Abstract
Free full text

Natural Killer Cell-activating receptor NKG2D mediates innate immune targeting of allogeneic neural progenitor cell grafts
Abstract
Cell replacement therapy holds promise for a number of untreatable neurological or psychiatric diseases but the immunogenicity of cellular grafts remains controversial. Emerging stem cell and reprogramming technologies can be used to generate autologous grafts that minimize immunological concerns but autologous grafts may carry an underlying genetic vulnerability that reduces graft efficacy or survival. Healthy allogeneic grafts are an attractive and commercially scalable alternative if immunological variables can be controlled. Stem cells and immature neural progenitor cells (NPC) do not express major histocompatibility complex (MHC) antigens and can evade adaptive immune surveillance. Nevertheless, in an experimental murine model, allogeneic NPCs do not survive and differentiate as well as syngeneic grafts, even when traditional immunosuppressive treatments are used. In this study, we show that natural killer (NK) cells recognize the lack of self-MHC antigens on NPCs and pose a barrier to NPC transplantation. NK cells readily target both syngeneic and allogeneic NPC, and killing is modulated primarily by NK-inhibiting “self” class I MHC and NK-activating NKG2D-ligand expression. The absence of NKG2D signaling in NK cells significantly improves NPC-derived neuron survival and differentiation. These data illustrate the importance of innate immune mechanisms in graft outcome and the potential value of identifying and targeting NK cell-activating ligands that may be expressed by stem cell derived grafts.
Introduction
Neural stem/progenitor cell (NPC) transplantation is a major focus of regenerative therapy for acute and chronic conditions including ischemic stroke, spinal cord injury, Parkinson disease (PD), amyotrophic lateral sclerosis (ALS), and Alzheimer disease (AD) [1, 2]. Immune signaling that results from the disease process itself as well as allospecific recognition of a graft can influence how the transplanted cells survive, proliferate, differentiate, and/or functionally integrate [3], each of which strongly influences therapeutic benefit. Numerous studies over the last two decades demonstrate that allogeneic grafts in the CNS survive long term, even after the withdrawal of immunosuppression [4–6] but there is increasing evidence that immune activation does occur [7, 8] and that immune mechanisms beyond classical pathways of allograft rejection potently influence graft outcome.
Allogeneic NPC grafts can be well tolerated due to minimal expression of MHC class I and class II and the co-stimulatory molecules CD40, CD80 and CD86. However, we and others have shown that T cells do target allogeneic NPC when MHC and costimulatory marker expression is elevated following exposure to inflammatory cytokines [9–11]. Adaptive immune recognition is important in these contexts and considerable efforts are underway to improve graft survival through immunosuppression [12, 13] or more sophisticated strategies to manipulate the host’s immune system to induce tolerance [14, 15].
Our recent work indicates that innate immune processes also play a prominent role in graft survival and differentiation [7]. Using a model of NPC replacement therapy in the adult mouse hippocampus, we have shown that attenuation of innate immune cytokine signaling can further improve the survival and neuronal differentiation of NPCs placed into the neurogenic zone of the adult hippocampus [7]. Here we provide evidence that natural killer (NK) cells, large granular lymphocytes that can lyse target cells without prior sensitization, play a significant role in this innate immune response and that preventing NK cell activation can substantially improve neuron abundance and survival in NPC allografts.
NK cell functions are regulated by a complex repertoire of NK-activating and inhibiting cell surface receptors. Among these, the killer cell immunoglobulin-like receptor (KIR) family (Ly49 family in mice) of receptors bind self MHC class I molecules (HLA-A, -B, and –C) [16, 17] resulting in suppressed NK cell activation. Consequently, the absence or low expression of MHC I makes NPCs potential targets for elimination by NK cells if the NPCs also express NK cell activating ligands. Activating NK cell ligands that are expressed during stress, infection, or transformation are recognized by several NK activation receptors, including NKG2D. These receptors signal to induce NK cell activation, production of cytokines, and degranulation of the cytotoxic mediators perforin and granzymes [16, 18]. In the present study, we show that mouse NPCs do express NK cell-activating ligands and are efficiently killed by NK cells. We also show that upregulation of self-MHC class I, but not allo-MHC class I, on NPC can reduce NK cell-mediated killing. Finally, we show that the primary NK cell activating ligand in mouse NPCs is Rae1 and that genetic loss of the Rae1 receptor NKG2D significantly improves the abundance and survival of neurons in NPC allografts.
Materials and Methods
Animals
C57BL/6J (H-2b) (B6) and BALB/c (H-2d) mice were purchased from Jackson Laboratory (Bar Harbor, ME). NKG2D−/− and NKp46−/− mice (both on the C57BL/6 background) were kindly provided by David Raulet (UC Berkeley). All mice were maintained in pathogen-free conditions. All animal studies were performed according to NIH humane use of animal guidelines and reviewed and approved by the Administrative Panel on Laboratory Animal Care (APLAC) at Stanford University.
Gene Expression Analysis
CEL file data was downloaded from the GEO repository and analyzed using dCHIP [19]. Data from GEO GSE18326 and GEO GSE18765 were analyzed separately. In brief, hybridization signal was first normalized using a running median for an invariant probe set and Model-based expression calculated with mismatch probe (PM/MM difference) background subtraction. Probe lists were then used to extract relative expression levels for the subset of probes corresponding to NK cell activating ligands, MHC class I, MHC class II and minor histocompatibility genes, as well as genes required for MHC class I-mediated antigen processing and presentation. %P calls is calculated as the fraction of arrays where signal is called present for a given probe set.
