Abstract
Free full text

Endothelial Heparan Sulfate 6-O-Sulfation Levels Regulate Angiogenic Responses of Endothelial Cells to Fibroblast Growth Factor 2 and Vascular Endothelial Growth Factor*
Abstract
Fibroblast growth factor 2 (FGF2) and vascular endothelial growth factor 165 (VEGF165) are potent pro-angiogenic growth factors that play a pivotal role in tumor angiogenesis. The activity of these growth factors is regulated by heparan sulfate (HS), which is essential for the formation of FGF2/FGF receptor (FGFR) and VEGF165/VEGF receptor signaling complexes. However, the structural characteristics of HS that determine activation or inhibition of such complexes are only partially defined. Here we show that ovarian tumor endothelium displays high levels of HS sequences that harbor glucosamine 6-O-sulfates when compared with normal ovarian vasculature where these sequences are also detected in perivascular area. Reduced HS 6-O-sulfotransferase 1 (HS6ST-1) or 6-O-sulfotransferase 2 (HS6ST-2) expression in endothelial cells impacts upon the prevalence of HS 6-O-sulfate moieties in HS sequences, which consist of repeating short, highly sulfated S domains interspersed by transitional N-acetylated/N-sulfated domains. 1–40% reduction in 6-O-sulfates significantly compromises FGF2- and VEGF165-induced endothelial cell sprouting and tube formation in vitro and FGF2-dependent angiogenesis in vivo. Moreover, HS on wild-type neighboring endothelial or smooth muscle cells fails to restore endothelial cell sprouting and tube formation. The affinity of FGF2 for HS with reduced 6-O-sulfation is preserved, although FGFR1 activation is inhibited correlating with reduced receptor internalization. These data show that 6-O-sulfate moieties in endothelial HS are of major importance in regulating FGF2- and VEGF165-dependent endothelial cell functions in vitro and in vivo and highlight HS6ST-1 and HS6ST-2 as potential targets of novel antiangiogenic agents.
Introduction
The activity of fibroblast growth factor 2 (FGF2)3 and VEGF165, which are potent pro-angiogenic growth factors, is regulated by HS, a component of proteoglycans. VEGF is overexpressed in various types of human cancers (1–4), whereas FGF2 has recently emerged as one of the factors implicated in the resistance to antiangiogenic therapies targeting VEGF/VEGF receptor pathway (5, 6). Previously we demonstrated that ovarian cancer endothelium expresses HS that has the capacity to assemble the FGF2/FGFR/HS signaling complex (7), thus highlighting the importance of HS structural properties in determining the formation and activity of trimolecular signaling complexes in tumor endothelium. HS is composed of alternating disaccharide units consisting of hexuronic acid linked to N-substituted glucosamine (8). After initial synthesis, HS modifications occur, including C5-epimerization of glucuronic acid into iduronic acid and variable sulfation at the 2-O-position of iduronate and 6-O-, 3-O-, and N-positions in glucosamine (8). Regions with high sulfate density (S domains) interact with a number of cytokines, including FGFs and VEGF165 (8, 9). Such regions are flanked by poorly sulfated sequences containing N-acetylated/N-sulfated glucosamine (transitional T-domains) and are separated by non-sulfated N-acetylated domains (NA domains) (8).
Regulation of 6-O-sulfate (6S) content in HS chain depends on HS6STs that catalyze 6-O-sulfation on N-sulfated or N-acetylated glucosamine and extracellular 6-O-endosulfatases, which desulfate at 6S (10–12). 6-O-Endosulfatases are dysregulated in many tumor types, including ovarian, breast, pancreatic, and hepatocellular carcinomas (13, 14). Re-expression of exogenous human 6-O-endosulfatase-1 leads to reduced growth of breast carcinoma xenograft tumors that also display lower microvessel density (15). Glucosamine 6-O-sulfation is also required in mouse embryonic development. For example, HS6ST-1-deficient mice show abnormal organ morphogenesis and aberrant placental angiogenesis where microvessel density is reduced to about 60% (16).
The outstanding question in the field is to what extent specific HS sulfation patterns are needed for cytokine activation. Several studies suggest that specific sulfation states are required for the binding and activity of FGF2. For example, FGF2 binds preferentially to HS that contains iduronate-2-O-sulfation and glucosamine-N-sulfate, whereas 6-O-sulfation is essential for the formation of the FGF2/FGFR/HS signaling complex and its mitogenic activity (17–19). Moreover, we previously showed that a synthetic structurally-defined 2-O- and N-sulfated dodecasaccharide (12-mer 2SNS) inhibited FGF2-dependent endothelial cell functions and signaling (20). In contrast, VEGF dimer formation requires highly sulfated HS domains, where sulfates at 2-O-, 6-O-, and N-positions significantly contribute to VEGF binding (21).
In this study we demonstrate that HS epitopes involving 6S are expressed at markedly higher levels in ovarian tumor endothelium than in normal ovarian vasculature. We also report that HS structures with reduced 6S in endothelial cells with down-regulated expression of HS6ST-1 and HS6ST-2 negatively affect FGF2- and VEGF165-dependent endothelial phenotypes in vitro and in vivo.
EXPERIMENTAL PROCEDURES
Cell Culture
HUVECs and PaSMCs were purchased from Lonza and cultured in EBM-2 medium supplemented with SingleQuot growth supplements (Lonza) up to passage 7 and SmBM medium supplemented with SmGM-2 SingleQuot kit supplements (Lonza) up to passage 15, respectively. Expression of endothelial cell markers CD31, CD146, and CD105 in HUVECs was confirmed by FACS analysis. Expression of smooth muscle actin α in PaSMCs was tested by immunofluorescence staining.
Immunofluorescence Staining of Ovarian Tumor and Normal Ovarian Tissue
Frozen tissue sections (7 μm) were fixed in acetone for 10 min, washed, blocked in 10% goat serum, and incubated overnight at 4 °C with HS sulfation-specific antibodies diluted 1: 25. Sections were washed, incubated for 1 h with an anti-Vesicular Stomatitis Virus Glycoprotein monoclonal antibody (P5D4, Sigma) diluted 1:25 and visualized with AlexaFluor488-conjugated anti-mouse IgG antibody (1:800; Invitrogen). All sections were double-stained with rabbit polyclonal anti-vWf antibody (Dako), which was visualized with AlexaFluor568-conjugated anti-rabbit IgG antibody (1:800; Invitrogen). Sections were mounted with Prolong media (Invitrogen) and analyzed by a Zeiss Axiovert 200M microscope.
HS Disaccharide Analysis
Cells were cultured for 36 h in the respective media supplemented with 10 μCi/ml of [3H]glucosamine hydrochloride. Cellular HS was extracted with 1% Triton X-100, pooled with the conditioned medium, digested overnight with 0.5 mg/ml Pronase (Sigma) at 37 °C, applied to DEAE-Sephacel anionic exchange column, and eluted with a gradient of 0.15 to 0.75 m NaCl. Residual chondroitin/dermatan sulfate was removed by digestion with 50 mIU chondroitinase ABC followed by DEAE-Sephacel chromatography. 3H-Labeled HS was digested to disaccharides using a mixture of 2 mIU of each heparinase I, II, and III (Grampian Enzymes), and disaccharides were applied to a Propac PA1 SAX-HPLC column (4.6 × 250 mm; Dionex) and run on an Agilent 1200 Series HPLC system. Disaccharides were eluted using 45 ml of linear gradients of 0–1 m NaCl (pH 3.5) at a flow rate of 1 ml/min. Fractions were counted on a Wallac 1409 scintillation counter. Disaccharides were identified by comparison to elution positions of non-radiolabeled standards (Iduron) which were detected by in line UV absorption at 232 nm.
Depolymerization of 3H-Labeled HS for Domain Analysis
Metabolically 3H-labeled HS was depolymerized by incubating with 2 mIU of heparinase I or III (Iduron) in 50 μl of digestion buffer (0.1 m sodium acetate, 0.1 mm calcium acetate, pH 7.0) at 37 °C for 4 h after which another 2 mIU was added for 16 h. The K5 lyase digestion was performed using 10 μg/ml K5 lyase (Iduron) in 25 mm Tris acetate (pH 8.5) buffer. HNO2 deaminative cleavage was performed by using the low pH method as described (22). Samples were dried down by centrifugal evaporation, redissolved in 100 μl of 1 m HNO2 solution, and incubated for 15 min at 20 °C. The reaction was stopped by the addition of 10 μl of 1 m Na2CO3, and fragments were resolved on a Bio-Rad P10 size exclusion column (100 × 1 cm) in 0.2 m NH4HCO3 at a flow rate of 4 ml/h. Fractions were counted on a Wallac 1409 scintillation counter.
