Abstract
Free full text

Functional MRI of Mnemonic Networks across the spectrum of Normal Aging, Mild Cognitive Impairment and Alzheimer’s Disease
Abstract
Functional magnetic resonance imaging (fMRI) is a non-invasive technique that has come into common use to examine neural network function in normal and impaired cognitive states. Using this promising type of analysis, researchers have identified the presence of anatomically distributed regions operating as large-scale neural networks, which are observed both during the performance of associative memory tasks and in the resting state. The assembly of these anatomically distinct regions into functional ensembles and their choreographed activation and deactivation sets the stage for complex behaviors such as the formation and retrieval of associative memories. We review progress in the use of task-related and task-free MRI to elucidate the changes in neural activity in normal older individuals, patients with mild cognitive impairment (MCI), and those with Alzheimer’s disease (AD) dementia, focusing on the altered activity of the default mode network (DMN) and medial temporal lobe (MTL). We place task-free fMRI studies into the larger context of more traditional, task-based fMRI studies of human memory, which have firmly established the critical role of the MTL in associative encoding. Lastly, we discuss the data from our group and others that suggests task-free MRI and task-based fMRI may prove useful as non-invasive biomarkers in studying the progression of memory failure over the course of AD.
Introduction
Alzheimer’s disease (AD) affects more than 1 of every 10 individuals over the age of 65 years, and is projected to affect over 12 million Americans by 2050 [1]. Clinically, the most salient feature of AD is a degradation of episodic memory, and advances in functional neuroimaging have allowed for the detailed study of the mechanisms underlying pathologic decrements in memory encoding. Functional MRI (fMRI) studies suggest that human memory function depends on the interplay between structures within the medial temporal lobe memory system (MTL) and large-scale, anatomically distributed neural networks, whose activity is critical for successful mnemonic function.
Among the several large-scale neural networks that have been described, the default mode network (DMN) has been a particular area of focus, as it shows decreasing functional connectivity in AD and changes its activity during the performance of cognitively demanding tasks, including associative memory tasks. Furthermore, recent studies suggest that amyloid beta deposition may preferentially occur in the DMN very early in the course of Alzheimer’s disease (AD, [2–6]), raising the intriguing possibility that amyloid-induced dysregulation of the DMN may be a consequence of the pathobiologic process in AD. Importantly, alterations in DMN functional connectivity have also been observed in subjects with higher-than-baseline risk of AD [3,7,8], including those with increased genetic risk (e.g., APOE ε4 carriers) and those who have been diagnosed with mild cognitive impairment (MCI, [9–11]).
In addition to their promise in differentiating normal aging from early stages of AD-related cognitive decline, fMRI studies of mnemonic function can be used as biomarkers in charting disease progress in dementing illnesses and as endpoints in clinical trials [12]. In the present review, we will outline recent research examining the nature of AD-associated alterations in activation and deactivation patterns during the performance of associative memory tasks. We will also discuss in detail work using task-free or resting state MRI to delineate neural networks whose function is altered with age, risk of AD, and progression to clinically-evident AD.
fMRI Techniques: A Very Brief Introduction
Functional MRI (fMRI) provides a non-invasive, in vivo technique to investigate the neural underpinnings of higher cognitive functions by measuring regional hemodynamic changes, which are, in turn, thought to be closely linked to underlying cellular activity [13,14]. In its most widely-used form, fMRI is used to infer regional increases in activity based on increases in the blood-oxygen-level-dependent (BOLD) contrast, while decreases in the BOLD signal indicate deactivation (e.g., [14]). More specifically, BOLD signal changes detected by fMRI are thought to represent integrated synaptic activity by measuring changes in blood flow, blood volume, and the blood oxyhemoglobin/deoxyhemoglobin ratio underlying such synaptic activity [15].
Task-related fMRI experiments compare the MR signal during one cognitive condition (e.g., memory encoding of novel stimuli) to a control task (e.g., viewing familiar stimuli) or to a passive baseline condition (e.g., visual fixation). This can be done in “block design” paradigms, in which stimuli of each cognitive condition are grouped together in blocks lasting 20–40 s, or in “event-related” paradigms, in which single stimuli from several different conditions are interspersed. In either case, analysis of the fMRI-derived BOLD signal are performed respecting the dynamics of the hemodynamic response itself, with a peak response typically observed 4–6 s after the stimulus onset.
In addition to task-related activations, analysis of the BOLD signal also allows for assessment of task-related decrements in activity, or “deactivations,” manifest by decreases in the BOLD response below the normal baseline observed [13,14]. Though often spatially adjacent, regions showing activations and deactivations are easily segregated from each other using conventional MRI processing techniques.
