS-causing agents, especially those which have been shown to infect Syrian hamsters without causing signs of disease.
4. HPS pathogenesis in hamsters
The pathogenesis of HPS in humans is a complex process which largely remains to be elucidated. Therefore, it is difficult to assess how closely HPS in hamsters recapitulates the pathogenic processes believed to be occurring in humans. To date, much of our understanding of HPS pathogenesis comes from limited autopsies performed on fatal cases as well as comparative studies aimed at correlating clinical observations and host responses with disease severity and outcome. While imperative, these studies are hampered by the quantity and timing of sample collection. Nevertheless, several studies have predicted HPS is an immunopathogenic disease associated with intense immune activation resulting in increased vascular permeability (Borges et al., 2006; Jonsson et al., 2010; Macneil et al., 2011). Although at present the mechanism(s) responsible is unknown, the prevailing hypothesis on vascular leakage associated with HPS is that it is due to T-cell mediated immunopathogenesis. This theory is supported by clinical studies which have found a positive correlation between T-cell counts and disease severity, as well as increased T-cells and cytokine secreting cells in organs from HPS cases (Terajima and Ennis, 2011). However, it is important to consider that correlation does not imply causation. Alterations in activated T-cells during the course of hantavirus infection might, or might not, contribute to HPS pathogenesis in humans.
Recently the hamster model was utilized to test the hypothesis of T-cell mediated HPS pathogenesis. Supporting the current postulate of human HPS, an accumulation of activated T-cells was observed in the lungs of infected hamsters which corresponded to disease progression and the onset of pulmonary manifestations. However, the course and outcome of HPS disease did not differ in animals depleted for T-cells by cyclophosphamide or CD4+ or CD8+ specific antibody treatments compared to non-depleted controls (Hammerbeck and Hooper, 2011). These experimental findings demonstrate that T-cells are not required for HPS in the hamster model, and suggest that T-cells are not required for HPS in humans. In a second study, ANDV infection in hamsters was shown to result in immune modulation with a global suppression of innate responses early after infection, followed by an aberrant and uncontrolled Th1/Th2 activation in lungs and heart, immediately preceding physical signs of disease (Safronetz et al., 2011b). Similarly, studies conducted on autopsy samples from HPS cases have found increased cytokine expression levels in lung or heart samples from fatal cases (Mori et al., 1999; Saggioro et al., 2007).
These observations implicate tissue-specific deleterious host responses in HPS disease progression, although the cell types responsible were not addressed in the hamster model. In light of these two studies, it is reasonable to assume that immune modulation (T-cell and non T-cell) occurs in HPS. Whether non T-cell immune response plays a causal role in HPS remains to be determined. In support of a role for non T-cell involvement, activated T-cells were shown to accumulate in the spleen of infected hamsters, however irregular immune responses were not observed in this organ (Hammerbeck and Hooper, 2011; Safronetz et al., 2011b). Combined, these two studies highlight the complexity of HPS pathogenesis and demonstrate the usefulness of the hamster model for hypothesis testing.
An important question which remains to be answered, and which could improve our understanding of HPS pathogenesis, is why ANDV (and to a lesser extent MAPV) infection results in lethal HPS disease in hamsters, while infection with other prominent etiological agents of HPS does not cause any signs of illness? Although, SNV is highly infectious in hamsters with a 50% infectious dose of 2 pfu, it achieves only low level dissemination with occasional immunohistochemical staining of infected cells in the endothelium and no detectable viremia by infectious assay or sensitive real-time RT-PCR (Wahl-Jensen et al., 2007). Despite low level replication, hamsters infected with SNV seroconvert and resist back-challenge with ANDV (Hooper et al., 2001). Choclo virus, a South American hantavirus phylogenetically similar to MAPV and ANDV and an etiological agent of HPS in humans, is also non-pathogenic in hamsters. However, unlike SNV, Choclo viral antigen is readily detectable throughout the endothelial cell lining of the lung microvasculature (Eyzaguirre et al., 2008). Despite similar replication kinetics as ANDV in hamster lungs, Choclo virus did not result in pulmonary edema or inflammatory cell infiltrates, demonstrating that replication alone is insufficient to cause disease. Interestingly, HTNV and PUUV infection of hamsters also results in high levels of viral antigen in the pulmonary endothelium (Kamrud et al., 1999; Schmaljohn et al., 1990). To date, studies addressing immune suppression and subsequent activation in hamsters following infection with non-pathogenic agents (like SNV or Choclo virus) have not been reported. These studies should be conducted as it seems plausible that ANDV is lethal in hamsters due to its direct and possibly unique ability to modulate the host responses in these animals.
In humans, receptor usage is one of the few differences between pathogenic and non-pathogenic hantaviruses, with the former shown to utilize the αvβ3 integrin, while the latter uses the αvβ1 integrin (Gavrilovskaya et al., 1999; Gavrilovskaya et al., 1998). Based on these findings, receptor usage of hantaviruses in hamsters has been questioned as a marker of pathogenesis with some suggesting that ANDV utilizes the αvβ3 integrin in hamsters, whereas non-pathogenic hantaviruses in hamsters use an alternate receptor (Matthys et al., 2010). However, one of the original studies conducted in hamsters attempted to ascribe pathogenesis to specific ANDV proteins by infecting animals with a reassortant virus containing the small and large genomic segments (encoding the nucleoprotein and polymerase, respectively) of SNV and the medium segment (encoding the glycoproteins, Gn and Gc) of ANDV. While the “S-A-S” virus was highly infectious in hamsters, it was non-lethal, suggesting the glycoproteins, and by extension the cellular receptor, are not solely responsible for disease in the model (McElroy et al., 2004).
Based on the recent findings of Choclo virus infection and MAPV disease, similar studies conducted in hamsters with reassortant viruses containing genomic segments of ANDV in the genetic background of more closely related South American viruses may help elucidate the viral determinants involved in lethal disease in hamsters. Alternatively, one could consider passaging HPS (or HFRS) causing viruses in hamsters in attempt at lethally adapting them. Although host adaptation of a pathogen is not an ideal situation for a disease model, determining the molecular changes associated with the acquisition of a lethal phenotype might provide exceptional insight into HPS pathogenesis in hamsters. A caveat of this approach is without a reverse genetics system to assess the impact of individual mutations this experimental approach would not be able to completely address this question.
5. Prevention strategies
To date, prevention strategies for HPS exclusively rely on educational campaigns aimed at minimizing contact with rodents and/or potentially contaminated rodent excreta/secreta. Based on the vast differences in incidence of disease, it is not surprising that several HFRS vaccines have been evaluated in clinical trials, whereas no HPS vaccine has reached that stage of development (Schmaljohn, 2009). The usefulness of an HPS vaccine in North America is debatable based on a relatively low frequency of disease which primarily occurs as sporadic cases. However, in South America the incidence of HPS is higher and multiple cases of HPS in the same temporal and geographical proximity are more common which suggests a vaccine could be beneficial. To date, the ANDV hamster model has been utilized in at least five vaccine studies which have provided unique insight into the development of hantavirus vaccines (Brown et al., 2011; Custer et al., 2003; Hooper et al., 2006; Martinez and Padula, 2012; Safronetz et al., 2009).