Cell Cultures
Primary NK cells were isolated from whole splenocytes using the EasySep® Mouse NK Cell Enrichment Kit (Stem Cell Technologies, Vancouver, BC). Enriched NK cell purity was verified as >90% and cells were cultured and expanded in complete RPMI 1640 (Mediatech, Manasses, VA) + 10% FCS (Serum Source International, Charlotte, NC) + 1% penicillin/streptomycin (Mediatech) supplemented with 50 µM 2-ME (Sigma-Aldrich, St. Louis, MO), 1% sodium pyruvate (Life Technologies, Invitrogen, Carlsbad, CA), 1% Non-essential amino acids (Life Technologies), 0.5% HEPES (Life Technologies), and 1000 IU/ml human rIL-2 (Biological Resources Branch, NCI, MD) for at least 72h before use in assays.
Primary NPCs were isolated from neonatal pups aged 1–6 days. Using methods previously described [20] whole brains (minus olfactory bulbs, cerebellum and brain stem) were pooled and enzymatically dissociated in a mixture of DNAse and Papain (Worthington Biochemical Corp., Lakewood, NJ). Neurospheres were cultured in proliferation medium consisting of Neurobasal-A with B27 additive (Gibco, Grand Island, NY), L-Glutamine, 20 ng/ml recombinant human fibroblast growth factor 2 (FGF2, Peprotech), and 20 ng/ml recombinant human epidermal growth factor, (EGF, Sigma). For experiments, single cell suspensions were generated by plating spheres onto Poly-D-ornithine (Sigma) / Laminin (Invitrogen) coated dishes and passaging once to obtain a monolayer. Attached cells were dissociated with Accutase (Innovative Cell Technologies, San Diego, CA) to maintain surface marker expression. Where indicated, wild type C57BL/6 or BALB/c NPCs were labeled with GFP via infection with replication deficient GFP-expressing concentrated lentivirus FUGW [21] and sorted on a FACS Vantage DiVa (Becton Dickinson, San Jose, CA) to obtain a stably expressing line. Where indicated, NPC were differentiated by standard growth factor withdrawal [7]. NPCs were cultured no longer than 15 passages.
Flow Cytometry
Flow cytometry was performed on a FACScan flow cytometer (BD) using CellQuest data acquisition (BD) and FlowJo analysis software (Tree Star, Ashland, OR). Briefly, cells were washed with FACS buffer (PBS containing 1% bovine serum albumin and 0.02% sodium azide) and incubated with the appropriate conjugated antibodies for 30 minutes on ice. Cells were again washed and resuspended in 0.2 ml FACS buffer, and 2 × 104 cells live cell events were collected and analyzed. Live cell populations were determined by propidium iodide exclusion and forward and side scatter characteristics. Antibodies for immunological characterization of NPC were as follows: anti-MHC class I, anti-MHC class II (both from eBioscience, San Diego, CA), anti-Rae-1, anti-Multi1, anti-H60 (all from R&D Systems, Minneapolis, MN). Recombinant mouse NKp46/NCR1/Fc chimeric fusion protein (R&D Systems) was used to probe for putative NKp46 ligands. CD30-Fc fusion protein was used as a negative control. Cells were incubated with 4 µg/106 cells of fusion protein for 30 min on ice, washed twice, and incubated with RPE-conjugated goat anti-human Fc Ab (1:200; Jackson ImmunoResearch Laboratories) for 30 min on ice, and propidium iodide was added to cells before flow analysis. Antibodies for NK cell characterization were as follows: anti-CD49b (eBioscience), anti-NK1.1 (AbD Serotec, Raleigh, NC), anti-CD3 (BD).
NK cell cytotoxicity assay
The JAM method to measure DNA fragmentation was used to detect NK cell cytotoxicity against NPC [22]. Briefly, target NPC were labeled with 3H-thymidine (1 µCi/ml; PerkinElmer, Boston, MA) with or without IFN-γ (10 ng/ml) (Peprotech, Rocky Hill, NJ) for at least 48 h. MHC class I upregulation was verified prior to performing cytotoxicity assay. YAC-1 control cells were labeled with 3H-thymidine (5 µCi/ml) for 24h. Washed target cells were plated with and without effector cells at 104 target cells/well in 96-well U-bottom plates. NK cells were washed prior to plating at varying E:T ratios for 4h. Cells were harvested onto a glass fiber filtermat (PerkinElmer) using a Tomtec Harvester 96 Mach II (Tomtec, Hamden, CT), and a Wallac 1205 betaplate reader (PerkinElmer). Percentage of specific killing was calculated as follows: (cpm of spontaneous lysis without effector cells − cpm of experimental killing)/(cpm of spontaneous lysis) × 100. Where indicated, NK cells were washed and then incubated with 50 ug/ml of NKG2D blocking antibody CD314, C7 (eBioscience) for 30 minutes at 37°C prior to assay.
NPC Transplantation
Monolayer GFP-labeled NPCs were dissociated with accutase, washed and prepared as a single cell suspensions for stereotaxic injection as previously described [20]. Cells were suspended in D-PBS with 100 ng/ml FGF at the concentration of 100 million cells per ml. 100,000 cells were stereotaxically transplanted into the hippocampi (A/P, −0.2 cm; M/L, ±0.14 cm; D/V, −0.23 cm, and A/P, −0.3 cm; M/L, ±0.22 cm; D/V, −0.26 cm) of mice two months of age.
Tissue Preparation and Immunohistochemistry
Two weeks after transplantation, mice were anesthetized and transcardially perfused with saline and 4% paraformaldehyde. Brains were removed, post fixed for 24 hrs and then equilibrated in phosphate buffered 30% sucrose. Free-floating 40-µm sections were collected on a freezing microtome and stored in cryoprotectant as described [23]. Immunostaining was performed as previously described [23] using the following primary antibodies and working concentrations: mouse anti-NeuN (1:500, Chemicon, Billerica, MA), rabbit anti-NG2 (1:1000, gift from William Stallcup), goat anti-Dcx (1:500, Santa Cruz Biotechnology, Santa Cruz, CA), guinea pig anti-GFAP (1:1000, Advanced Immunochemicals, Long Beach, CA) rabbit anti-GFP (1:1000, Molecular Probes, Carlsbad, CA), rat anti-CD4 (1:500, eBioscience), rat anti-CD8 (Biotinylated, 1:300, eBioscience). To detect NK cells in the brain, we tested CD49b, NK1.1, and NKp46, and found the most reproducible and specific labeling in the brain with goat anti-NKp46/NCR1 (1:500, R&D Systems). Donkey secondary antibodies were used at 1:500 for all cases (Jackson ImmunoResearch, West Grove, PA).
