Abstract
Free full text

TRAIL suppresses tumor growth in mice by inducing tumor-infiltrating CD4+CD25+ Treg apoptosis
Abstract
Tumor necrosis factor-related apoptosis-inducing ligand (TRAIL), a promising and novel anticancer cytokine, specifically kills numerous tumor cells by apoptosis. However, some malignancies are resistant to TRAIL treatment in clinical trials, thus limiting its therapeutic potential. In the present study, the TRAIL-resistant murine hepatocellular carcinoma cell line Hepa1-6 was used to elucidate the physiological significance of TRAIL resistance, especially with respect to the immune regulatory function of TRAIL. Hepa1-6 cells were resistant to TRAIL-induced apoptosis in vitro; however, intratumoral injection of recombinant soluble TRAIL inhibited tumor growth and prolonged survival time in tumor-bearing mice. Local TRAIL treatment decreased the number of intratumoral CD4+CD25+Foxp3+ regulatory T cells (Tregs) but did not affect CD4+CD25+Foxp3+ Tregs in the draining lymph nodes and spleen. Further investigation showed that TRAIL induced apoptosis of tumor-activated CD4+CD25+Foxp3+ Tregs, but not of CD4+CD25− T cells. Moreover, mouse TRAIL receptor DR5 expression was detected on the surface of the tumor-infiltrating CD4+CD25+Foxp3+ Tregs, but not on naïve CD4+CD25+Foxp3+ Tregs. Interestingly, intratumoral injection of TRAIL not only decreased the number of CD4+CD25+Foxp3+ Tregs but also increased the number of tumor-specific CD8+ CTL and augmented their cytotoxicity to the tumor cells. These data provide the novel evidence for an immune regulatory function of TRAIL and may shed light on the clinical application of TRAIL.
Introduction
Tumor necrosis factor-related apoptosis-inducing ligand (TRAIL) efficiently induces apoptosis in numerous tumor cells and transformed cells but not in the majority of normal cells, suggesting that TRAIL could be a promising anticancer biotherapeutic [1–3]. To date, most studies on TRAIL have been focused on its ability to induce apoptosis in various cancer cells. Clinical trials demonstrated that systemically administered recombinant soluble TRAIL is promising and safe, unlike Fas ligand (FasL), which induces fulminant hepatic failure, and tumor necrosis factor-α (TNFα), which causes a systemic inflammatory response.
According to accumulating data, TRAIL not only functions to induce cell apoptosis but also works as a key effector molecule in the immune system. TRAIL−/− mice are more susceptible to autoimmune arthritis and diabetes [4], and TRAIL-R−/− mice infected with various pathogens display enhanced innate immune responses [5]. Mice carrying knockouts of TRAIL or its receptor show no developmental abnormalities, but display enhanced tumor metastasis, suggesting that TRAIL and TRAIL receptors are involved in the regulation of tumor immune surveillance [6–8]. TRAIL is expressed by immune effector cells and plays a role in shaping and regulating the immune system. It is likely that TRAIL is constitutively expressed on murine natural killer (NK) cells in liver and acts as an IFN-γ-dependent natural suppressor of spontaneous tumor development and tumor metastasis by TRAIL-sensitive tumor cells [9]. Stimulation of dendritic cells with IFN-β or IFN-γ increases the expression of TRAIL and enhances the cytotoxic effect of dendritic cells on tumor cells [10, 11]. Thus, TRAIL plays an important role in regulating the innate immune response of NK and dendritic cells. In addition to the physiological role of TRAIL in tumor immune surveillance involving NK and dendritic cells, TRAIL is also implicated in CD4+ cytotoxic T lymphocytes (CTL). CD4+ T cell clones constitutively express TRAIL, which is fully or at least partially responsible for cytotoxicity against certain tumor cells [12]. However, the immunological and physiological functions and mechanisms of TRAIL are not yet well understood.
In the present study, we found that a murine hepatocellular cell line, Hepa1-6, was resistant to TRAIL-induced apoptosis in vitro. However, intratumoral injection of recombinant soluble TRAIL in Hepa1-6 tumor-bearing mice inhibited tumor growth and significantly prolonged animal life span. These results suggest that TRAIL may play an important immune regulatory function in vivo. Indeed, a follow-up study using the Hepa1-6 animal model demonstrated that TRAIL inhibited tumor growth by suppressing tumor-infiltrating CD4+CD25+Foxp3+ regulatory T cells (Tregs) and augmenting tumor-specific CTL. These results provide further evidence for the immune regulatory function of TRAIL.
Materials and methods
Mice and tumor cell lines
BALB/c and C57BL/6 mice (5–6 weeks of age) were purchased from Vital River Experimental Animal Company (Beijing, China). DR5−/− mice were kindly provided by Dr. Tak Mak (University of Toronto). All mice were used in accordance with institutional guidelines for animal care approved by the committee on the use and care of animals, Chinese Academy of Medical Sciences. A murine hepatoma cell line Hepa1-6 established from C57L/J mice and hybridoma PC61.5 were purchased from American Type Culture Collection (Manassas, VA, USA) and maintained according to the recommendations of the supplier.
