Abstract
Free full text

Editor's choice
DNase Inhibits Gardnerella vaginalis Biofilms In Vitro and In Vivo
Abstract
Bacterial vaginosis is a highly prevalent and poorly understood polymicrobial disorder of the vaginal microbiota, with significant adverse sequelae. Gardnerella vaginalis predominates in bacterial vaginosis. Biofilms of G. vaginalis are present in human infections and are implicated in persistent disease, treatment failure, and transmission. Here we demonstrate that G. vaginalis biofilms contain extracellular DNA, which is essential to their structural integrity. Enzymatic disruption of this DNA specifically inhibits biofilms, acting on both newly forming and established biofilms. DNase liberates bacteria from the biofilm to supernatant fractions and potentiates the activity of metronidazole, an antimicrobial agent used in the treatment of bacterial vaginosis. Using a new murine vaginal colonization model for G. vaginalis, we demonstrate >10-fold inhibition of G. vaginalis colonization by DNase. We conclude that DNase merits investigation as a potential nonantibiotic adjunct to existing bacterial vaginosis therapies in order to decrease the risk of chronic infection, recurrence, and associated morbidities.
(See the editorial commentary by Marrazzo on pages 1481–3.)
Bacterial vaginosis is a common polymicrobial disorder of the vaginal microbiota and can have adverse outcomes, such as ascending reproductive tract infections; enhanced acquisition of sexually transmitted diseases, including human immunodeficiency virus infection; and preterm birth [1–4]. Both a defined etiology and optimal treatment strategies for bacterial vaginosis have remained elusive [5].
Gardnerella vaginalis, a gram-positive coccobacillus, is a constituent of the vaginal microbiota that grows to high concentrations in the bacterial vaginosis milieu [6]. While it may be detected in women without bacterial vaginosis [7], quantitative polymerase chain reaction studies have demonstrated that high levels of G. vaginalis are associated with bacterial vaginosis diagnoses [8]. G. vaginalis produces vaginolysin, a cholesterol-dependent cytolysin that may be important in the pathogenesis of and local immune responses to bacterial vaginosis [9], and the organism is efficient at biofilm growth [10, 11]. G. vaginalis biofilms have been demonstrated to be present on the surface of the vaginal epithelium in women with, but not women without, bacterial vaginosis [12]. Such biofilms may have relevance to persistence or recurrence of bacterial vaginosis, as organisms in the biofilm state are less susceptible to antibiotic therapy [13]. The G. vaginalis biofilm has been hypothesized to be relevant to bacterial vaginosis transmission [14]. However, the factors important in the formation and maintenance of G. vaginalis biofilms remain incompletely characterized.
Extracellular DNA (eDNA) has been shown to be a factor in the structural stability of biofilms in a variety of bacterial species [15–17]. Here we show that eDNA plays an important role in the establishment and maintenance of G. vaginalis biofilms. Enzymatic targeting of eDNA represents a new strategy for therapy or prevention of bacterial vaginosis that may complement existing antimicrobial approaches.
METHODS
Bacterial Strains and Culture Techniques
G. vaginalis strains 14 018 and 49 145 were purchased from ATCC. Strain ARG3 has been previously described [18]. Strain ARG37 is a streptomycin-resistant, mouse-passaged derivative of strain 14 018. For in vitro experiments, strains from frozen stocks (temperature, –80°C) were cultured on human blood bilayer–Tween agar (Becton Dickinson) and grown at 37°C in 5% CO2 for 48 hours. Single colonies were then inoculated into biofilm medium (ie, brain heart infusion broth supplemented with 0.3% glucose, 0.3% starch, and 1 µg/mL amphotericin [10]) or GV medium (brain heart infusion broth supplemented with 10% fetal bovine serum [HyClone], 5% Fildes enrichment [Remel], and 1 µg/mL amphotericin) and grown overnight without shaking at 37°C in 5% CO2. For determination of growth curves, G. vaginalis strains were grown planktonically in GV medium at 37°C in 5% CO2 in the presence of DNase or vehicle control. The OD595 was assessed at the indicated intervals.
For experiments involving DNA quantification during growth curves, 1-mL aliquots were removed at the indicated intervals and centrifuged. Cell pellets were discarded, and supernatants were retained. DNA was extracted from the supernatant portion with chloroform:phenol:isoamyl alcohol (ratio, 25:24:1), precipitated with 100% ethanol, washed with 70% ethanol, and resuspended in 100 µL of phosphate-buffered saline prior to quantification by UV spectroscopy.