In Vitro Assays for Endothelial Cells
HUVEC spheroids were prepared as described (23). Spheroids were resuspended in EBM-2 media without FGF2 and VEGF165 and embedded in fibrin gels by mixing spheroids with fibrinogen (2.5 mg/ml; Sigma), aprotinin (0.15 units/ml; Sigma), and thrombin (0.0625 units; Sigma). FGF2 (5 ng/ml; Lonza) or VEGF165 (2.5 ng/ml; Lonza) were added to the media overlaying fibrin gels for 24 h. Phase contrast images were taken using Zeiss Axiovert 200M microscope (Zeiss). Images were analyzed using MetaMorph image analysis software (Molecular Devices). A three-dimensional HUVEC tube formation assay in fibrin gel was performed as described (20).
Co-culture of PaSMCs and HUVECs in a 96-well dish in EBM-2 media was performed as described (24). After 5 days, cells were fixed in 4% paraformaldehyde, permeabilized with 0.25% Triton X-100, blocked with 5% FBS, and incubated with anti-human CD31 antibody (Dako; 1:200) and anti-smooth muscle actin α antibody (Epitomics; 1:200). After washing, primary antibodies were visualized with AlexaFluor488-conjugated anti-mouse or AlexaFluor565-conjugated anti-rabbit secondary antibodies (1:800) and analyzed by fluorescence microscopy at X20 magnification (Solent Scientific).
Preparation of Conditioned Media
HUVECs were seeded in a 6-well plate at a confluence of ~80%. After a day of culturing, cells were washed with PBS, and 0.5 ml of EBM-2 media lacking FGF2, VEGF, and heparin was added to the cells. 48 h later media were collected and centrifuged, and the supernatant was collected. When required, 2 ml of conditioned media was incubated with 10 mIU/ml each of heparinases I, II, and III in 50 μl of heparinase buffer (100 mm sodium acetate and 0.2 mm calcium acetate) at 37 °C for 4 h. A further 10 mIU/ml were added for another 4 h. Enzymes were inactivated by heating to 70 °C for 3 min. Control conditioned medium was incubated with 50 μl of heparinase buffer alone and treated as heparinase-digested media.
Binding of 3H-Labeled Endothelial HS to FGF2 Affinity Columns
To prepare the FGF2 affinity column, 1 ml of activated Affi-Gel 10 (Bio-Rad) was mixed with 1 mg of FGF2 (PreproTech) in 3 ml of coupling buffer (10 mm HEPES, pH 7.0) at 4 °C for 2 h. To prevent cross-linking to Affi-Gel matrix through the glycosaminoglycan binding site, FGF2 was preincubated with 1 mg/ml acetylated porcine mucosal heparin prepared by acetylation of free amine groups as described (25). Coupling was terminated by incubating at room temperature for 1 h with 0.1 m ethanolamine HCl (pH 8.0) and extensive washing with a buffer containing 10 mm Tris-HCl (pH 7.0) and 1 m NaCl, before equilibrating in 10 mm Tris-HCl (pH 7.0) buffer. 3H-Labeled HS was applied to the column, and collected eluate was reapplied four times. The bound material was eluted stepwise with four 1-ml washes of increasing concentrations of NaCl, and 3H content was measured using Wallac 1409 scintillation counter.
FGF2 Binding to Endothelial Cell Surface
Binding of FGF2 to HUVEC surface was determined by using FGF2 biotinylated fluorokine kit (R&D Systems) as described (20).
FGF2 Binding to Ovarian Tumor Sections
Ovarian tumor sections, which were prepared from fresh frozen tumor tissue, were fixed in 4% paraformaldehyde, washed in PBS, treated with 0.05% Pronase for 10 min to expose HS epitopes, and blocked in 5% FBS and 5% nonfat milk. Recombinant human FGF2 (R&D Systems; 50 nm) was added to the tumor sections for 3 h at room temperature. Unbound FGF2 was washed with PBS, and sections were stained with anti-FGF2 (R&D Systems; 1:100), anti-HS (clone 10E4, Seikagaku Corp.; 1:100), and anti-vWf (Dako; 1:100) antibodies. After washing, the sections were incubated sequentially with the respective secondary antibodies. The slides were mounted with Prolong mounting media (Invitrogen), and staining was visualized using Zeiss Axiovert 200M microscope.
Immunoblotting
Cells were plated in 6-well plates at 1 × 105 cells/well in EBM-2 medium lacking SingleQuot growth supplements (Lonza) and supplemented with 0.1% FBS. After 18 h, starvation cells were stimulated with FGF2 for 10, 15, and 30 min. Preparation of cell lysates, immunoblotting, and antibodies are described in Cole et al. 20.
Internalization of FGFR1
HUVECs were starved in EBM-2 media without supplements (Lonza) containing 1% FBS overnight and then incubated with 20 ng/ml FGF2 for 45 min on ice. Cells were washed with PBS and either fixed in 4% paraformaldehyde or transferred to 37 °C for 10 and 30 min before fixation. Cells were permeabilized in 0.1% Triton X-100, blocked in 5% FBS, and incubated with mouse monoclonal anti-FGFR1 antibody (clone 19B2, Millipore; 1:100) overnight at 4 °C. After washes, FGFR1 was visualized with the secondary biotinylated rabbit polyclonal anti-mouse IgG (Dako) diluted 1:400 and Cy3-streptavidin conjugate (Invitrogen) diluted 1:25. Anti-EEA1 antibody (Cell Signaling) diluted 1:100 was used to visualize early endosomes. Microscopy was performed using Zeiss Axiovert 200M microscope at ×40 magnification.
Spheroid-derived Vasculature Formation in Mice
All procedures were performed with local ethical approval under the Home Office Project license PPL 44/3212 granted to Dr. Kaye Williams. HUVEC spheroids each containing 1000 cells were generated and prepared for injections as described (23). FGF2 (RELIATech) was used at 1 μg/ml. Spheroids in Matrigel/fibrin/Methocel mix were injected subcutaneously into the left hand flank adjacent to the hind limb of anesthetized adult female CBA nu/nu mice. The plugs were removed after 21 days fixed overnight in 4% paraformaldehyde and embedded in paraffin blocks. Twenty sections were prepared from each plug. Three sections in the middle and the end of sectioning were selected and used for immunostaining with anti-human CD31 and anti-human CD34 antibodies as described (23). Multiple images were taken with a Zeiss Axiovert 200M microscope to cover the complete area of each section. The number and the size of fluorescent particles was evaluated using ImageJ software. Blood vessel mural cell coverage was visualized by double staining of sections with anti-human CD34 and Cy-3-labeled anti-α-SMA antibodies. For each cell line four plugs were established and analyzed.
Statistical Analysis
Data are expressed as the mean ± S.E. For comparison of groups, the Student's t test was used. A level of p < 0.05 was considered as statistically significant.
RESULTS
Spatial Distribution of HS Epitopes in Ovarian Tumors and Normal Ovarian Tissue
In previous studies we demonstrated that ovarian cancer endothelial and stromal HS had the capacity to assemble the FGF2/FGFR1/HS complex (7). Therefore, we were interested in HS structures characteristic to ovarian tumor endothelium that could potentially mediate responses to HS binding angiogenic factors. We used phage display antibodies that have distinct HS binding affinities depending on specific sulfated motifs in the HS chain (supplemental Table S1) (26, 27) to examine 14 ovarian tumors, mostly of serous histology, for the distribution of sulfation-specific HS epitopes. HS4C3 antibody, which detects N-/6-O-/3-O-sulfated HS, and AO4B08 antibody, which binds to 2-O-/N-/6-O-sulfated motifs, stained tumor tissue much more intensely than EV3C3 and RB4AE12 antibodies that are specific for 2-O-/N-sulfated and N-/6-O-sulfated sequences, respectively (Fig. 1A). The staining with HS4C3 and AO4B08 antibodies was detected exclusively in tumor endothelium, which was visualized with the antibody to endothelial cell marker vWf, whereas no staining was detected in ovarian tumor cells (Fig. 1B). Enumeration of vessels that were positive for the epitopes recognized by HS4C3, AO4B08, EV3C3, and RB4AE12 antibodies in each of the tumor samples showed that in the majority of tumors more than 50% of blood vessels were recognized by HS4C3 and AO4B08 antibodies (Fig. 1C and supplemental Table S2). Conversely, the majority of the tumor blood vessels did not bind RB4AE12 antibody, whereas the EV3C3 antibody detected less than 50% of blood vessels in a proportion of the tumors (Fig. 1C and supplemental Table S2). These data suggest that N-/6-O-/3-O- and 2-O-/N-/6-O-sulfated sequences are characteristic to ovarian tumor endothelium. We evaluated HS sulfation-specific staining intensity by scoring it as strong, moderate, or low (supplemental Table S2). Strong to moderate staining with HS4C3 and AO4B08 antibodies was detected in the vasculature of ~70% of tumors (Fig. 1D). Both EV3C3 and RB4AE12 antibodies bound poorly to tumor endothelium (Fig. 1D).
