Abstract
Free full text

Very Small Embryonic-Like Stem Cells from the Murine Bone Marrow Differentiate into Epithelial Cells of the Lung
Associated Data
Abstract
The view that adult stem cells are lineage restricted has been challenged by numerous reports of bone marrow (BM) derived cells giving rise to epithelial cells. Previously, we demonstrated that non-hematopoietic bone marrow cells are the primary source of BM derived lung epithelial cells. Here we tested the hypothesis that very small embryonic like cells (VSELs) are responsible for this engraftment. We directly compared the level of BM derived epithelial cells after transplantation of VSELs, hematopoietic stem/progenitor cells, or other nonhematopoietic cells. VSELs clearly had the highest rate of forming epithelial cells in the lung. By transplanting VSELs from donor mice expressing H2B-GFP under a type 2 pneumocyte specific promoter, we demonstrate that this engraftment occurs by differentiation and not fusion. This is the first report of VSELs differentiating into an endodermal lineage in vivo, thereby potentially crossing germ layer lineages. Our data suggest that Oct4+ VSELs in the adult BM exhibit broad differentiation potential.
Introduction
Stem cells are characterized by their ability to both differentiate into various cell types and self-renew to maintain their own populations. Pluripotent stem cells derived from the mammalian embryo can give rise to all differentiated cell types, a trait that is generally thought to be restricted to embryonic cells. By contrast, adult stem cells are lineage-restricted and support the maintenance of tissues that undergo constant turnover, such as the blood, skin, lung and intestine. For example, in the bone marrow (BM), hematopoietic stem cells (HSC) give rise to all blood cell types, while mesenchymal stem cells (MSC) give rise to bone, cartilage and adipose cells. However, in recent years numerous reports have challenged this dogma by demonstrating that BM derived cells can give rise to differentiated cells of multiple non-hematopoietic organs, including the lung. In one of these studies, Krause et al. [1] used a BM cell population enriched for small cells that do not express markers of differentiated blood cells (lineage negative) and are able to home to the BM. These studies challenge the view that adult stem cells have restricted potential, and suggest that adult stem cells from one tissue are able to give rise to cell types of a different lineage, a phenomenon termed “plasticity.”
The population of BM derived cells that can give rise to epithelial cells has remained elusive. We recently demonstrated that non-hematopoietic (lineage negative) BM cells are the primary source of BM-derived lung epithelial cells by showing that they consistently gave rise to surfactant protein C (SPC) positive lung epithelial cells in SPC-knockout (SPC-KO) recipient mice, while hematopoietic BM cells do not [2]. Here, we used this model to identify the subpopulation of non-hematopoietic BM cells that is capable of giving rise to lung epithelial cells. Murine very small embryonic like cells (VSELs) are small (2–6 µm), lineage negative, CD45 negative stem cells in the BM that express Oct4 and Nanog, and give rise to cells of three germ layer lineages in vitro [3]. To test the hypothesis that VSELs are the non-hematopoietic cell population of the BM that is responsible for lung epithelial engraftment, VSELs were isolated and their ability to give rise to type 2 (T2) lung epithelial cells was compared to all other cell types in the non-hematopoietic BM fraction (non-VSELs). The data show that BM-derived lung epithelial cells arise predominantly from VSELs and only very rarely from non-VSELs, and that VSELs differentiate into SPC-positive type 2 pneumocytes in the lung in the absence of fusion, activating the SPC promoter and expressing SPC mRNA. These results identify VSELs as the primary source of BM-derived lung epithelial cells.
Materials and Methods
Mice
SPC-KO mice [4] were a kind gift from J. Whitsett (Cincinnati Children’s Hospital), and were crossed to Tg(ACTB-DsRed*MST)1Nagy/J mice (Jackson Laboratory), which constitutively express dsRed, in our facility. Wild type (WT) C57BL/6 and Tg(HIST1H2BB/EGFP)1Pa/J mice were from Jackson Laboratory. SPC-H2B-GFP mice [5] were generated in the laboratory of Carla Kim (Boston Children’s Hospital).
Sorting of VSELs and non-VSELs, BM transplantation
VSELs were isolated as described [3]. Briefly, BM was flushed from femurs and tibias using PBS with 2% FBS, resuspended and filtered through a 70 µm cell strainer. After RBC lysis, cells were stained with the following antibodies: PE-conjugated anti-CD45R/B220, anti-Gr-1, anti-TCRαβ, anti-TCRγδ, anti-CD11b and anti-Ter119, biotin-conjugated anti-Ly-6A/E (Sca-1), PECy5-conjugated Streptavidin, and APC-Cy7-conjugated anti CD45 (all from BD Biosciences). Antibodies were used at saturating concentrations, and cells were incubated 30 min on ice, washed twice, and sorted on a MoFlo cytometer (Cytomation). VSELs from one donor mouse (900–1500) or 100,000 non-VSEL’s were injected into the retro-orbital plexus of each SPC-KO recipient mouse that had been lethally irradiated with 1000 cGy from a Cs-137 source along with 500,000 recipient type (SPC-KO) WBM cells for radioprotection. As negative controls, SPC-KO mice were transplanted with 2 million WBM cells from SPC-KO mice (also referred to as SPC-KO mice) and treated and analyzed in the same fashion as mice receiving VSELs or non-VSELs. HSPC (50,000/recipient) were transplanted without additional cells.