The pattern of activated and deactivated brain regions modeled in block or event-related design paradigms allows for the identification of brain regions and networks whose activity is modulated by the experimental task. These activation and deactivation patterns can be compared to performance in memory tasks, allowing a window into altered neuronal function in successful vs. unsuccessful memory encoding trials. By creating contrasts between disparate behavioral or cognitive conditions (e.g., fixation vs. memory encoding), the dynamic nature of the BOLD signal coupled with the relatively static hemodynamic response to activity allows for the inference of brain regions which are selectively activated or inactivated by a task – i.e, “task-positive” or “task-negative” brain regions [16].
Enriching our understanding of task-positive and task-negative brain networks, are so-called “task-free,” or “resting state,” or functional connectivity MRI (fcMRI) studies, in which statistical correlations in BOLD signal dynamics while the brain is at rest have allowed for the identification of several large-scale neural networks composed of widely anatomically separated brain regions (e.g., [16,17]). Typically, task-free or resting-state studies examine correlations in BOLD signal while subjects undergo short (typically 4–6 minute) functional MRI scans. Though there is some variability in the instructions given at the beginning of such scans, the majority of resting-state studies simply ask subjects to stay awake with either eyes open or closed, sometimes with the added instruction to keep their gaze on a fixation cross. Importantly, the large-scale networks that have been observed in resting state studies (e.g., Figure 1A) are also observed in task-based fMRI, and in many cases were first identified using task-based imaging designs.
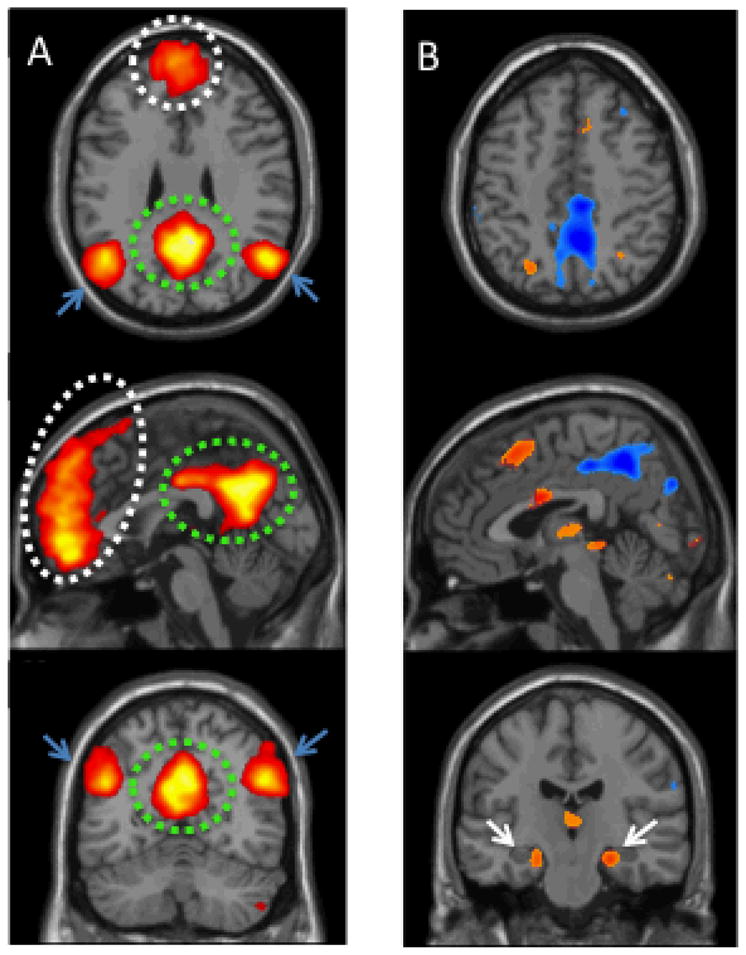
(A) 31 young, normal subjects underwent resting state fMRI. Independent component analysis (ICA) of this data yielded a network component representative of the DMN, including the posterior cingulate/precuneus (green circle), medial prefrontal cortex (white circle), and regions within the lateral parietal lobes (blue arrows). (map threshold p <0.0001); (B) The DMN node of the precuneus shows decreased activity during the performance of novel face-name association encoding task, whereas medial temporal lobe (including the hippocampus; white arrows) show increased activity. (map threshold p<.0005; using novel vs. repeated face-name presentations as a contrast, decreasing activity noted in blue, increasing activity in red. Schultz, Chhatwal, Sperling, unpublished data).
As we will discuss in more detail, the connectivity between the regions comprising the DMN is progressively diminished in subjects with MCI and AD dementia (e.g., [18]) as well as by advancing age [19–22] and with increasing amyloid deposition [3,7,22,23]. While these functional connectivity changes have been most well-studied in the DMN, similar changes are also seen within several other large scale, functionally connected networks [24].