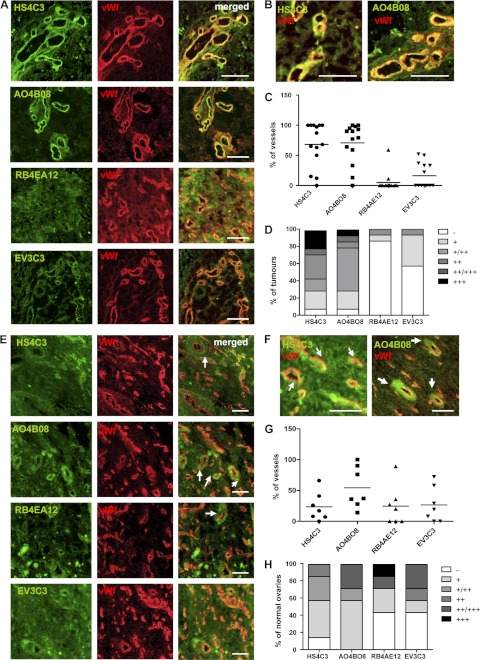
Ovarian tumor vasculature and normal ovarian tissue display distinct HS epitopes. A and B, frozen ovarian tumor sections were stained with HS sulfation-specific antibodies (green) and the antibody to vWf (red) to visualize endothelium. Images show specific HS epitope staining patterns with predominant staining in tumor endothelium. HS structures recognized by HS4C3 and AO4BO8 antibodies are highly expressed in tumor endothelium. Scale bars represent 100 μm. C, quantification of blood vessels that are positive for staining with HS4C3, AO4BO8, RB4EA12, and EV3C3 antibodies in each of the 14 ovarian tumors is shown. The percentage of HS epitope-positive vessels versus vWf-positive vessels is shown. The average percentage of blood vessels in all tumor samples recognized by HS4C3 and AO4BO8 antibodies is significantly higher than that of RB4EA12- and EV3C3-positive blood vessels. D, the intensity of endothelial HS staining was scored as strong (+++), moderate (++), low (+), or no staining (−). The percentage of tumors that show a particular staining intensity in endothelium is shown. E, distribution of specific HS epitopes identified by HS4C3, AO4BO8, RB4EA12, and EV3C3 antibodies (green) in normal ovarian tissue sections is shown. Images show lower levels of expression of epitopes recognized by HS4C3 and AO4BO8 antibodies in normal ovarian endothelium when compared with tumor endothelium (Fig. 1A). Some blood vessels show a lack of co-localization of HS epitope staining (green) with the staining for vWf (red; white arrows). Scale bars represent 100 μm. F, images show enlarged areas of sections co-stained with HS4C3 or AO4BO8 and anti-vWf antibodies where specific HS epitopes are detected in perivascular regions (white arrows). Scale bars, 50 μm. G, shown is quantification of blood vessels that show positive vascular/perivascular staining with HS4C3, AO4BO8, RB4EA12, and EV3C3 antibodies in normal ovaries. The percentage of blood vessels that are recognized by HS sulfation-specific antibodies in each normal ovarian sample is shown. H, intensity of endothelial/perivascular HS staining was scored as in Fig. 1D.
The above data suggest that specific, highly sulfated HS sequences were present in tumor endothelial HS, although it is unclear whether this is a generic finding or one associated with cancer. Thus, we compared normal ovarian tissue staining patterns with those in tumors. N-/6-O-/3-O-Sulfated (HS4C3) and 2-O-/N-/6-O-sulfated (AO4B08) motifs were less prevalent in normal ovarian vasculature (Fig. 1E). Moreover, some of the blood vessels showed perivascular staining for HS4C and AO4B08 epitopes (Fig. 1, E and F, white arrows). Enumeration of normal ovarian blood vessels with vascular/perivascular HS4C3 or AO4B08 staining showed significantly fewer positive vessels than in tumor vasculature, whereas more vessels expressed epitopes recognized by EV3C3 and RB4AE12 antibodies (Fig. 1, G and C, and supplemental Table S3). The expression levels of epitopes detected by HS4C3 or AO4B08 antibodies were also lower in normal ovarian tissue, particularly that of HS4C3 antibody (Fig. 1, H and D), whereas staining with EV3C3 and RB4AE12 antibodies was more prominent in normal ovarian vasculature than in ovarian tumor endothelium (Fig. 1, H and D). These data suggest that HS in malignant endothelium is highly sulfated, bear significant amounts of the 6S moiety, and uniquely also contain 3-O-sulfated sequences.
Down-regulation of 6-O-Sulfation in Endothelial Cells
Because we discovered high levels of N-/6-O-/3-O and N-/2-O-/6-O sulfation in most tumor blood vessels, we further focused on the biological significance of 6S in endothelial cells. We down-regulated HS6ST-1 and HS6ST-2 in HUVECs using retroviral shRNA vectors specifically targeting either HS6ST-1 or HS6ST-2 (supplemental Table S4). Retroviral transduction of HUVECs with shRNAs against HS6ST-1 (sh6ST1-1 and sh6ST1-2) or HS6ST-2 (sh6ST2-1 and sh6ST2-2) produced stable cell lines with reduced expression of either HS6ST-1 or HS6ST-2 when compared with cells transduced with nonspecific shRNA (NS) (Fig. 2A).
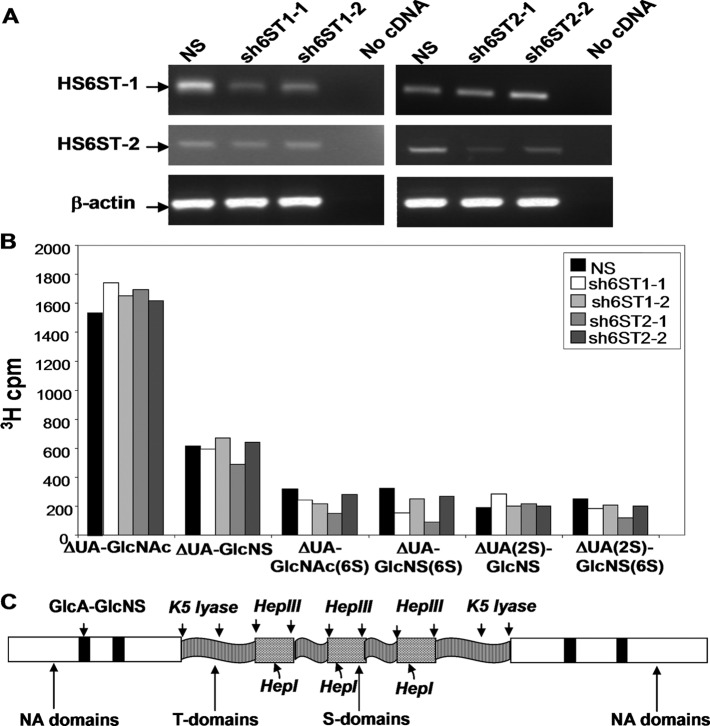
Down-regulation of HS6ST-1 and HS6ST-2 reduces 6-O-sulfation in endothelial cell HS. A, reduction of HS6ST-1 and HS6ST-2 gene expression in HUVECs transduced with shRNAs targeting either HS6ST-1 or HS6ST-2 is shown. mRNA levels of HS6ST-1 and HS6ST-2 genes were evaluated by RT-PCR. NS, nonspecific shRNA. B, 3H-labeled HS extracted from NS, sh6ST1-1, sh6ST1-2, sh6ST2-1, and sh6ST2-2 HUVEC cell lines was depolymerized to completion with heparinases I, II, and III and separated on HPLC-SAX. The amount of 3H in each disaccharide peak is expressed as cpm per disaccharide. C, shown is a schematic representation of domain organization in HS extracted from HUVECs expressing nonspecific shRNA (NS), shRNA targeting HS6ST-1 (sh6ST1-2), or shRNA targeting HS6ST-2 (sh6ST2-1). HS from all cell lines showed identical HS domain organization (supplemental Fig. S1–S3). HS domain analysis suggests that multiple short S domains alternating with shorter T domains form composite extended sulfated domains flanked by longer T domains and separated by large non-sulfated NA domains.
Having reduced HS6ST-1 or HS6ST-2 levels in HUVECs, we characterized the HS disaccharide composition in these cell lines. The down-regulation of HS6ST-1 resulted in a 36 and 22% decrease in 6-O-sulfation in sh6ST1-1 and sh6ST1-2 cells, respectively (Table 1, Fig. 2B). Similarly, reduced expression of HS6ST-2 led to a decrease in 6-O-sulfation by 39 and 11% in sh6ST2-1 and sh6ST2-2 cells, respectively (Table 1, Fig. 2B). The levels of 2-O-sulfation were unaffected; however, the sh6ST1-1 and sh6ST2-1 cells exhibited reduced N-sulfation of 16.2 and 11.6% in addition to their loss of 6S (Table 1).