Confocal microscopy
All confocal microscopy was performed using a Zeiss 510 confocal microscope (Thornwood, New York). Appropriate gain and black-level settings were determined on control tissues stained with secondary antibodies alone. Upper and lower thresholds were always set using the range indicator function to minimize data loss through saturation. Cell counts were limited to the hilus, the granule cell layer, and the subgranule zone. The proportion of GFP+ cells displaying a lineage-specific phenotype was determined by scoring the colocalization of cell phenotype markers with GFP using confocal microscopy. Split panel and z-axis analysis were used for all counting. All counts were performed using multi-channel configuration with a 63× objective and electronic zoom of 0.7. When possible, 100 or more GFP+ cells were scored for each marker per animal. Each cell was manually examined in its full 'z'-dimension and only those cells for which the nucleus was unambiguously associated with the lineage-specific marker were scored as positive.
Statistics
Differences between more than two groups or conditions were tested with parametrical two-way analysis of variance (ANOVA), using Bonferroni-Dunnett corrections as appropriate. Asterisks indicate post-hoc significance levels. Differences between two groups were tested using unpaired two-tail Student’s t test. The level of significance was set at α=0.05. Asterisks indicate significance levels.
Results
NPC express NK cell-activating ligands and minimal MHC class I
To determine if NPC express ligands for NK cell activating or inhibitory receptors that would influence NK cell function, gene expression data from our earlier work (GEO accession GSE18326 [24]) as well as expression data from Beckervor der Sandforth et al (GEO accession GSE18765 [25]) was evaluated for immune molecule transcripts. Expression patterns for activating and inhibitory ligands were remarkably consistent across two genotypes and several cellular subtypes located in the subventricular ventricular zone (Fig 1) and revealed that NPCs expressed genes that both inhibit and activate NK cells. Transcripts for members of the NKG2D receptor ligand family Rae1 (Raet1a-e) [26] were detected. Mult1 transcripts were called present on most arrays but expressed at very low levels [27]. This was similar for the NK cell-activating minor histocompatibility antigen H60 [28]. Inhibitory receptors predominantly recognize self MHC class I [29] and the array data indicated the expression of the classical MHC class I genes, H-2K, D, and L, as well as low levels of other class I or class II genes. NPCs express notable levels of H47 transcript which encodes a minor histocompatibility epitope implicated in T cell activation [30–32] and which is speculated to have structural features that may activate NKG2D when presented by class I MHC [30]. Transcripts for the beta-2 microglobulin (β2M) subunit of MHC class I were present and transcripts for proteins involved in MHC class I-mediated antigen processing and presentation were also present. A notable exception was the very low to absent expression of transcripts for the antigen peptide transporters Tap1 and Tap2 [33]. Both are required for antigen loading, MHCI assembly, and cell surface presentation. Expression patterns in NPCs were similar to those seen in populations enriched for other cell types such as astrocytes and differentiating neuronal progenitors. Patterns were also consistent across different strains of mice, indicating that immune-related transcript abundance is conserved across several cell types in the CNS.

NK cell activating and inhibitory ligand expression. Gene expression data from cultured NPCs (GEO GSE18326[24]) and prospectively isolated stem/progenitor cells from the mouse subependymal zone (GEO GSE18765[25]) were analyzed for NK cell-related ligand transcript abundance. Probe sets are ordered vertically by MHC class for each data set. For each probe, the % of arrays where the transcript was called “present” (%P calls) is indicated along with the relative expression value for the probe set. NK-relevant genes that are consistently expressed or absent are highlighted.
To determine whether gene products were present on the cell surface, single cell suspensions of NPC were analyzed by immunofluorescent staining and flow cytometry. Consistent with other reports [9, 11, 34], and the very low abundance of Tap1/2 transcripts, NPC express little to no cell surface MHC class I (Fig 2A). Additionally, NPC show robust surface expression of the NKG2D ligand, Rae1 (Fig 2B, top panels). Consistent with lower transcript abundance, NPC do not express detectable levels of other NKG2D ligands, H60 or MULT-1 (Fig 2B, middle and lower panels). Since specific NKp46 ligands have not been identified, an NKp46Fc chimeric protein was used to investigate putative NKp46 binding proteins expressed on NPC. As shown in Fig 2C, NKp46Fc bound to NPC suggesting that NKp46 ligands are expressed on NPC. Taken together, these data indicate that NPC express very low levels of MHC class I and moderate levels of the NKG2D ligand Rae1, as well as ligands for NKp46, suggesting NPC may be targets for NK cell-mediated lysis.