Biofilm Assays
G. vaginalis cultures were grown in biofilm medium to an OD600 of 0.6 and diluted at a ratio of 1:10 in fresh biofilm medium. A 200-µL final volume was added to sterile polystyrene 96-well plates (BD Falcon) and incubated at 37°C in 5% CO2 for 24 hours. Biofilms were washed twice with sterile phosphate-buffered saline, air-dried for 1 hour, and stained with 200 µL of safranin (Fisher). Excess stain was removed, and the biofilms were washed twice with sterile phosphate-buffered saline and photographed using a flatbed scanner prior to being solubilized in 200 µL of 33% acetic acid. The OD462 of the solubilized stain was measured on a computerized plate reader (Tecan), and percentage values for treatment conditions were normalized to wells with untreated bacteria (100%). Colony-count experiments were identical to biofilm assays, except that supernatants and residual biofilms were assessed by quantitative culture.
For experiments using DNase on newly forming biofilms, the final volume of 200 µL/well was composed of 180 µL of culture with 20 µL of purified bovine pancreas DNase I (Sigma) or vehicle control (HBSS; Mediatech). Heat inactivation of DNase was for 15 minutes at 70°C. Experiments on established biofilms were identical to those on newly formed biofilms, except that a 200-µL volume of culture was first incubated for 24 hours in 96-well plates. After 24 hours, excess medium was removed, the remaining biofilm was washed twice with sterile phosphate-buffered saline, and fresh medium with or without DNase and/or metronidazole was added at the indicated concentrations. Biofilms were then incubated for another 24 hours in the same conditions, and the remainder of the experiment was conducted as described above.
Microscopy
Overnight cultures were prepared as described above. These were diluted 10-fold into glass-bottomed dishes (Mattek) or 8-well chamber slides (Nunc). Treatments were with DNase (final concentration, 100 µg/mL) or vehicle control. Bacteria were incubated for 24 hours, excess medium was removed, and the biofilms were air-dried for 1 hour. The biofilms were then stained with DAPI or propidium iodide and examined with an inverted fluorescent microscope (Zeiss AxioObserver).
Murine Vaginal Colonization Model
Experiments using mice were approved by the Columbia University review board. Eight-week-old female C57BL/6J mice were administered streptomycin 5 g/L in drinking water, beginning 2 days prior to inoculation (ie, day –2). On days –2 and 0, estrus cycles were synchronized by subcutaneous injection with 500 µg of estradiol [19]. On days 0 and 1, mice were inoculated intravaginally with ARG37 (5 × 106 colony-forming units) and DNase (final concentration, 100 µg/mL) or vehicle control in 5% gelatin. Vaginal swabs were collected on day 2 for quantitative culture on human blood bilayer–Tween agar with 50 µg/mL streptomycin.
Data Presentation and Statistics
Results for in vitro experiments were plotted as means ± standard error of the mean and analyzed for statistical significance via analysis of variance with the Tukey posttest, using Prism 5.0 software (GraphPad). For the murine colonization model, groups of mice (5 per group) were plotted as the colonization density of individual mice, and medians were compared using the Mann-Whitney test. The heat map for the checkerboard experiment was constructed in Excel (Microsoft).
RESULTS
G. vaginalis Forms Biofilms That Contain eDNA and Are Inhibited by DNase
Fluorescence microscopy revealed that G. vaginalis strains ATCC 49 145 and ARG3 grow in thick, 3-dimensional microcolonies on glass surfaces (Figure (Figure11A and and11C). Staining of individual bacteria and large areas outside the organisms was evident, likely corresponding to an eDNA-containing biofilm matrix. In contrast, growth in the presence of purified DNase led to marked inhibition of the biofilm mode of growth (Figure (Figure11B and and11D), with individual organisms visible and only rare and significantly smaller microcolonies observed. There was greatly reduced staining of matrix, suggestive of decreased eDNA due to digestion by DNase.