TABLE 1
The effect of endothelial HS6ST-1 and HS6ST-2 knock-down on HS disaccharide composition
ΔUA, uronic acid; GlcNS, N-sulfated glucosamine.
Frequency | |||||
---|---|---|---|---|---|
NS | sh6ST1-1 | sh6ST1-2 | sh6ST2-1 | sh6ST2-2 | |
% | |||||
HS disaccharide structure | |||||
![]() ![]() ![]() ![]() | 48.2 | 54.4 | 51.6 | 56.4 | 50.5 |
![]() ![]() ![]() ![]() | 19.2 | 18.6 | 21.0 | 19.1 | 20.0 |
![]() ![]() ![]() ![]() | 10.0 | 7.6 | 6.8 | 6.9 | 8.7 |
![]() ![]() ![]() ![]() | ND | ND | ND | ND | ND |
![]() ![]() ![]() ![]() | 10.1 | 4.8 | 7.9 | 5.3 | 8.4 |
![]() ![]() ![]() ![]() | 6.0 | 8.9 | 6.3 | 7.0 | 6.2 |
![]() ![]() ![]() ![]() | 7.9 | 5.7 | 6.5 | 5.2 | 6.2 |
Sulfation position | |||||
![]() ![]() ![]() ![]() | 43.2 | 38.0 | 41.7 | 36.6 | 40.8 |
![]() ![]() ![]() ![]() | 13.9 | 14.6 | 12.8 | 12.2 | 12.4 |
![]() ![]() ![]() ![]() | 28.0 | 18.1 | 21.2 | 17.4 | 25.3 |
To investigate if HS6ST-1 and HS6ST-2 down-regulation affected the HS domain structure, [3H]glucosamine-labeled HS was subjected to three different enzyme, and HNO2 treatments and the resulting fragments were analyzed by size-exclusion chromatography. The chromatographic profiles of HS from NS, sh6ST1-2, and sh6ST2-1 cells were similar (supplemental Fig. S1–S3 and Table S5), indicating that HS domain organization in cells with reduced 6-O-sulfation remained unchanged. Interpretation of HS domain organization analysis described in the supplemental data Experimental Procedures shows that short S domains alternate with short K5 lyase resistant structures (T-domains) embedded in non-sulfated regions (NA domains) (Fig. 2C). HS chains contain groups of 2–3 S domains composed of 6 or 8 monosaccharides, separated by even shorter T domains and forming a composite structure spaced by extended N-acetyl sequences (NA domains) (Fig. 2C). Such short sequences in composite S domains would be sufficient to bind FGF2 (28, 29).
Specific Endothelial Cell Functions Are Compromised by Reduced 6-O-Sulfation in Vitro
To investigate whether reduced 6-O sulfation in discrete domains of endothelial HS affects specific FGF2- and VEGF165-dependent endothelial cell functions, we performed endothelial sprouting and tube formation assays using HUVECs with down-regulated HS6ST-1 or HS6ST-2. Endothelial spheroids generated from NS, sh6ST1-1, sh6ST1-2, sh6ST2-1, and sh6ST2-2 cells were embedded in fibrin gels and stimulated with either FGF2 or VEGF165 for 24 h. Evaluation of the sprouting area showed almost complete ablation of FGF2- and VEGF165-induced sprouting in all cell lines with reduced 6-O-sulfation when compared with the control NS cells (Fig. 3, A and B). This defect was rescued by culture media conditioned by wild-type HUVECs (Fig. 3C), whereas media that were treated with heparinases I, II, and III to degrade shed HS lost its ability to rescue endothelial cell sprouting (Fig. 3D). This indicates that soluble HS fragments with a higher 6-O-sulfate content are capable of compensating for the cell surface HS function. Conversely, the media conditioned by sh6ST1-2 and sh6ST2-1 cells inhibited control NS cell sprouting in response to FGF2 and VEGF165 (Fig. 3E), suggesting that HS with reduced 6-O-sulfation acts as an inhibitor of FGF2 and VEGF165 in the presence of the wild-type cell surface HS. To evaluate whether FGF2-induced sprouting could be mediated by VEGF165 expression, we performed a sprouting assay in the presence of FGF2 and VEGF neutralizing antibody. Supplemental Fig. S4 shows that neither VEGF165 expression was induced in FGF2-stimulated HUVEC monolayers nor was FGF2-dependent sprouting affected in the presence of neutralizing antibody to VEGF.
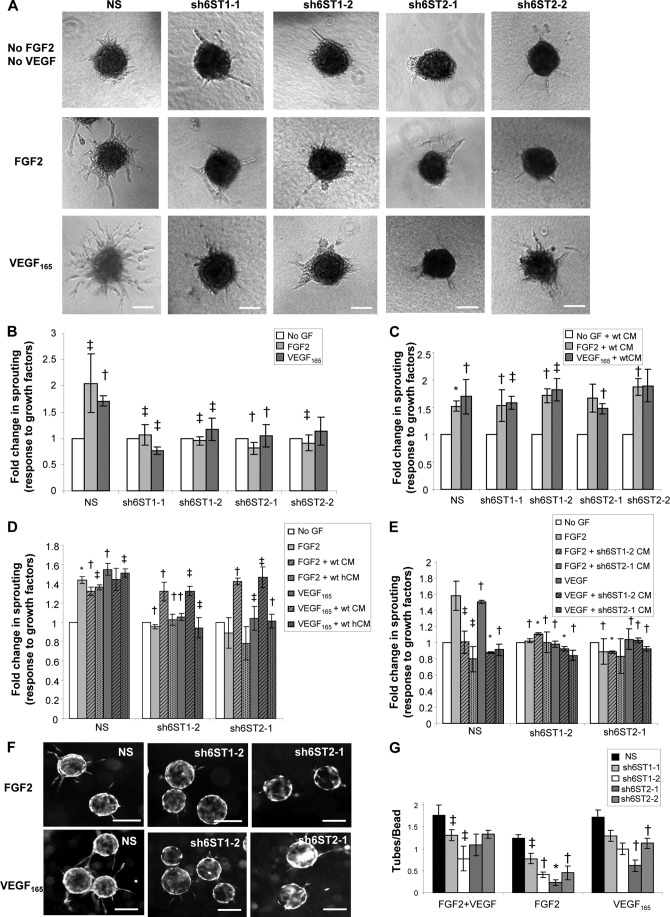
Reduced 6-O-sulfation affects endothelial cell sprouting and tube formation. A, endothelial spheroid sprouting in response to FGF2 and VEGF165 is significantly affected by reduced levels of HS6ST-1 and HS6ST-2 expression. Endothelial cell spheroids were embedded in fibrin gels and either cultured in EBM-2 media lacking FGF2 and VEGF165 or treated with 5 ng/ml FGF2 or 2.5 ng/ml VEGF165 for 24 h. Scale bars represent 200 μm. B and C, the area of outgrowing sprouts from each spheroid was evaluated using the Metamorph program by measuring and subtracting the area of each spheroid without sprouts from the area including outgrowing sprouts. The areas of sprouts without and with growth factor stimulation were compared. The area of emerging sprouts from non-stimulated spheroids represents a control. 20–30 spheroids were analyzed per each experiment, and three independent experiments were performed. In C, stimulation of spheroids with FGF2 and VEGF165 was performed in the presence of conditioned media (CM) collected from wild-type HUVECs. GF, growth factors FGF2 and VEGF165. The values in B and C are shown as the mean ± S.E. (n = 3). *, p < 0.001; †, p < 0.01; ‡, p < 0.05. D, spheroids were cultured in wild-type HUVEC-conditioned media that was either treated with heparinases I, II, and III (hCM) or was incubated in heparinase buffer alone (CM). The experiment was performed twice, and results are presented as the mean ± S.E. *, p < 0.001; †, p < 0.01; ‡, p < 0.05. E, conditioned media (CM) from sh6ST1-2 or sh6ST2-1 cells inhibits control NS spheroid sprouting in response to FGF2 and VEGF165. Results are derived from two independent experiments and are shown as the mean ± S.E. *, p < 0.001; †, p < 0.01; ‡, p < 0.05. F, Cytodex-3 beads were coated with NS, sh6ST1-2, and sh6ST2-1 HUVECs and embedded in fibrin gels that were treated with either FGF2 (10 ng/ml) or VEGF165 (2.5 ng/ml) for 6 days. Tubes were visualized by staining with Calcein-AM. Scale bars, 100 μm. G, the average number of tubes per bead (50–100 beads were evaluated per each experiment) was quantified in all cell lines treated with FGF2, VEGF165, or with FGF2 and VEGF165 combined. The experiment was performed three times, and results are shown as the mean ± S.E. *, p < 0.001; †, p < 0.01; ‡, p < 0.05.