Antibodies
Anti-mouse CD3-FITC, CD4-FITC, CD25-PE and CD8α-PE antibody were purchased from BD Biosciences (San Diego, CA, USA). Anti-mouse CD25-APC, Foxp3-PE, CD4-PE, DR5-PE, TRAIL-PE, IFN-γ-FITC, Fc block antibody and various isotype controls were purchased from eBioscience (San Diego, CA, USA).
Determination of TRAIL-induced apoptosis in vitro
Bulk splenocytes from BALB/c or DBA/2 mice were depleted of red blood cells (RBCs) and incubated in 6-well plates with human TRAIL protein for 24 h, in the absence or presence of 10 μg/mL of TRAIL-neutralizing Ab (generated by our laboratory) or control Ab. Soluble recombinant endotoxin-free TRAIL was provided by Shenzhen Xinpeng Bioengineering Ltd. (Shenzhen, China). Cells were stained with anti-CD4-FITC, anti-CD25-APC, and 7-AAD followed by flow cytometry for dead cells (7-AAD+) on an Accuri C6 flow cytometer (Accuri Cytometers, Inc., Ann Arbor, MI). CD4+CD25− and CD4+CD25+ T cell populations were gated, respectively. Data were analyzed by using the CFlow sampler analysis software.
Subcutaneous tumor xenografts and assessment of growth
The Hepa1-6 cells (4 × 106) were injected into the subcutaneous (s.c.) tissue of the right dorsal flank of 6-week-old female C57BL/6 mice. Tumor growth was monitored three times a week and volume (V) was calculated using the formula V = 1/2 × length × (width)2. When the tumor volume was approximately 100 mm3 (about 10 days postinoculation), the animals were randomized into two groups. The TRAIL group was given six intratumoral injections of TRAIL at a dose of 15 mg/kg every 2 days, and the control group received normal saline. Mice either died or were culled when they reached ethical end points of palpable tumors >2 cm, more than 20 % weight loss or meeting any other ethical end point criteria for euthanasia. The overall survival of individual mice was calculated from birth to ethical end point or death from tumor. Survival distribution was estimated using the method of Kaplan–Meier.
Isolation of CD4+CD25+ T cells
Mouse CD4+CD25+ Treg cell populations were isolated from mouse spleen or lymph node using a CD4+CD25+ Treg isolation kit with the MidiMACS™ separator, according to the manufacturer’s protocols (Miltenyi, Bergisch Gladbach, Germany). Briefly, single cell suspension was suspended in PBS(pH 7.2) containing 0.5 % BSA and 2 mM EDTA; incubated with a biotin-antibody cocktail against CD8 (Ly2), CD11b (membrane-activated complex 1), CD45R (B220), CD49B (DX5), and Ter-119 for 10 min at 4 °C;and depleted of non-CD4+ T cells using microbead-conjugated, antibiotin mAb. In parallel, cells were stained with PE-labeled anti-CD25 mAb. The cell suspension was loaded on a LD column and placed in the magnetic field of a magnetic-activated cell sorting (MACS) separator; unlabeled splenocytes were then flowed through. The fraction remaining in the column was enriched for CD4+ T cells. To isolate CD4+CD25+ T cells, the PE-labeled CD25+ T cells in the enriched CD4+ T cell fraction were labeled magnetically with anti-PE microbeads and separated by MACS sorting. Positively sorted CD4+CD25+ T populations were always >95 % pure, as confirmed by flow cytometry (FCM).
Tumor-infiltrating lymphocyte preparation
Tumor tissue was cut into small fragments and digested in medium with 2 % FBS, 0.05 % collagenase IV, 0.005 % Dnase I for 1 h at 37 °C. The resulting cell suspension was passed over a 30 μm nylon filter and then overlaid on 40 %/70 % Percoll (Pharmacia. Uppsala, Sweden) gradient and centrifuged at 400 g. Leukocytes were recovered from the interphase.
In vivo CD25 depletion
The hybridoma PC61.5, secreting anti-CD25 mAb, was cultivated in DMEM medium (GIBCO). PC61.5 mAb was purified from culture supernatants with a protein G column (Pharmacia. Uppsala, Sweden) and dialyzed against PBS. Tumor-bearing mice were injected intraperitoneally (i.p.) with 0.5 mg anti-mouse CD25 or isotype control (Rat IgG1). Depletion of CD25+ cells was verified by flow cytometry.
Assessment of tumor-specific T cell immunity
The cytotoxic function of the activated T lymphocytes was determined by lactate dehydrogenase (LDH) cytotoxicity assay kit (Roche). Bulk splenocytes taken from mice after administration with TRAIL were restimulated with mitomycin C-treated tumor cells at a 10:1 ratio in the presence of 20 U/mL mouse IL-2 for 5 days. Stimulated splenocytes (used as effectors) were cocultured with tumor target cells for 12 h. CTL activity was analyzed following the manufacturer’s instructions. Briefly, after the effector cells cocultured with target cells in a 96-well round bottom plate, the plate was centrifuged and the supernatants were transferred to another flat-bottom enzyme-linked immunostaining assay (ELISA) plate. The LDH detection mixture (100 μL) was added to each well, and the plate was incubated at room temperature in the dark for 30 min. Absorbance was measured with an ELISA reader at 490 nm. The spontaneous release of LDH by target or effector cells was assayed by incubating target cells in the absence of effector cells and vice versa, and the maximum LDH release was determined by incubating the target cells in 1 % Triton X-100 in assay medium. The percentage of cell-mediated cytotoxicity was determined by the following equation: cytotoxicity (%) = [(mixture of effectors and targets-effector control)/(maximum- spontaneous)] × 100 %.