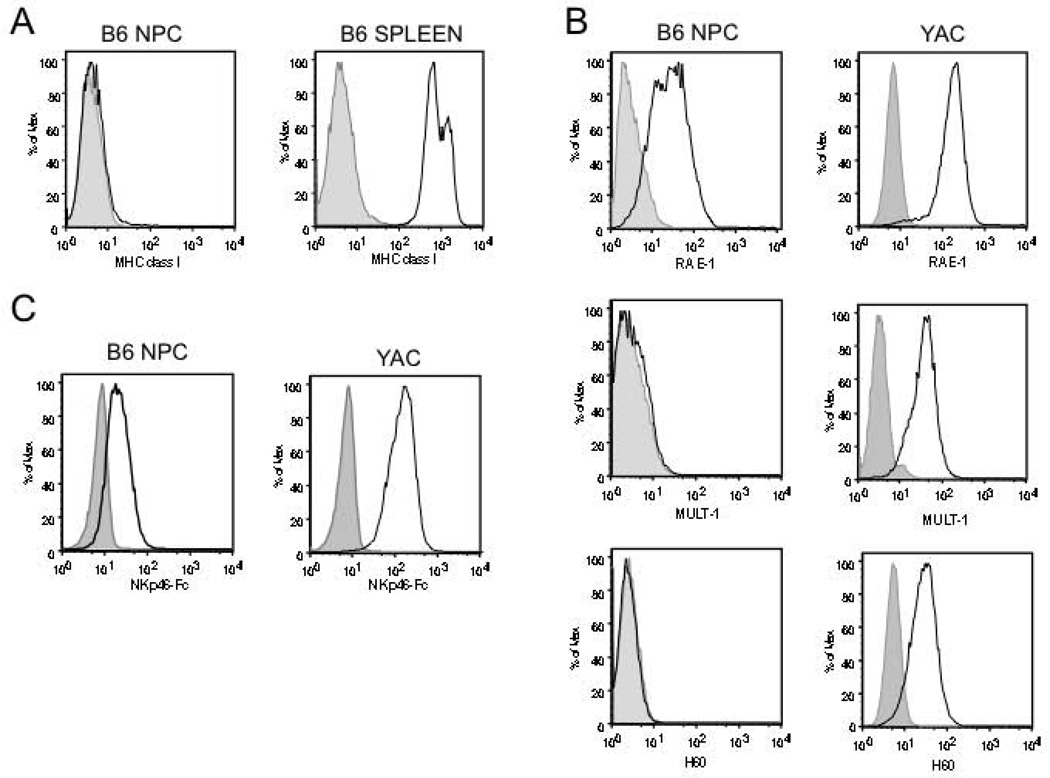
Expression of MHC class I and NK cell activating ligands on NPC. A, Expression of MHC class I on NPC or splenocytes (positive control). B, Rae-1, MULT-1, and H60 expression on NPC or YAC-1 cells (positive control). C, NPC or YAC-1 were incubated with NKp46-Fc or an unrelated fusion protein CD30-Fc. All test antibodies or fusion proteins are shown as solid lines, isotype or fusion protein controls are in shaded grey.
NK Cells Lyse Both Syngeneic and Allogeneic NPC
To test whether NK cells can target NPC in vitro, NPC cytotoxicity was assessed using a DNA fragmentation assay [22]. Primary wild type (WT) C57BL/6 NK cells were cultured with allogeneic BALB/c or syngeneic C57BL/6 NPC for 4 hours and cytotoxicity determined. NK cells readily kill syngeneic or allogeneic NPC over a wide range of effector to target (E:T) ratios, at levels comparable to the killing of YAC-1 cells, an NK cell tumor target (Fig 3A). To determine the role of self-MHC in the observed NK cell recognition of NPC, NPC targets were treated with IFN-γ (10 ng/ml) to upregulate cell surface MHC class I prior to NK cell-NPC co-culture. Within 48h hours of IFN-γ treatment, surface expression of MHC class I on NPC is robustly upregulated (Fig 3B). B6 NPC targets expressing high levels of self MHC class I showed significantly reduced killing by syngeneic B6 NK cells, consistent with the ability of self MHC to inhibit NK cell cytotoxicity (Fig 3C). In contrast, high levels of allogeneic MHC class I did not significantly alter B6 NK cell killing of BALB/c derived-NPC (Fig 3D). These findings suggest that upregulation of self-MHC in an inflammatory context can inhibit NK cell killing of syngeneic NPC, but allogeneic NPCs remain vulnerable to NK-mediated lysis.

A, C57BL/6 NK cells incubated with syngeneic NPC (dashed line), allogeneic NPC (dotted line), or YAC-1 (solid line) at a range of E:T ratios. B, FACS analysis for MHC class I of NPC treated with IFN-γ (dotted line), untreated NPC (solid line), isotype (grey). C, D, C57BL/6 NK cells incubated with C) syngeneic NPC (closed squares), IFNγ-treated allogeneic NPC (open squares) or D) allogeneic NPC (closed triangles), IFNγ-treated allogeneic NPC (open triangles). The data shown are means of several replicates (n ≥ 5) ± SEM. * p < 0.05, **p < 0.01, ***p<0.001.
NK Cell Lysis of NPC is NKG2D-Dependent and NKp46-Independent
Since NPC were shown to express activating ligands for both NKG2D and NKp46 (Fig 2B, C), we next asked how these two receptors are involved in NK cell cytotoxicity of NPC. To address this, NK cells were isolated from mice deficient for the NKG2D or NKp46 receptors (both on the C57BL/6 background) and cultured with syngeneic and allogeneic NPC in cytotoxicity assays. The absence of NKG2D in NK cells significantly protected both syngeneic and allogeneic NPC targets and yielded approximately two fold less killing than WT NK cells at an E:T ratio of 1:1 (Fig 4A,B). Similarly, NKG2D-deficiency protected YAC-1 control target cells against NK mediated lysis (Fig 4E). To ensure that the observed decrease in cytotoxicity did not reflect an intrinsic defect in cytotoxic function of NK cells from NKG2D−/− mice, experiments were repeated using WT NK cells treated with the NKG2D neutralizing antibody, CD314-C7. When WT NK cells and syngeneic NPC were cultured in the presence of the NKG2D blocking antibody, CD314-C7, cytotoxicity was diminished to levels comparable to those observed with NKG2D−/− NK cells (Fig 4C). Treatment of syngeneic NPC with IFN-γ modestly reduced killing by NKG2D−/− NK cells but had no effect on killing of allogeneic NPC (Supplemental Figure 1). In contrast, loss of NKp46 in NK cells was not protective to either syngeneic or allogeneic NPC targets, indicating that the molecules on NPC that bind the NKp46Fc probe do not significantly affect NK cell lysis of NPC (Fig 4D, E). Together, these data suggest that NK cell lysis of NPC in the mouse relies principally on a balance between self-MHC class I inhibitory signals and NKG2D-mediated stimulatory signals.