Gardnerella vaginalis forms 3-dimensional biofilms that are inhibited by DNase. G. vaginalis strain 49 145 was grown on glass supports for 24 hours and treated with vehicle control (A and C) or with DNase (B and D). Two-dimensional images of DAPI-stained biofilms (A and B) or 3-dimensional reconstruction from serial z-stack images or propidium iodide–stained biofilms (C and D) demonstrate both bacteria-associated and extracellular DNA. Similar results were obtained with G. vaginalis strain ARG3 (data not shown).
eDNA Is Released During G. vaginalis Growth
G. vaginalis culture in vitro led to a long lag phase (duration, approximately 10 hours), followed by exponential growth (Figure (Figure2).2). We examined the timing of DNA release in G. vaginalis cultures over time by extracting and precipitating DNA from growing cultures. The DNA was extracted from cell-free supernatants after centrifugation to optimize isolation of eDNA only. Growth was in large flasks to allow enough volume for removal of supernatant for DNA extraction, but shaking was not performed; biofilm growth on the flask bottom and walls was evident. We noted that eDNA release peaked in the early exponential-growth phase (Figure (Figure2),2), consistent with autolysis or active release, rather than bacterial lysis, in the stationary-growth phase.

Gardnerella vaginalis releases extracellular DNA (eDNA) in early stationary-growth phase. Strain 49 145 was incubated for 18 hours, with OD595 recorded as a measure of bacterial growth (; left axis). eDNA was quantified by spectroscopy from the cell-free supernatant after extraction and precipitation (•; right axis). Trend lines are plotted for the OD curve (dashed line) and eDNA concentrations (solid line). The experiment was repeated 3 times; a representative experiment is shown.
DNase Digestion of eDNA Prevents Formation of G. vaginalis Biofilms