Statistical analysis
Kaplan–Meier tests were performed for survival data. For other data, results were expressed as mean values with standard deviation (SD). Student’s t-test was used to evaluate statistical significance. P values were considered to be statistic significant when <0.05.
Results
Hepa1-6 cells are resistant to TRAIL in vitro but sensitive in vivo
Mouse hepatoma cells Hepa1-6 were treated with various concentrations of TRAIL for 24 h. The viability of cells was determined by MTT assay. As shown in Fig. 1a, TRAIL cannot induce obvious cell death of Hepa1-6; even at the high concentration of 1,000 ng/ml compared with TRAIL-sensitive cells U251 human glioma cells, whose viability reduction was 45.49 ± 0.97 % at 10 ng/ml, and TRAIL-moderate sensitive 4T1 mouse mammary tumor cells, Hepa1-6 was a distinct TRAIL-resistant cell line. Consistent with the results of the MTT assay, procaspase-9 and procaspase-3 were not activated in Hepa1-6 cells after TRAIL treatment (Fig. 1b). The Hepa1-6 s.c. tumor mouse model was established (see Materials and Methods) to analyze the efficacy of TRAIL in vivo. To our surprise, the growth of Hepa1-6 s.c. tumor in mice was significantly suppressed in the group that received TRAIL intratumorally. As shown in Fig. 1c, the mean tumor volume on day 33 following Hepa1-6 cell inoculation, which was 607.24 ± 95.42 mm3 in the control group, was reduced to 221.84 ± 71.82 mm3 in the TRAIL treatment group, representing a substantial 45 % decrease (P < 0.01) in tumor growth (Fig. 1c). Day 33 tumor weights of TRAIL-treated mice were also reduced compared with tumors in the PBS control groups (0.49 ± 0.09 g vs. 0.75 ± 0.12 g, P < 0.01) (Fig. 1d). To confirm whether TRAIL could confer a survival benefit in this model, thirty mice injected in the same way with Hepa1-6 cells were randomized into two groups. Ten days later, these mice received an intratumoral injection of PBS or TRAIL. As shown in Fig. 1e, PBS injection group showed initial mortality on day 49, while the first mortality in the TRAIL-treated group was on day 73.
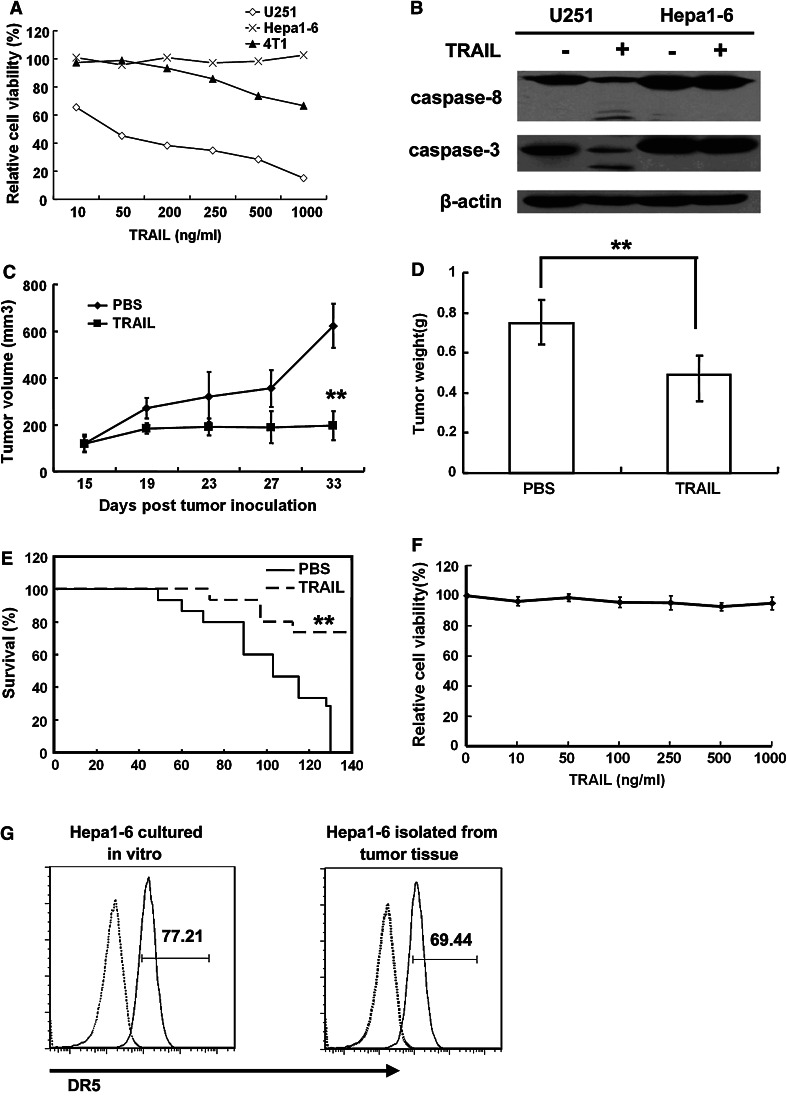
Hepa1-6 cells are resistant to TRAIL-induced apoptosis both in vitro and in vivo. a U251 and Hepa1-6 cells were treated with indicated concentrations of TRAIL for 24 h and cell viability was determined by MTT assay. b Western blot assay was performed to test the effect of TRAIL on proteolytic cleavage of caspase-9 and caspase-3 in U251 and Hepa1-6 cells. Cells were treated for 4 h with 100 ng/ml TRAIL. c, d Intratumoral administration of TRAIL inhibits the growth of s.c. Hepa1-6 tumors in mice. C57BL/6 mice were injected s.c. with 4 × 106 Hepa1-6 cells. On day 11, Hepa1-6 tumor-bearing mice were treated with 15 mg/kg TRAIL or the same volume of PBS by intratumoral injection once a