To test the impact of the lower 6-O-sulfation level on FGF2- and VEGF165-dependent endothelial tube formation, we coated Cytodex 3 microcarriers with NS, sh6ST1-1, sh6ST1-2, sh6ST2-1, and sh6ST2-2 cells and embedded them into fibrin gels. FGF2 or VEGF165 stimulation resulted in the growth of vessel-like structures from HUVEC-coated beads (Fig. 3F; shown for NS, sh6ST1-2, and sh6ST2-1 cells). All cell lines with reduced 6-O-sulfation showed a defect in angiogenic growth factor-induced tube formation, although inhibition of FGF2-mediated tube formation was more profound than that induced by VEGF165 (Fig. 3G). HUVECs with the lowest 6-O-sulfate content (sh6ST2-1) had the greatest reduction in FGF2- and VEGF165-induced tube formation; 82 and 65%, respectively (Fig. 3G).
Our data demonstrated that soluble wild-type HS rescues FGF2- and VEGF165-dependent endothelial cell sprouting in cells with down-regulated HS6ST-1 and HS6ST-2 expression (Fig. 3C). We investigated whether sprouting and tube formation could also be rescued by the cell surface HS on neighboring cells. To address this question, we generated endothelial spheroids from NS, sh6ST1-1, sh6ST1-2, sh6ST2-1, and sh6ST2-2 cells mixed in a 1:1 ratio with the GFP-transduced HUVECs. Fluorescence and brightfield imaging of angiogenic growth factor-stimulated chimeric spheroids embedded in fibrin gels revealed that individual sprouts were composed of either a mixture of wild-type GFP-positive HUVECs and sh6ST1-1, sh6ST1-2, sh6ST2-1, or sh6ST2-2 cells, where GFP-positive cells could be detected either at the tip or the stalk of a sprout (Fig. 4A, shown for NS and sh6ST2-1 cells, white arrows), NS, or sh6ST2-1 cells alone (Fig. 4A, black arrowheads) or only GFP-positive HUVECs (Fig. 4A, white arrowheads). Quantification of the sprouting area using brightfield images revealed that GFP-HUVECs failed to rescue the defect in endothelial cell sprouting (Fig. 4B). Examining the same question with respect to endothelial tube formation, we co-cultured PaSMCs with control or HS6ST down-regulated HUVECs in the presence or absence of FGF2 and VEGF165 for 5 days. Staining for CD31 demonstrated the formation of tube-like structures that were covered by a confluent monolayer of PaSMCs (Fig. 4, C and D). However, soluble exogenous VEGF165 failed to stimulate endothelial tube formation (Fig. 4E), which was consistent with the published data (24). In contrast, FGF2 increased the area of the tubular network by 30% in control cells (Fig. 4E), whereas cells with reduced 6-O-sulfation failed to respond to FGF2 (Fig. 4E). Significant differences in HS composition in HUVECs and PaSMCs were detected (Table 1 and supplemental Table S6). Total sulfation in PaSMC HS was lower than that of HUVECs (73 versus 85%), the greatest difference being in 2-O-sulfation, which was 17% higher than that seen in NS HUVECs (supplemental Table S6). Although the level of N-sulfation was comparable with those seen in HUVECs, the 6-O-sulfation level was 9.6% lower than that found in the HS of HUVECs with down-regulated HS6STs (supplemental Table S6). These data suggest that endothelial cell HS has a unique function in regulating endothelial cell responses to angiogenic growth factors.
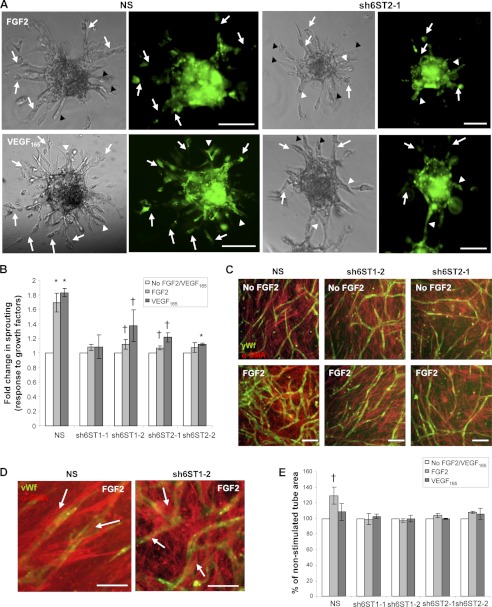
HS on neighboring wild-type HUVECs and smooth muscle cells does not rescue glucosamine 6-O-sulfation-dependent endothelial cell sprouting and tube formation. A and B, endothelial spheroids were generated by combining HUVECs expressing nonspecific shRNA (NS), shRNAs targeting HS6ST-1 (sh6ST1-1, sh6ST1-2), or shRNAs targeting HS6ST-2 (sh6ST2-1 and sh6ST2-2) with HUVECs expressing GFP at 1:1 ratio. Representative images of chimeric NS and GFP-HUVEC and sh6ST2-1 and GFP-HUVEC spheroids are shown in A. White arrows show sprouts composed of a mixture of GFP-expressing cells (green) and NS or sh6ST2-1 cells. Black arrowheads indicate sprouts that consist of only NS or sh6ST2-1 cells, whereas the white arrowheads show sprouts that are composed of GFP-HUVECs alone. Sprout outgrowth was tested in the presence of FGF2 or VEGF165 at 5 and 2.5 ng/ml concentration, respectively. Scale bars in A represent 200 μm. The area of sprout outgrowth was evaluated in brightfield images using Metamorph as described in Fig. 3, B and C. Sprouting in the absence of FGF2 or VEGF165 represents a control. 20–30 spheroids were analyzed per each experiment, and three independent experiments were performed. The values are represented as the mean ± S.E. (n = 3). *, p < 0.001; †, p < 0.01. C, PaSMCs and NS, sh6ST1-2, and sh6ST2-1 cell lines were admixed before plating and co-cultured for 5 days in the presence or absence of FGF2 (5 ng/ml) or VEGF165 (2.5 ng/ml; images not shown). Cells were stained for vWf (green) and α-SMA (red) to visualize endothelial tubes and the smooth muscle cell monolayer, respectively. FGF2-induced increase in PaSMC-mediated endothelial tube formation is compromised in HUVECs with reduced HS6ST-1 or HS6ST-2 expression. The scale bar represents 100 μm. D, representative images of NS or sh6ST1-2 cell co-cultures with PaSMCs demonstrate smooth muscle cell coverage of vWf-positive endothelial tubes. White arrows show smooth muscle cells lining endothelial tubes. Scale bars, 25 μm. E, the endothelial tube network area was evaluated using ImageJ software. The control represents the tube area formed in the absence of FGF2 and VEGF165 and is expressed as 100% for each cell line. Treatments were performed in triplicate in two independent experiments and are expressed as mean ± S.D. †, p < 0.01.
Binding of FGF2 to HS and to Endothelial Cell Surface Is Not Affected by Reduced 6-O-Sulfation
Our data showed that lowering 6-O-sulfation levels leads to significantly reduced FGF2-dependent endothelial tube formation, whereas VEGF165 was less sensitive to a lower 6-O-sulfate content. To assess if compromised FGF2 phenotypic effects were a consequence of reduced affinity of HS to FGF2, we analyzed the strength of interaction between FGF2 and HS extracted from control HUVECs or HUVECs with reduced expression of HS6ST-1 (sh6ST1-2) and HS6ST-2 (sh6ST2-1). 3H-Labeled HS, generated through metabolic labeling of cellular HS, was applied to a FGF2 affinity column and step-eluted with increasing concentrations of NaCl. Binding of HS with reduced 6-O-sulfate content to FGF2 at physiological NaCl concentrations (0.15 m) was 20% greater than that of control (NS) HS (Fig. 5A), consistent with the notion that binding between FGF2 and HS depends on 2-O and N-sulfate content.