We used quantitative in vitro assays to assess the effect of DNase on newly forming and established G. vaginalis biofilms. The addition of exogenous DNase decreased de novo biofilm formation by G. vaginalis in a dose-dependent manner (Figure (Figure33A). The maximal effect was seen with a concentration of 100 µg/mL, which resulted in approximately 80% reduction in biofilm biomass (P < .001). Heat-inactivation eliminated the biofilm inhibitory property of DNase, with no significant difference between biofilms that were and those that were not treated with inactivated DNase untreated (Figure (Figure33B). This result implicates the enzymatic activity of DNase in the biofilm inhibitory effect. Importantly, addition of purified DNase did not affect planktonic growth of G. vaginalis (Figure (Figure33C), consistent with inhibition of biofilm formation, rather than bacterial killing, as the likely mechanism of action.
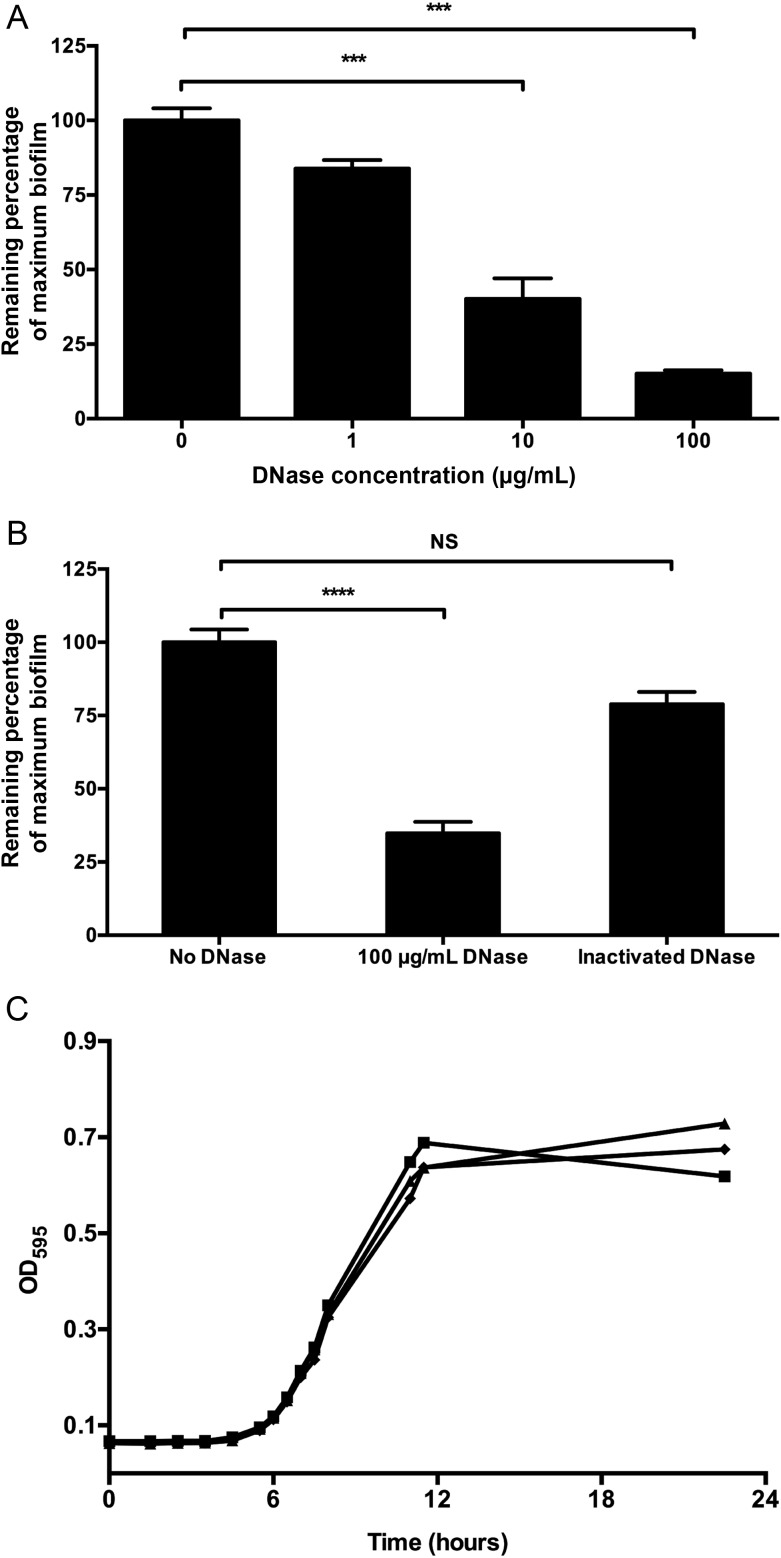
DNase inhibits the de novo formation of Gardnerella vaginalis biofilms. A, Strain 49 145 was incubated with DNase at the indicated concentrations or in vehicle control for 24 hours, and biofilm biomass was quantified by the safranin assay. Normalization (100%) was to control-treated G. vaginalis. B, Heat inactivation of DNase abrogates its biofilm inhibiting properties. C, DNase at various concentrations (, vehicle control; ♦, 8 µg/mL;
, 200 µg/mL) does not inhibit planktonic growth of G. vaginalis over 24 hours. ***P < .001; ****P < .0001.
DNase Disrupts Established G. vaginalis Biofilms
Similar to its effect on forming biofilms, DNase also disrupted previously established biofilms (Figure (Figure44A). The maximal effect (approximately 50% inhibition at 100 µg/mL DNase) was less than that observed when the enzyme was added prior to biofilm formation, possibly reflecting decreased penetration of active enzyme into the biofilm. The effect was rapid, with maximal inhibition occurring within 15 minutes of exposure (Figure (Figure44B). Inhibition after 15 minutes of treatment was comparable to that observed after 24 hours of DNase exposure (Figure (Figure44A and and44B). Consistent with the finding that DNase did not inhibit planktonic bacterial growth (Figure (Figure33C), we found that DNase treatment of established biofilms did not significantly affect overall bacterial number but did liberate a substantial number of organisms from the biofilm fraction into the supernatant (Figure (Figure44C).