Immunofluorescence on lung tissue sections
One lobe of the lung was fixed in 4% paraformaldehyde, paraffin embedded, cut into 5µm sections, deparaffinized and treated with antigen retrieval solution (Retrievagen A, BD Biosciences) for 20 min in steam. After blocking with 5% donkey-serum and mouse-on-mouse blocking reagent (MOM-kit, Vectorlabs), sections were stained with polyclonal rabbit anti-SPC (Millipore), mouse anti-TTF1 (clone 8G7G3/1, DAKO) followed by Alexa-555-conjugated donkey anti-rabbit secondary antibody (Invitrogen). For staining of TTF1 in violet, tyramide amplification was performed. A biotin-conjugated anti-mouse antibody (Abcam) was followed by streptavidin-HRP and biotin-XX-tyramide (Invitrogen). The amplified biotin-signal was then detected with streptavidin-Alexa 405. SPC and TTF-1 double positive cells were analyzed in detail on a Leica SP5 confocal microscope (Leica Microsystems, Wetzlar, Germany).
Lung harvest and lung single cell suspension
After being anesthetized with ketamine/xylazine, mice underwent thoracotomy and right ventricular perfusion as described [6]. The left lung lobe was tied off and processed for paraffin embedding. The remaining lung was infused with Dispase I (Roche) in DMEM medium followed by 1% low melting agarose. After cooling the agarose, the lung was digested for 1h at 37°C, and dissociated on a GentleMACS tissue dissociator (Miltenyi Biotec, Bergisch-Gladbach, Germany). DNAse (100 units/ml, Roche) was added, and after incubation at 37°C for 15min, cells were filtered through 70 µm and 40 µm cell strainers. Cells were washed with DMEM medium and processed for either ImageStream analysis or cell sorting.
ImageStream analysis
Cells were fixed with 4% paraformaldehyde, washed in PBS, and permeabilized in buffer containing 0.5% saponin and 1% BSA. Where indicated, cells were then stained with guinea pig anti-SPC (kind gift from J. Whitsett), rabbit anti-bovine wide spectrum cytokeratin (DAKO), goat anti-GFP/YFP (Abcam), rat anti-mouse CD45 (BD Pharmingen) and rat anti-mouse F4/80 (EBiosciences), followed by Alexa 555-conjugated goat anti guinea pig, Alexa 488-conjugated donkey anti goat, Alexa 568-conjugated donkey anti rabbit and biotin conjugated donkey anti-rat secondary antibodies (Invitrogen) followed by Streptavidin PE-Cy5 (BD Biosciences). For experiments with DsRed, SPC was stained in Alexa 647 using rabbit anti-SPC (Millipore) followed by donkey anti rabbit Alexa 647 (Invitrogen). Nuclei were stained with DAPI (Invitrogen). Cells were analyzed on a two-camera ImageStream imaging cytometer (Amnis Corporation, Seattle USA) and analyzed as previously described [2], and shown schematically in Figure S1.
T2 cell sorting and PCR for SPC-mRNA
Dissociated lung cells were suspended in DMEM, and stained with APC-conjugated anti-CD45,-CD31,-Gr-1,-Ter119,-CD11b,-B220,-CD3e (BD Biosciences), PE/Cy7-conjugated anti-mouse CD326 (Epcam, Biolegend), Pacific Blue-anti-mouse CD49f (Biolegend) and DAPI. Sorting was performed on a BD-FACS-Aria cell sorter with low pressure settings and a 100µm nozzle. APC and DAPI-negative (viable), Epcam and CD49f-positive T2 cells were collected directly into RNA-lysis buffer (Qiagen). RNA was isolated using the RNeasy Mini kit (Qiagen) and reverse transcribed into cDNA using random primers and Superscript RTIII reverse transcriptase (Invitrogen). SPC mRNA was quantified by real-time PCR using SYBR Green. Primers were designed to bind in exons 2 and 3 of SPC-mRNA, such that no product is generated from the SPC-KO allele lacking exon 2. Relative quantification of SPC mRNA expression was calculated using the comparative CT method, and normalized to the endogenous control gene β2-microglubulin. Expression levels were calculated as fold difference compared to SPC-KO controls, which were set to 1. Melting curves were analyzed and gel electrophoresis was performed to confirm the correct size of the amplification and the absence of nonspecific products.
Results
Transplantation of VSELs vs. Lin− CD45− non-VSELs into Surfactant Protein C-Knockout Mice
VSELs were isolated from the BM of WT mice as described [3]. Briefly, flow cytometry size beads were used to create a gate that includes events between 2 and 10 µm in size. From this gate, Lin− and Sca-1 positive cells were gated, which include Lin− Sca-1+ CD45+ hematopoietic stem and progenitor cells (HSPCs) and Lin−Sca-1+ CD45− VSELs. The smaller size of Lin−Sca1+CD45− VSELs compared to Lin−Sca1+CD45+ HSPC was evident on the forward scatter scale (Figure S3E). Freshly isolated VSELs were transplanted into lethally irradiated SPC-KO mice (Figure 1). SPC is exclusively expressed by T2 cells in the lung, and its expression is completely absent in SPC-KO mice. Thus, T2 cells derived from donor BM cells can be specifically identified based on expression of SPC in the lungs of SPC-KO recipients. Recipient type (SPC-KO) whole BM (WBM) cells were co-transplanted for radioprotection. Freshly isolated VSELs expressed high levels of Oct4 and Nanog by immunofluorescence and PCR, whereas HSPC, isolated as controls, were negative for Oct4 and Nanog by both immunofluorescence (Figure 1G) and PCR (Figure S3). To isolate all non-hematopoietic cells that are not VSEL’s, a slightly different sorting strategy was employed, in which Lin-negative, CD45-negative cells that did not fall into the small (2–6µm) VSEL-gate were isolated (Figure 1E and F). These cells will be termed “non-VSELs,” and were negative for Oct4 by immunofluorescence and PCR (data not shown).