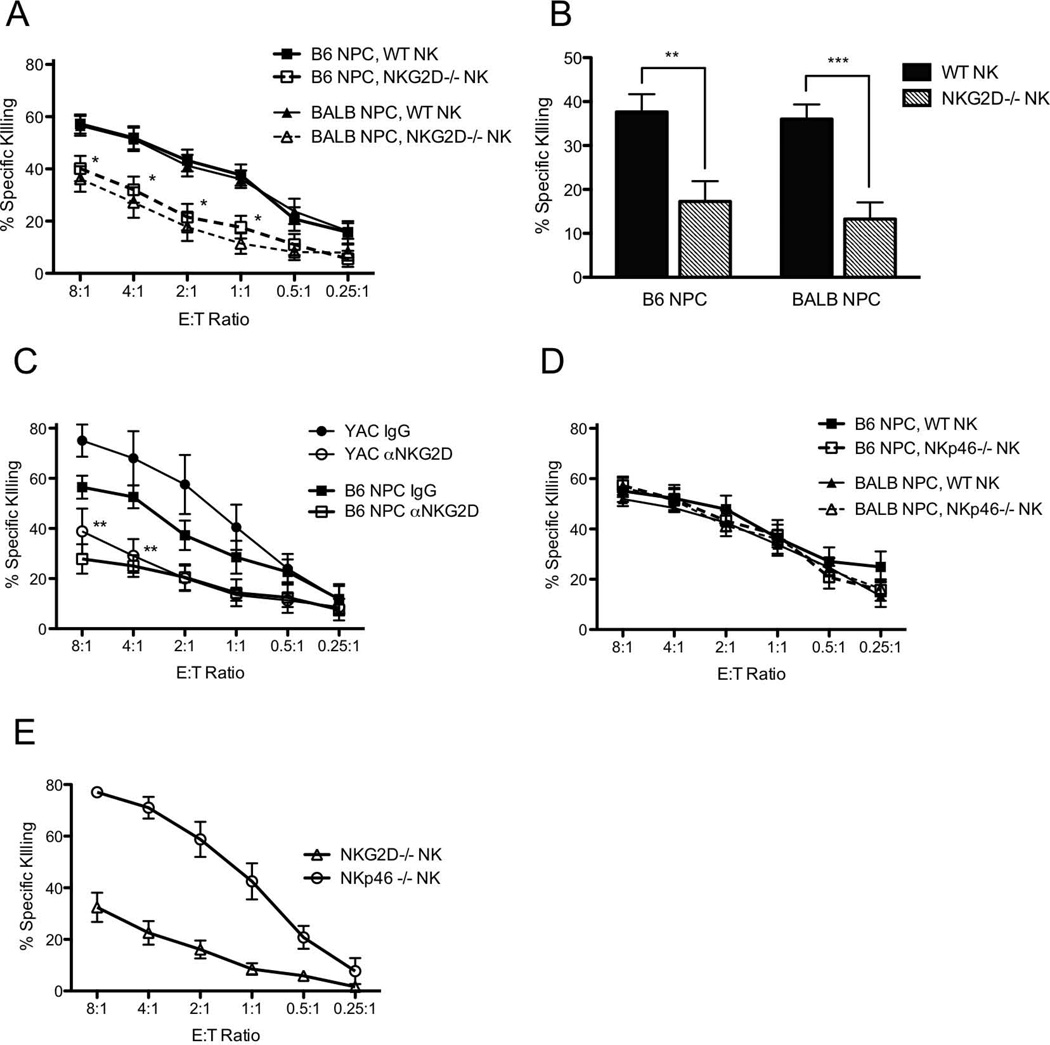
A, WT C57BL/6 NK cells (solid lines, closed symbols) or primary NKG2D−/− NK cells (dashed lines, open symbols) were incubated with B6 syngeneic (squares) or BALB/c allogeneic (triangles) NPC. Data analyzed by 2-way ANOVA, with post-hoc pairwise testing. B, Specific killing at E:T 1:1, as seen in (A), compared with Student’s T-test. WT NK cells (black bars), NKG2D−/− NK cells (gray bars). C, WT NK cells incubated with NPC (squares) or YAC-1 (circles) in the presence of NKG2D blocking antibody, αNKG2D Ab (open symbols) or isotype control IgG (closed symbols). D, WT C57BL/6 NK cells (solid lines, closed symbols) or primary NKp46−/− NK cells (dashed lines, open symbols) were incubated with B6 syngeneic (squares) or BALB/c allogeneic (triangles) NPC. WT killing curves are the same as those shown in (A). E, NKG2D−/− NK (triangles), and NKp46−/− NK (circles) incubated with positive control YAC-1 target cells. All data shown are means of several replicates (n ≥ 4) ± SEM. *p<0.05, ** p < 0.01, ***p<0.001.
NK Cells Traffic to Site of NPC Transplantation
To determine if NK cells are recruited into the brain following a NPC transplant, mice were injected with 1µl of either saline (sham) or GFP-expressing allogeneic NPC (1×105 cells/µl) and sacrificed at 8 hours or 1, 3, 5, 7, or 14 d later. Tissue sections were prepared and labeled with isotype control or anti-NKp46 antibodies to identify NK cells. Numerous NKp46+ cells can be visualized at the GFP+ allograft site (Fig 5A) with peak infiltration observed at 7 days post transplant. NK cells are also found in the nearby choroid plexus, which is responsible for production of cerebral-spinal fluid (CSF) and acts as the blood-CSF barrier (Fig 5B, right panel). Taken together with our in vitro findings, these results indicate that host NK cells traffic to the site of NPC transplantation and may influence transplant outcome.
