Abstract
Free full text

Specialized filopodia direct long-range transport of Shh during vertebrate tissue patterning
Abstract
The ability of signaling proteins to traverse tissues containing tightly packed cells is of fundamental importance for cell specification and tissue development, however, how this is achieved at a cellular level remains poorly understood1. For over a century, the vertebrate limb bud has served as a paradigm to study cell signaling during embryonic development2. Here we optimize single cell real-time imaging to delineate the cellular mechanisms for how signaling proteins, such as Sonic Hedgehog (Shh), that possess membrane-bound covalent lipid modifications transverse long distances within the limb bud in vivo. By directly imaging Shh ligand production under native regulatory control, our findings show that Shh is unexpectedly produced in the form of a particle that remains associated with the cell via long cytoplasmic extensions that span several cell diameters. We show that these cellular extensions are a specialized class of actin-based filopodia with novel cytoskeletal features that have not been previously described. Strikingly, particles containing Shh traffic along these extensions with a net anterograde movement within the field of Shh cell signaling. We further show that in Shh responding cells specific subsets of Shh co-receptors, including Cdo and Boc, actively distribute and co-localize in specific micro-domains within filopodial extensions, far from the cell body. Stabilized interactions are formed between filopodia containing Shh ligand and those containing co-receptors over a long-range. These results suggest that contact-mediated release propagated by specialized filopodia contributes to the delivery of Shh at a distance. Together, these studies identify an important mode of communication between cells that significantly extends our understanding of ligand movement and reception during vertebrate tissue patterning.
The regulated movement of key signaling proteins within tissues is a central feature of metazoan development that remains poorly understood at the cellular level1. Several mechanisms have been proposed to distribute signaling molecules including free diffusion, transcytosis, and directed transport of signaling receptors via filopodia, including those termed cytonemes within invertebrate embryos3–5. An example of a key signaling protein is Sonic Hedgehog (Shh), which is instrumental in patterning the early embryo6. During limb development, Shh is produced by the zone of polarizing activity (ZPA), a small group of mesenchymal cells at the posterior margin of the limb bud, and acts over a long-range to specify the number and identity of digits produced7. How tight control in Shh distribution across several cell diameters is established remains poorly understood. Here, we combine unique genetic and live cell imaging approaches to investigate mechanisms of long-range cell signaling underlying tissue patterning during vertebrate embryonic development.
In order to image living chick embryos under the regulatory control of specific spatial and temporal regulatory elements, we developed a piggyBac transposon-mediated stable integration approach8. This genetically tractable expression system coupled with optimized confocal microscopy (Methods) enabled imaging of embryos (Fig. 1a, Supplementary Fig. 1) at single-cell and subcellular resolution. At first, we expressed membrane-tethered fluorescent proteins that illuminate individual mesenchymal cells within the developing limb bud in a mosaic fashion (Fig. 1b). Through this approach we uncovered an unexpected, intricate network of thin cellular extensions present on these cells spanning several cell diameters, which orient in multiple directions from the cell body along the anterior-posterior (A–P), proximal-distal (Pr-D) and dorsal-ventral (D–V) axis (Fig. 1e, Supplementary Fig. 2). All mesenchymal cells possess multiple cellular extensions up to 150 µm long, with an average length of 34.27 +/− 9.6µm (n=24), revealing a surprising morphology to these cells (Fig. 1c–e and Supplementary Movie 1). These extensions are remarkably fine, approximately 200 nm in diameter, at the resolving limit of conventional microscopy and can be labeled with membrane-bound fluorescent proteins (Fig. 1) but not with cytoplasmic fluorescent proteins (Supplementary Fig. 3). They are capable of elongating, at a maximum rate of 150 nm/sec, retracting, and traversing the complex three-dimensional extracellular matrix of the limb bud (Fig. 1f–h, Supplementary Fig. 2, Supplementary Movies 2 and 3). Importantly, despite their dynamic nature, they form highly stabilized long-range interactions between cells thereby revealing a novel and complex landscape of cell-cell interactions (Supplementary Fig. 2, Supplementary Movies 4 and 5). Importantly, these cytoplasmic extensions are not amenable to conventional fixation, which severely disrupts their structure and may have precluded their prior identification (Supplementary Fig. 5).