day for 6 times (n = 5). The tumor size (c) and tumor weight (d) were determined. Replicates were averaged, and the error bars represent the SEM of five mice per time point (**P < 0.01). e Intratumoral administration of TRAIL prolongs the survival of mice with s.c. Hepa1-6 tumors. Hepa1-6 tumors were established in C57BL/6 mice on day 0. Mice receiving TRAIL survived for significantly longer periods than mice in PBS control groups (n = 15, **P < 0.01). f Ex vivo analysis of Hepa1-6 tumor cells. The Hepa1-6 cells separated from s.c. tumor were cultured and treated with indicated concentrations of TRAIL for 8 h, and cell viability was determined by MTT assay. g Expression of DR5 on Hepa1-6 cultured in vitro or isolated from tumor tissue was determined by FACS. Hepa1-6 cells were stained with PE-labeled anti-DR5 (solid line) or with PE-labeled Armenian hamster IgG as control (dotted line). The results from one representative experiment (of three) are shown
To confirm whether Hepa1-6 cells themselves become sensitive to TRAIL treatment in tumor-bearing mice, tumor tissue was resected. As shown in Fig. 1f, the Hepa1-6 cells separated from s.c. tumors remained resistant to TRAIL. Results from FACS analysis demonstrated that the level of DR5 expression on the surface of the cells freshly separated from s.c. tumor was identical to Hepa1-6 cell line cultured in vitro (Fig. 1g), clearly indicating that the Hepa1-6 sensitivity to TRAIL in vivo was not related to DR5 expression.
Intratumoral injection of TRAIL leads to CD4+CD25+Foxp3+ Treg apoptosis
To understand the mechanism behind the different sensitivities of Hepa1-6 cells to TRAIL in vitro and in vivo, tumor-infiltrating immune cells from Hepa1-6 tumors were isolated and identified by flow cytometry. As shown in Fig. 2a, b, the populations of tumor-associated macrophages (TAMs) and myeloid-derived suppressor cells (MDSC) were not changed, while TRAIL treatment caused a significant decrease in the number of intratumoral CD4+CD25+ T cells and an increase in the number of CD8+ T cells (Fig. 2c, d, P < 0.05). To clearly define intratumoral CD4+CD25+ T cells, we evaluated the intracellular expression of Foxp3 in these cells. As shown in Fig. 2e, above 85 % of these cells co-express Foxp3, indicating that the populations consist of Treg. A tumor-infiltrating lymphocyte suspension was prepared from the s.c. tumor treated with TRAIL and assessed for DR5 expression in CD4+CD25+Foxp3+ T cells. As shown in Fig. 3a, DR5 expression was increased in the tumor-infiltrating CD4+CD25+Foxp3+ T cells compared with naïve CD4+CD25+Foxp3+ T cells isolated from spleen of mice without tumors. Interestingly, no DR5 expression was detected on the surface of CD4+CD25+Foxp3+ T cells from spleen and lymph node of tumor-bearing mice (data not shown).