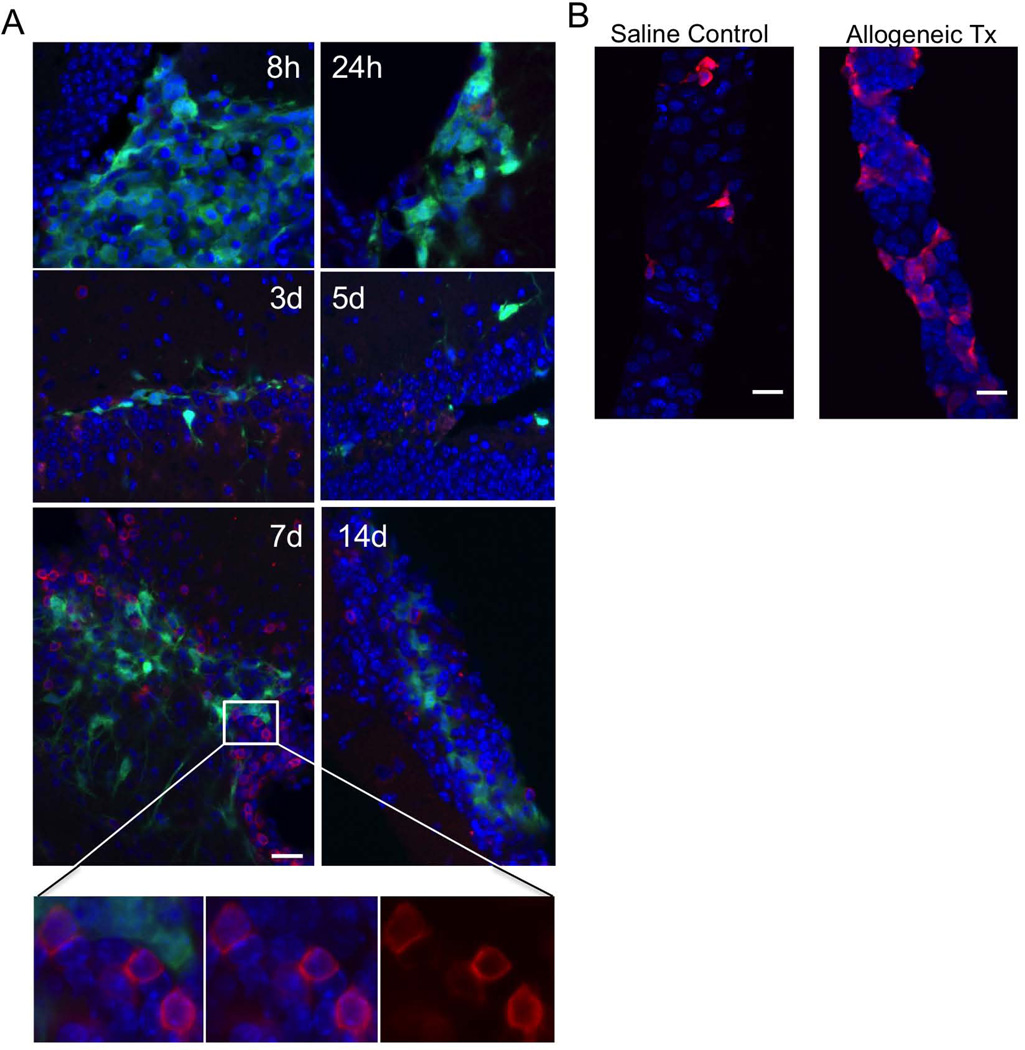
Representative images of A, Transplant site (dentate gyrus) at several time points after allograft. B, Choroid plexus 1 day after sham injection (left panel), 7 days after allograft (right panel). Blue, DAPI; Green, GFP. Red, NKp46. 40× magnification, 225×225µm field of view, Scale bars, 20 um. For close up images of NK cells, 40× magnification, digital zoom=3, 75×75µm field of view.
NPC Allografts Produce More Neurons in NKG2D Deficient Hosts
We first determined whether loss of NKG2D influenced the relative abundance of endogenously produced young neurons in the DG. NKG2D−/− and WT brains were collected and stained for doublecortin (Dcx) and NeuN. In response to signaling present in the adult DG, NPCs will divide and differentiate into granular layer neurons. These newborn neurons initially express Dcx and then gradually begin to express NeuN as they mature. Ultimately Dcx expression is extinguished in mature NeuN+ neurons. The relative number of cells that are positive for Dcx alone (immature), Dcx/NeuN (transitional), or NeuN alone (mature) provides an indication of the relative abundance and maturation rate of new neurons in the DG. The relative abundance of newborn neurons was not significantly different between NKG2D−/− and WT animals (Fig 6A, B).

A, Numbers of Dcx+ and NeuN+ cells in a defined surveyed area of the dentate gyrus. B, Ratio of Dcx+ cells to NeuN+ cells in the rostral arm of the left hippocampus. C, Total number of surviving GFP+ cells in the hippocampus two weeks post transplant. WT n=7, NKG2D−/− n=9, *p≤0.05. D, Proportion of GFP+ cells within the DG expressing Dcx or NeuN two weeks post transplant. NKG2D−/− ALLO n=11, NKG2D SYN n=5, WT ALLO n=7, WT SYN n=10, *p<0.05. **p<0.01. E, NPC were differentiated in vitro for 7 days and analyzed for Rae1, Tuj1, and Map2ab expression by flow cytometry on days 4 and 7. F-G, Representative images of WT ALLO (F), NKG2D−/− ALLO (G), and mature NeuN+ (G, zoomed panel) neurons derived from NPC grafts. Green, GFP; Red, NeuN; White, Dcx. Yellow arrows indicate cells triple positive for GFP, Dcx, and NeuN. 40× magnification, 225×225µm field of view, Scale bars, 20 um. For close up images of transplanted cells, 40× magnification, digital zoom=3, 75×75µm field of view.