Currently, the most successful vaccine strategy against hantaviruses are plasmids carrying the medium genomic segment (expressing the glycoproteins) of hantaviruses. These DNA based vaccines have proven to be highly effective at inducing neutralizing antibodies and preventing infection with etiological agents of HFRS in several animal models, including hamsters (Hammerbeck and Hooper, 2010). HTNV and PUUV medium genome segment-based plasmids, and a combination of the two, were recently tested in a Phase 1 clinical trial and were shown to be capable of eliciting high-titer neutralizing antibodies in humans (Boudreau et al., 2012). Interestingly, an ANDV based DNA vaccine, which elicited a strong neutralizing antibody response in NHPs, was found to be non-immunogenic in hamsters (Custer et al., 2003). It is unclear why hamsters did not respond to immunization with the DNA based vaccine; however it is plausible that the ANDV glycoproteins were not recognized by the hamster innate responses and although the vaccination failed, this may hint at an underlying reason why ANDV is lethal in hamsters. Further work on this hypothesis is underway. Nevertheless, over-expression of ANDV glycoproteins in hamsters using recombinant replication competent vesicular stomatitis virus (VSV) vectors results in the development of neutralizing antibodies and provides sterile protection against a lethal challenge, even when administered as few as 14 days prior to challenge (Brown et al., 2011). Additionally, immunization of hamsters with replication deficient adenovirus vectors expressing individual ANDV structural proteins (Gn, Gc, or NP) also resulted in complete protection from lethal disease(Safronetz et al., 2009). Although the protective responses associated with immunization of individual structural proteins were insufficient to completely prevent virus replication in hamsters (as determined by detection of ANDV RNA in tissues), these studies demonstrated immunity to the ANDV Gn protein is more effective at reducing ANDV replication than immunity to the Gc or NP antigens. Additionally, the protective responses associated with immunization of the recombinant adenovirus vectors was not associated with the induction of neutralizing antibodies, suggesting that a cellular immune response, or possibly a strong non-neutralizing antibody response, is capable of preventing HPS. In partial support of this, monoclonal antibodies specific for HTNV nucleoprotein were shown to prevent lethal HTNV infection in new-borne mice (Yoshimatsu et al., 1996). Recently, a study demonstrated that it was possible to protect Syrian hamsters from lethal HPS caused by ANDV by vaccinating with a purified NP antigen (Martinez and Padula, 2012).
6. Treatment strategies
Treatment of HPS in humans generally relies on supportive measures including intubation, mechanical ventilation, and in severe cases extracorporeal membrane oxygenation (Dietl et al., 2008; Jonsson et al., 2008). At present there are no published reports of successful treatment specially targeting HPS. Furthermore, relatively few modalities have been evaluated against HPS disease in the lethal hamster model.
At least two trials on the effectiveness of intravenous ribavirin against HPS have been conducted in humans, and although both studies were hindered by low enrollment rates, the results suggested no beneficial effect of ribavirin when treatment was initiated after symptom onset (Chapman et al., 1999; Mertz et al., 2004). Recently, ribavirin was evaluated in the hamster model of HPS, and while treatment was highly effective at preventing lethal disease, even at low doses, efficacy was dependent on early initiation of treatment (Safronetz et al., 2011a). The parallels between the human trials and the recent studies in hamsters further validate the use of the model for evaluating future therapies. Although no other antiviral agents have been tested against HPS in either humans or hamsters, some, including T-705, have shown promise in vitro and should be evaluated using in vivo models (Buys et al., 2011).
Antibody therapy has been successfully utilized as postexposure treatment for several viral diseases, including those caused by high-consequence pathogens such as Junin virus (Enria et al., 2008). To date clinical trials have not been published on the efficacy of immune therapy for the treatment of HPS, though experimental evidence suggest passive transfer of neutralizing antibodies can protect against hantavirus infection and disease (Arikawa et al., 1992; Custer et al., 2003; Hooper et al., 2008; Medina et al., 2007; Schmaljohn et al., 1990; Xu et al., 2002; Zhang et al., 1989). In the hamster model, administration of polyclonal sera from NHPs or rabbits previously immunized with a DNA vaccine expressing the ANDV medium segment as late as 5 days post-infection remained highly effective at preventing lethal disease (Custer et al., 2003; Hooper et al., 2008). More recently, the ANDV hamster model was used to demonstrate the postexposure efficacy of naturally despeciated (Fc fragment removed) IgY antibody purified from the eggs of ducks vaccinated with the ANDV DNA vaccine (Brocato et al., 2012). These findings are supported by clinical observations suggesting that neutralizing antibody titers are inversely correlated with disease severity (Bharadwaj et al., 2000). Similarly, patients with low levels of viremia at the time of hospital admission tend to have a milder course of disease (Xiao et al., 2006).
7. Promising avenues of research
The therapeutic modalities tested in the hamster model thus far have proven highly effective at preventing lethal HPS disease when administered relatively early after exposure (Brocato et al., 2012; Brown et al., 2011; Custer et al., 2003; Hooper et al., 2008; Safronetz et al., 2011a) (Figure 2). These studies have provided insight into reducing HPS disease associated with potential exposures like needle stick injuries, rodent bites or close contacts of confirmed cases of HPS associated with ANDV; however, they are not optimal in a clinical scenario where most cases of HPS are diagnosed post-symptom onset. At present, no therapeutic strategy has successfully reversed or reduced HPS disease progression post-symptom onset in hamsters, with most treatments evaluated demonstrating significantly reduced or no efficacy following the onset of viremia.
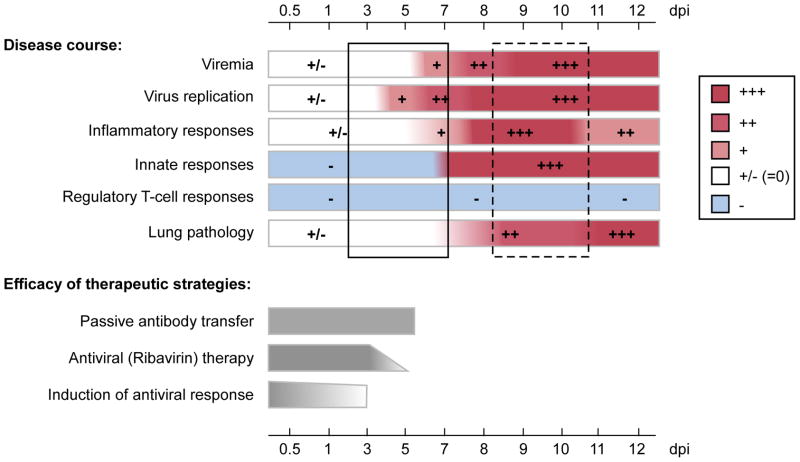
To date, all postexposure modalities tested in the hamster model of HPS have only been successful at preventing lethal disease if administered prior to the onset of viremia. Passive transfer of neutralizing antibodies has demonstrated the best postexposure protective efficacy with 100% survival observed even when antibodies are administered as late as 5 days postinfection. Antiviral (ribavirin) therapy is 100% efficacious when therapy is initiated within 3 days postinfection. Non-specific induction of innate antiviral responses is capable of protecting animals however only when administered early after infection. The solid box shows the current therapeutic window for treating HPS in hamsters. The hatched box indicates the clinically relevant therapeutic window based on onset of physical signs of disease. Attempts to protect hamsters during this period have largely failed. Note that the disease course can be extended depending on the route and/or dose of challenge. For example, the time to lethal disease in hamsters >8 weeks of age following an intranasal challenge of 2000 plaque forming units is 17.5 days (Hooper et al., 2008).