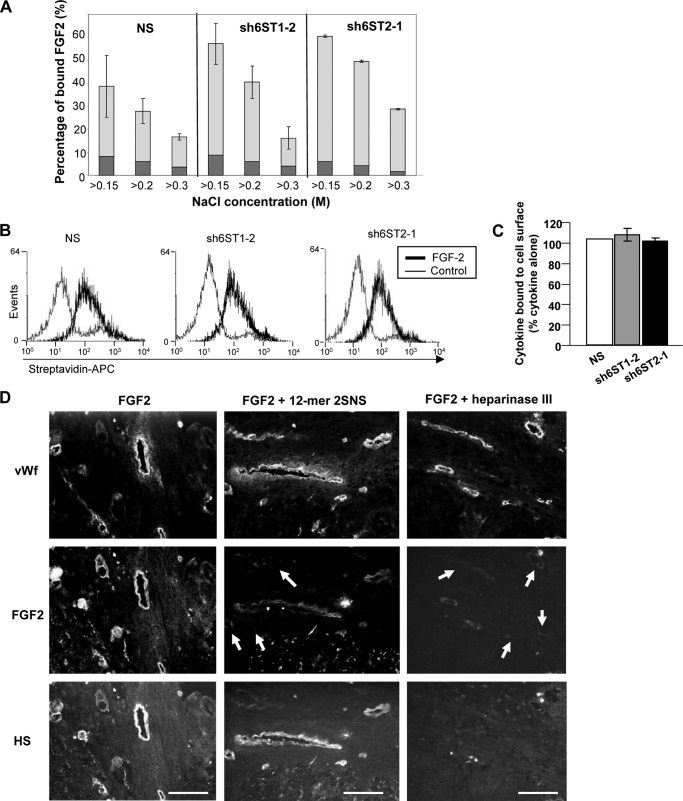
Reduced 6-O-sulfation levels in endothelial cell HS does not affect FGF2 binding to HS and to the cell surface. A, binding of HUVEC HS to immobilized FGF2. 3H-Labeled HS extracts from HUVECs expressing nonspecific shRNA (NS) or shRNAs targeting HS6ST-1 (sh6ST1-2) or HS6ST-2 (sh6ST2-1) were applied to FGF2 affinity column and eluted stepwise with 0.15, 0.2, and 0.3 m NaCl. Binding of 3H-labeled HS from NS, sh6ST1-2, and sh6ST2-1 cells to FGF2 is expressed as a percentage of material bound at NaCl concentrations above 0.15, 0.2, and 0.3 m (light gray bars). Background binding to a control unmodified Affi-Gel 10 column is depicted in dark gray. Results are represented as the mean ± S.D. (n = 2). B, NS, sh6ST1-2, and sh6ST2-1 HUVECs were mixed with biotin-labeled FGF2 and incubated at 4 °C. The binding to endothelial cell surface was determined by the addition of streptavidin-APC (streptavidin conjugated to Allophycocyanin) and analyzed by FACS. A control histogram represents binding of nonspecific biotinylated protein to endothelial cell surface. C, the percentage of FGF2 binding to sh6ST1-2 and sh6ST2-1 HUVECs when compared with NS HUVECs (100%) is shown as the mean ± S.D. (n = 2). D, FGF2 was allowed to bind to ovarian tumor sections in the absence or presence of synthetic structurally defined dodecasaccharide 2SNS (12-mer 2SNS; left and middle panels, respectively). Alternatively, tumor sections were pretreated with heparinase III before incubating with FGF2 to remove HS from the cell surface (right panels). Endothelium was visualized by staining with anti-vWf antibody (top panels), bound FGF2 was detected by staining with antibody to FGF2 (middle panels), and HS was visualized with 10E4 antibody (lower panels). White arrows show reduced FGF2 binding to endothelium when FGF2 was admixed with 12-mer 2SNS or when tumor sections were pretreated with heparinase III. Scale bars represent 100 μm.
We addressed the same question at the cellular level. To investigate if reduced 6-O-sulfation changes FGF2 binding to the surface of HUVECs, we treated NS, sh6ST1-2, and sh6ST2-1 cells with biotinylated FGF2 at 4 °C to prevent internalization of FGF2 and determined its levels on the cell surface by FACS analysis. As shown in Fig. 5, B and C, the levels of FGF2 and VEGF165 bound to control NS cells and cells with reduced HS6ST-1 (sh6ST1-2) or HS6ST-2 (sh6ST2-1) expression were unchanged.
Previously we showed that synthetic structurally defined 2-O- and N-sulfated dodecasaccharide (12-mer 2SNS) was able to compete with HS for FGF2 and VEGF165 binding (20), suggesting that 6-O-sulfates are not essential for binding to these growth factors. Therefore, we tested if 12-mer 2SNS prevents binding of FGF2 to ovarian tumor endothelium. As expected, binding of FGF2 to tumor endothelium was significantly reduced by 12-mer 2SNS (Fig. 5D, middle panels). Thus, these data support the concept that the interaction between HS and FGF2 is independent of the 6S content in HS.
6-O-Sulfation Levels Regulate FGF2-induced Receptor Phosphorylation and FGFR1 Internalization
Although the binding of FGF2 to HS with reduced 6-O-sulfation was unaffected, we investigated whether the activities of FGFR/FGFR signaling complexes were compromised. The phosphorylation level of FRS2 in control cells increased after stimulation with FGF2 at 10 and 15 min, whereas cells with the lower levels of 6-O-sulfation (sh6ST1-2 and sh6ST2-1) manifested reduced phosphorylation of FRS2 at these time points followed by reduced phospho-Erk levels after a 15-min treatment with FGF2 (Fig. 6A).
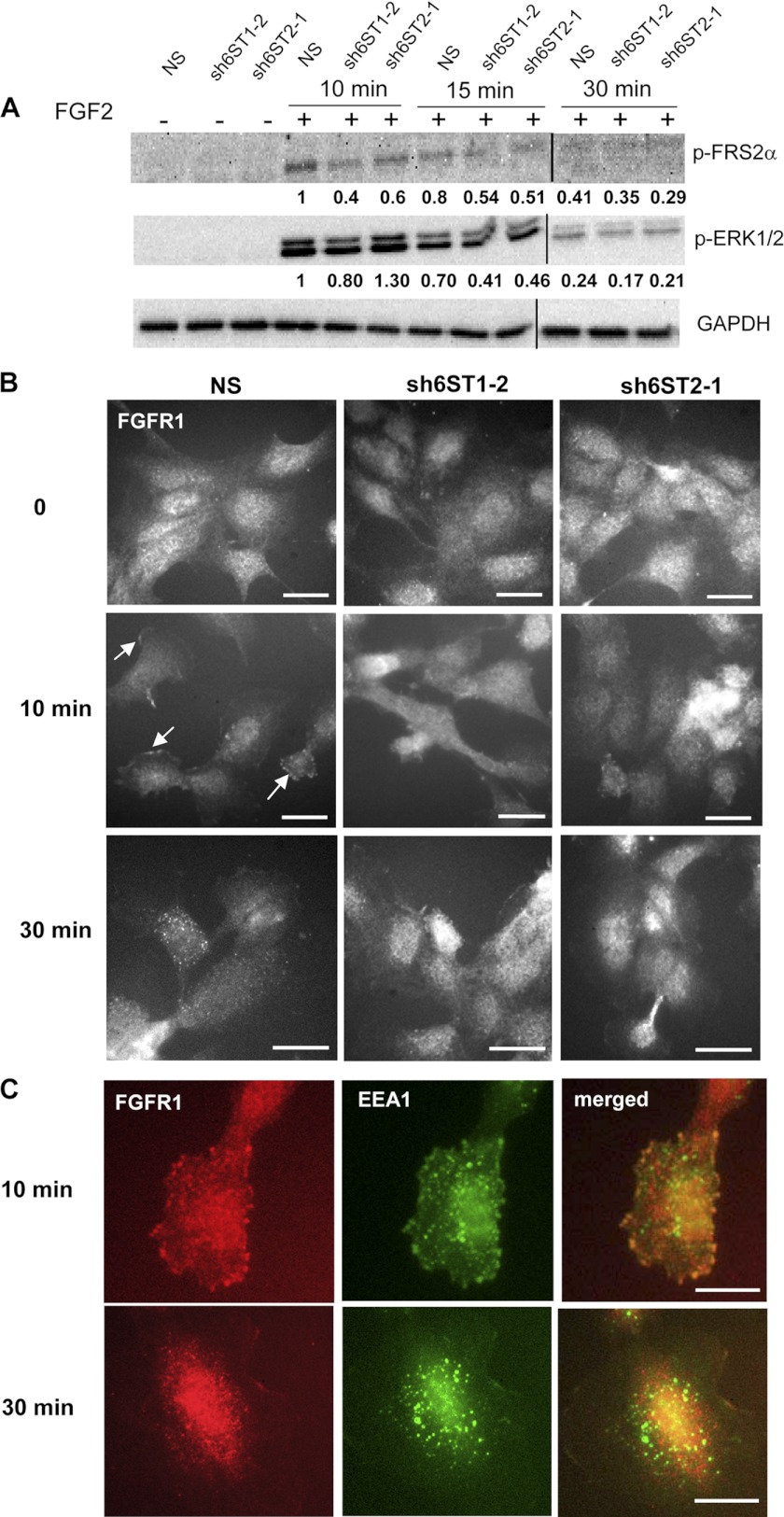
Glucosamine 6-O-sulfation level impacts upon growth factor-induced receptor phosphorylation and FGFR1 internalization. A, serum-starved HUVECs expressing either nonspecific shRNA (NS) or shRNAs targeting HS6ST-1 (sh6ST1-2) or HS6ST-2 (sh6ST2-1) were stimulated with FGF2 (20 ng/ml) for the indicated time points. Phosphorylated FRS2 and Erk were detected by Western blotting. Equal protein loading was monitored by probing with the anti-GAPDH antibody. The values below each blot represent an average normalized -fold change in the intensities of bands as compared with the bands corresponding to FGF2 stimulated NS cells for 10 min. The normalized -fold change was derived from two independent experiments. The lysates that were prepared from cells stimulated for 30 min were loaded on a separate gel. GAPDH protein levels are equal in both blots. B, FGF2 was allowed to bind to NS, sh6ST1-2, and sh6ST2-1 HUVECs on ice. Cells were washed and then returned to 37 °C for 10 and 30 min. Cells were stained with anti-FGFR1 antibody to detect spatiotemporal localization of FGFR1 in response to FGF2 stimulation. White arrows show peripheral staining for FGFR1 at the cell membrane after 10-min of stimulation with FGF2 in NS cells. After 30 min of FGF2 stimulation, in a number of NS cells FGFR1 vesicular staining was detected in the perivascular area. sh6ST1-2 and sh6ST2-1 cells show uniform FGFR1 staining at all time points. Scale bars represent 25 μm. C, NS cells were treated as in B and co-stained with anti-FGFR1 (red) and anti-EEA1 (green) antibodies. Scale bars, 10 μm.