Sorting strategy for isolation of VSELs and non-hematopoietic non-VSEL-cells, experimental approach and expression of Oct4 and Nanog in VSELs isolated from BM. A: FSC-SSC gate was set around events ranging from 2–10µm using flow cytometry size beads. B–D: VSELs were sorted from this gate (R1) as lineage-negative and Sca-1 positive cells (R2), that are also CD45-negative (R3), while HSPC are CD45-positive (R4). E and F: Sorting of non-VSEL cells. Excluding the cells between 2 and 6µm, Lin− CD45− negative cells were sorted (Lin− CD45− Non-VSELs). G: Immunofluorescent staining of freshly isolated VSELs or HSPC for Oct4 (green) and Nanog (red). Murine embryonic stem cells (ESC) served as positive control. Images were acquired with a 63× objective. H: Experimental approach: VSELs, Lin negative, CD45-negative CD45− Non-VSELs or HSPC were sorted from WT BM and transplanted into SPC-KO recipients, and 2 months post transplant, lung tissue was harvested and analyzed.
VSELs are the primary source of BM-derived lung epithelial cells
In order to accurately quantitate BM-derived T2 cells, we used imaging flow cytometry, which allows for single cell analysis without interference of cell overlay or background fluorescence, and for detailed image analysis, including calculation of fluorescence levels and the number of bright spots within a cell. Lung tissue from SPC-KO mice transplanted with VSELs, non-VSELs or HSPCs was harvested 2 months after transplantation and dissociated into single cells by enzymatic digestion. Single cells were fixed, permeabilized and stained for alveolar T2 cell specific SPC, epithelial specific cytokeratin, and blood cell markers (CD45 and F4/80). Only cells negative for both CD45 and F4/80 were further analyzed. Images were then assessed in detail by image analysis software (Amnis IDEAS application) for cells exhibiting intracellular SPC and cytokeratin (Figure S1). In T2 cells from WT mice, SPC is expressed in intracellular lamellar bodies, resulting in a vesicular staining pattern (Figures 2A). Cells with a fluorescent signal in the SPC-channel (yellow) were further analyzed only if they contained at least two distinct, SPC-positive vesicles and the signals in the cytokeratin and SPC channels did not co-localize, to exclude auto-fluorescence (Supplemental Figure 1 A–F). SPC-positive cells were then individually, manually compared to the phenotype seen in WT cells, and only counted as donor derived T2 cells if they were intact in the brightfield and displayed similar morphology and staining pattern as WT T2 cells in addition to being cytokeratin+. SPC+ donor derived T2 cells were present in 27 out of 28 mice transplanted with VSELs. Absolute numbers of SPC+ cells are given in Supplemental Figure 1G. Quantitative analysis showed that 4% of all T2 pneumocytes (normalized to WT controls, which were set to 100%) expressed SPC and thus were donor derived in mice receiving VSELs. In contrast, only 0.36% of T2 cells were donor derived mice receiving non-VSELs, and (Figure 2B). With this approach, T2 cells expressing SPC were never detected in untransplanted SPC-KO mice (Figure 2B), nor in mice transplanted with only SPC-KO BM cells (data not shown).
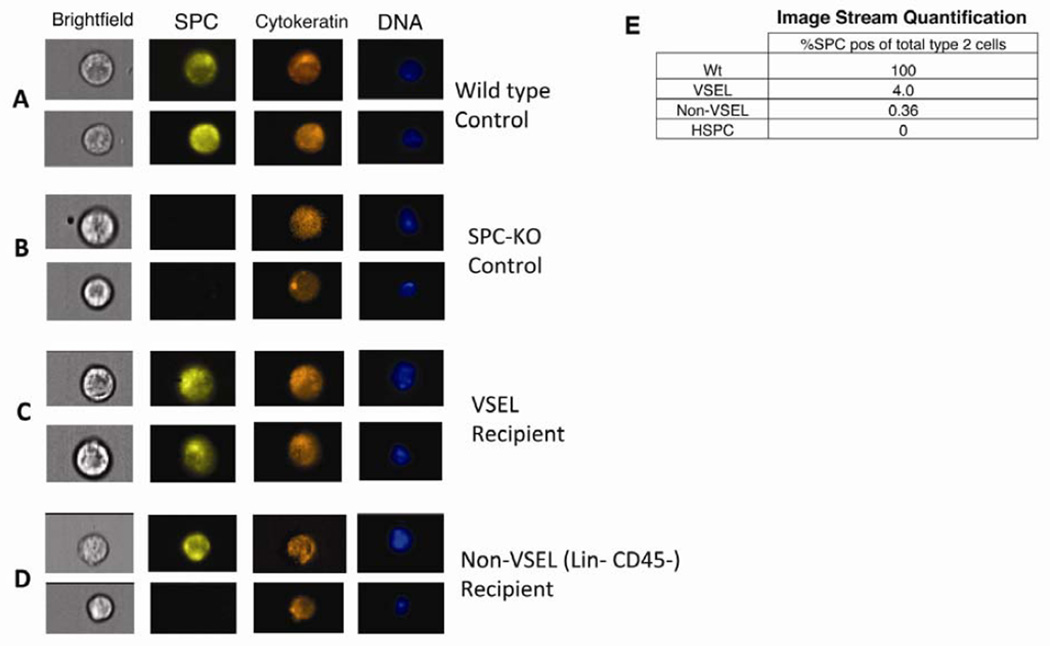
VSELs give rise to T2 cells: Detection by ImageStream. Isolated cells from lung tissue harvested from transplant recipients after 2 months were analyzed for SPC (yellow) and cytokeratin (orange). Nuclei were stained with 4',6-diamidino-2-phenylindole (DAPI, blue). Cells were analyzed using an ImageStream imaging flow cytometer with 40× magnification. Examples of 2 cells from each group are shown. A: T2 cells from a WT control express SPC in intracellular vesicles as well as cytoplasmic cytokeratin. B and D: Lung cells from SPC-KO mice transplanted with non-VSELs or HSPC express cytokeratin but not SPC. C: SPC-positive T2 cells derived from VSELs express SPC in intracellular vesicles and cytokeratin. E: Percentages of T2 cells derived from donor BM detected by ImageStream calculated based on the number of T2 cells among CD45-negative, round cells in corresponding WT samples, which was set to 100%. See also supplemental figures S1 and S3.