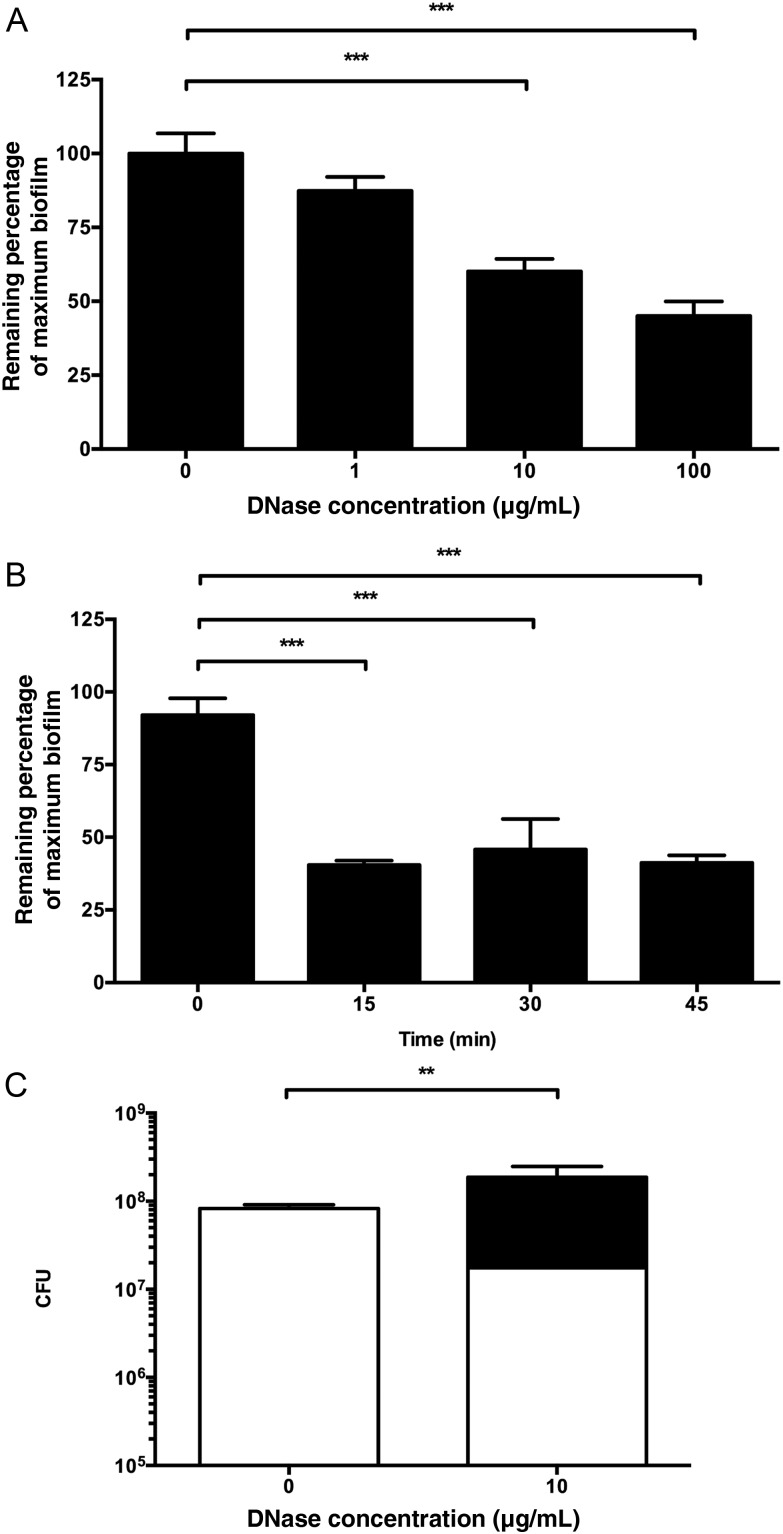
DNase disrupts previously established biofilms. A, Gardnerella vaginalis biofilms were grown for 24 hours prior to treatment with DNase or vehicle control. B, A total of 100 µg/mL of DNase was active on biofilms within 15 minutes. C, G. vaginalis density in biofilm fraction (white) and supernatant fraction (black). Statistical comparison is for supernatant organisms. Total organisms were not statistically significantly different. **P < .01; ***P < .001.
DNase Potentiates the Effect of Antibiotics on G. vaginalis Biofilms
Because bacteria within biofilms have decreased sensitivity to antibiotic killing [20], we hypothesized that DNase-mediated release of biofilm-associated organisms might potentiate the effects of antibiotic therapy. Prior work involving gram-negative uropathogens and Staphylococcus aureus has demonstrated additive or synergistic interactions between antimicrobials and DNase [21, 22].