To test if FGF2 stimulation changes subcellular localization of FGFR1 in control NS cells and in cells that display reduced 6-O-sulfation (sh6ST1-2 and sh6ST2-1), we incubated cells with FGF2 on ice and then returned them for indicated time points to 37 °C. FGFR1 distribution in NS control cells changed after stimulation with FGF2 from uniform staining at time 0 to either continuous or discreet punctate staining at plasma membrane after 10 min and accumulation in intracellular vesicles in the perinuclear region after 30 min (Fig. 6B). In contrast, the majority of sh6ST1-2 and sh6ST2-1 cells stimulated with FGF2 for 15 and 30 min displayed uniform FGFR1 staining with occasional accumulation of FGFR1 in intracellular vesicles in some cells (Fig. 6B). After a 10-min incubation at 37 °C NS cells with punctate FGFR1 staining displayed a large proportion of the receptor in early/sorting endosomes as shown by co-localization of FGFR1 with EEA1, a marker associated with early endosomes (Fig. 6C). In contrast, after 30 min, internalized FGFR1 no longer co-localized with EEA1 (Fig. 6C). These data suggest that down-regulation of 6-O-sulfation affects receptor activation, internalization, and accumulation in early endosomes.
FGF2-dependent Angiogenesis in Vivo Is Regulated by Glucosamine 6-O-Sulfate Levels
Because reduced 6-O-sulfation impacted on FGF2-dependent endothelial tube formation in vitro to a greater extent than VEGF165, we examined the effect of 6-O-sulfate reduction in FGF2-dependent angiogenesis model in vivo. Matrigel/fibrin plugs containing NS, sh6ST1-2, and sh6ST2-1 cell spheroids were maintained subcutaneously in mice for 21 days to allow vessels to develop. After dissection, plugs were analyzed for human endothelial cell-derived vasculature, which was evident in all three cell lines as demonstrated by staining with antibodies to human CD31 and CD34 (Fig. 7, A and B). However, quantification of the number of tubes in multiple sections showed that tube density was reduced by ~50% in plugs generated from sh6ST1-2 and sh6ST2-1 cell spheroids when compared with control NS spheroids (Fig. 7C). In addition, tubes generated from sh6ST1-2 and sh6ST2-1 cell spheroids were on average 50% shorter than those generated from the control NS cell spheroids (Fig. 7D). To determine whether reduced 6-O-sulfation had an effect on recruitment of mouse mural cells to human vasculature, plugs were stained with the antibody to murine α-smooth muscle actin (α-SMA). Quantification of vessels with co-localized human CD34 and murine α-SMA staining revealed no differences in mural cell coverage between control, sh6ST1-2, and sh6ST2-1 endothelial tubes (Fig. 7E), suggesting that mural-endothelial cell interactions were not affected by reduced 6S expression.
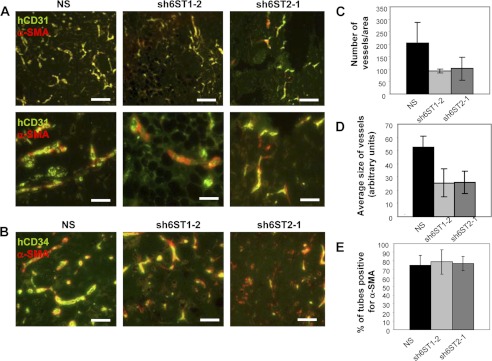
Glucosamine 6-O-sulfation level regulates angiogenesis in vivo. A and B, HUVEC spheroids form vasculature in Matrigel/fibrin plugs containing FGF2 in mice. Vasculature derived from HUVEC spheroids with reduced levels of HS6ST-1 (sh6ST1-2) or HS6ST-2 (sh6ST2-1) display areas with lower density of blood vessels when compared with the vasculature originating from HUVECs expressing nonspecific shRNA (NS). Blood vessels were visualized by staining for human CD31 (green; A) or human CD34 (green; B). Host mural cell coverage of human vasculature is visualized by staining for mouse α-SMA (red). Scale bars in the upper panels in A represent 100 μm. Scale bars in the lower panels in A and in B represent 50 μm. C, microvessel density is expressed as an average number of fluorescent objects (staining for hCD31) determined by ImageJ program and normalized per area of analyzed sections from each of the four plugs. The average number of vessels derived from four plugs is shown as the mean ± S.D. D, fluorescent object size was determined by ImageJ program in sections stained for hCD31. Four plugs were analyzed per each cell line as in C. Results show average vessel size as the mean ± S.D. (n = 4). E, blood vessels were evaluated for positive staining for host α-SMA (red). Two hundred blood vessels were evaluated in each plug. Four plugs were analyzed per each cell line, and an average number of vessels positive for mouse α-SMA is shown as mean ± S.D.
DISCUSSION
The obligate requirement of angiogenic cytokines for HS highlights the importance of understanding HS structure-function relationships if we are to develop novel anti-angiogenic agents that target this mechanism. In this study we investigated HS in tumors from patients with advanced stage ovarian cancer and demonstrated that 6S-containing motifs were overrepresented in ovarian tumor endothelium when compared with normal ovarian blood vessels. By down-regulating HS6ST-1 or HS6ST-2, we have defined significant relationships between the level of 6-O-sulfates in endothelial cell HS and FGF2- and VEGF165-dependent endothelial cell functions.
The ability of ovarian tumor endothelium to assemble the FGF2/FGFR/HS signaling complexes (7) raises the question about HS structural properties required for the formation and activity of tri-molecular signaling complexes in tumor endothelium. Using antibodies specific for defined HS sulfation sequences we detected increased 2-O-/N-/6-O- and N-/6-O/3-O-sulfate levels in the endothelium of ovarian tumors, thus highlighting the importance of 6-O-sulfate moieties in tumor endothelial HS that supports FGF2 and VEGF165 signaling. In addition, our previous study showed that heparanase and 6-O-endosulfatase-1 were abundantly expressed by ovarian tumor cells, but not endothelium, thus explaining the lack of biologically active 6-O-sulfated HS in ovarian tumor cells (30).
Our data suggest that 6-O-sulfates in extended composite S domains are of major importance in regulating endothelial cell functions, such as endothelial cell sprouting and tube formation. Moreover, HS with reduced 6-O-sulfate content has an inhibitory function, as endothelial cell-shed soluble HS with reduced 6-O-sulfation inhibits wild-type spheroid sprouting in response to angiogenic growth factors (Fig. 3E). This is consistent with the published data showing that de-6-O-sulfated heparin (18) or uniformly 2-O and -N-sulfated synthetic dodecasaccharide (20) are effective inhibitors of various cellular functions (18, 20). Our results also imply that cell surface HS with reduced 6-O-sulfation may act as an inhibitor of the wild-type neighboring cells, as chimeric endothelial spheroids composed of a mixture of wild-type HUVECs and HUVECs with down-regulated HS6ST expression fail to respond to angiogenic growth factors (Fig. 4, A and B). The inability of wild-type HS shed from the GFP-expressing cells in chimeric spheroids to rescue a sprouting defect may be due to low local HS concentration when compared with HS concentration in HUVEC monolayer-conditioned media that efficiently rescues sprouting (Fig. 3C). Alternatively, chimeric spheroid-shed HS with reduced 6-O-sulfation may have a dominant inhibitory effect on the neighboring wild-type GFP-expressing cells. An in vitro tube formation model involving co-culture of HUVECs with smooth muscle cells suggests that endothelial cell HS might have a cell-autonomous function, as smooth muscle cells do not rescue endothelial tube formation deficiency in response to FGF2 in HUVECs with down-regulated HS6ST-1 or HS6ST-2 (Fig. 4, C–E). Moreover, HUVECs with reduced 6-O-sulfation form fewer and smaller tubes in mice, and host mural cells, which effectively interact with the tubes, are unable to compensate for 6-O-sulfate deficient endothelial function (Fig. 7). These data are also supported by another study where xenograft tumor growth was reduced in animals with endothelial-targeted deletion of HS-modifying enzyme Ndst1 through the deficiency of vessel infiltration and branching (31). Endothelial HS in these animals showed reduced sulfation at N-, 2-O- and 6-O-positions, thus demonstrating the importance of either specific sulfation pattern or overall charge in endothelial cell HS (31). In contrast to our study, wild-type embryonic stem cells or perivascular smooth muscle cells rescued the inability of Ndst1/2 knock-out mouse embryoid bodies, which lacked N- and 2-O-sulfation but retained low levels of 6-O-sulfation, to develop vascular capillary-like structures in response to VEGF165 (32). The ability of HS to act in trans in this model might reflect the difference between stem cell and mature endothelial cell behavior. Alternatively, it may also suggest that 2-O-sulfated iduronate and N-sulfated glucosamine are essential for inhibitory activity of HS.