Detection of lung epithelial cells derived from VSELs in tissue sections
In order to assess whether VSEL-derived T2 pneumocytes exhibit T2 pneumocyte-specific morphology and location within lung tissue, donor derived SPC positive pneumocytes were assessed by confocal microscopy for SPC and TTF1 in lung tissue from SPC-KO mice transplanted with VSELs, HSPC or Lin−CD45− non-VSELs from WT mice. Again, analysis was performed 2 months post-transplant. In paraffin embedded tissue sections, SPC in intracellular lamellar bodies has a vesicular staining pattern, and the transcription factor TTF1 is expressed in the nuclei of the same cells (Figure 3A). T2 pneumocytes in WT lungs expressing SPC and TTF1 are localized in alveoli at alveolar junctions (Figure 3A). High power confocal microscopy images and cross sections through a series of images taken in different planes from the top to the bottom (z-stack) of T2 cells from WT mice confirm that the TTF1+ nucleus is surrounded by SPC+ lamellar bodies (Figure 3A). This staining pattern and morphology was used as a standard to identify SPC and TTF1 double positive cells in SPC-KO recipients. In SPC-KO mice, SPC expression is absent, but TTF1 positive T2 pneumocytes are present at alveolar junctions (Figure 3B). VSEL-derived, SPC-positive T2 cells in SPC-KO recipients were appropriately localized to the alveolar junction (Figure 3C, D, arrows), and cross sections (see z-stack) revealed SPC protein in lamellar bodies surrounding the nucleus (Figure 3C, D right panel). As expected, no SPC+ TTF1+ T2 cells were identified in lung tissue from SPC-KO mice transplanted with SPC-KO WBM.
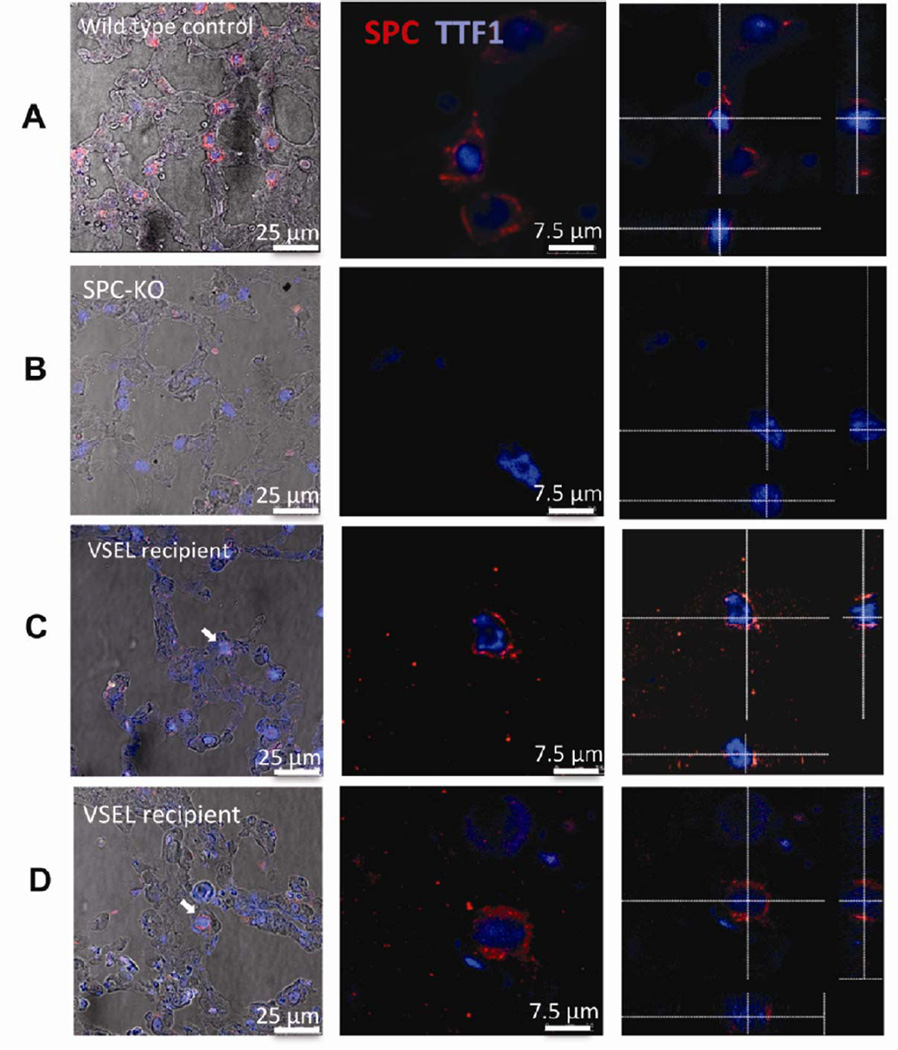
SPC-positive T2 cells derived from VSELs detected on tissue sections. Lung tissue was stained for SPC (red) and TTF1 (blue). VSEL derived T2 cells are marked with arrows. A: T2 cells from WT controls express SPC in intracellular compartments and co-express nuclear TTF1 B: T2 cells in SPC-KO lungs transplanted with non-VSELs express nuclear TTF1, but not SPC. C and D: Lungs from SPC-KO mice that received VSELs contain donor derived T2 cells located at the alveolar junction (arrows). Confocal microscopic images were taken with a 63× lens. The left-most panels show images taken at low power, merged with brightfield images to show localization of T2 cells at the alveolar junction. Middle panel: High power. Right panel: Images were taken in 0.4 µm steps from the top to the bottom of each cell. Optical cross sections through one focal plane show SPC expression in cytoplasmic vesicles surrounding the nucleus, excluding cell overlay. Cross-hairs indicate planes of optical x and y sections projected on bottom and right side.