Intratumoral injection of TRAIL decreased intratumoral CD4+CD25+Foxp3+ Tregs. Hepa1-6 tumors were established in C57BL/6 mice on day 0. The mice were injected intratumorally with TRAIL at indicated concentration beginning on day 11 once a day for 6 times. After the last injection, TAMs in the single cell suspension of tumor tissue were quantified by staining with anti-F4/80-PE. MDSCs were quantified by staining with anti-CD11b-FITC and anti-Gr1-APC (a), (b). Results from one experiment representative (of four) are shown. Columns, mean of data from four mice; bars, SD. c,d CD4+CD25+ T cells among tumor-infiltrating lymphocytes (TILs) dissociated from the tumor tissue were stained with anti-CD4-FITC and anti-CD25-APC, gated on CD4+ T cells. CD3+CD8+ cells were stained with anti-CD3-FITC and anti-CD8-PE and followed by FACS. Results from one experiment representative (of four or five) are shown. Columns, mean of data from four or five mice (*P < 0.05). e TILs were dissociated from the tumor tissue; cells were first stained with anti-CD4-FITC and anti-CD25-APC and then stained intracellularly with anti-Foxp3-PE. Cells were analyzed by flow cytometry and were gated on CD4+ T cells
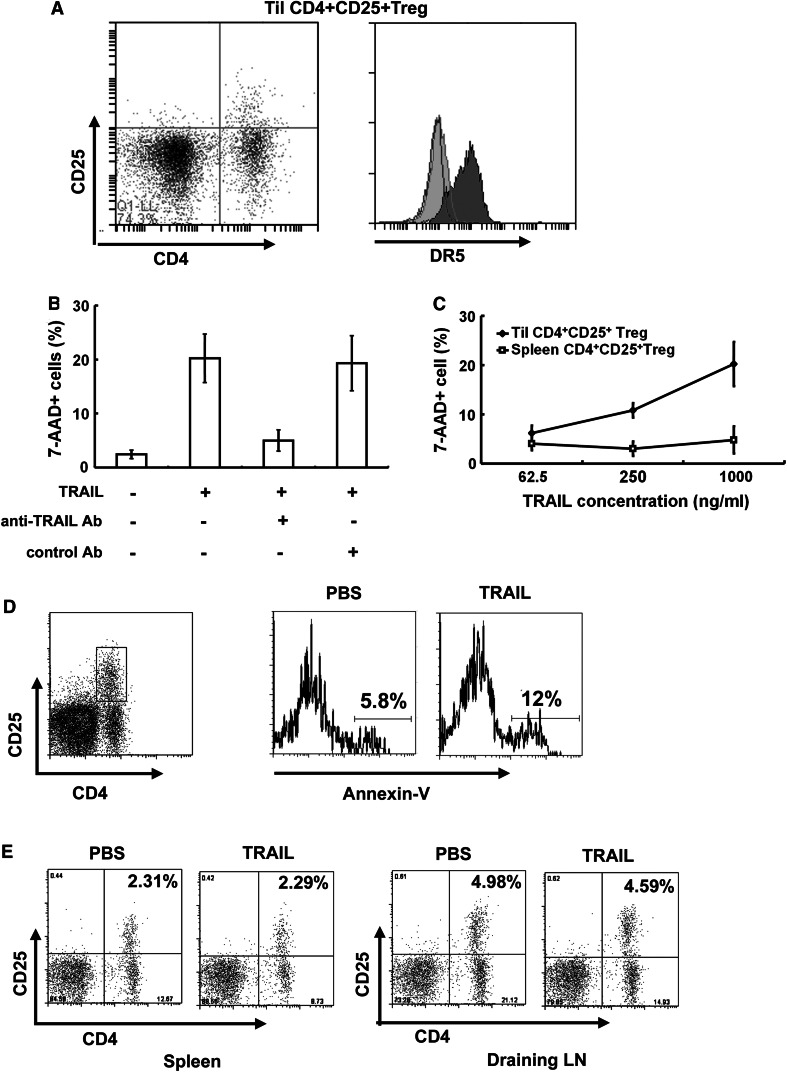
TRAIL induced intratumoral-activated CD4+CD25+ Treg apoptosis. a FACS analysis of DR5 expression on freshly isolated CD4+CD25+ cells from murine spleen and TILs. Cells were stained with anti-CD4-FITC, anti-CD25-APC and anti-DR5-PE and analyzed by flow cytometry, gated on CD4+D25+ population. Open area, isotype control; Light gray filled area, CD4+CD25+ cells from murine spleen; Dark gray filled area, CD4+CD25+ cells from tumor-infiltrating lymphocytes. Results from one experiment representative of three were shown. b Lymphocytes from tumor issue were incubated for 24 h with 100 ng/ml of TRAIL, alone or together with 10 μg/ml of TRAIL-neutralizing Ab or control Ab. Cells were stained with anti-CD4-PE, anti-CD25-APC and 7-AAD and then examined by flow cytometry, gated on CD4+CD25+ populations. Columns, mean of three independent experiments; bars, SD. c Lymphocytes from spleen or tumor issue were incubated for 24 h with indicated concentrations of TRAIL. The cells were quantified as above (b). Plots are mean of three independent experiments. d Hepa1-6 tumor was established in C57BL/6 mice on day 0. The mice were injected intratumorally with TRAIL at indicated concentration on day 11 once a day for 6 times. After the last injection, TILs were dissociated from the tumor tissue. Apoptosis of CD4+CD25+Treg was determined by staining the cells with anti-CD4-PE, anti-CD25-APC and Annexin V-FITC and then analyzing by flow cytometry, gated on CD4+ CD25+ T cells. The Annexin V+ CD4+CD25+ fraction of the gated cells was concerned as apoptotic CD4+CD25+ Treg cells. Results from one experiment representative of four were shown. e CD4+CD25+ Treg cells in cell suspensions from spleen and draining lymph node were quantified as above (d). Results from one experiment representative of four were shown
The susceptibility of CD4+CD25+Foxp3+ Tregs to TRAIL-mediated apoptosis in vitro was determined. A tumor-infiltrating lymphocyte suspension prepared from the s.c. tumor was directly incubated with TRAIL, and the cell viability in each subset of T cells was determined by staining with anti-CD4, anti-CD25, and 7-AAD followed by flow cytometry gating for CD4+CD25+ population. As shown in Fig. 3b, c, TRAIL effectively induced cell death in CD4+CD25+Foxp3+Treg. The specificity of TRAIL was verified by the prevention of cell death by TRAIL-neutralizing Ab, but not by control Ab. However, CD4+CD25+Foxp3+ T cells from the spleen of the tumor-burden mice were not sensitive to TRAIL (Fig. 3c). These results indicate that tumor-infiltrating CD4+CD25+Foxp3+ Tregs are sensitive to TRAIL-mediated apoptosis in vitro.