To determine if loss of NKG2D protects NPC allografts placed in the DG, NPC were labeled with GFP using lentiviral transduction as previously described [7]. Single cell suspensions of GFP-expressing BALB/c or C57BL/6 NPC were prepared and introduced by stereotaxic injection into the DG of WT C57BL/6 or NKG2D−/− mice. After 2 weeks, the mice were perfused and the brains were evaluated by immunostaining to characterize the survival and phenotype of the GFP+ transplanted cells. The total number of surviving GFP+ NPCs was quantified using unbiased stereology and the fraction of NPCs adopting a neuronal fate estimated by confocal co-localization of GFP with Dcx and NeuN.
The survival of GFP+ cells was increased by loss of NKG2D (Fig 6C, p≤0.05). Allografts in NKG2D−/− hosts showed significantly more neurogenesis than wild type hosts, with 3 times more transitional and mature neurons expressing NeuN (Fig 6D, p<0.01, 39.2% ± 4.3, n=11 vs. 13.2% ± 2.5, n=7 NeuN single positive and Dcx/NeuN double-positive cells). Neurogenesis in NKG2D−/− mice receiving NPC allografts is restored to levels comparable to that seen in WT animals receiving syngeneic transplants. Similar results were observed in NKG2D−/− hosts that received syngeneic grafts. Most GFP+ neurons in the WT hosts expressed Dcx only whereas a significant fraction of graft-derived cells in NKG2D−/−hosts also co-expressed NeuN (Fig 6 D, F, G), suggesting that NKG2D-mediated effects were most pronounced on neurons of a slightly more mature phenotype. To determine if this result is consistent with Rae-1 expression on neurons of varying maturation states, NPC were differentiated in vitro for 7 days and analyzed for class III β-tubulin (Tuj1), Map2ab, and Rae-1 expression on days 4 and 7 by immunofluorescent staining and flow cytometry. Early progenitors and newborn neurons, expressing Tuj1 and no Map2 on day 4, express moderate to high levels of Rae1 (Fig 6E, left panels). By day 7, maturing neurons expressing Map2 and reduced Tuj1 show diminished Rae1 expression, consistent with previous reports that analyzed Rae expression levels in the developing and adult brain (Fig 6E, right panels) [26, 35].
Discussion
NPC and other stem cell-like transplants in the CNS have long been thought to benefit from immune privilege [3, 36, 37], resulting from reduced or absent MHC expression and the CNS environment. The CNS tightly regulates leukocyte entry and adaptive immune recognition [3, 38] and the absence of MHC is beneficial for evasion of adaptive immune responses. However, the absence of MHC also triggers a “Missing-self” response by NK cells of the innate immune system [29]. Still, several reports have indicated that NPC, as well as other stem cell populations, are resistant to NK cell lysis [9, 39–41]. In contrast, we have demonstrated that NK cells target both syngeneic and allogeneic NPC as efficiently as they target the NK-sensitive mouse lymphoma line, YAC-1. Other reports have shown the susceptibility of embryonic stem cell (ESC) and induced pluripotent stem cell (iPSC) lines to NK-mediated lysis [42], indicating that innate recognition and killing is not unique to CNS transplantation. The varying results observed across these studies may be a result of inconsistent NK activation, since the cytotoxic activity of NK cells ex vivo strongly depends on their activation state. We find that NK cell susceptibility can be attenuated by increasing self MHC expression, suggesting that evoking MHC I expression prior to transplant may be beneficial for autologous grafts. Clearly, this approach is not recommended for allografts due to enhanced adaptive immune recognition and rejection. The divergence of MHC class I effects between autologous and allogeneic cellular grafts highlights the importance of tailoring immune interventions to match the allogeneic or autologous context of the approach.
Neuronal survival and integration is a central goal in many therapeutic strategies and our present data is clinically relevant to both allogeneic and autologous cellular grafts. If the cell-intrinsic disease in autologous cell transplant can be overcome [43, 44], then a transplantation approach to restore or augment CNS function would need to consider increasing self-MHC expression on stem cell autografts and blocking activating ligand expression. In mouse models, we have shown that NPC express high levels of Rae1, an NKG2D ligand, in agreement with other reports [11, 42], and are the first to report the presence of NKp46 ligands on NPC. Indeed, blocking NKG2D/Rae1 interactions in vitro, either genetically or with soluble antibodies, partially protects NPC from lysis, indicating that Rae1 is a significant stimulus of NK cytolytic activity. While we show that NKp46 ligands do not appear to play a role in NK cell-mediated lysis, NKp46 activation may influence cytokine production or other NK cell functions, leading to changes in subsequent immune recognition, activation, graft survival, and/or differentiation. Further studies to address this possibility are underway.
Rae1 is not expressed in most normal adult tissue, but is present in early embryonic stages, particularly in the nervous system [35, 45] and is expressed at high levels in embryonic stem cells [39]. Interestingly, it has recently been demonstrated that Rae1 expression persist in the subventricular zone (SVZ) of adult mice [46], an area of ongoing neurogenesis. NKG2D expression was not detected in the SVZ and Rae1 was shown to play a non-immune role in supporting SVZ-derived neural stem cell proliferation. While Rae1 expression on NPC in culture may function independent of the immune system, it is likely that following transplantation, inflammation and endogenous TLR “danger signals” may increase or maintain Rae1 expression in graft-derived cells as they differentiate [47, 48], since NPC have been reported to express TLR [49, 50]. In our specific model of NPC reconstitution in adult neurogenic zones, such effects may also influence endogenous NPC activity and fate. Loss of Rae1 expression as NPC differentiate and mature, as demonstrated in vitro, may protect them from NKG2D-mediated recognition in vivo. This suggests that absence of NKG2D signaling likely benefits NPC most early after transplantation allowing them to survive and further differentiate. This is reflected in the increased number of mature neurons observed in grafts placed in NKG2D−/− hosts.