VSELs give rise to lung epithelial cells by differentiation and not fusion
To assess whether SPC+ cells in recipients of VSELs arise from fusion events or transfer of RNA as opposed to differentiation of the BM derived cells, SPC-H2B-GFP mice, which express nuclear H2B-GFP under the T2 cell specific SPC-promoter [5], were used as bone marrow donors. VSELs were isolated from SPC-H2B-GFP mice and transplanted into SPC-KO mice that were also transgenic for ubiquitously expressed red fluorescent protein, DsRed (DsRed+SPC-KO mice, Figure 4A). If VSELs give rise to T2 cells by fusion, the resulting cell would express H2B-GFP, SPC and DsRed. If VSELs give rise to T2 cells by differentiation without fusion, the resulting cell would express SPC and GFP but not DsRed (Figure 4B). Lung tissue from Ds-Red-SPC-KO mice transplanted with either VSELs or non-VSELs was harvested 2 months after transplantation and analyzed for SPC (red), H2B-GFP (green), DsRed (orange) and CD45/F4/80 (not shown) by ImageStream analysis. Nuclei were stained with DAPI. Similar to a previous study [5], in 8 week old SPC-H2B-GFP control mice, 62% of SPC+ cells expressed H2B-GFP (310 out of 500 SPC+ cells, Figure 4C and G), and H2B-GFP was never expressed in SPC-negative cells. In DsRed+SPC-KO mice transplanted with DsRed−SPC-KO BM, no SPC+ or H2B-GFP+ cells were identified (Figure 4D and G). In 9 DsRed+SPC-KO recipients transplanted with VSELs, a total of 164 SPC+ cells derived from VSELs were identified, out of which 101 cells expressed H2B-GFP from the SPC promoter (Figure 4E and G). All of the SPC+ cells were DsRed negative (Figure 5E and G). These data demonstrate that VSELs activate the SPC-promoter during differentiation into T2 cells, and that this differentiation does not involve fusion or transfer of mRNA. Rare SPC+ cells derived from Non-VSELs also expressed H2B-GFP from the SPC promoter and lacked expression of DsRed, indicating that a rare cell type in this population is capable of differentiating into T2 cells (Figure 5F and G).
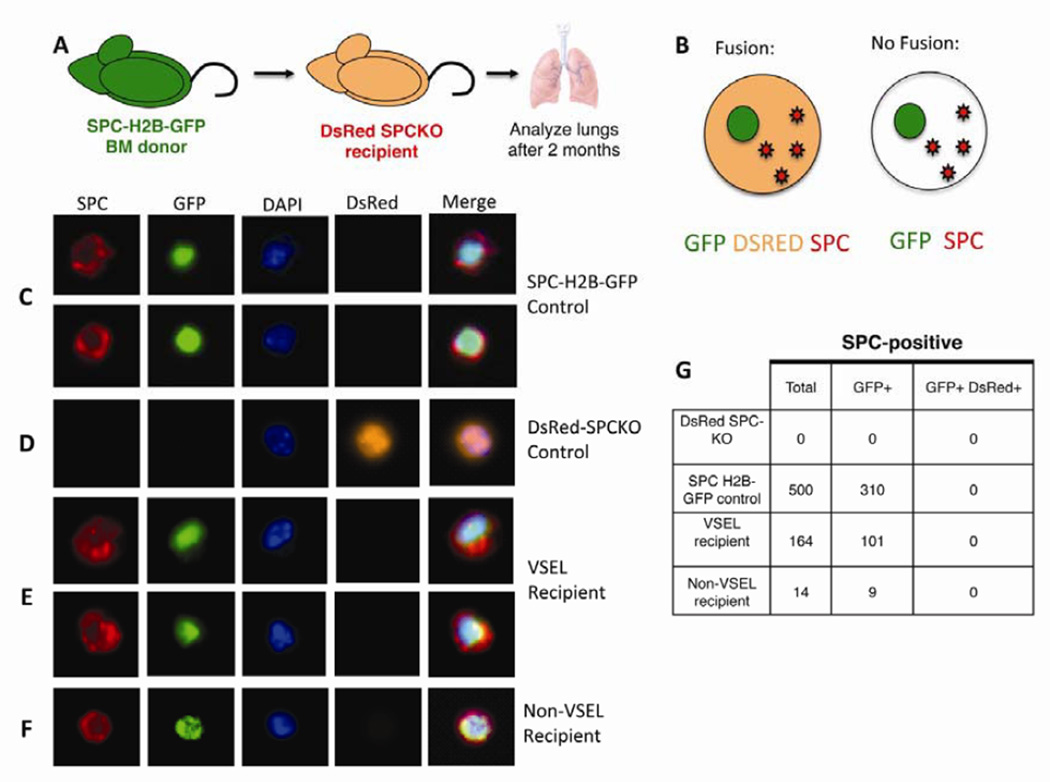
VSELs differentiate into T2 cells, activating the SPC-promoter. A: VSELs and Non-VSELs were isolated from mice expressing the nuclear H2B-GFP under the SPC-promoter and transplanted into SPC-KO recipients expressing red fluorescent protein (DsRed) under the ubiquitous beta-actin promoter. B: If VSELs give rise to T2 cells by differentiation without fusion, the resulting cell would express SPC and GFP but not DsRed. C–G: Isolated lung cells were analyzed by Imagestream for SPC (red), GFP (green), DsRed (orange) and CD45 (not shown). Nuclei were stained with DAPI. C: T2 cells from SPC-H2B-GFP WT (SPC+) controls express SPC and nuclear GFP but not DsRed. D: T2 cells from DsRed+SPC-KO mice express DsRed but not SPC or GFP. E: VSEL derived T2 cells express SPC and GFP, but not DsRed. F: Rare T2 cells derived from non-VSELs express SPC and GFP but not DsRed. Images were acquired with a 63× objective G: In DsRed+SPC=KO mice, 40–60% of T2 cells are positive for DsRed. Of 41 VSEL derived SPC-positive T2 cells, 32 were positive for SPC-H2B-GFP and none expressed DsRed.