Memory Circuits Underlying Memory Formation
Based on a wealth of human and animal literature, evidence strongly supports the hypothesis that memory function relies on anatomically distributed networks of brain regions, both cortically and subcortically. A variety of functional MRI memory tasks have been employed to probe specific components of memory function in humans. The details of these task paradigms are beyond the scope of this review, but in general, memory tasks can be classified as episodic, semantic, or working memory paradigms. Within episodic memory, specific tasks have been used to probe encoding versus retrieval processes, most often focusing on associative or relational memory processing. In particular, connections between the hippocampi and parahippocampal structures with cortical regions in the medial temporal lobes (MTL), appear to be crucial in the process of binding novel associations into cohesive episodic memory traces and in forming new associations between pieces of previously unrelated information [25–27]. fMRI studies in both young and older subjects have demonstrated that associative memory paradigms produce robust activation of the anterior hippocampal formation [28,29]. In addition to simply being activated or “turned on” during learning processes, the BOLD response of the MTL is modulated according to the novelty and salience of the mnemonic association being presented. In accordance with this idea, the robust MTL activation observed with novel stimulus presentations is decreased with repeated presentations of the stimuli, suggesting that the extent of MTL activation is related to novelty of the association being learned [30–33]. An illustration of MTL activation during a novel encoding task (in this case a face-name associative encoding task) from our own data is shown in Figure 1B.
Beyond the activation of the MTL, recent fMRI studies also suggest that successful memory encoding requires that other brain regions be actively disengaged during task performance. Regions within the DMN in particular, have been identified as critically important in this manner [34,35]. Recent work from our group and others suggests that the extent to which DMN deactivation takes place correlates with the success of the encoding trial itself [35–37].
The DMN includes the posterior cingulate extending into the precuneus, lateral parietal, and medial prefrontal regions (Figure 1A). Both by 18F-fludeoxyglucose positron emission tomography (FDG-PET) and fMRI, it has been shown that the DMN is more metabolically active at rest and shows decreases in activity during the performance cognitively demanding tasks, such as associative memory tasks ([16,38]; Figure 1B). In this respect, the DMN can be considered the archetype of a “task-negative” network for associative memory, or a network in which a decrease in activity is appropriate for the successful performance of the task. Other networks, composed of brain regions activated during associative memory encoding (“task-positive” networks) including the dorsal attention-system or salience network, have also been identified, though they have been less well studied (e.g., [39,40,40]).
Intriguingly, while many of these networks were first recognized in task-based fMRI studies, the correlated patterns of activity that define task-positive and task-negative networks are also observable in the absence of a specific task. This, in turn, suggests that the networks seen in task and task-free MRI represent a part of the underlying organization of the brain [15,17,41]. As we will discuss, diminished functional connectivity (measured by functional connectivity MRI, or fcMRI) between regions of the task-negative network, in particular, correlate with diminished mnemonic function in AD.
It remains unclear whether the hippocampus and related structures in the MTL should be considered as part of the DMN. While structures in the MTL are often functionally connected with the DMN at rest [42], the successful performance of associative memory tasks leads to task-appropriate, uncoupled activity within the MTL and the DMN. Successful associative encoding has generally been linked to increased MTL activity and profoundly decreased activity within the “task-negative” DMN (e.g., [34,37], Figure 1B) and that this normal uncoupling between MTL and DMN during encoding tasks may be disrupted with age and increasing amyloid burden [22,35]. Additionally, while we will focus our attention on the role of the DMN in associative memory tasks, it should be noted that many non self-referential, attention-demanding tasks lead to decreased DMN activity [15], and that DMN deactivation is not unique to memory tasks.
fMRI ALTERATIONS IN NORMAL AGING
Owing to their dependence on the medial temporal lobe memory system, associative memory tasks are particularly useful in detecting subtle memory impairments in both normal aging and in dementia [43–45]. Though there are a variety of MTL-dependent associative encoding tasks available, our group has largely focused on tasks where subjects learn the association between a novel face and name. This choice of paradigm owes to the relative difficulty of this task, its cross-modal nature, and the high frequency with which patients complain of remembering faces and names when presenting to memory disorder clinics.
Our initial studies in aging utilized a “block design” fMRI paradigm, comparing fMRI activation during the encoding of novel face–name pairs to the viewing of repeatedly presented face–name pairs. The face–name pairs used were drawn from a large library (>500) faces across a wide range of ages, and varied in gender and ethnicity. Each face is paired with a fictional name, taken from Internet lists of the most popular names from each decade, with test subjects prompted to learn the association between the face and name.