The abrupt onset of pulmonary manifestations combined with the rapid deterioration to life-threatening disease suggests medical interventions for the treatment of HPS will need to preferentially target the lungs and be fast-acting. The results of the ribavirin trials in both humans and hamsters suggest that reducing viral replication alone will be insufficient to treat HPS after the onset of pulmonary deficiencies. Based on the pathophysiology of disease, a multifaceted approach utilizing synergistic components to minimize viral replication and most importantly reverse the deleterious host immune responses will likely be required. Nevertheless, efforts to evaluate novel antiviral agents should continue with the hope of identifying effective modalities which can be utilized in post exposure scenarios or as a component of combination therapy.
The recent identification of cellular pathways which potentially contribute to the disruption of endothelium also provides targets for therapeutics which can be tested in vivo. Most notable are the recent findings that ANDV infection of primary lung endothelial cells results in increased secretion of vascular endothelial growth factor (VEGF) which subsequently results in decreased vascular endothelial cadherin (VE-cadherin), a main component of adherens junctions (Taddei et al., 2008). The internalization and degradation of VE-cadherin is initiated after VEGF binds VEGF-R2 and results in the disruption of the pulmonary endothelium leading to edema. In vitro studies have suggested the VEGF-VEGF-R2-VE-cadherin cascade is involved in the dysregulation of the vascular endothelium leading to increased permeability and therefore propose a target for the prevention of HPS (Gorbunova et al., 2010; Shrivastava-Ranjan et al., 2010). Consistent with this, VEGF-R2 and Src kinases inhibitors have been shown to decrease endothelial cell permeability in vitro (Gorbunova et al., 2011). The hamster model offers an attractive in vivo option to further this research and evaluate possible compounds, many of which are already FDA approved for clinical use, to block this pathway,(Gorbunova et al., 2011).
Recently, Brown and colleagues demonstrated that treatment of hamsters with a recombinant VSV construct expressing the Ebola virus glycoprotein on days 1 or 3 post-ANDV exposure is sufficient to protect 100% and 67% of animals, respectively (Brown et al., 2011). While the mechanism of the non-specific protection is not fully understood, the authors demonstrated the treatment resulted in the induction of strong type I interferon responses, which may have inhibited ANDV replication early after infection. These studies suggest the possibility of immune modulation in preventing HPS and are the basis for on-going work aimed at reversing the deleterious host responses associated with HPS by the induction of potent regulatory responses, which appear to be absent in HPS in humans and hamsters (Borges et al., 2008; Safronetz et al., 2011b). It is important to note that if HPS is an immune-mediated disease, then therapies aimed at modulating the host immune responses in hamsters may not necessarily directly translate to humans. However, based on the apparent similarities of HPS in hamsters and humans, at the very least, the model can identify promising targets or pathways and provide proof of concept for immunotherapeutics which will ultimately advance treatment strategies for clinical use.
8. Future challenges
An often overlooked application of disease models is addressing diagnostic challenges. Clinically, HPS is commonly identified by the abrupt onset of respiratory distress. This late diagnosis based on clinical presentation severely hampers the development and implementation of therapeutics. The hamster model offers a unique opportunity to evaluate early markers of disease which could help direct research aimed at developing rapid diagnostic tests for HPS. Early and rapid diagnosis of HPS will widen the therapeutic window for treating severe disease and with the development of potent therapeutics, ultimately help lower the mortality rates associated with HPS. However, a major limitation for this work is the lack of hamster specific reagents. Unlike the ubiquitously used laboratory mouse for which a vast array of specific or cross-reactive reagents is readily available, hamster models suffer from a deficiency of commercially available reagents (Tables 4 and and5).5). The hamster genome has not been sequenced. This is inexcusable when one considers that the hamster is one of the most widely used laboratory animals across multiple scientific disciplines. The paucity of genetic data has impaired the development of screening tools like micro-arrays and recombinant hamster cytokines and other reagents. Further, attempts to characterize cross-reactive antibodies for the detection and quantification of cytokines in Syrian hamsters have been largely unproductive, although some rodent platforms cross-react with hamster chemokines (Table 4) (Gowen et al., 2010; Safronetz et al., 2011b). Recent advances, including the development or characterization of antibodies recognizing lymphocyte markers for Syrian hamsters as well as real-time RT-PCR assays to monitor changes in immunological responses in hamsters have been already proven useful in disease models for SV-40, Nipah virus and HPS (Hammerbeck and Hooper, 2011; McNees et al., 2009; Rockx et al., 2011; Safronetz et al., 2011b; Zivcec et al., 2011). As hamsters continue to be used as models for HPS and other infectious diseases, it is imperative that specific reagents be developed.