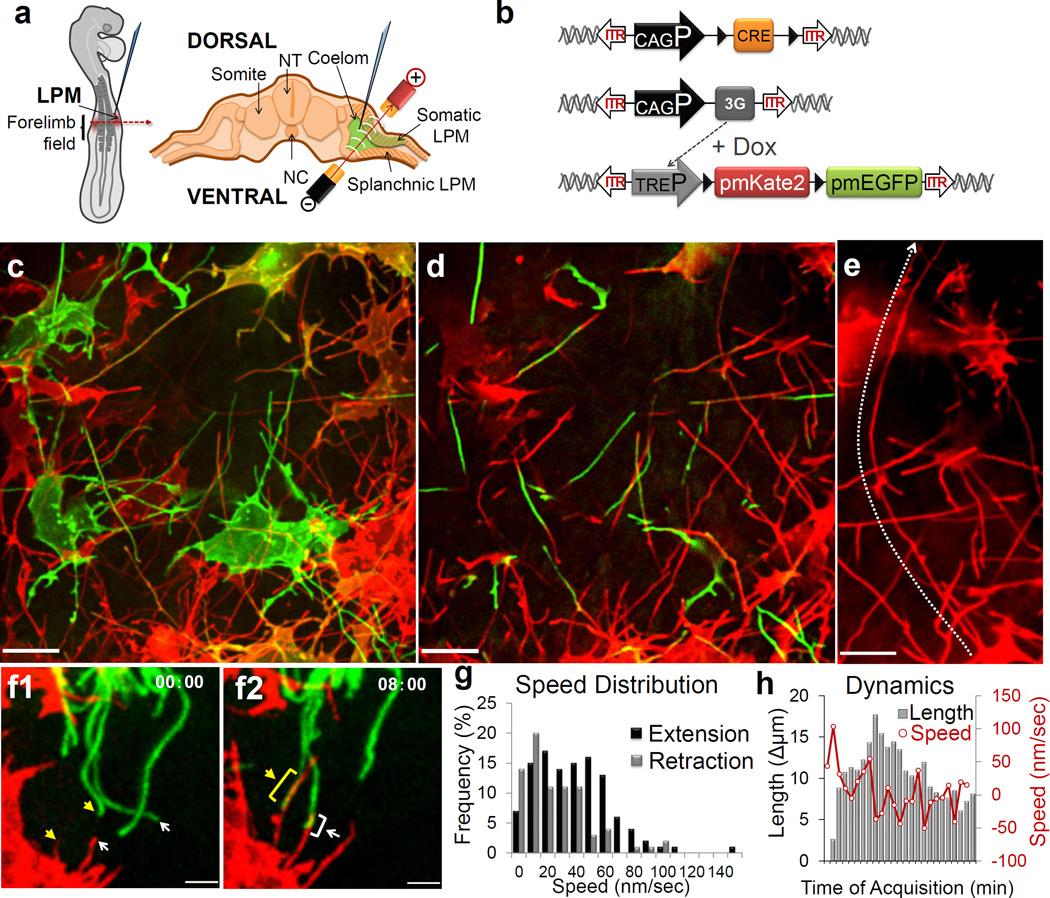
Mesenchymal cells of the developing limb bud possess long and highly dynamic cytoplasmic extensions. (a) Left: HH14 chick embryo indicating the site of DNA injection. The red dashed line indicates the cross-sectional plane, Right: Microinjected DNA in the coelom is shown in green, electrodes position is indicated (LPM)(NC: Notochord; NT: Neural Tube). (b) Diagram of piggyBac transposon system resulting in integration of transposon inverted terminal repeat (ITR) flanked expression cassettes by piggyBac transposase. Cre recombinase flanked by loxP sites (black triangles) results in mosaic labeling from a loxP-containing reporter construct, containing membrane palmitoylated mKate2 or mEGFP expressed via the tetracycline responsive element (TRE). Ubiquitous promoter (CAGP), doxycycline (DOX) inducible transactivator protein (3G). (c) Confocal z-series acquired in vivo from a HH21 limb bud reveals an intricate network of cellular extensions (Supplementary Movie 1). Scale = 10µm. (d) Single x-y plane, from c, highlighting the network of long cytoplasmic extensions among mesenchymal cells. (e) A representative long extension, from c, 75 µm marked by line. Scale = 10µm. (f) Example of an interaction between two cytoplasmic extensions. Interaction starts at tips (f1, asterisk) and then extends until both extensions overlap (f2, asterisks) (see also Supplementary Movie 4). Scale = 3µm. Time in min:sec. (g) Frequency distribution of extending (black) and retracting (grey) velocities, n = 8. (h) Extension dynamics. Gray bars represent net length change in µm. Red line represents the mean velocity, nm/sec. X-axis ticks = 1 minute intervals.