We next assessed the susceptibility of these intratumoral CD4+CD25+ Foxp3+ Tregs to TRAIL in vivo. After intratumoral administration of TRAIL, the tumor-infiltrating lymphocyte suspension was prepared from the injected tumors 12 h after the last administration. The presence of CD4+CD25+Foxp3+ Treg undergoing apoptosis in the cell suspension was evaluated by flow cytometry. Compared with PBS-injected control tumors, TRAIL-treated tumors contained significantly more CD4+CD25+Foxp3+ Tregs undergoing apoptosis, as assessed by their Annexin V+ status, demonstrating that TRAIL administration increases apoptosis of intratumoral CD4+CD25+Foxp3+ Tregs in vivo.
We further determined whether intratumoral TRAIL treatment could result in systemic depletion of CD4+CD25+Foxp3+ Tregs. After TRAIL treatment, cell suspensions prepared from the tumor draining lymph nodes and spleen were each quantified for live CD4+CD25+Foxp3+ Tregs. As shown in Fig. 3e, TRAIL treatment did not decrease CD4+CD25+Foxp3+ Tregs in the spleen or tumor draining lymph nodes. Collectively, these data indicate that intratumoral TRAIL protein treatment leads to local but not systemic decreases in CD4+CD25+Foxp3+ Tregs.
Tregs play an important role in TRAIL-induced anti-Hepa1-6 tumor activity
Although the experiments above establish the contribution of local CD4+CD25+ Foxp3+ Tregs depletion to antitumor activity, they do not exclude the possibility that TRAIL may enhance the antitumor efficacy through other mechanisms. To this end, tumor-infiltrating CD4+CD25+Foxp3+ Tregs were depleted with anti-CD25 mAb to further explore the mechanism of TRAIL function. As shown in Fig. 4a, anti-CD25 mAb was purified from culture supernatants of the PC61.5 hybridoma (ATCC, Bethesda, MD, USA) and verified by positive staining of ConA-activated mouse splenocytes. Anti-CD25 mAb (0.5 mg) was i.p. injected into the Hepa1-6 tumor-bearing mice two days before TRAIL treatment. As shown in Fig. 4b, tumor growth was significantly suppressed in the Treg depletion group (PC61), and additional administration of TRAIL (PC61 + TRAIL) did not decrease the Hepa1-6 tumor size. These results suggest that depletion of tumor-infiltrating CD4+CD25+Foxp3+ Tregs could abolish the antitumor activity of TRAIL. Furthermore, administration of TRAIL could not inhibit Hepa1-6 tumor growth in DR5−/− mice (Fig. 4c). In conclusion, we demonstrate further that TRAIL-induced Treg apoptosis plays an important role in TRAIL-induced anti-Hepa1-6 tumor activity.

CD4+CD25+ Tregs play an important role in TRAIL-induced anti-Hepa1-6 tumor efficacy. a Staining of 3 day’s ConA-activated mouse splenocytes with anti-mouse CD25 antibody. ConA-activated mouse splenocytes stained with control rat IgG1 (open area) or 0.25 μg of purified anti-mouse CD25 (gray filled area) followed by anti-rat IgG FITC. Splenocytes without ConA stimulation were used as control (black filled area). Results from one experiment representative of three were shown. b Hepa1-6 tumor was established in C57BL/6 mice on day 0. For Treg depletion, anti-CD25 mAb was injected i.p. before TRAIL administration. Two days after the anti-CD25 mAb injection, Hepa1-6 tumor-bearing mice were treated with 15 mg/kg TRAIL or same volume of PBS by intratumoral injection once a day for 5 times (n = 5). Plots are mean of five mice at each time point
CTL response was enhanced in Hepa1-6 tumors
Previous studies suggest that Treg cells suppress proliferation, IFN-γ secretion and cytolytic function of CD8+ T cells [13, 14]. In the present study, we have shown increased CD8+ T cells in Hepa1-6 s.c. tumors following TRAIL injection (Fig. 2c). CD8+ T cell IFN-γ secretion and cytolytic function were further analyzed by flow cytometry and cytolytic assays. The tumor-infiltrating lymphocytes were stimulated with 50 ng/ml PMA and 1 μg/ml ionomycin for 4 h in the presence of 10 μg/ml BFA. As shown in Fig. 5a, IFN-γ-positive CD8+ cells increased from 1.22 % in the control group to 6.33 % in the TRAIL-treated group. Splenocytes from TRAIL-treated tumor-bearing mice showed marked CTL activity against the Hepa1-6 tumor cells, and the CTL activity was Hepa1-6 tumor cell-specific, as lysis of unrelated control 4T1 tumor cells was significantly lower. Meanwhile, splenocytes from tumor-bearing mice without treatment showed less CTL activity against the Hepa1-6 tumor cells than splenocytes from TRAIL-treated tumor-bearing mice (Fig. 5b). These data demonstrate that CTL response was enhanced in Hepa1-6 tumor injected with TRAIL.