In this manner, we compared young subjects to older (cognitively-normal) subjects on the learning of a face-name pairs, some of which were shown only once during the encoding phase, and some of which were shown multiple times during the encoding phase [46]. We found that young and older subjects showed an overall similar pattern of fMRI activation for the novel faces, as compared to repeatedly presented faces. Specifically, both young and older subjects activated the anterior hippocampal formation during novel encoding, although the older group’s hippocampal activation was more limited in extent. Comparing trials where the face presented was novel and trials where the face had been seen before, young and older controls differed primarily in the pattern of prefrontal and parietal cortex activation. Older subjects showed less prefrontal and greater parietal activation than young when seeing novel versus repeated stimuli. Interestingly, this difference was driven by continued prefrontal activation to repeated stimuli in the older controls, suggesting a deficit in the ability to appropriately decrease activity in these areas after repeated presentations of the same stimulus (we refer to this phenomenon as “repetition suppression”).
To follow up on this finding and to improve encoding success in older subjects, we next utilized an event-related fMRI paradigm where all of the stimuli were repeated multiple times during the encoding phase [32]. We found that during the initial encoding of successfully encoded face–name pairs (those face-name pairs that subjects recalled with high confidence in later testing), older subjects showed robust anterior hippocampal activation, similar to the pattern we had observed in young subjects with single encoding trials [47]. However, while older subjects did suppress activity in the hippocampus when face-name pairs were repeated, the prefrontal cortex showed continued activation above baseline even on the third encoding trial (i.e., a deficit of repetition suppression). These results suggest that while repeated exposures led to greater retention in older individuals, the memory benefits of repeated stimulus exposure in normal aging did not involve an increase in activation of the medial temporal lobe, but a failure of repetition suppression in the prefrontal cortex.
To further dissect the role of MTL activation in successful face-name associative memory, we next compared young and older subjects during associative encoding with a large number of face–name pairs, each shown only once [35]. Our results demonstrated that while older subjects performed more poorly overall than younger subjects on this difficult task, both younger and older subjects showed significantly greater hippocampal activation during the encoding of face–name pairs that were successfully remembered as compared to forgotten pairs. We found a similar extent and magnitude of activation in the hippocampus comparing young and older controls. This suggests that in both younger and non-demented older subjects, hippocampal activation during associative encoding is predictive of successful memory encoding, but also demonstrates that the magnitude of hippocampal activation between young and old subjects is not different.
As part of this same series of studies, we also examined areas of the brain that showed task-related deactivations during successful encoding trials. We found that young subjects showed a very significant deactivation in the precuneus and posterior cingulate (PPC) during successful encoding compared to forgotten stimuli, and that this PPC deactivation was statistically similar to results seen in older subjects, when we included both high and low-performing older subjects in the same group. However, when we subdivided the older group on the basis of their post-scan test performance, we found that the high-performing older subjects showed greater deactivation in the PPC than did lower-performing older subjects, hinting that the magnitude of task-appropriate PPC deactivation correlates with associative memory performance.
Our experiments in cognitively intact older control subjects demonstrate that cognitively normal older subjects are able to activate their hippocampus during successful associative encoding [35,47–49]. Similar results have been observed by other groups, both during memory encoding and retrieval [50,51]. Taken together, the data using face-name associations suggests that age- related decrements in memory performance may be due more to alterations in the activity patterns in cortical regions or in the connections between the medial temporal lobe and neocortical regions, rather than by an inability to activate the hippocampus during associative encoding.
This fits well with studies from numerous other groups suggesting that activity in temporoparietal (medial and inferior) and posterior midline cortical regions (especially in the PPC), is suppressed during successful memory encoding, and that task-appropriate activation of the MTL during successful encoding may reciprocally depend on the temporally appropriate deactivation of the task-negative DMN [18,52,53]. Though more work remains to be done, the deactivation of the DMN observed during such experiments have been interpreted to represent the reallocation of neural resources needed for efficient cognitive processing and successful task performance. With respect to normal aging, the failure to suppress activation in regions of the DMN, particularly the posteromedial cortices, has been associated with failed encoding and subsequently poor memory performance [35].
To specifically address the relationship between task-appropriate deactivation of the DMN and successful encoding, our group designed a series of studies to examine the deactivation of the DMN as a function of aging and amyloid status in cognitively normal, young and elderly individuals [22,37]. First, we compared 26 young subjects to 41 cognitively normal older individuals on a task in which subjects were asked to remember face-name pairs which were repeated multiple times, generating a session in which novel encoding, encoding of repeated stimuli, and retrieval could be separated in the analysis. These studies demonstrated similar patterns of bilateral activation in the hippocampus were present in young and older subjects, again suggesting that changes in hippocampal activation per se does not account for poorer associative memory performance in older adults.