FACS-sorting of GFP-positive VSEL-derived T2 cells and expression of SPC-mRNA. Donor derived, GFP-positive type 2 pneumocytes were sorted from SPC-KO mice transplanted with VSELs from SPC-H2B-GFP donors and analyzed for expression of WT SPC-mRNA A: Expression of Epcam and CD49f, gated on CD45−CD31−Lin− and DAPI- (viable) cells. B: Epcam+ CD49f+ double positive cells containing all SPC-positive cells were assessed for expression of GFP in a SPC-H2B-GFP mouse. GFP-positive cells were sorted. C: Epcam+ CD49f+ double positive cells in SPC-KO lungs contain 0.7% autofluorescent cells in the GFP+ channel. GFP− negative cells and autofluorescent cells were sorted. D: In SPC-KO recipients of SPC-H2B-GFP VSELs, GFP-positive cells were sorted. E: Expression of WT SPC-mRNA in GFP-positive T2 cells isolated from SPC-KO recipients. Fold increase of expression is shown compared to T2 cells from SPC-KO controls, which was set to 1.
Expression of SPC-mRNA in sorted type 2 pneumocytes from SPC-KO mice receiving VSELs
To confirm expression of WT SPC mRNA in VSEL-derived type 2 pneumocytes, we modified a method to enrich for T2 cells by flow cytometry. A previous study had shown that SPC-positive cells in the murine lung can be found within a population that expresses Epcam and CD49f and lacks markers of blood and endothelial cells [7]. Using lung cells isolated from SPC-H2B-GFP mice and gating on cells lacking CD45, CD31 and hematopoietic lineage markers and excluding dead cells, all SPC-H2B-GFP positive cells were contained with the Epcam and CD49f-double positive population (Supplemental Figure S2A), and Epcam and CD49f-double positive cells in WT mice were 90% SPC-positive (Supplemental Figure S2B). In SPC-H2B-GFP mice, 62% of Epcam and CD49f positive T2 cells express GFP (Figure 5A and B). SPC-KO mice were transplanted with VSELs from SPC-H2B-GFP donors or donors with ubiquitous expression of H2B-GFP. Lung cells were isolated from transplant recipients, and T2 cells expressing GFP were sorted by gating on Epcam and CD49f-double positive cells that also expressed GFP (Figure 5D). With this method, between 100 and 1500 donor derived GFP-positive T2 cells were isolated from each recipient lung. As positive and negative controls, Epcam and CD49f-positive T2 cells were sorted from WT and SPC-KO mice (Figure 5). Expression of WT mRNA in donor derived cells was assessed by real time PCR using primers binding in exons 2 and 3 of WT SPC-mRNA, such that no product is generated from the SPC-KO allele lacking exon 2. Wildtype SPC mRNA expression was detected in T2 cells isolated from 5 of 8 mice transplanted with VSELs, and expression levels were increased between 32 to 512 fold compared to SPC-KO controls (Figure 5E). The absence of detectable SPC-mRNA expression in two GFP+ samples collected form VSEL-recipients could be due to low engraftment levels in these animals and the presence of autofluorescent cells in the Epcam and CD49f double positive population, as low numbers of positive cells are seen in the GFP+ gate even in untransplanted SPC-KO mice (Figure 5C), which lack GFP mRNA expression. Freshly isolated VSELs do not express SPC by immunofluorescence or qPCR (Figure S3), nor do they express other lung epithelial genes including CCSP or cytokeratin (Figure S3). Therefore, expression of SPC, cytokeratin, TTF1, Epcam and CD49f in VSEL-derived T2 cells clearly demonstrates upregulation of epithelial genes by differentiation.
Discussion
Our data demonstrate that VSELs from the BM differentiate into epithelial cells of the lung, and that this process is independent of cell fusion. Of all the cell types in the bone marrow, VSELs have the highest rate of forming epithelial cells in the lung, thereby generating a cell type from a different from their presumed mesodermal germ layer derivation.
This challenges the traditional view that all adult stem cells are lineage restricted, as VSELs seem to be an adult cell population with broad differentiation potential across germ layers. Such potential is thought to be restricted to cell types involved in embryonic development. One theoretical explanation for this phenomenon is that VSELs are quiescent remnants that are deposited in mammalian tissues during development, a hypothesis originally formulated by Ratajczak et al [8]. Since VSELs exhibit a similar gene expression profile as epiblast derived cells and primordial germ cells [9], they may originate from this cell population during development. Relatively high expression of Oct4 and Nanog as well as broad differentiation potential in vivo, as demonstrated in this study, support the notion that VSELs could be derived from a very primitive cell population.