CTL response was enhanced after intratumoral TRAIL injection. a Intratumoral injection of TRAIL led to the increase in IFN-γ + cells. TILs were incubated with PMA and ionomycin followed by staining with anti-IFN-γ and anti-CD8 antibody. Numbers shown are the percentages of IFN-γ-positive cells in CD8+ T cells. b Bulk splenocytes from TRAIL-cured or without TRAIL-treated mice were restimulated for 5 days with mitomycin C-treated tumor cells at a 10:1 ratio in the presence of IL-2. Stimulated splenocytes (effectors) were incubated for 12 h with Hepa1-6 (specific target) or 4T1 (nonspecific target) tumor cells at indicated effector/target (E:T) ratios. CTL activity was determined by lactate dehydrogenase cytotoxicity assay kit. Plots are mean of three independent experiments (solid line with diamond E: splenocytes from TRAIL-treated Hepa1-6 tumor-burden mice, T: Hepa1-6; solid line with square E: splenocytes from Hepa1-6 tumor-burden mice without TRAIL treatment, T: Hepa1-6; solid line with triangle E: splenocytes from TRAIL-treated Hepa1-6 tumor-burden mice, T: 4T1)
Discussion
The clinical safety of TRAIL or TRAIL receptor targeting agents and their ability to mediate apoptosis in cancer cells have made them potential therapeutics [1–3]. The anticancer activity of TRAIL was initially thought to be a result of the preferential expression of DR4 and DR5 in cancerous tissue, thereby conferring enhanced sensitivity to death compared with normal tissue that preferentially expressed TRAIL decoy receptors. However, many tumor cells intrinsically acquire resistance to TRAIL-induced cell death, which limits the clinical application of TRAIL. In this study, we found that murine Hepa1-6 hepatoma cells are resistant to TRAIL in vitro; however, we also found that TRAIL significantly suppresses the growth of Hepa1-6 s.c. tumor in mice and prolongs the survival of tumor-burden mice. This result enabled us to investigate the immunological function of TRAIL in a TRAIL-resistant animal cancer model. The resulting data demonstrated that the soluble recombinant TRAIL suppressed liver tumor growth by inducing tumor-infiltrating CD4+CD25+Foxp3+ Treg apoptosis. These results represent further supporting evidences for the immune regulatory function of TRAIL and enable the exploration of a novel strategy for cancer therapy by TRAIL-induced CD4+CD25+Foxp3+ Treg apoptosis.
Tumor immunity is in part autoimmunity, and the mechanisms for maintaining immunological self-tolerance may hinder effective tumor immunity. Recent studies indicate that Tregs play a critical role in suppressing immune responses and inducing immune tolerance to cancer and infectious diseases. Tregs can inhibit tumor-specific CD8+ and CD4+ T cell effector functions through incompletely understood mechanisms, including cell–cell contact and/or the production of soluble factors such as IL-10 or TGF-β [15–17]. Recent studies showed that CD4+ Tregs are capable of suppressing priming and activation but also cytotoxicity of activated antigen-specific CD8+ T cells effector sites other than a growing tumor [18]. In addition, Tregs also inhibit the function of NK cells, B cells and other immune cells [13, 14, 19, 20]. Large numbers of CD4+CD25+ Tregs are present in tumors and draining lymph nodes in tumor-bearing rodents and in cancer patients. Importantly, the CD4+CD25+ Tregs present in tumors decrease the ratios of CD8+ T cells to CD4+CD25+ Tregs in those tumors and correlate with poor prognosis in patients with breast cancer [21], gastric cancer [22] and ovarian cancer [23]. Therefore, depletion of CD4+CD25+ Tregs or attenuation of their suppressive function may enhance antitumor immunity. For rapid clinical translation of this concept, some mAbs that are specific for cell surface molecules predominantly expressed by Tregs or that are specifically able to modulate Treg function have been developed [24, 25]. Small molecules such as CpG and cyclophosphamide, which affect Treg function or survival, are also able to enhance tumor immunity [26, 27]. Denileukin diftitox, which is a recombinant fusion protein consisting of IL-2 and diphtheria toxin, has been approved by the FDA (USA) for treating subcutaneous T cell leukemia/lymphoma by depleting CD4+CD25+ Treg cells [28]. Interestingly, it has recently been shown in vitro that Tregs are highly susceptible to FasL-mediated apoptosis [29], and depleting intratumoral CD4+CD25+ Tregs by FasL protein transfer enhances the therapeutic efficacy of adoptive T cell transfer [30]. In the present study, we observed high susceptibility to TRAIL receptor-mediated apoptosis both in CD4+CD25+Foxp3+ Tregs cultured in vitro and in intratumoral CD4+CD25+Foxp3+ Tregs in vivo, providing further evidence for this direction.