Actin-associated markers, including the high affinity F-actin probe Utrophin calponin homology domain (UCHD)-EGFP and Moesin-EGFP, decorate the entire length of cytoplasmic extensions, revealing that these structures are actin-based filopodia (Fig. 2a, Supplementary Fig. 4a). Unlike actin markers, tubulin cytoskeleton markers, including Tau and EB3, only label the proximal base of a subset of mesenchymal filopodia (data not shown). We next examined the localization of a plus ended actin motor, Myosin X9. Strikingly, Myosin X-GFP moves to the distal tips of the filopodia, where it accumulates, thereby revealing that actin motors can move along these structures (Fig. 2b, data not shown).
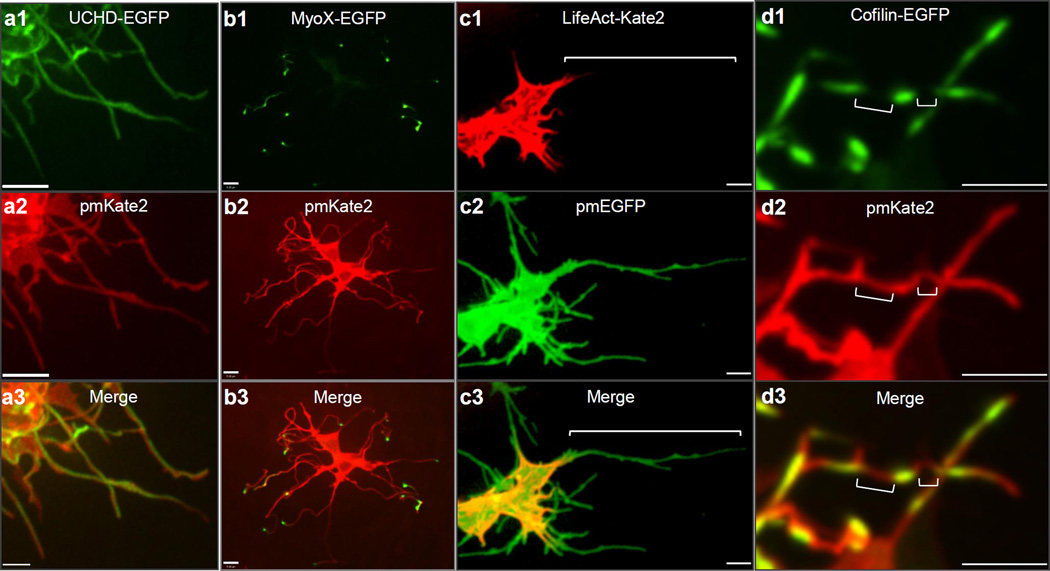
Limb mesenchymal cytoplasmic extensions are a class of specialized actin-based filopodia. (a1–3) UCHD-EGFP demonstrating that membrane labeled pmKate2 filopodia extensions contain actin filaments. Scale = 3µm. (b1–3) Myosin X-EGFP is localized to each pmKate2 labeled filopodium and is concentrated at the distal tip. (c1–3) LifeAct-Kate2 marks only the proximal aspect of pmEGFP labeled filopodia and does not label the entire extension, shown by bracket. (d1–3) Cofilin-EGFP is present in interrupted domains along the filopodia, negative regions shown with brackets. Scale = 5µm.
Limb bud mesenchymal cytoplasmic extensions also possess distinct cytoskeletal features compared to typical filopodia, commonly characterized as actin-based linear extensions of the cell membrane with limited lengths up to 10µm10. For example, LifeAct is a highly specific marker of filopodia in eukaryotic cells, however it unexpectedly only labels the proximal base of limb mesenchyme cytoplasmic extensions (Fig. 2c) but not their distal tips, reflecting a distinguishing feature. This is consistent with the fact that LifeAct does not label certain forms of highly modified actin, or actin that is highly coated with binding proteins11. Additional specific features are also evident in the actin depolymerization factor cofilin, which has mainly been implicating in extending lamellipodial protrusions12. Strikingly, cofilin-EGFP rapidly accumulates to the tips of limb mesenchymal filopodia and its subsequent retraction back to the cell soma prefigures the rapid and dynamic retraction of filopodia extensions (Supplementary Fig. 4b, Supplementary Movie 6). Cofilin-EGFP is also frequently localized to specific microdomains along these filopodia that are interrupted rather than labeling the entire process (Fig. 2d). Fascin, which enhances cofilin severing12, also labels filopodial extensions (Supplementary Fig. 4c).Together, these findings demonstrate that limb mesenchyme filopodia possess unique properties, which include their considerable lengths, highly dynamic behaviors, and complex geometries. Our initial attempts to perturb mesenchymal filopodia formation employing known molecular pathways (Methods) have proven ineffective, for example through conditional inactivation of Cdc42 in the limb bud (data not shown).