Previous work demonstrated that VSELs can give rise to pancreatic cells, neurons and myotubes in vitro [3]. Although data demonstrating differentiation of VSELs in vivo are rare, murine and human VSELs show differentiation into mesodermal cells of the osteoblastic lineage [10, 11]. In the murine study, VSELs were isolated from mice expressing GFP under control of the ubiquitous beta actin promoter, incorporated into gelatin sponge implants, and transplanted under the skin of immunodeficient mice. GFP expression co-localized with expression of the osteoblastic marker Runx-2 or with expression of PPARγ in the VSEL-sponges that contained ossicles. Non-hematopoietic mesenchymal stem cells from the BM readily differentiate into osteoblasts. Since the non-hematopoietic compartment of the BM contains VSELs, differentiation into the osteoblastic lineage suggests a potential relationship between VSELs and mesenchymal stem cells. We hypothesize that in the BM, there might be a continuum of non-hematopoietic stem cells, ranging from primitive, pluripotent stem cells (VSELs) to cells with intermediate potential (some of which may be able to give rise to lung epithelial cells) to purely mesenchymal cells that are largely restricted to mesenchymal tissues.
There was no hematopoietic engraftment from the non-hematopoietic BM cell fraction or from VSELs in our study, which is in line with previous data showing that VSELs do not contribute to hematopoiesis after BM transplantation [3, 12, 13]. A recent study showed that after culture on OP9 stromal cells and subsequent passaging in cytokine-containing methycellulose medium [12, 13], VSELs can adopt a hematopoietic fate and give rise to hematopoietic cells 25 days after initial isolation. Thus, VSELs can differentiate down the hematopoietic lineage, but the as yet undetermined stimuli necessary are lacking under the conditions tested in our study. Another study assessed cardiomyocyte differentiation of GFP+ VSELs after transplantation into infarcted hearts [14]. GFP+ cells expressing α-sarcomeric actinin were identified in the regenerating heart, suggesting that VSELs had differentiated into cardiomyocytes in vivo.
However, both of these prior studies, cytoplasmic GFP was used as a marker for VSEL-derived cells in the recipients. Cytoplasmic GFP results in diffuse cell labeling that can be difficult to distinguish from autofluorescence, especially in tissue sections. In our study, VSEL-derived type 2 pneumocytes were identified in the SPC-KO recipients based on expression of SPC in T2 cells derived from WT donor cells and based on expression of nuclear H2B-GFP. Nuclear expression of H2B-GFP is distinct from autofluorescence and allows specific detection of GFP-positive cells, as autofluorescence is not present in nuclei. Expression of H2B-GFP from the SPC-promoter allowed us to detect differentiation of VSELs into SPC-positive T2 cells that activate the SPC-promoter of the transgene. SPC mRNA and protein were detected in T2 cells derived from VSELs, but absent in freshly isolated VSELs, providing additional evidence that VSELs fully differentiated into T2 cells. In addition, VSEL-derived T2 cells express other epithelial cell specific genes, such as TTF1, cytokeratin, Cd49f and Epcam. In future studies, transcriptome analysis of isolated VSEL-derived T2 cells will help to clarify whether differentiation is complete and functional assays will be performed on isolated VSEL-derived T2 cells.
Single cell analysis by ImageStream was the most sensitive method of quantification of rare SPC+ cells in the lung. Single cell analysis allows for each cell to be analyzed independently without cell overlay or background fluorescence, facilitating detection of low fluorescent, donor derived SPC-positive cells. Paraffin embedded lung tissue sections, while providing excellent preservation of morphology, contain high levels of autofluorescence, complicating the detection and quantification of donor derived SPC+ cells on tissue sections.
Lin−CD45−non-VSEL-cells give rise to T2 cells at about 10-fold reduced levels compared to VSELs, even though we transplanted 100 fold higher cell numbers (1,000 VSELs vs. 100,000 non-VSELs). One explanation could be that the purity of sorted cell populations is never 100%, and it is possible that the non-VSEL fraction is contaminated with rare VSELs, especially since the separating feature is cell size, and bone marrow cells contain a continuum of different cell sizes rather than clearly separate populations, and it is possible that some rare VSELs might be larger than 6µm.
Another possible explanation is that there may be a separate, even more rare cell type in the non-hematopoietic BM compartment with the potential to differentiate into lung epithelial cells. A previous study by Wong et al. [15] describes a population of BM cells that expresses Clara cell specific protein (CCSP), and differentiates into epithelial cells lineages in vitro and in vivo. These cells, which may already be partially committed to the epithelial lineage, differ from VSELs in that they 1) express CD45, 2) are larger, and 3) proliferate in culture. VSELs do not express SPC or CCSP based on our qPCR data (Figure S3) and published microarray data [16]. Our data are in line with a previous study in which small, lineage negative stem cells from the bone marrow gave rise to epithelial cells of the lung [1]. We hypothesize that VSELs were contained in the cell population that was used in this previous study.
Conclusion
Our data demonstrate that VSELs from the BM are capable of fully differentiating into epithelial cells of the lung, specifically type 2 pneumocytes, activating the SPC-promoter and expressing SPC-mRNA and protein. This process is independent of cell fusion. This is the first report of VSELs differentiating into an endodermal lineage in vivo. While the biological function of VSELs is still unknown, our data demonstrate that Oct4+ VSELs in the adult BM exhibit broad differentiation potential by giving rise to lung epithelial cells. Future experiments will assess the full differentiation potential of VSELs in vivo, which may help elucidate their potential role in tissue repair and maintenance, as well as the mechanisms controlling their differentiation.