TRAIL can increase the number of mTg-specific CD4+CD25+ Tregs, inhibit autoimmune responses and prevent the progression of experimental autoimmune thyroiditis (EAT) [31], revealing a novel mechanism by which TRAIL could inhibit autoimmune disease. We report here for the first time that TRAIL can induce CD4+CD25+Foxp3+ Treg apoptosis in the mouse liver tumor model. Resting Tregs from spleens of mice without tumor were resistant to TRAIL. Furthermore, no DR5 expression was detected on the surface of naïve CD4+CD25+Foxp3+ Treg, but DR5 expression was increased in the tumor-infiltrating CD4+ CD25+ Foxp3+ Tregs. However, DR5 expression in the CD4+CD25+ Foxp3+ Treg stimulated by CD3/CD28 antibody in vitro was not detectable and these activated CD4+CD25+ Foxp3+ Tregs were not sensitive to TRAIL (data not shown). As previously reported, the tumor itself or tumor microenvironmental factors could activate Tregs [32]. Although the levels of Tregs in the peripheral blood of patients with cancer were not significantly higher than in healthy donors, Tregs in patients with cancer had significantly greater suppressive functionality than Tregs from healthy donors [33]. In our model, DR5 expression increased only in the tumor-infiltrating activated CD4+ CD25+Foxp3+ Tregs, indicating that an as-yet unclear mechanism enhanced DR5 expression in CD4+CD25+Foxp3+ Tregs and made them sensitive to TRAIL. Clarifying the underlying molecular mechanisms may allow for the development of new cancer therapy strategies.
In addition to depleting Tregs, TRAIL may influence tumor microenvironment through other mechanisms to inhibit the growth of Hepa1-6 tumor. We did not detect immune cells in tumors other than macrophages, MDSC, CD4+ T cells, CD8+ T cells and CD4+CD25+ Foxp3+ Tregs. Still, the result of CD4+CD25+Foxp3+ Treg depletion experiments can exclude to some extent other mechanisms.
In summary, we have demonstrated that TRAIL induces apoptosis of tumor-activated CD4+CD25+Foxp3+ Tregs possibly due to their enhanced DR5. This study is expected to provide further insights into the physiological function of TRAIL and broaden our knowledge of TRAIL in the context of cancer therapy.
Acknowledgments
This work was supported in part by the Natural Science Foundation (No. 30772495, 30972699, 91029375, 30972684) and the State Key Basic Research Program (No. 2007CB507404).
Conflict of Interest
The authors declare that they have no conflict of interest.
Contributor Information
Juan Shi, Phone: +86-10-6529-6409, Fax: +86-10-6510-5102, Email: moc.oohay@ttnaujihs.
Dexian Zheng, Phone: +86-10-6529-6409, Fax: +86-10-6510-5102, Email: nc.ude.cmup@xdgnehz.
References
Articles from Cancer Immunology, Immunotherapy : CII are provided here courtesy of Springer
Citations & impact
Impact metrics
Citations of article over time
Article citations
TRAIL promotes the polarization of human macrophages toward a proinflammatory M1 phenotype and is associated with increased survival in cancer patients with high tumor macrophage content.
Front Immunol, 14:1209249, 21 Sep 2023
Cited by: 5 articles | PMID: 37809073 | PMCID: PMC10551148
Dose-related immunomodulatory effects of recombinant TRAIL in the tumor immune microenvironment.
J Exp Clin Cancer Res, 42(1):216, 22 Aug 2023
Cited by: 2 articles | PMID: 37605148 | PMCID: PMC10464183
Bone Marrow Mesenchymal Stromal Cell-mediated Resistance in Multiple Myeloma Against NK Cells can be Overcome by Introduction of CD38-CAR or TRAIL-variant.
Hemasphere, 5(5):e561, 21 Apr 2021
Cited by: 12 articles | PMID: 33898931 | PMCID: PMC8061681
Regulatory T Cell-Derived TRAIL Is Not Required for Peripheral Tolerance.
Immunohorizons, 5(1):48-58, 22 Jan 2021
Cited by: 2 articles | PMID: 33483333 | PMCID: PMC8663370
Mechanisms of Apoptosis Resistance to NK Cell-Mediated Cytotoxicity in Cancer.
Int J Mol Sci, 21(10):E3726, 25 May 2020
Cited by: 49 articles | PMID: 32466293 | PMCID: PMC7279491
Review Free full text in Europe PMC
Go to all (21) article citations
Similar Articles
To arrive at the top five similar articles we use a word-weighted algorithm to compare words from the Title and Abstract of each citation.
[Changes of regulatory T cell number in hepatocellular carcinoma-bearing mice and its relationship with tumor growth].
Zhonghua Zhong Liu Za Zhi, 29(5):342-345, 01 May 2007
Cited by: 0 articles | PMID: 17892128
Tumor necrosis factor-related apoptosis-inducing ligand inhibits experimental autoimmune thyroiditis by the expansion of CD4+CD25+ regulatory T cells.
Endocrinology, 150(4):2000-2007, 13 Nov 2008
Cited by: 32 articles | PMID: 19008314 | PMCID: PMC2659286
Blockade of TGF-β signaling to enhance the antitumor response is accompanied by dysregulation of the functional activity of CD4+CD25+Foxp3+ and CD4+CD25-Foxp3+ T cells.
J Transl Med, 17(1):219, 09 Jul 2019
Cited by: 26 articles | PMID: 31288845 | PMCID: PMC6617864
Immunosuppressive drugs on inducing Ag-specific CD4(+)CD25(+)Foxp3(+) Treg cells during immune response in vivo.
Transpl Immunol, 27(1):30-38, 14 May 2012
Cited by: 32 articles | PMID: 22613676