To determine the functional role of mesenchymal filopodia, we employed genetic strategies to precisely label specific cellular populations with membrane bound fluorescent proteins along the A–P axis of the mouse limb bud with respect to Shh signaling. This revealed that Shh producing cells within the limb bud ZPA extend long filopodia (Supplementary Fig. 6a and Supplemental Movie 7), which can orient along the A–P axis as well as the Pr-D axis, with a further bias towards the apical ectodermal ridge that maintains the Shh-FGF feedback loop13 (Supplementary Fig. 7a, b). Moreover, mesenchymal cells that respond to Shh, within the anterior limb bud, also extend similar filopodia (Supplementary Fig. 6b) however they show a bias in orientation along the A–P axis (Supplemental Fig. 7a, c). Thereby, these results demonstrate that both Shh producing cells as well as responding cells extend specialized filopodia within the Shh signaling field.
To determine the possible role of limb mesenchyme filopodia with respect to Shh signaling, we visualized key components of the Shh signaling pathway. To image Shh ligand, we developed a tightly regulated expression system directed by the endogenous G. gallus Shh minimal promoter and limb-specific enhancer element (ZRS)14 coupled with doxycycline inducible control allowing for transient gene expression in a small number of cells within the ZPA (Supplementary Fig. 8a). Shh is produced as a precursor protein that undergoes autocatalytic processing to yield an amino-terminal signaling protein (ShhP) containing cholesterol and palmitate moieties15. Shh fusion proteins with monomeric EGFP were constructed, including ShhP-EGFP that retains the cholesterol modification and ShhN-EGFP that does not, but produces a brighter and more photostable fluorescent fusion protein (Supplementary Fig. 8b, Methods). Consistent with the fact that ShhN, can activate Shh signaling within the limb bud16 we find that upon ectopic expression of ShhN-EGFP, Patched (Ptc1) a marker of Shh activation, is expanded anteriorly within the limb bud (Supplementary Fig. 8e). Importantly, expression of these Shh fusion proteins under doxycycline inducible control does not perturb endogenous Shh signaling as revealed by Ptc1 expression, nor limb development and skeletal patterning (Supplementary Fig. 8d).
Imaging of ShhN-EGFP under native regulatory control in the ZPA, reveals that it is unexpectedly produced in the form of a particle approximately 200 nm in size (Fig. 3a, b2). These particles are never observed within the extracellular space, but remain associated with the Shh producing cell via long filopodial extensions. Imaging of ShhP-EGFP also reveals that cholesterol modified Shh is similarly produced as a particle associated with filopodial extensions and also displays more uniform localization along these extensions (Fig. 3b1, Supplementary Fig. 8c). Importantly, such particles are an intrinsic property of the Shh molecule, as they are not formed by expression of cytoplasmic, palmitoylated or cholesterol modified EGFP (data not shown). Shh particles only traffic to specific subsets of filopodia emanating from the same cell, revealing tight selectivity and regulation over this process (Fig. 3c).