Table 4
Serological reagents which were developed for or cross-react with Syrian hamsters
Cross-reactive antibodies (clone)a
| |
Mouse MHC II (14–4–4s) | Rat CD4 (GK1.5) |
Mouse Thy1.2 (HO-13–4) | Rat CD8β (341) |
| |
Syrian hamster specific antibodies (clone)b
| |
MHC II (H42A, HAL4A, HAL16A, HAT16A, HAT17A) | Pan lymphocyte (HAB6A) |
Dendritic cell marker (HAB4A) | CD45 (HASA25A) |
T cell (HAT24A, HAL3A, HAL9A, HAB1A, HAT19A) | CD18 (BAQ30A) |
B cell (HASA7A, HAL24A)
| |
Cross-reactive cytokine/chemokine assays
c, d,e
| |
Rat GM-CSF | Rodent VCAM-1 |
Rat Leptin | Rodent von Willebrand Factor |
Rat GRO/KC | Rodent VEGF |
Rat/Rodent IL-1α | Rodent MDC |
Mouse MIG | Rodent Stem Cell factor |
Mouse IL-13 | Rodent GCP-2 |
Rodent IP-10 | Rodent MCP-3 |
Rodent M-CSF | Rodent MIP-2 |
Rodent MCP 1 | Rodent MIP-3β |
Table 5
Molecular tools designed for Syrian hamsters a
Category | Assays described |
---|---|
House-keeping | β2 microglobulin, β-actin, Hypoxanthine phosphoribosyl-transferase (HPRT), Ribosomal protein L-18 (RPL-18) |
Anti-inflammatory/immunoregulatory | Interleukin (IL)-4, IL-10, Forkhead box P3 (FoxP3), Transforming growth factor (TGF)-β1, TGF- β2, TGF-β3, TGF-β type I receptor, MHC II α chain |
Pro-apoptotic/cell regulatory | B-cell lymphoma 2 (Bcl-2) associated protein, Platelet endothelial cell adhesion molecule (PECAM) |
Anti-apoptotic | Bcl-2 |
Cell junction integrity | E-cadherin, Tight junction protein 2, Junction adhesion molecule, Claudulin-1, Occuldin, Matrix metalloproteinase-2, Tissue inhibitor of matrix metalloproteinase-2 |
Clotting | Fibrinogen A α chain |
Vascular growth | Vascular endothelial cell growth factor |
Pro-inflammatory/Chemotaxis/T-cell activation | IL-1β, IL-2, IL-2 receptor α, IL-6, IL-6 transducer, IL-12p35 and p40, IL-21, IP-10, Intercellular adhesion molecule 1, Signal transducer and activator of transcription (STAT) 1, STAT-1β, STAT-2, Interferon (IFN) γ, Interferon regulatory factor (IRF)-1, IRF-2, Tumor necrosis factor (TNF) α, p75 TNF membrane receptor, Myxovirus resistance protein 2, Protein kinase R, IFNα inducible protein p27, CD83, Macrophage inflammatory protein 3α, Nitric oxide synthase (NOS) 2, inducible NOS, Complement C3d region, Complement components 5 and C1qBP, Chemokine ligands 17 and 22, epithelial mucin |
9. Concluding remarks
The intent of an animal model is to provide insight into the pathogenesis of disease for the purpose of developing and evaluating efficacy of medical countermeasures. Highlighting this point, the FDA’s Animal Rule outlines the necessary requirements of an animal model if it is to be used in the initial approval of therapeutic modalities or vaccines in situations where human efficacy studies are not ethical or feasible, as is the case for high consequence, emerging viral pathogens (Table 6). According to the FDA guidelines, an ideal disease model should faithfully recapitulate the characteristics of the human condition as closely as possible in an adult, immunocompetent animal following infection with a wild-type (i.e., not host-adapted) etiological agent of human disease at a realistic dose via an appropriate route of exposure (FDA, 2009). The studies conducted to date in the hamster model of HPS suggest it meets all the requirements of the FDA Animal Rule. Thus, hamsters represent a convenient and robust disease model which currently provides the best in vivo opportunity to study hantavirus pathogenicity as well as evaluating potential medical countermeasures against these high consequence pathogens.
Table 6
Characteristics of an ideal animal model of infectious diseasea
The challenge agent should:
|
The host should:
|
The disease condition in the animal host should:
|
Acknowledgments
The authors thank Anita Mora for preparing the figures and Martha Thayer for conducting the literature searches (both from Division of Intramural Research, National Institute of Allergy and Infectious Disease, National Institutes of Health, (DIR, NIAID, NIH)).
Footnotes
Publisher's Disclaimer: This is a PDF file of an unedited manuscript that has been accepted for publication. As a service to our customers we are providing this early version of the manuscript. The manuscript will undergo copyediting, typesetting, and review of the resulting proof before it is published in its final citable form. Please note that during the production process errors may be discovered which could affect the content, and all legal disclaimers that apply to the journal pertain.
References
- Arikawa J, Yao JS, Yoshimatsu K, Takashima I, Hashimoto N. Protective role of antigenic sites on the envelope protein of Hantaan virus defined by monoclonal antibodies. Arch Virol. 1992;126:271–281. [Europe PMC free article] [Abstract] [Google Scholar]
- Bharadwaj M, Nofchissey R, Goade D, Koster F, Hjelle B. Humoral immune responses in the hantavirus cardiopulmonary syndrome. J Infect Dis. 2000;182:43–48. [Abstract] [Google Scholar]
- Billings AN, Rollin PE, Milazzo ML, Molina CP, Eyzaguirre EJ, Livingstone W, Ksiazek TG, Fulhorst CF. Pathology of Black Creek Canal virus infection in juvenile hispid cotton rats (Sigmodon hispidus) Vector Borne Zoonotic Dis. 2010;10:621–628. [Europe PMC free article] [Abstract] [Google Scholar]
- Borges AA, Campos GM, Moreli ML, Souza RL, Aquino VH, Saggioro FP, Figueiredo LT. Hantavirus cardiopulmonary syndrome: immune response and pathogenesis. Microbes Infect. 2006;8:2324–2330. [Abstract] [Google Scholar]
- Borges AA, Campos GM, Moreli ML, Moro Souza RL, Saggioro FP, Figueiredo GG, Livonesi MC, Moraes Figueiredo LT. Role of mixed Th1 and Th2 serum cytokines on pathogenesis and prognosis of hantavirus pulmonary syndrome. Microbes Infect. 2008;10:1150–1157. [Abstract] [Google Scholar]
- Boroja M, Barrie JR, Raymond GS. Radiographic findings in 20 patients with Hantavirus pulmonary syndrome correlated with clinical outcome. AJR Am J Roentgenol. 2002;178:159–163. [Abstract] [Google Scholar]
- Botten J, Mirowsky K, Kusewitt D, Bharadwaj M, Yee J, Ricci R, Feddersen RM, Hjelle B. Experimental infection model for Sin Nombre hantavirus in the deer mouse (Peromyscus maniculatus) Proc Natl Acad Sci U S A. 2000;97:10578–10583. [Europe PMC free article] [Abstract] [Google Scholar]
- Boudreau EF, Josleyn M, Ullman D, Fisher D, Dalrymple L, Sellers-Myers K, Loudon P, Rusnak J, Rivard R, Schmaljohn C, Hooper JW. A Phase 1 clinical trial of Hantaan virus and Puumala virus M-segment DNA vaccines for hemorrhagic fever with renal syndrome. Vaccine. 2012;30:1951–1958. [Abstract] [Google Scholar]
- Brocato R, Josleyn M, Ballantyne J, Vial P, Hooper JW. DNA Vaccine-Generated Duck Polyclonal Antibodies as a Postexposure Prophylactic to Prevent Hantavirus Pulmonary Syndrome (HPS) PLoS One. 2012;7:e35996. [Europe PMC free article] [Abstract] [Google Scholar]
- Brown KS, Safronetz D, Marzi A, Ebihara H, Feldmann H. Vesicular stomatitis virus-based vaccine protects hamsters against lethal challenge with Andes virus. J Virol. 2011;85:12781–12791. [Europe PMC free article] [Abstract] [Google Scholar]
- Buys KK, Jung KH, Smee DF, Furuta Y, Gowen BB. Maporal virus as a surrogate for pathogenic New World hantaviruses and its inhibition by favipiravir. Antivir Chem Chemother. 2011;21:193–200. [Europe PMC free article] [Abstract] [Google Scholar]
- Campen MJ, Milazzo ML, Fulhorst CF, Obot Akata CJ, Koster F. Characterization of shock in a hamster model of hantavirus infection. Virology. 2006;356:45–49. [Abstract] [Google Scholar]
- Castillo C, Naranjo J, Sepulveda A, Ossa G, Levy H. Hantavirus pulmonary syndrome due to Andes virus in Temuco, Chile: clinical experience with 16 adults. Chest. 2001;120:548–554. [Abstract] [Google Scholar]
- CDC. Hantavirus pulmonary syndrome (hantavirus disease) (HPS) 2010 case definition. 2010 http://www.cdc.gov/osels/ph_surveillance/nndss/print/hantaviruscurrent.htm.