However, when we turned our attention to the DMN, we observed significant alterations in activity. Specifically, we observed that younger subjects efficiently deactivated the PPC, a central node within the DMN, during successful encoding trials. Furthermore, the magnitude of both the hippocampal activation and the de-activation of the PPC decreased with repeated presentations of the same face/name pair in both young and low-amyloid burden elderly subjects [22,49,54]. However, when we separated out older subjects with high amyloid burden, we found that this high-amyloid burden group was less efficient at deactivating the PPC, suggesting a link between the amyloid deposition and a failure of task-appropriate deactivations of the DMN [22].
Our findings are consistent with previous studies in young [46,55] and healthy older subjects [49]. Additionally, though there have been several studies in which decreased hippocampal activation is seen when comparing older and younger subjects (see [56] for a review), many of these studies included all encoding trials, rather than analyzing only those in which the subject demonstrates that they have correctly remembered the association. Synthesizing these results with those of prior studies indicates that both MTL activation and DMN deactivation are key features of successful associative encoding.
In turn, these results implicate the failure of DMN deactivation in older subjects as a central feature of decreased memory performance seen in normal aging. Mechanistically, this also suggests that a choreographed interplay between task-positive and task-negative networks is needed to engage the neural substrates of associative memory, and that both the task-positive and task-negative networks alter their activity to novel vs. repeated stimuli.
Task-free MRI in Normal Aging
Elegant recent work has demonstrated that many of the same neural networks (defined by coordinated activity during the performance of tasks) are observable even in the absence of a task to constrain and direct neural activity (e.g., [17]). By analyzing the temporal characteristics of spontaneous fluctuations of the BOLD signal (in the absence of a task to direct neural activity) maps of brain regions showing coordinated patterns of activity can be derived. By inference and comparison to task-based MRI studies, we can observe many of the same task-positive and task-negative networks even in the absence of a specific task. Analysis of spontaneous BOLD fluctuations of this sort has been referred to as task-free, resting state, functional connectivity MRI (fcMRI), and a number of other terms.
Using fcMRI as a tool to define the formation of large scale neural networks in the human brain, it appears that the DMN develops in adolescence [57], though it is readily observed in age groups ranging from the teens to the very-old [19,57]. Using the fcMRI technique, it has repeatedly been shown that the level of connectedness between different anatomically distant regions comprising the DMN is compromised with normal aging, in MCI, and can be quite advanced in AD. This data coupled with compatible diffusion-tensor imaging data [58], has led to the hypothesis that a decreased functional and structural connectivity in large-scale neural networks such as the DMN may underpin the diminished mnemonic function seen in normal aging [19,59].
With respect to normal aging, the few studies that have examined resting state connectivity in very advanced age have suggested that decreased functional connectivity is seen with increased age, regardless of cognitive status. Buckner and colleagues have elegantly demonstrated that both the PPC and ventromedial frontal cortex (central nodes of the posterior and anterior segments of the DMN, respectively) showed decreased intrinsic connectivity with the other regions of the DMN in older subjects [19]. These same authors also demonstrated that functional connectivity between the anterior and posterior nodes of the DMN was significantly associated with memory performance on neuropsychologic testing, as well as with other measures of cognitive and executive function.
Task and Task-free fMRI in those at high risk for AD dementia
Very recently, attention has turned to determining whether changes in DMN connectivity are observable in subjects who are cognitively normal, but who have risk factors that put them at increased risk of developing AD. Thus far, populations with high levels of fibrillar amyloid burden and carriers of the apolipoprotein E (APOE ε4) allele have been studied.
Hedden, Buckner and colleagues recently demonstrated that cognitively-normal subjects with high fibrillar amyloid burden (as measured by Pittsburgh Compound B PET imaging) have decreased functional connectivity within the DMN [7], again hinting at a link between amyloid deposition and the strength of the functional connections between the nodes of the DMN. Decreased DMN functional connectivity in subjects with high amyloid burden was also observed by Sheline, Mintun and colleagues, whose data also demonstrate decreased connectivity between the MTL and PPC in subjects with a high amyloid burden [3,60].
Decreases in posterior DMN connectivity were also observed in a large study of APOE ε4 carriers [8], though amyloid status was not assessed. These same investigators observed that connectivity within another large-scale network (which is generally considered a task-positive network), the salience network, was actually increased in ApoEε4 carriers as compared to controls, suggesting that universally decreased connectivity is not present in the ApoEε4 carrier state.
The central question of whether increased amyloid burden itself (in the absence of cognitive decline) can contribute to the decreased connectivity in large-scale neural networks is being intensely studied. Studies from our group and others have demonstrated that connectivity between the PPC and several key regions in the default mode and other large-scale networks is reduced even in cognitively-normal persons with high burden of fibrillar amyloid [7,60]. These results fit well with the observation that subtly reduced whole-brain connectivity with the PPC is observable even in asymptomatic carriers of high amyloid burden, and that this decreased connectivity is more striking in MCI [61]. Furthermore, the presence of high amyloid burden may itself alter the balance between task-negative and task-positive networks during memory encoding [22,60].