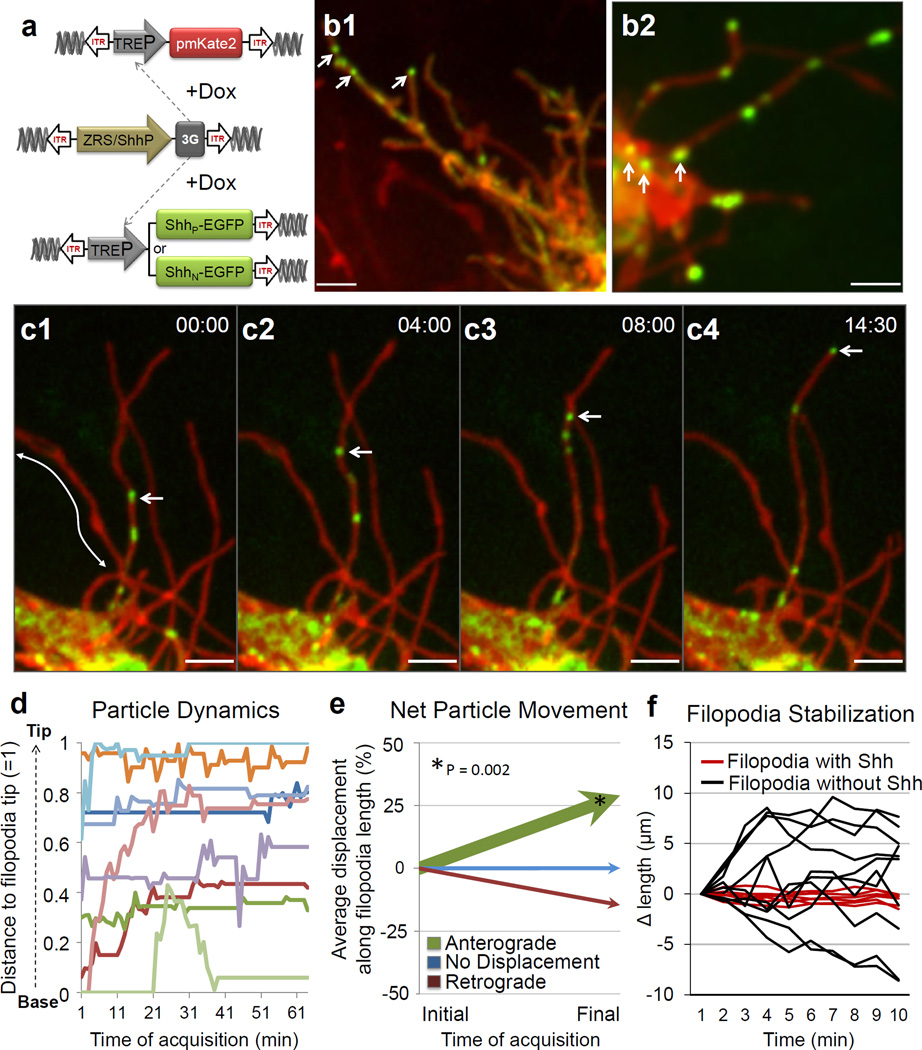
Live cell imaging of Shh ligand production and trafficking within the limb bud. (a) Schematic of piggyBac-mediated integration of transposon flanked expression cassettes (ITR). The G. gallus Shh minimal promoter (ShhP) and ZRS element direct spatial expression in the limb ZPA of doxycycline (DOX) inducible transactivator protein (3G) which in turn allows for the temporal control of ShhN-EGFP or ShhP-EGFP and pmKate2. (b1) A representative Shh producing cell harboring multiple long filopodia with ShhP-EGFP present in discrete particles as well as in a more diffuse form localized along these extensions. (b2) ShhN-EGFP is produced as a particle visualized within the cell soma (arrows) as well as along the filopodia. (c1–4) A representative timelapse showing anterograde ShhN-EGFP particle movement (indicated by arrow) that accumulates at the tip of the certain filopodia but not others (arrows). (Supplementary Movie 8). Time in min:sec, interval 4 frames/sec. (d) Graph demonstrating the movement of ShhN-EGFP particles relative to the filopodium, normalized distance to filopodia base = 0 and filopodia tip = 1. (e) Graph demonstrating the net vectors of particle (n=38) displacement represented as a percentage of the total filopodia length that particles transverse, anterograde (green), no displacement (blue, < 5%) and retrograde displacement (red). The relative thickness of each vector reflects the percentage of particles within each category.. There is a statistically significant, net anterograde movement of Shh particles away from the cell soma, p < 0.002. (f) Shh-harboring filopodia (red line) are statistically more stabilized than filopodia without Shh (black line), p < 0.001, n=200 timepoints. Scale = 3µm.
High-speed, real-time imaging revealed that ShhN-EGFP particles move in both anterograde and retrograde directions along filopodia (Fig. 3c, d, Supplementary Movie 8), with a statistically significant net anterograde movement away from the cell body (p < 0.002) (Fig. 3e, Supplementary Fig. 9). The maximum velocity of anterograde particle movement, 120 nm/sec, is consistent with actin-based myosin motors17. Moreover, filopodia harboring Shh particles are stabilized and less dynamic than non-particle containing filopodia (Fig. 3f, p < 0.001). These findings reveal that filopodia can distribute Shh ligand at a distance from the cell body. It remains to be determined whether the Shh cholesterol modification, which has been proposed to either promote18 or restrict16 the spread of Shh, has additional functions in filopodial transport. To our knowledge, this is the first in vivo demonstration of Shh ligand production and movement, revealing an unexpected role for filopodia in this process.