- Chapman LE, Mertz GJ, Peters CJ, Jolson HM, Khan AS, Ksiazek TG, Koster FT, Baum KF, Rollin PE, Pavia AT, Holman RC, Christenson JC, Rubin PJ, Behrman RE, Bell LJ, Simpson GL, Sadek RF. Intravenous ribavirin for hantavirus pulmonary syndrome: safety and tolerance during 1 year of open-label experience. Ribavirin Study Group. Antivir Ther. 1999;4:211–219. [Abstract] [Google Scholar]
- Custer DM, Thompson E, Schmaljohn CS, Ksiazek TG, Hooper JW. Active and passive vaccination against hantavirus pulmonary syndrome with Andes virus M genome segment-based DNA vaccine. J Virol. 2003;77:9894–9905. [Europe PMC free article] [Abstract] [Google Scholar]
- Dietl CA, Wernly JA, Pett SB, Yassin SF, Sterling JP, Dragan R, Milligan K, Crowley MR. Extracorporeal membrane oxygenation support improves survival of patients with severe Hantavirus cardiopulmonary syndrome. J Thorac Cardiovasc Surg. 2008;135:579–584. [Abstract] [Google Scholar]
- Duchin JS, Koster FT, Peters CJ, Simpson GL, Tempest B, Zaki SR, Ksiazek TG, Rollin PE, Nichol S, Umland ET, et al. Hantavirus pulmonary syndrome: a clinical description of 17 patients with a newly recognized disease. The Hantavirus Study Group. N Engl J Med. 1994;330:949–955. [Abstract] [Google Scholar]
- Easterbrook JD, Zink MC, Klein SL. Regulatory T cells enhance persistence of the zoonotic pathogen Seoul virus in its reservoir host. Proc Natl Acad Sci U S A. 2007;104:15502–15507. [Europe PMC free article] [Abstract] [Google Scholar]
- Ebihara H, Yoshimatsu K, Ogino M, Araki K, Ami Y, Kariwa H, Takashima I, Li D, Arikawa J. Pathogenicity of Hantaan virus in newborn mice: genetic reassortant study demonstrating that a single amino acid change in glycoprotein G1 is related to virulence. J Virol. 2000;74:9245–9255. [Europe PMC free article] [Abstract] [Google Scholar]
- Enria DA, Briggiler AM, Pini N, Levis S. Clinical manifestations of New World hantaviruses. Curr Top Microbiol Immunol. 2001;256:117–134. [Abstract] [Google Scholar]
- Enria DA, Briggiler AM, Sanchez Z. Treatment of Argentine hemorrhagic fever. Antiviral Res. 2008;78:132–139. [Europe PMC free article] [Abstract] [Google Scholar]
- Eyzaguirre EJ, Milazzo ML, Koster FT, Fulhorst CF. Choclo virus infection in the Syrian golden hamster. Am J Trop Med Hyg. 2008;78:669–674. [Europe PMC free article] [Abstract] [Google Scholar]
- FDA. Guidance for Industry: Animal Model- Essential Elements to Address Efficacy Under the Animal Rule. 2009 Jan;2009 http://www.fda.gov/downloads/Drugs/GuidanceComplianceRegulatoryInformation/Guidances/ucm078923.pdf. [Google Scholar]
- Fulhorst CF, Milazzo ML, Duno G, Salas RA. Experimental infection of the Sigmodon alstoni cotton rat with Cano Delgadito virus, a South American hantavirus. Am J Trop Med Hyg. 2002;67:107–111. [Abstract] [Google Scholar]
- Gavrilovskaya IN, Shepley M, Shaw R, Ginsberg MH, Mackow ER. beta3 Integrins mediate the cellular entry of hantaviruses that cause respiratory failure. Proc Natl Acad Sci U S A. 1998;95:7074–7079. [Europe PMC free article] [Abstract] [Google Scholar]
- Gavrilovskaya IN, Brown EJ, Ginsberg MH, Mackow ER. Cellular entry of hantaviruses which cause hemorrhagic fever with renal syndrome is mediated by beta3 integrins. J Virol. 1999;73:3951–3959. [Europe PMC free article] [Abstract] [Google Scholar]
- Gorbunova E, Gavrilovskaya IN, Mackow ER. Pathogenic hantaviruses Andes virus and Hantaan virus induce adherens junction disassembly by directing vascular endothelial cadherin internalization in human endothelial cells. J Virol. 2010;84:7405–7411. [Europe PMC free article] [Abstract] [Google Scholar]
- Gorbunova EE, Gavrilovskaya IN, Pepini T, Mackow ER. VEGFR2 and Src kinase inhibitors suppress Andes virus-induced endothelial cell permeability. J Virol. 2011;85:2296–2303. [Europe PMC free article] [Abstract] [Google Scholar]
- Gowen BB, Holbrook MR. Animal models of highly pathogenic RNA viral infections: hemorrhagic fever viruses. Antiviral Res. 2008;78:79–90. [Abstract] [Google Scholar]
- Gowen BB, Julander JG, London NR, Wong MH, Larson D, Morrey JD, Li DY, Bray M. Assessing changes in vascular permeability in a hamster model of viral hemorrhagic fever. Virol J. 2010;7:240. [Europe PMC free article] [Abstract] [Google Scholar]
- Hammerbeck CD, Hooper JW. Hantavirus Vaccines. In: Levin MM, editor. New Generation Vaccines. 4. Informa Healthcare; New York: 2010. pp. 905–913. [Google Scholar]
- Hammerbeck CD, Hooper JW. T cells are not required for pathogenesis in the Syrian hamster model of hantavirus pulmonary syndrome. J Virol. 2011;85:9929–9944. [Europe PMC free article] [Abstract] [Google Scholar]
- Hooper JW, Kamrud KI, Elgh F, Custer D, Schmaljohn CS. DNA vaccination with hantavirus M segment elicits neutralizing antibodies and protects against seoul virus infection. Virology. 1999;255:269–278. [Abstract] [Google Scholar]
- Hooper JW, Larsen T, Custer DM, Schmaljohn CS. A lethal disease model for hantavirus pulmonary syndrome. Virology. 2001;289:6–14. [Abstract] [Google Scholar]
- Hooper JW, Custer DM, Smith J, Wahl-Jensen V. Hantaan/Andes virus DNA vaccine elicits a broadly cross-reactive neutralizing antibody response in nonhuman primates. Virology. 2006;347:208–216. [Abstract] [Google Scholar]
- Hooper JW, Ferro AM, Wahl-Jensen V. Immune serum produced by DNA vaccination protects hamsters against lethal respiratory challenge with Andes virus. J Virol. 2008;82:1332–1338. [Europe PMC free article] [Abstract] [Google Scholar]
- Huggins JW, Kim GR, Brand OM, McKee KT., Jr Ribavirin therapy for Hantaan virus infection in suckling mice. J Infect Dis. 1986;153:489–497. [Abstract] [Google Scholar]
- Hutchinson KL, Rollin PE, Peters CJ. Pathogenesis of a North American hantavirus, Black Creek Canal virus, in experimentally infected Sigmodon hispidus. Am J Trop Med Hyg. 1998;59:58–65. [Abstract] [Google Scholar]
- ICTV. Virus Taxonomy. 2009 http://ictvonline.org/virusTaxonomy.asp.