Task-based fMRI Alterations in Mild Cognitive Impairment
As the emphasis on early diagnosis of AD has grown, so has the effort to better characterize the earliest stages of cognitive decline using fMRI. Work from our group suggests that older subjects with very early mild cognitive impairment (MCI) show evidence of increased MTL activation during task performance, and that the extent and location of hyperactivation is related to memory task demands and memory performance [48,62]. We hypothesize that MTL hyperactivation in very early stages of MCI may be a compensatory response to maintain memory performance in the setting of early AD pathology.
This period of MTL hyperactivation during associative memory tasks [63] appears to be followed by the failure of task-appropriate activation in the MTL in more impaired MCI subjects [49,54]. We found that less impaired MCI subjects [64] showed greater hippocampal activation compared to older controls, whereas more-impaired MCI subjects showed very little hippocampal activation, similar to AD subjects [49]. Longitudinal studies suggest that the decrease in hippocampal activation seen in MCI appears to progress over time [65].
Together, these results suggests that there is a nonlinear trajectory of memory-related fMRI activity over the course of MCI and AD, with an initial phase of hippocampal hyperactivation during encoding in pre-symptomatic and very mildly impaired subjects, followed by decreased activation with progression of pathology and memory impairment. Similar findings were seen by another research group in more recent, longitudinal studies [66,67]. Notably, this pattern contrasts with the relative stability of hippocampal activation seen with normal aging.
On a network level, there is evidence that subjects with MCI (as compared to those with normal aging) showed a decreased ability to deactivate the DMN during the performance of associative memory tasks [68]. Furthermore, studies from our group have demonstrated that a strong, reciprocal relationship between DMN deactivation and hippocampal activation is seen in cognitively-normal older adults during the performance of a face-name associative memory task, and that this reciprocal pattern breaks down in MCI and AD [49].
In this way, task fMRI has shown evidence of specific alterations in memory networks that may be able to differentiate the process of normal aging from early, preclinical and prodromal stages of AD. Further work is clearly needed to understand the relationship of these alterations to amyloid pathology and to more precisely determine what point in the disease process MTL hyper-activation and failure of DMN deactivation occurs. Future studies that combine fMRI with amyloid PET imaging, as well as volumetric MRI should prove particularly valuable in detecting the earliest pathological alterations.
Resting State Studies in MCI
The use of resting state analyses to identify alterations in functional connectivity that distinguish normal aging from MCI and AD have gained momentum in recent years, though relatively few studies have thus far been completed. Several recent studies indicate that changes in the functional, intrinsic connectivity in task-positive and task-negative networks occurs early in the progression from normal aging to MCI, and suggests that these changes may eventually be useful in identifying individuals at higher risk of progression to AD.
Specifically, several groups have now reported that finding of decreased connectivity within the posterior DMN (especially the PPC) is seen in subjects with amnestic MCI as compared to controls [9–11]. Additionally, these same studies have demonstrated that there is decreased connectivity seen between the MTL and the PPC, suggesting that the degraded interplay between the MTL memory system and the task-negative DMN may underlie early mnemonic dysfunction.
Importantly, this sort of decreased connectivity within the posterior DMN appears to be present even prior to significant atrophy of the involved regions, raising the possibility that pathologic inefficiencies in connectivity between the MTL and the posterior DMN may be very early events in those slated to progress to MCI and AD dementia. As such, the altered functional connectivity in the posterior DMN could potentially be used to identify individuals at high risk of progressing to AD prior to the irreversible neuronal loss and atrophy seen as individuals progress to advanced MCI and AD (e.g., [9,66,67]). Furthermore, as aberrant connectivity is better defined, it can be combined with other imaging, biochemical, and clinical biomarkers to identify at-risk populations in whom early treatments may be the most effective at preventing progression to AD dementia.
fMRI and alterations in MTL and DMN function in AD
Unlike the preserved activation of the MTL seen with normal aging, numerous fMRI studies in patients with clinically diagnosed AD dementia have reported decreased fMRI activation in MTL during memory encoding tasks [46,69–75]. Though we will not delve into the specific characteristics of each task in the present discussion, a wide variety of mnemonic tasks (using diverse stimuli including, faces [68,75,76], face-name pairs [46], scenes [69], line-drawings [71], geometric shapes [72]) have shown decreases in hippocampal and/or parahippocampal activity in AD patients compared to healthy older controls during the encoding of novel stimuli.