To determine the precise localization of ShhN-EGFP within filopodia, we employed an optimized split GFP complementation system19. Strikingly, ShhN-spGFP11 can physically interact and complement an extracellular leaflet associated GPI-spGFP1–10 to produce a GFP positive signal along the extracellular surface of limb mesenchyme filopodia in vivo (Supplementary Fig. 10a, d). We also cultured mesenchymal cells following electroporation of ShhN-spGFP11 and applied a synthesized spGFP1–10 peptide exogenously to the media (Methods) that similarly produced a GFP fluorescent signal along the filopodial membrane, but not when cells do not express ShhN-spGFP11, or express a control GFP1–10 cytoplasmic fragment (Supplementary Figure 10b–d). In addition, ectopic expression of ShhN-spGFP11 tethered to the membrane as a result of its interaction with GPI-spGFP1–10 leads to the ectopic expansion of Ptc1 expression (Supplementary Fig. 10e). Although the split GFP complementation system displays remarkable antibody affinity for GFP fragments20, we cannot exclude that ectopic activation of Ptc1 may also derive from a freely diffusible form of ShhN-spGFP11 that we cannot detect. Collectively, these experiments suggest that ShhN-EGFP is localized to the extracellular leaflet of the filopodial membrane where it is competent to signal and interact with Shh receptors.
We next visualized additional Shh signaling components involved in the reception of Shh in vivo. In addition to Ptc, which serves as the identified receptor for Shh21, additional co-receptors of Shh, including the transmembrane proteins Cell adhesion molecule down-regulated by oncogenes (Cdo) and Brother Of Cdo (Boc), are necessary for long-range Shh signaling22, 23. Interestingly, within the limb bud these co-receptors are only expressed in Shh responding cells24. In contrast to other components of the Shh signaling pathway, such as Ptc-YFP and Smoothened-GFP that localize to primary cilia (Supplementary Fig. 11), our live imaging reveals that Cdo-GFP and Boc-GFP do not. Rather, they exhibit striking localization to discrete microdomains along long filopodial (average size 2.4 +/− 1.5 µm; range 0.6–8.5 µm) that remain static and display little lateral movement (Fig. 4a–b). Moreover, there is substantial colocalization of these co-receptors to these microdomains within only a subset of filopodia emanating from individual Shh-responding mesenchymal cells, reflecting tight spatial regulation (Fig. 4c). Interestingly, there is a statistically significant stabilization of the filopodia containing Shh co-receptors (Fig. 4d, p < 0.001). The molecular role of Cdo and Boc in transducing long-range signaling have been poorly understood, although their binding to Shh is independent from Ptc25. The unexpected co-localization of these co-receptors on micro-domains along filopodia suggests that they may participate in relaying activation of the pathway at a distance from the cell soma.
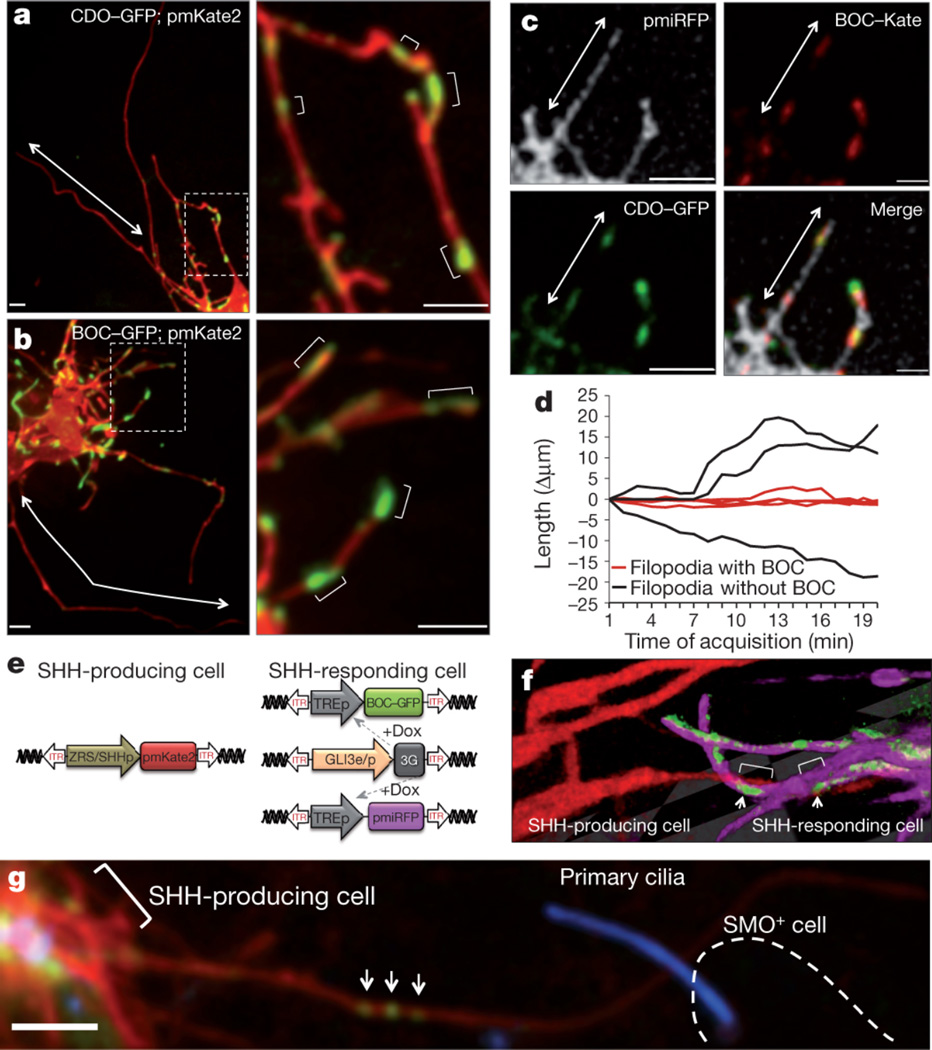
Filopodia on Shh responding cells display an exquisite distribution and co-localization of Shh co-receptors that interact with Shh producing filopodia. (a1) Live imaging of Cdo-GFP and (b1) Boc-GFP expression in defined microdomains along the filopodial membrane, within subsets of filopodia but not others (arrows). (a2–b2) Higher magnification of Fig. a1 and b1 showing multiple positive microdomains of co-receptor localization (brackets) interspersed along the filopodia membrane. (c1–4) Cdo-GFP and Boc-Kate2 are colocalized along microdomains of filopodia (arrow) labeled with membrane-associated near-infrared fluorescent protein (pmiRFP). (d) Boc-harboring filopodia (red line) are statistically more stabilized than filopodia without Boc (black line), p < 0.001, n=160 