To best approximate a physiologically relevant condition, we used a range of concentrations of DNase and metronidazole, an antibiotic used in the treatment of bacterial vaginosis in clinical settings, in a checkerboard-type assay examining effects on established G. vaginalis 49 145 biofilms. At the highest concentrations of antibiotic or DNase tested, the effect of one agent overwhelmed any potential additional effect seen with the other. However, at lower concentrations, the combination of DNase and metronidazole led to greater biofilm disruption than either agent alone (Figure (Figure5).5). The magnitude of these effects was limited, but the potential to enhance the efficacy of low concentrations of antibacterial agents against organisms in biofilms is potentially relevant to the therapeutic setting, in which it may be difficult to achieve high concentrations of single agents at mucosal surfaces.

Interaction of DNase and metronidazole on preformed Gardnerella vaginalis biofilms. DNase was added at 167 µg/mL final concentration (bottom row), with 10-fold dilutions in each subsequent row above (top row, vehicle control). Metronidazole was added at 1.67 mg/mL final concentration (right column), with 5-fold dilutions in each subsequent column to the left (left column, vehicle control). The remaining percentage of the maximum biofilm was calculated as in prior figures, and a heat map (red, >75% remaining; green, 50%–75%; blue, <50%) was constructed.
DNase Decreases Colonization Density of G. vaginalis In Vivo
There are no previously published reports of a murine model for G. vaginalis vaginal colonization. We used a spontaneous streptomycin-resistant mutant of G. vaginalis that had been passaged through murine vaginal colonization twice. This strain, ARG37, was stocked and used for future mouse experiments. Streptomycin-treated and estrus-synchronized female mice were colonized vaginally on 2 consecutive days with approximately 106 colony-forming units and 100 µg/mL DNase or vehicle control. Colonization was assessed by quantitative culture of vaginal swabs 48 hours after initial colonization. DNase treatment at the time of inoculation reduced median colonization density by >10-fold (Figure (Figure66).
DISCUSSION
Bacterial vaginosis is a significant risk factor for preterm birth and other adverse outcomes [1]. Treatment failure and recurrence are extremely common [23], and antibiotic therapy alone does not appear to affect rates of preterm birth [24]. G. vaginalis biofilms persist in vivo and may be a major factor in the refractory nature of this condition [13]. We demonstrate in this report that G. vaginalis biofilms contain eDNA that can be enzymatically targeted to inhibit or disrupt biofilms. This finding complements those of an earlier report, which suggested that proteinaceous factors are the primary mediators of G. vaginalis biofilm resistance to peroxide and lactic acid stresses [10].
Some bacteria release eDNA in the stationary-growth phase as a result of bacterial lysis. For G. vaginalis, we noted maximal release in early exponential-growth phase, consistent with active secretion or autolysis of a bacterial subpopulation. Staphylococcus aureus genes involved in purine biosynthesis have been implicated in eDNA release into biofilms, and in Enterococcus faecalis, sortase genes have been shown to be involved, as well [17, 25]. The autolysin gene itself has been implicated in a number of bacterial species [26, 27]. Unfortunately, techniques for genetic manipulation of G. vaginalis have not yet been reported. Thus, we are currently unable to address the role of specific G. vaginalis gene products in biofilm formation.
In addition to inhibiting de novo biofilm formation, DNase liberates G. vaginalis from existing biofilms into the supernatant fraction without killing, thus decreasing biofilm density and potentially enhancing the effect of antimicrobials. The nonantibiotic nature of DNase is attractive for a therapeutic intervention, as minimizing bacterial killing may decrease selection for resistance mechanisms. DNase/antibiotic interactions have been demonstrated by other groups [21, 22] but rarely with organisms for which the biofilm mode of growth is so important to pathogenesis. We showed that low concentrations of DNase and metronidazole had improved efficacy, compared with either agent alone.
To provide proof of principle of the in vivo therapeutic or preventive usefulness of DNase, we developed a new murine model of vaginal colonization by G. vaginalis and demonstrated that DNase treatment decreases the colonization density of G. vaginalis. This represents the first report of a murine model for G. vaginalis carriage. Because of limited colonization density and duration, we were unable to assess the effect of DNase on well-established biofilms in vivo, a situation that would better approximate bacterial vaginosis in humans. However, we noted a significant effect of DNase at 48 hours of colonization, a finding consistent with a role for biofilm formation at an early stage of the interaction between Gardnerella organisms and the vaginal mucosal surface. The baseline composition of the murine vaginal microbiota and its alteration in the setting of G. vaginalis colonization remain unknown but are areas of active investigation.