In line with Johnson and colleagues [77] our recent work demonstrates that the normal suppression of MTL activity seen when face-name stimuli are repeated (repetition suppression) is significantly impaired in AD [33,78]. These data suggest that intact MTL repetition suppression may be part of the successful encoding and early consolidation process, and that failure of MTL repetition suppression is a potential indicator of impaired MTL and memory function early in the course of AD [79]. A recent meta-analysis also reported that AD patients showed greater encoding-related activation in ventrolateral prefrontal, orbitofrontal, dorsolateral prefrontal, superior temporal, and fusiform regions, compared to controls [80]. Specifically, regions involved in the so-called “cognitive control network” (including the dorsolateral prefrontal, posterolateral parietal, anterior cingulate, frontoinsular cortex) were also less engaged in AD compared to controls, indicating the contribution of cortical network dysfunction contributes to impaired mnemonic function in AD [80].
Decreases in activation in the hippocampus and cortical regions are accompanied by evidence of increased neural activity in other regions, hinting at a more complex underlying pattern of network level dysregulation in AD, rather than simply a universal reduction in network activity. In particular, increased activity prefrontal cortical regions has been observed in AD patients performing memory tasks [46,80,81]. Though the interpretation of these increased prefrontal activations is still quite debatable (and is not seen in all encoding paradigms described – see [71,74]), it has been suggested that increased prefrontal activation may represent a compensatory effort of the degraded memory system to use alternative strategies to complete cognitive tasks.
From a network perspective, Lustig, Andrews-Hanna, Buckner and colleagues have suggested that a failure of the DMN deactivation may be a crucial piece in understanding the degradation of memory in aging and dementia [19,82]. They observed that the patterns of deactivation seen in the nodes of the DMN varied with both aging and the development of dementia. Roughly equal deactivation in lateral parietal regions was observed with age, but no further decrease was seen in subjects with AD. This contrasted to the pattern seen in the PPC, where significant differences between young adults and older adults without dementia were observed, coupled with a more profound loss of task-appropriate deactivation in subjects with AD [82].
Resting state studies in AD
A major difficulty in interpreting resting state studies in AD, especially as it becomes more advanced, is the widespread atrophy in many brain regions, and especially within the DMN. This change in structure is accompanied (and likely preceded) by decreases in brain glucose metabolism in these same regions [2,83] even early on in the course of AD [84]. Additionally, recent data suggest that decreases in functional connectivity in the PPC node of the DMN precede the profound gray matter atrophy seen in the PPC in AD [85], though it seems that the medial temporal lobes show even earlier atrophy within the AD disease course [85,86]. Complicating matters, there have been reports in which decreased DMN fcMRI in AD has not been readily observed, though this may well be due to methodological differences and the inclusion of subjects with advanced AD (in whom the resultant atrophy complicates fcMRI analysis). In one recent report of this type, Kenny and colleagues report that using a non-standard seed region within the precuneus, they were unable to demonstrate reduced precuneus connectivity with other portions of the brain in a small cohort of subjects with advanced AD [87]. However, despite these complexities, the present data demonstrate a convincing pattern of progressively decreasing functional connectivity within the DMN in AD that is more profound than that seen in normal aging (for an excellent recent review see [88]).
Specifically, comparing elderly subjects with and without dementia, reduced connectivity with the hippocampus and the PPC node of the DMN was seen in subjects with early AD as compared to elderly controls without dementia [18]. Focusing on individuals early in the course of AD (ostensibly those with the smallest amounts of atrophy), a variety of groups have shown decreases in the connectivity of the hippocampus to the mPFC and PPC nodes of the DMN [89], of the PCC to the rest of the DMN [18,90], and an impaired balance between task-positive and task-negative networks [91].
Though the DMN has been the most heavily studied large-scale network in AD, several studies have examined AD-related changes in the functional connectivity of other networks [24], and on the overall functional architecture of the brain as a whole [91–93]. Most germane to this discussion, Brier, Ances and colleagues [24] describe decreasing connectivity within the DMN, salience, dorsal attention, sensory-motor, and control networks in subjects with mild AD (CDR of 1.0). Intriguingly, these same authors note that the interplay between these large-scale networks may also be disrupted in AD, as evidenced by decreased internetwork correlations in subjects with AD as compared to controls.
However, despite the widespread nature of these connectivity changes, there may be discernible underlying patterns that are indicative of AD. For instance, differing patterns of atrophy are seen in AD as compared to frontotemporal dementia [94,95] and other neurodegenerative diseases [96], suggesting that distinctive patterns of altered fcMRI may be identifiable in early and moderate AD. Additionally, recent evidence suggests that focusing on the interplay between the DMN and other large scale networks (especially those networks that are usually anti-correlated with the DMN) may yield the most specific insights into AD-related network dysfunction [24]. By expanding on these concepts, the hope is that the pattern of changing fcMRI can be used in concert with other imaging and CSF biomarkers [97] to differentiate dementing illnesses [87,92,98]. Conceptually, this notion fits well with the elegant studies of Seeley and colleagues, suggesting that a signature pattern of decreased metabolism, atrophy, and degradation of network connectivity may underlie the dysfunction of specific neural circuits in neurodegenerative dementias [96].