timepoints. (e) Expression system to specifically label Shh producing cells and Shh responding cells in the same limb bud. (f) Representative 3D image of a filopodia from a Shh producing cell (pmKate2 - red) that interacts with domains of Boc-GFP (green) along the filopodia membrane (pmiRFP, fuscia) of Shh responding cell. Arrows show interaction along Boc microdomains (brackets). (g) Shh producing cell, indicated by pmKate2 and marked by bracket, with a long filopodium containing ShhN-EGFP particles (arrows) that contacts a Smoothened positive cell, Smo+, (outlined by a dashed line). Smoothened-BFP localization to the cilium is a marker of Shh pathway activation. Scale = 3µm.
To simultaneously and precisely mark Shh producing and responding cells we further designed a hybrid Gli3 enhancer and promoter element (Methods, Fig. 4e). Employing this dual expression system, we find that filopodia of Shh-producing cells directly interact with filopodia of Shh-responding cells that harbor micro-domains of Boc-EGFP (Fig. 4f). Similar stabilized interactions are evident between filopodia containing Shh ligand and Shh responding cells that have undergone pathway activation as revealed by Smoothened-BFP localization to cilia (Fig. 4g). Together, these results reveal that Shh producing and responding cells interact at a distance through filopodial membrane-to-membrane contacts containing Shh ligand and co-receptors.
Our live imaging studies have identified a specialized class of filopodia with distinct cytoskeletal features that localize and traffic components of the Shh signaling pathway, uncovering an important mechanism for the distribution of signaling molecules within tissues. The highly stabilized interactions between filopodia containing Shh ligand and those containing Shh co-receptors strongly suggest that long-range activation of signaling may be mediated through direct receptor-ligand interactions between cell membranes at a distance. Indeed, Shh producing and responding cells can extend filopodia as long as 150 µm in length encompassing the entirety of the 300 µm field of Shh signaling18 within the limb bud. Interestingly, mesenchymal filopodia may share certain properties with those of cellular extensions previously described within invertebrate embryos5, 26 and these findings are consistent with earlier studies including electron microscopy carried out over 30 years ago describing the presence of fine cellular extensions on limb bud mesenchyme27, 28. Future studies will be required to determine whether such “specialized filopodia” are an inherent feature of many, additional cell types that may have escaped prior detection in fixed and stained samples. Moreover, an outstanding question is whether such cellular extensions rely on unique cellular machinery for their generation, structure, and ability to traffic signaling molecules. For example, our findings revealing that Shh is produced in the form of a particle, which traffics along these filopodial extensions in a highly directional manner, suggests that a yet unidentified molecular motor may be responsible for the movement of Shh within tissues. It is intriguing to speculate that the mechanisms for delivery of proteins, and perhaps other molecules such as nucleic acid, along specialized filopodial networks offer a new paradigm for controlling the precise distribution of information during embryonic development, regeneration, and pathological processes such as cancer metastasis. Considering the diverse cellular milieus in which signaling molecules have been shown to act, specialized filopodia may be a more adapted feature of certain signaling centers that operate alone or in concert with other proposed models for ligand distribution, including free diffusion through extracellular space.