These findings raise the possibility of using DNase as a therapeutic or preventive measure for bacterial vaginosis either alone or in combination with antibiotics. There is precedent for safe, repeated use of DNase formulations in humans, both in the setting of cystic fibrosis, in which it is used to break down inspissated mucus, and as an intrapleural agent in combination with tissue plasminogen activator in the treatment of pleural infection [28, 29]. Further work to characterize optimal intravaginal DNase formulations, dosing, and schedules is warranted.
Notes
Financial support. This work was supported by the National Institutes of Health (grants R01 AI092743; and R21 AI098654; [to A. J. R.], T32 AI007531; [to S. R. H., via Lisa Saiman], and K23 HD065844 [to T. M. R.]) and a Columbia University Summer Undergraduate Research Fellowship (to T. S.).
Potential conflicts of interest. Columbia University has filed a patent application for the use of DNase to break down Gardnerella biofilms. A. J. R. and S. R. H. are listed as inventors on this application. All other authors report no potential conflicts.
All authors have submitted the ICMJE Form for Disclosure of Potential Conflicts of Interest. Conflicts that the editors consider relevant to the content of the manuscript have been disclosed.
References
Articles from The Journal of Infectious Diseases are provided here courtesy of Oxford University Press
Full text links
Read article at publisher's site: https://doi.org/10.1093/infdis/jit047
Read article for free, from open access legal sources, via Unpaywall:
https://academic.oup.com/jid/article-pdf/207/10/1491/18067773/jit047.pdf
Citations & impact
Impact metrics
Citations of article over time
Alternative metrics
Smart citations by scite.ai
Explore citation contexts and check if this article has been
supported or disputed.
https://scite.ai/reports/10.1093/infdis/jit047
Article citations
Antibiofilm Agents for the Treatment and Prevention of Bacterial Vaginosis: A Systematic Narrative Review.
J Infect Dis, 230(3):e508-e517, 01 Sep 2024
Cited by: 0 articles | PMID: 38680027 | PMCID: PMC11420799
Review Free full text in Europe PMC
Biofilm inhibition/eradication: exploring strategies and confronting challenges in combatting biofilm.
Arch Microbiol, 206(5):212, 14 Apr 2024
Cited by: 3 articles | PMID: 38616221
Review
Gardnerella Revisited: Species Heterogeneity, Virulence Factors, Mucosal Immune Responses, and Contributions to Bacterial Vaginosis.
Infect Immun, 91(5):e0039022, 18 Apr 2023
Cited by: 5 articles | PMID: 37071014 | PMCID: PMC10187134
Review Free full text in Europe PMC
Migration of surface-associated microbial communities in spaceflight habitats.
Biofilm, 5:100109, 24 Feb 2023
Cited by: 5 articles | PMID: 36909662 | PMCID: PMC9999172
Treatment of Pseudomonas aeruginosa infectious biofilms: Challenges and strategies.
Front Microbiol, 13:955286, 26 Aug 2022
Cited by: 26 articles | PMID: 36090087 | PMCID: PMC9459144
Review Free full text in Europe PMC
Go to all (51) article citations
Similar Articles
To arrive at the top five similar articles we use a word-weighted algorithm to compare words from the Title and Abstract of each citation.
The biofilm in bacterial vaginosis: implications for epidemiology, diagnosis and treatment.
Curr Opin Infect Dis, 26(1):86-89, 01 Feb 2013
Cited by: 63 articles | PMID: 23221767
Review
Lysozyme as a cotreatment during antibiotics use against vaginal infections: An in vitro study on Gardnerella vaginalis biofilm models.
Int Microbiol, 19(2):101-107, 01 Jun 2016
Cited by: 10 articles | PMID: 27845497
Vaginal biofilms and bacterial vaginosis: of mice and women.
J Infect Dis, 207(10):1481-1483, 19 Feb 2013
Cited by: 10 articles | PMID: 23431034
Screening of Compounds against Gardnerella vaginalis Biofilms.
PLoS One, 11(4):e0154086, 25 Apr 2016
Cited by: 29 articles | PMID: 27111438 | PMCID: PMC4844189
Funding
Funders who supported this work.
NIAID NIH HHS (4)
Grant ID: R33 AI098654
Grant ID: T32 AI007531
Grant ID: R21 AI098654
Grant ID: R01 AI092743
NICHD NIH HHS (1)
Grant ID: K23 HD065844