We discovered that the levels of sulfation in HUVEC HS are much higher than those reported for bovine aorta endothelial cells (33, 34). In particular, 6-O-sulfation is almost six times higher than that seen in bovine aorta endothelial cells, the HS of which is still capable of binding FGF2. This suggests that some of the 6-O-sulfated residues in HUVECs may be redundant with respect to FGF2 binding. On the other hand, our data show that even a low level of reduction in 6-O-sulfation, for example by ~10% in sh6ST2-2 cells (Table 1), leads to diminished response of endothelial cells to angiogenic growth factor stimulation (Fig. 3). These observations suggest that although there may be some redundancy in terms of the amount of 6-O-sulfates needed for binding of FGF2, these moieties are critical for the activity of FGF2. The inference is that loss of 6-O-sulfation in the short S domains of HUVEC HS has a significantly negative impact on the activity of ternary complex and signaling. Consistent with this idea, a recent study reported that one additional sulfate in an HS-derived decasaccharide containing a defined number of sulfates at 2-O- and 6-O-positions significantly improved the ability of this oligosaccharide to potentiate FGF2 signaling in cells lacking endogenous HS (35). Together these data suggest that the critical level of sulfation at specific positions is the key factor in regulating FGF2-induced signaling.
FGF2-FGFR1 signaling was compromised in response to FGF2 in cells with lower levels of HS6ST-1 or HS6ST-2, although the binding of angiogenic growth factors to HS isolated from these cells or to the cell surface was unaffected (Figs. 5 and and6).6). FGF2 forms tri-molecular complexes involving receptors and HS (36–38). Because ligand-induced FGFR activation can initiate distinct signaling pathways that lead to cell proliferation, migration, or cell differentiation (39), it is possible that HS structural characteristics play an important role in regulating conformational states of FGF/FGFR complexes that would determine specific endothelial cell phenotypes. Therefore, reduced 6-O-sulfation levels might not prevent trimolecular complex formation but might affect the structural features of the complex and the downstream signaling events. Furthermore, we found that FGF2-induced peripheral accumulation of FGFR1 and its association with early endosomes at a time of maximal phosphorylation of FGFR1 adaptor protein FRS2 in control cells was undetectable in cells with down-regulated HS6ST-1 or HS6ST-2 (Fig. 6).
The structure-function relationships for HS and angiogenic growth factors could be exploited in designing short HS sequences with optimal binding to angiogenic growth factors and/or receptors, but that lack activating moieties. Our work has demonstrated that endothelial 6-O-sulfation in alternating S and NA/NS domains is of major importance in supporting endothelial cell responses to angiogenic growth factors and that reducing 6-O-sulfation leads to significant inhibition of these responses. Our work suggests that there might be a critical number of 6-O-sulfates located in specific HS domains that are required to support ligand-induced angiogenic signaling through receptors. These results will be further exploited through our organic synthesis program that generates synthetic structurally defined antiangiogenic oligosaccharides.
Acknowledgments
Tumor samples were obtained from the Manchester Cancer Research Centre Biobank (UK) which is approved by South Manchester Research Ethics Committee (07/H1003/161). The role of the Manchester Cancer Research Centre Biobank is to distribute samples and, therefore, cannot endorse studies performed or the interpretation of results.
*This work was supported by Cancer Research United Kingdom Project Grant C2075/A11994 and Medical Research Council Developmental Pathway Funding Scheme G0902173/1.
This article contains supplemental Tables S1–S6, Figs. S1–S4, and Experimental Procedures.
3The abbreviations used are:
- FGF2
- fibroblast growth factor 2
- VEGF165
- vascular endothelial growth factor
- HS
- heparan sulfate
- HUVEC
- human umbilical vein endothelial cell
- PaSMCs
- pulmonary artery smooth muscle cells
- vWf
- von Willebrand factor
- Ndst1
- N-acetylglucosamine N-deacetylase/N-sulfotransferase 1
- NA domain
- N-acetylated domain
- 2S
- 2-O-sulfate
- 6S
- 6-O-sulfate
- HS6ST-1
- HS 6-O-sulfotransferase 1
- 2SNS
- 2-O- and N-sulfated
- α-SMA
- α-smooth muscle actin
- NS
- nonspecific shRNA
- FGFR
- FGF receptor.
REFERENCES
Articles from The Journal of Biological Chemistry are provided here courtesy of American Society for Biochemistry and Molecular Biology
Full text links
Read article at publisher's site: https://doi.org/10.1074/jbc.m112.384875
Read article for free, from open access legal sources, via Unpaywall:
https://europepmc.org/articles/pmc3476281?pdf=render
Citations & impact
Impact metrics
Citations of article over time
Alternative metrics
Article citations
The extracellular heparan sulfatase SULF2 limits myeloid IFNβ signaling and Th17 responses in inflammatory arthritis.
Cell Mol Life Sci, 81(1):350, 14 Aug 2024
Cited by: 0 articles | PMID: 39141086 | PMCID: PMC11335274
Trauma promotes heparan sulfate modifications and cleavage that disrupt homeostatic gene expression in microvascular endothelial cells.
Front Cell Dev Biol, 12:1390794, 24 Jul 2024
Cited by: 0 articles | PMID: 39114570 | PMCID: PMC11303185
Reproducing extracellular matrix adverse remodelling of non-ST myocardial infarction in a large animal model.
Nat Commun, 14(1):995, 22 Feb 2023
Cited by: 1 article | PMID: 36813782 | PMCID: PMC9945840
Validation of Recombinant Heparan Sulphate Reagents for CNS Repair.
Biology (Basel), 12(3):407, 04 Mar 2023
Cited by: 0 articles | PMID: 36979099 | PMCID: PMC10044841
HS, an Ancient Molecular Recognition and Information Storage Glycosaminoglycan, Equips HS-Proteoglycans with Diverse Matrix and Cell-Interactive Properties Operative in Tissue Development and Tissue Function in Health and Disease.
Int J Mol Sci, 24(2):1148, 06 Jan 2023
Cited by: 9 articles | PMID: 36674659 | PMCID: PMC9867265
Review Free full text in Europe PMC
Go to all (45) article citations
Data
Data behind the article
This data has been text mined from the article, or deposited into data resources.
BioStudies: supplemental material and supporting data
Protein structures in PDBe
-
(1 citation)
PDBe - 2SNSView structure
Similar Articles
To arrive at the top five similar articles we use a word-weighted algorithm to compare words from the Title and Abstract of each citation.
Ovarian cancer cell heparan sulfate 6-O-sulfotransferases regulate an angiogenic program induced by heparin-binding epidermal growth factor (EGF)-like growth factor/EGF receptor signaling.
J Biol Chem, 289(15):10488-10501, 22 Feb 2014
Cited by: 40 articles | PMID: 24563483 | PMCID: PMC4036170
HSulf-1 inhibits angiogenesis and tumorigenesis in vivo.
Cancer Res, 66(12):6025-6032, 01 Jun 2006
Cited by: 92 articles | PMID: 16778174
Epac1 increases migration of endothelial cells and melanoma cells via FGF2-mediated paracrine signaling.
Pigment Cell Melanoma Res, 27(4):611-620, 09 May 2014
Cited by: 23 articles | PMID: 24725364 | PMCID: PMC4283731
Anti-FGF2 approaches as a strategy to compensate resistance to anti-VEGF therapy: long-pentraxin 3 as a novel antiangiogenic FGF2-antagonist.
Eur Cytokine Netw, 20(4):225-234, 01 Dec 2009
Cited by: 39 articles | PMID: 20167562
Review
Funding
Funders who supported this work.
Cancer Research UK (1)
Grant ID: C2075/A11994
Medical Research Council (1)
Grant ID: G0902173/1