- Jonsson CB, Hooper J, Mertz G. Treatment of hantavirus pulmonary syndrome. Antiviral Res. 2008;78:162–169. [Europe PMC free article] [Abstract] [Google Scholar]
- Jonsson CB, Figueiredo LT, Vapalahti O. A global perspective on hantavirus ecology, epidemiology, and disease. Clin Microbiol Rev. 2010;23:412–441. [Europe PMC free article] [Abstract] [Google Scholar]
- Kamrud KI, Hooper JW, Elgh F, Schmaljohn CS. Comparison of the protective efficacy of naked DNA, DNA-based Sindbis replicon, and packaged Sindbis replicon vectors expressing Hantavirus structural genes in hamsters. Virology. 1999;263:209–219. [Abstract] [Google Scholar]
- Klein SL, Bird BH, Glass GE. Sex differences in immune responses and viral shedding following Seoul virus infection in Norway rats. Am J Trop Med Hyg. 2001;65:57–63. [Abstract] [Google Scholar]
- Klingstrom J, Plyusnin A, Vaheri A, Lundkvist A. Wild-type Puumala hantavirus infection induces cytokines, C-reactive protein, creatinine, and nitric oxide in cynomolgus macaques. J Virol. 2002;76:444–449. [Europe PMC free article] [Abstract] [Google Scholar]
- Klingstrom J, Hardestam J, Lundkvist A. Dobrava, but not Saaremaa, hantavirus is lethal and induces nitric oxide production in suckling mice. Microbes Infect. 2006;8:728–737. [Europe PMC free article] [Abstract] [Google Scholar]
- Kurata T, Tsai TF, Bauer SP, McCormick JB. Immunofluorescence studies of disseminated Hantaan virus infection of suckling mice. Infect Immun. 1983;41:391–398. [Europe PMC free article] [Abstract] [Google Scholar]
- Lee HW, Lee PW, Baek LJ, Song CK, Seong IW. Intraspecific transmission of Hantaan virus, etiologic agent of Korean hemorrhagic fever, in the rodent Apodemus agrarius. Am J Trop Med Hyg. 1981;30:1106–1112. [Abstract] [Google Scholar]
- Lee PW, Yanagihara R, Gibbs CJ, Jr, Gajdusek DC. Pathogenesis of experimental Hantaan virus infection in laboratory rats. Arch Virol. 1986;88:57–66. [Abstract] [Google Scholar]
- Lundkvist A, Kallio-Kokko H, Sjolander KB, Lankinen H, Niklasson B, Vaheri A, Vapalahti O. Characterization of Puumala virus nucleocapsid protein: identification of B-cell epitopes and domains involved in protective immunity. Virology. 1996;216:397–406. [Abstract] [Google Scholar]
- Macneil A, Nichol ST, Spiropoulou CF. Hantavirus pulmonary syndrome. Virus Res. 2011;162:138–147. [Abstract] [Google Scholar]
- Martinez VP, Bellomo C, San Juan J, Pinna D, Forlenza R, Elder M, Padula PJ. Person-to-person transmission of Andes virus. Emerg Infect Dis. 2005;11:1848–1853. [Europe PMC free article] [Abstract] [Google Scholar]
- Martinez VP, Padula PJ. Induction of protective immunity in a Syrian hamster model against a cytopathogenic strain of Andes virus. J Med Virol. 2012;84:87–95. [Abstract] [Google Scholar]
- Matthys VS, Gorbunova EE, Gavrilovskaya IN, Mackow ER. Andes virus recognition of human and Syrian hamster beta3 integrins is determined by an L33P substitution in the PSI domain. J Virol. 2010;84:352–360. [Europe PMC free article] [Abstract] [Google Scholar]
- McElroy AK, Bray M, Reed DS, Schmaljohn CS. Andes virus infection of cynomolgus macaques. J Infect Dis. 2002;186:1706–1712. [Abstract] [Google Scholar]
- McElroy AK, Smith JM, Hooper JW, Schmaljohn CS. Andes virus M genome segment is not sufficient to confer the virulence associated with Andes virus in Syrian hamsters. Virology. 2004;326:130–139. [Abstract] [Google Scholar]
- McKee KT, Jr, Kim GR, Green DE, Peters CJ. Hantaan virus infection in suckling mice: virologic and pathologic correlates. J Med Virol. 1985;17:107–117. [Abstract] [Google Scholar]
- McNees AL, Vilchez RA, Heard TC, Sroller V, Wong C, Herron AJ, Hamilton MJ, Davis WC, Butel JS. SV40 lymphomagenesis in Syrian golden hamsters. Virology. 2009;384:114–124. [Europe PMC free article] [Abstract] [Google Scholar]
- Medina RA, Mirowsky-Garcia K, Hutt J, Hjelle B. Ribavirin, human convalescent plasma and anti-beta3 integrin antibody inhibit infection by Sin Nombre virus in the deer mouse model. J Gen Virol. 2007;88:493–505. [Abstract] [Google Scholar]
- Mertz GJ, Miedzinski L, Goade D, Pavia AT, Hjelle B, Hansbarger CO, Levy H, Koster FT, Baum K, Lindemulder A, Wang W, Riser L, Fernandez H, Whitley RJ. Placebo-controlled, double-blind trial of intravenous ribavirin for the treatment of hantavirus cardiopulmonary syndrome in North America. Clin Infect Dis. 2004;39:1307–1313. [Abstract] [Google Scholar]
- Milazzo ML, Eyzaguirre EJ, Molina CP, Fulhorst CF. Maporal viral infection in the Syrian golden hamster: a model of hantavirus pulmonary syndrome. J Infect Dis. 2002;186:1390–1395. [Abstract] [Google Scholar]
- Mori M, Rothman AL, Kurane I, Montoya JM, Nolte KB, Norman JE, Waite DC, Koster FT, Ennis FA. High levels of cytokine-producing cells in the lung tissues of patients with fatal hantavirus pulmonary syndrome. J Infect Dis. 1999;179:295–302. [Abstract] [Google Scholar]
- Murphy ME, Kariwa H, Mizutani T, Tanabe H, Yoshimatsu K, Arikawa J, Takashima I. Characterization of in vitro and in vivo antiviral activity of lactoferrin and ribavirin upon hantavirus. J Vet Med Sci. 2001;63:637–645. [Abstract] [Google Scholar]
- Neumann K, Michaux J, Lebedev V, Yigit N, Colak E, Ivanova N, Poltoraus A, Surov A, Markov G, Maak S, Neumann S, Gattermann R. Molecular phylogeny of the Cricetinae subfamily based on the mitochondrial cytochrome b and 12S rRNA genes and the nuclear vWF gene. Mol Phylogenet Evol. 2006;39:135–148. [Abstract] [Google Scholar]