Acknowledgments
We wish to thank Mariusz Ratajczak and Celina Juliano for valuable input and helpful discussions, Ewa K. Zuba Zurma and Magda Kucia for technical advice with VSEL sorting, Gouzel Tokmoulina for expert cell sorting; and Stephanie Donaldson for assistance with animal care and breeding.
This work was supported by the following funding: HL073742 and DK61846 to DSK, the Yale Center of Excellence in Molecular Hematology (DK072442), the Yale Cancer Center (CA16359), and 1U01HL099997-01, RO1 HL090136, U01 HL100402, a Basil O’Conner March of Dimes Starter Award, and the Harvard Stem Cell Institute to CFK.
Footnotes
Author contributions: S.K.: Conception and design, collection and assembly of data, data analysis and interpretation, manuscript writing; H.J.: Collection and assembly of data; P.X.Z.: collection and assembly of data; E.B.: data analysis and interpretation, writing of manuscript; K.H.: Collection and assembly of data; J-h.L.: Provision of study material; C.K.: Provision of study material; D.K.: Conception and design, data analysis and interpretation, manuscript writing.
The authors declare no conflicts of interest.
See www.StemCells.com for supporting information available online.
References
Full text links
Read article at publisher's site: https://doi.org/10.1002/stem.1413
Read article for free, from open access legal sources, via Unpaywall:
https://academic.oup.com/stmcls/article-pdf/31/12/2759/41982313/stmcls_31_12_2759.pdf
Citations & impact
Impact metrics
Article citations
The universal stem cell.
Leukemia, 36(12):2784-2792, 28 Oct 2022
Cited by: 8 articles | PMID: 36307485 | PMCID: PMC9712109
Review Free full text in Europe PMC
Proteomic Analysis of Murine Bone Marrow Very Small Embryonic-like Stem Cells at Steady-State Conditions and after In Vivo Stimulation by Nicotinamide and Follicle-Stimulating Factor Reflects their Germ-Lineage Origin and Multi Germ Layer Differentiation Potential.
Stem Cell Rev Rep, 19(1):120-132, 20 Aug 2022
Cited by: 3 articles | PMID: 35986128 | PMCID: PMC9823037
Bone Marrow-Derived VSELs Engraft as Lung Epithelial Progenitor Cells after Bleomycin-Induced Lung Injury.
Cells, 10(7):1570, 22 Jun 2021
Cited by: 11 articles | PMID: 34206516 | PMCID: PMC8303224
SARS-CoV-2 Entry Receptor ACE2 Is Expressed on Very Small CD45- Precursors of Hematopoietic and Endothelial Cells and in Response to Virus Spike Protein Activates the Nlrp3 Inflammasome.
Stem Cell Rev Rep, 17(1):266-277, 01 Feb 2021
Cited by: 108 articles | PMID: 32691370 | PMCID: PMC7370872
Is COVID-19 a New Hematologic Disease?
Stem Cell Rev Rep, 17(1):4-8, 01 Feb 2021
Cited by: 59 articles | PMID: 32399806 | PMCID: PMC7217340
Go to all (45) article citations
Data
Data behind the article
This data has been text mined from the article, or deposited into data resources.
BioStudies: supplemental material and supporting data
Similar Articles
To arrive at the top five similar articles we use a word-weighted algorithm to compare words from the Title and Abstract of each citation.
Bone Marrow-Derived VSELs Engraft as Lung Epithelial Progenitor Cells after Bleomycin-Induced Lung Injury.
Cells, 10(7):1570, 22 Jun 2021
Cited by: 11 articles | PMID: 34206516 | PMCID: PMC8303224
Mouse Bone Marrow VSELs Exhibit Differentiation into Three Embryonic Germ Lineages and Germ & Hematopoietic Cells in Culture.
Stem Cell Rev Rep, 13(2):202-216, 01 Apr 2017
Cited by: 40 articles | PMID: 28070859
Nonhematopoietic cells are the primary source of bone marrow-derived lung epithelial cells.
Stem Cells, 30(3):491-499, 01 Mar 2012
Cited by: 25 articles | PMID: 22162244 | PMCID: PMC3725285
Very small embryonic-like stem cells: characterization, developmental origin, and biological significance.
Exp Hematol, 36(6):742-751, 01 Jun 2008
Cited by: 72 articles | PMID: 18474305 | PMCID: PMC2430762
Review Free full text in Europe PMC
Funding
Funders who supported this work.
Basil O'Conner March of Dimes Starter Award
Harvard Stem Cell Institute
NCI NIH HHS (2)
Grant ID: CA16359
Grant ID: P30 CA016359
NHLBI NIH HHS (6)
Grant ID: R01 HL090136
Grant ID: U01 HL100402
Grant ID: HL073742
Grant ID: R01 HL073742
Grant ID: R01 HL093004
Grant ID: U01 HL099997
NIDDK NIH HHS (4)
Grant ID: DK61846
Grant ID: P30 DK072442
Grant ID: DK072442
Grant ID: R01 DK061846
Wellcome Trust (1)
Regulatory signalling networks between stem and niche cells in lung regeneration.
Dr Joo-Hyeon Lee, University of Cambridge
Grant ID: 107633
Yale Center of Excellence in Molecular Hematology (7)
Grant ID: DK072442
Grant ID: HL073742
Grant ID: DK61846
Grant ID: U01 HL100402
Grant ID: 1U01HL099997-01
Grant ID: RO1 HL090136
Grant ID: CA16359