METHODS SUMMARY
A spatially and temporally regulated piggyBac transposition system was developed to express fluorescent fusion proteins in a heritable fashion within the developing chick embryonic limb bud. The regulated expression of introduced transgenes was stable throughout embryogenesis and did not perturb normal limb development. Cellular morphology and characterization of specialized filopodia was assessed through the use of membrane-associated fluorescent proteins and selected markers of the actin and tubulin cytoskeleton. Molecular components of the Shh signaling pathway were visualized as fusion proteins under endogenous regulatory control. High-resolution live imaging of the embryonic limb bud was performed on custom designed spinning disk confocal systems allowing for the visualization of multiple fluorescently coupled proteins in real time, at high spatial resolution.
Acknowledgments
We thank Dyche Mullins for helpful discussion on the actin cytoskeleton as well as Gail Martin and members of the Barna lab for discussion and critical reading of the manuscript. We thank Katrina Cabaltera for technical assistance. This work was supported by Spanish Ministry of Education and Science (E.L.), Program for Breakthrough Biomedical Research, UCSF (M.B.), the March of Dimes Basil O’Connor Scholar Research Award (M.B.), and the NIH R21AR062262 award (M.B.).
Footnotes
Author Contributions: M.B. conceived and supervised the project; T.A.S, E.L, and M.B. designed experiments; T.A.S. and E.L. performed experiments. All authors analyzed the data, critically discussed the results, and contributed towards writing and preparation of the manuscript.
The authors declare no competing financial interests.
References
Full text links
Read article at publisher's site: https://doi.org/10.1038/nature12157
Read article for free, from open access legal sources, via Unpaywall:
https://europepmc.org/articles/pmc4197975?pdf=render
Citations & impact
Impact metrics
Citations of article over time
Alternative metrics
Smart citations by scite.ai
Explore citation contexts and check if this article has been
supported or disputed.
https://scite.ai/reports/10.1038/nature12157
Article citations
The cellular protrusions for inter-cellular material transfer: similarities between filopodia, cytonemes, tunneling nanotubes, viruses, and extracellular vesicles.
Front Cell Dev Biol, 12:1422227, 05 Jul 2024
Cited by: 1 article | PMID: 39035026 | PMCID: PMC11257967
Review Free full text in Europe PMC
Phalloidin-PAINT: Enhanced quantitative nanoscale imaging of F-actin.
Biophys J, 123(18):3051-3064, 03 Jul 2024
Cited by: 0 articles | PMID: 38961624 | PMCID: PMC11427775
Distinct expression patterns of Hedgehog signaling components in mouse gustatory system during postnatal tongue development and adult homeostasis.
PLoS One, 19(6):e0294835, 07 Jun 2024
Cited by: 0 articles | PMID: 38848388
Hedgehog on the Move: Glypican-Regulated Transport and Gradient Formation in <i>Drosophila</i>.
Cells, 13(5):418, 27 Feb 2024
Cited by: 1 article | PMID: 38474382 | PMCID: PMC10930589
Review Free full text in Europe PMC
Cytoneme signaling provides essential contributions to mammalian tissue patterning.
Cell, 187(2):276-293.e23, 02 Jan 2024
Cited by: 8 articles | PMID: 38171360
Go to all (217) article citations
Other citations
Wikipedia
Data
Data behind the article
This data has been text mined from the article, or deposited into data resources.
BioStudies: supplemental material and supporting data
Similar Articles
To arrive at the top five similar articles we use a word-weighted algorithm to compare words from the Title and Abstract of each citation.
Filopodia: the cellular quills of hedgehog signaling?
Dev Cell, 25(4):328-330, 01 May 2013
Cited by: 6 articles | PMID: 23725760
Cytoneme delivery of Sonic Hedgehog from ligand-producing cells requires Myosin 10 and a Dispatched-BOC/CDON co-receptor complex.
Elife, 10:e61432, 11 Feb 2021
Cited by: 31 articles | PMID: 33570491 | PMCID: PMC7968926
Manifestation of the limb prepattern: limb development in the absence of sonic hedgehog function.
Dev Biol, 236(2):421-435, 01 Aug 2001
Cited by: 285 articles | PMID: 11476582
How the embryo makes a limb: determination, polarity and identity.
J Anat, 227(4):418-430, 07 Aug 2015
Cited by: 56 articles | PMID: 26249743
Review
Funding
Funders who supported this work.
NIAMS NIH HHS (2)
Grant ID: R21AR062262
Grant ID: R21 AR062262