- Padula PJ, Edelstein A, Miguel SD, Lopez NM, Rossi CM, Rabinovich RD. Hantavirus pulmonary syndrome outbreak in Argentina: molecular evidence for person-to-person transmission of Andes virus. Virology. 1998;241:323–330. [Abstract] [Google Scholar]
- Rockx B, Brining D, Kramer J, Callison J, Ebihara H, Mansfield K, Feldmann H. Clinical outcome of henipavirus infection in hamsters is determined by the route and dose of infection. J Virol. 2011;85:7658–7671. [Europe PMC free article] [Abstract] [Google Scholar]
- Safronetz D, Hegde NR, Ebihara H, Denton M, Kobinger GP, St Jeor S, Feldmann H, Johnson DC. Adenovirus vectors expressing hantavirus proteins protect hamsters against lethal challenge with andes virus. J Virol. 2009;83:7285–7295. [Europe PMC free article] [Abstract] [Google Scholar]
- Safronetz D, Haddock E, Feldmann F, Ebihara H, Feldmann H. In vitro and in vivo activity of ribavirin against Andes virus infection. PLoS One. 2011a;6:e23560. [Europe PMC free article] [Abstract] [Google Scholar]
- Safronetz D, Zivcec M, Lacasse R, Feldmann F, Rosenke R, Long D, Haddock E, Brining D, Gardner D, Feldmann H, Ebihara H. Pathogenesis and Host Response in Syrian Hamsters following Intranasal Infection with Andes Virus. PLoS Pathog. 2011b;7:e1002426. [Europe PMC free article] [Abstract] [Google Scholar]
- Saggioro FP, Rossi MA, Duarte MI, Martin CC, Alves VA, Moreli ML, Figueiredo LT, Moreira JE, Borges AA, Neder L. Hantavirus infection induces a typical myocarditis that may be responsible for myocardial depression and shock in hantavirus pulmonary syndrome. J Infect Dis. 2007;195:1541–1549. [Abstract] [Google Scholar]
- Sanada T, Kariwa H, Nagata N, Tanikawa Y, Seto T, Yoshimatsu K, Arikawa J, Yoshii K, Takashima I. Puumala virus infection in Syrian hamsters (Mesocricetus auratus) resembling hantavirus infection in natural rodent hosts. Virus Res. 2011;160:108–119. [Abstract] [Google Scholar]
- Schmaljohn C. Vaccines for hantaviruses. Vaccine. 2009;27(Suppl 4):D61–64. [Abstract] [Google Scholar]
- Schmaljohn CS, Chu YK, Schmaljohn AL, Dalrymple JM. Antigenic subunits of Hantaan virus expressed by baculovirus and vaccinia virus recombinants. J Virol. 1990;64:3162–3170. [Europe PMC free article] [Abstract] [Google Scholar]
- Schountz T, Prescott J, Cogswell AC, Oko L, Mirowsky-Garcia K, Galvez AP, Hjelle B. Regulatory T cell-like responses in deer mice persistently infected with Sin Nombre virus. Proc Natl Acad Sci U S A. 2007;104:15496–15501. [Europe PMC free article] [Abstract] [Google Scholar]
- Seto T, Nagata N, Yoshikawa K, Ichii O, Sanada T, Saasa N, Ozaki Y, Kon Y, Yoshii K, Takashima I, Kariwa H. Infection of Hantaan virus strain AA57 leading to pulmonary disease in laboratory mice. Virus Res. 2012;163:284–290. [Abstract] [Google Scholar]
- Shrivastava-Ranjan P, Rollin PE, Spiropoulou CF. Andes virus disrupts the endothelial cell barrier by induction of vascular endothelial growth factor and downregulation of VE-cadherin. J Virol. 2010;84:11227–11234. [Europe PMC free article] [Abstract] [Google Scholar]
- Sironen T, Klingstrom J, Vaheri A, Andersson LC, Lundkvist A, Plyusnin A. Pathology of Puumala hantavirus infection in macaques. PLoS One. 2008;3:e3035. [Europe PMC free article] [Abstract] [Google Scholar]
- St Jeor SC. Three-week incubation period for hantavirus infection. Pediatr Infect Dis J. 2004;23:974–975. [Abstract] [Google Scholar]
- Taddei A, Giampietro C, Conti A, Orsenigo F, Breviario F, Pirazzoli V, Potente M, Daly C, Dimmeler S, Dejana E. Endothelial adherens junctions control tight junctions by VE-cadherin-mediated upregulation of claudin-5. Nat Cell Biol. 2008;10:923–934. [Abstract] [Google Scholar]
- Tamura M, Asada H, Kondo K, Tanishita O, Kurata T, Yamanishi K. Pathogenesis of Hantaan virus in mice. J Gen Virol. 1989;70 ( Pt 11):2897–2906. [Abstract] [Google Scholar]
- Terajima M, Ennis FA. T cells and pathogenesis of hantavirus cardiopulmonary syndrome and hemorrhagic fever with renal syndrome. Viruses. 2011;3:1059–1073. [Europe PMC free article] [Abstract] [Google Scholar]
- Toro J, Vega JD, Khan AS, Mills JN, Padula P, Terry W, Yadon Z, Valderrama R, Ellis BA, Pavletic C, Cerda R, Zaki S, Shieh WJ, Meyer R, Tapia M, Mansilla C, Baro M, Vergara JA, Concha M, Calderon G, Enria D, Peters CJ, Ksiazek TG. An outbreak of hantavirus pulmonary syndrome, Chile, 1997. Emerg Infect Dis. 1998;4:687–694. [Europe PMC free article] [Abstract] [Google Scholar]
- Torres-Perez F, Wilson L, Collinge SK, Harmon H, Ray C, Medina RA, Hjelle B. Sin Nombre virus infection in field workers, Colorado, USA. Emerg Infect Dis. 2010;16:308–310. [Europe PMC free article] [Abstract] [Google Scholar]
- Vial PA, Valdivieso F, Mertz G, Castillo C, Belmar E, Delgado I, Tapia M, Ferres M. Incubation period of hantavirus cardiopulmonary syndrome. Emerg Infect Dis. 2006;12:1271–1273. [Europe PMC free article] [Abstract] [Google Scholar]
- Wahl-Jensen V, Chapman J, Asher L, Fisher R, Zimmerman M, Larsen T, Hooper JW. Temporal analysis of Andes virus and Sin Nombre virus infections of Syrian hamsters. J Virol. 2007;81:7449–7462. [Europe PMC free article] [Abstract] [Google Scholar]