Concluding Remarks
The use of fMRI in examining mnemonic function in normal aging, preclinical AD, MCI, and AD dementia has helped define critical nodes supporting successful associative encoding. These individual nodes form anatomically distributed neural networks that demonstrate specific modulation of activity during encoding and retrieval. The carefully choreographed interplay of the activity in task-negative and task-positive networks appears to be a critical feature in successful memory formation, and this process appears to be impaired in AD dementia, even at early stages.
The DMN and other large-scale, anatomically distributed networks are easily observed at rest, suggesting that their presence represents not only a critical component of successful performance during cognitive tasks, but also a fundamental aspect of the functional organization of the brain. Similar to the failure of network deactivation seen in task-based studies of individuals with AD, the functional connectivity of these large scale, resting-state networks is progressively diminished in the transition from normal aging to MCI, and from MCI to early and late AD.
The robust changes seen in functional imaging studies of normal aging, MCI, and AD help to elucidate the underlying neural architecture of human memory and provide the field with a potentially sensitive, non-invasive biomarker that is closely linked to the neural machinery of memory. Going forward, the hope is that these promising fMRI techniques can be used to complement more traditional CSF and serum biomarkers to improve our understanding of early memory failure, facilitate earlier diagnosis and earlier intervention with experimental therapeutics in AD.
Acknowledgments
Funding: NIA K24 AG035007 (Reisa Sperling PI)
The authors wish to thank Dr. Aaron Schultz for his assistance with the included figure and for helpful discussions regarding this manuscript.
Footnotes
The authors declare no conflicts of interest.
Disclosures: Dr. Chhatwal has nothing to disclose. Dr. Sperling has served as a consultant and/or site investigator for Bayer, Bristol-Myers-Squibb, Eisai, Janssen, Kyowa Hakko Kirin, Merck, Pfizer, Roche, and Satori, and has received funding support from Pfizer, BMS Trials, and Janssen.
References
Full text links
Read article at publisher's site: https://doi.org/10.3233/jad-2012-120730
Read article for free, from open access legal sources, via Unpaywall:
https://europepmc.org/articles/pmc3736339?pdf=render
Citations & impact
Impact metrics
Citations of article over time
Article citations
The hemodynamic response function as a type 2 diabetes biomarker: a data-driven approach.
Front Neuroinform, 17:1321178, 05 Jan 2024
Cited by: 0 articles | PMID: 38250018 | PMCID: PMC10796780
Changes in hemodynamic response function components reveal specific changes in neurovascular coupling in type 2 diabetes.
Front Physiol, 13:1101470, 10 Jan 2023
Cited by: 6 articles | PMID: 36703928 | PMCID: PMC9872943
The effect of combination pretreatment of donepezil and environmental enrichment on memory deficits in amyloid-beta-induced Alzheimer-like rat model.
Biochem Biophys Rep, 32:101392, 23 Nov 2022
Cited by: 2 articles | PMID: 36438603 | PMCID: PMC9694055
Alzheimer's disease amyloid-β pathology in the lens of the eye.
Exp Eye Res, 221:108974, 21 Feb 2022
Cited by: 8 articles | PMID: 35202705 | PMCID: PMC9873124
It Is Time to Study Overlapping Molecular and Circuit Pathophysiologies in Alzheimer's and Lewy Body Disease Spectra.
Front Syst Neurosci, 15:777706, 18 Nov 2021
Cited by: 4 articles | PMID: 34867224 | PMCID: PMC8637125
Review Free full text in Europe PMC
Go to all (38) article citations
Similar Articles
To arrive at the top five similar articles we use a word-weighted algorithm to compare words from the Title and Abstract of each citation.
Differential medial temporal lobe and default-mode network functional connectivity and morphometric changes in Alzheimer's disease.
Neuroimage Clin, 23:101860, 18 May 2019
Cited by: 16 articles | PMID: 31158694 | PMCID: PMC6545401
Anterior and posterior MTL networks in aging and MCI.
Neurobiol Aging, 36 Suppl 1:S141-50, S150.e1, 28 Sep 2014
Cited by: 31 articles | PMID: 25444600 | PMCID: PMC4342050
Basal functional connectivity within the anterior temporal network is associated with performance on declarative memory tasks.
Neuroimage, 58(2):687-697, 21 Jun 2011
Cited by: 53 articles | PMID: 21722740
Functional MRI studies of associative encoding in normal aging, mild cognitive impairment, and Alzheimer's disease.
Ann N Y Acad Sci, 1097:146-155, 01 Feb 2007
Cited by: 139 articles | PMID: 17413017
Review