- Wichmann D, Grone HJ, Frese M, Pavlovic J, Anheier B, Haller O, Klenk HD, Feldmann H. Hantaan virus infection causes an acute neurological disease that is fatal in adult laboratory mice. J Virol. 2002;76:8890–8899. [Europe PMC free article] [Abstract] [Google Scholar]
- Xiao R, Yang S, Koster F, Ye C, Stidley C, Hjelle B. Sin Nombre viral RNA load in patients with hantavirus cardiopulmonary syndrome. J Infect Dis. 2006;194:1403–1409. [Abstract] [Google Scholar]
- Xu X, Ruo SL, McCormick JB, Fisher-Hoch SP. Immunity to Hantavirus challenge in Meriones unguiculatus induced by vaccinia-vectored viral proteins. Am J Trop Med Hyg. 1992;47:397–404. [Abstract] [Google Scholar]
- Xu Z, Wei L, Wang L, Wang H, Jiang S. The in vitro and in vivo protective activity of monoclonal antibodies directed against Hantaan virus: potential application for immunotherapy and passive immunization. Biochem Biophys Res Commun. 2002;298:552–558. [Abstract] [Google Scholar]
- Yanagihara R, Amyx HL, Gajdusek DC. Experimental infection with Puumala virus, the etiologic agent of nephropathia epidemica, in bank voles (Clethrionomys glareolus) J Virol. 1985;55:34–38. [Europe PMC free article] [Abstract] [Google Scholar]
- Yanagihara R, Amyx HL, Lee PW, Asher DM, Gibbs CJ, Jr, Gajdusek DC. Experimental hantavirus infection in nonhuman primates. Arch Virol. 1988;101:125–130. [Abstract] [Google Scholar]
- Yoo YC, Yoshimatsu K, Yoshida R, Tamura M, Azuma I, Arikawa J. Comparison of virulence between Seoul virus strain SR-11 and Hantaan virus strain 76–118 of hantaviruses in newborn mice. Microbiol Immunol. 1993;37:557–562. [Abstract] [Google Scholar]
- Yoshimatsu K, Arikawa J, Tamura M, Yoshida R, Lundkvist A, Niklasson B, Kariwa H, Azuma I. Characterization of the nucleocapsid protein of Hantaan virus strain 76–118 using monoclonal antibodies. J Gen Virol. 1996;77 ( Pt 4):695–704. [Abstract] [Google Scholar]
- Young JC, Hansen GR, Graves TK, Deasy MP, Humphreys JG, Fritz CL, Gorham KL, Khan AS, Ksiazek TG, Metzger KB, Peters CJ. The incubation period of hantavirus pulmonary syndrome. Am J Trop Med Hyg. 2000;62:714–717. [Abstract] [Google Scholar]
- Zaki SR, Greer PW, Coffield LM, Goldsmith CS, Nolte KB, Foucar K, Feddersen RM, Zumwalt RE, Miller GL, Khan AS, et al. Hantavirus pulmonary syndrome. Pathogenesis of an emerging infectious disease. Am J Pathol. 1995;146:552–579. [Europe PMC free article] [Abstract] [Google Scholar]
- Zhang XK, Takashima I, Hashimoto N. Characteristics of passive immunity against hantavirus infection in rats. Arch Virol. 1989;105:235–246. [Abstract] [Google Scholar]
- Zivcec M, Safronetz D, Haddock E, Feldmann H, Ebihara H. Validation of assays to monitor immune responses in the Syrian golden hamster (Mesocricetus auratus) J Immunol Methods. 2011;368:24–35. [Europe PMC free article] [Abstract] [Google Scholar]
Full text links
Read article at publisher's site: https://doi.org/10.1016/j.antiviral.2012.06.002
Read article for free, from open access legal sources, via Unpaywall:
https://europepmc.org/articles/pmc3425723?pdf=render
Citations & impact
Impact metrics
Citations of article over time
Alternative metrics
Smart citations by scite.ai
Explore citation contexts and check if this article has been
supported or disputed.
https://scite.ai/reports/10.1016/j.antiviral.2012.06.002
Article citations
Preclinical evaluation of a fully human, quadrivalent-hantavirus polyclonal antibody derived from a non-human source.
mBio, 15(10):e0160024, 11 Sep 2024
Cited by: 0 articles | PMID: 39258903 | PMCID: PMC11481879
Bivalent VSV Vectors Mediate Rapid and Potent Protection from Andes Virus Challenge in Hamsters.
Viruses, 16(2):279, 11 Feb 2024
Cited by: 0 articles | PMID: 38400054 | PMCID: PMC10893017
Human-to-Human Transmission of Andes Virus Modeled in Syrian Hamsters.
Emerg Infect Dis, 29(10):2159-2163, 01 Oct 2023
Cited by: 0 articles | PMID: 37735788 | PMCID: PMC10521624
Two point mutations in protocadherin-1 disrupt hantavirus recognition and afford protection against lethal infection.
Nat Commun, 14(1):4454, 24 Jul 2023
Cited by: 0 articles | PMID: 37488123 | PMCID: PMC10366084
Animal models of mpox virus infection and disease.
Infect Med (Beijing), 2(3):153-166, 04 Jun 2023
Cited by: 4 articles | PMID: 38073883 | PMCID: PMC10699680
Review Free full text in Europe PMC
Go to all (50) article citations
Similar Articles
To arrive at the top five similar articles we use a word-weighted algorithm to compare words from the Title and Abstract of each citation.
A lethal disease model for hantavirus pulmonary syndrome.
Virology, 289(1):6-14, 01 Oct 2001
Cited by: 163 articles | PMID: 11601912
Depletion of Alveolar Macrophages Does Not Prevent Hantavirus Disease Pathogenesis in Golden Syrian Hamsters.
J Virol, 90(14):6200-6215, 24 Jun 2016
Cited by: 6 articles | PMID: 27099308 | PMCID: PMC4936146
Hantavirus pulmonary syndrome.
Virus Res, 162(1-2):138-147, 17 Sep 2011
Cited by: 90 articles | PMID: 21945215
Review
T cells are not required for pathogenesis in the Syrian hamster model of hantavirus pulmonary syndrome.
J Virol, 85(19):9929-9944, 20 Jul 2011
Cited by: 59 articles | PMID: 21775442 | PMCID: PMC3196444
Funding
Funders who supported this work.
Intramural NIH HHS (1)
Grant ID: Z99 AI999999
NIAID NIH HHS (1)
Grant ID: Y99 AI999999