Though immune responses in the brain are distinct from those in the periphery, the brain is not devoid of a robust immune system or incapable of mounting both innate and adaptive responses to tissue injury, degenerative disease, or infection in the CNS (reviewed in [38]). When the immune stimulus or injury is sufficiently large, as it would be in any diseased state contemplated for cellular therapy, resident glial cells (both astrocytes and microglia) are capable of activation and production of a full complement of cytokines and chemokines that mediate the recruitment and activation of cells from the peripheral immune system. We noted recruitment of NK cells from the periphery after allotransplantation, even in an otherwise healthy brain. The surgery required for NPC transplant disrupts the blood-brain barrier (BBB), which may assist the recruitment of peripheral immune cells. NK cell-trafficking through the choroid plexus is also robust after allotransplant and, unlike the intact BBB, the choroid plexus lacks endothelial tight junctions that normally limit leukocyte diapedesis [51]. It is plausible that NK cells enter the graft through both microvasculature and CSF via the choroid plexus.
Consistent with the ability of NK cells to target NPC in an NKG2D-mediated manner, survival and neuronal differentiation of NPC transplants is improved in NKG2D deficient animals. Since NKG2D expression is not detected in normal brain and NKG2D deficient mice have normal endogenous neurogenesis, the absence of NKG2D signaling is most likely to benefit graft outcome through immune cells that are recruited to the brain following transplantation. In vitro, we have shown that Rae1-NKG2D signaling in NK cells is important in NK-mediated lysis. In vivo, additional cell types may contribute to NKG2D effects. NK cells, NKT cells, and some gamma-delta (γδ) T cells constitutively express NKG2D. CD8+ T cells also express NKG2D upon activation [35, 52]. NKG2D functions as a costimulatory receptor for CD8+ T and γδ T cells following encounter with cells expressing NKG2D ligands. This results in enhanced effector functions and increased cell proliferation [53]. In NKT cells, NKG2D can act as a costimulatory molecule or can activate TCR-independent cytolysis, similar to NK cells [54]. Since NKG2D expression is not restricted to a single cell type in vivo, the improvement in graft outcome could reflect a direct role for NK cells or NKT cells, and/or reflect a disruption of the ability of these innate cells to effectively potentiate a subsequent adaptive immune response. It is also possible that lack of NKG2D costimulation results in a dampened CD8+ T cell cytotoxic response. However, syngeneic grafts benefit similarly to allogeneic grafts in NKG2D-deficient animals suggesting that the observed effect of NKG2D deficiency is primarily due to an NK cell mediated effect and not to an adaptive, T cell-mediated response.
Conclusion
Our findings demonstrate that NPC express NK cell activating ligands that make them highly susceptible to killing by syngeneic and allogeneic NK cells. We show that mouse NPC are targeted primarily through a Rae1-NKG2D interaction, and that transplant outcome is improved in the absence of NKG2D signaling in the host. These findings suggest that therapeutic strategies that deplete NKG2D expressing immune effectors, block the interaction of NKG2D and its ligands, or block cell activation after NKG2D ligation hold promise for improving both autologous and allogeneic graft outcome in the CNS.
Supplementary Material
Supp FigureS1
Supplemental Figure 1: Effect of IFN-γ treatment on cytotoxicity of NPC by NKG2D−/− NK cells:NKG2D−/− NK cells were incubated with A) syngeneic NPC (closed squares) or syngeneic NPC treated with IFN-γ (open squares) and B) allogeneic NPC (closed triangles) or IFN-γ-treated allogeneic NPC (open triangles) and cytotoxicity determined. The data shown are means of several replicates (n ≥ 5) ± SEM.
Acknowledgements
We would like to thank Zhiguo Chen for surgical expertise.
This work was supported by grants to OMM from the American Society for Transplantation and to TDP from the California Institute of Regenerative Medicine (CIRM RC1-00131) and the National Institutes of Health/NIMH (R01 MH071472 and RO1 NS076756)
Footnotes
Lori Phillips: Conception and design, Collection and/or assembly of data, Data analysis and interpretation, manuscript writing
Elizabeth Gould: Collection and/or assembly of data
Harish Babu: Collection and/or assembly of data, Data analysis and interpretation, Provision of study material
Sheri Krams: Conception and design, Data analysis and interpretation, Provision of study material
Theo Palmer: Conception and design, Financial support, Administrative support, Data analysis and interpretation, Manuscript writing, Final approval of manuscript
Olivia Martinez: Conception and design, Financial support, Administrative support, Data analysis and interpretation, Manuscript writing, Final approval of manuscript
References
Full text links
Read article at publisher's site: https://doi.org/10.1002/stem.1422
Read article for free, from open access legal sources, via Unpaywall:
https://academic.oup.com/stmcls/article-pdf/31/9/1829/41979785/stmcls_31_9_1829.pdf
Citations & impact
Impact metrics
Citations of article over time
Smart citations by scite.ai
Explore citation contexts and check if this article has been
supported or disputed.
https://scite.ai/reports/10.1002/stem.1422
Article citations
Stem cell-based interventions for the prevention and treatment of intraventricular haemorrhage and encephalopathy of prematurity in preterm infants.
Cochrane Database Syst Rev, 2:CD013201, 15 Feb 2023
Cited by: 2 articles | PMID: 36790019 | PMCID: PMC9932000
Review Free full text in Europe PMC
IL27 Signaling Serves as an Immunologic Checkpoint for Innate Cytotoxic Cells to Promote Hepatocellular Carcinoma.
Cancer Discov, 12(8):1960-1983, 01 Aug 2022
Cited by: 14 articles | PMID: 35723626 | PMCID: PMC9357073
Maintenance of Hypoimmunogenic Features via Regulation of Endogenous Antigen Processing and Presentation Machinery.
Front Bioeng Biotechnol, 10:936584, 22 Jul 2022
Cited by: 1 article | PMID: 36032723 | PMCID: PMC9416868
Mouse CD8+NKT-like cells exert dual cytotoxicity against mouse tumor cells and myeloid-derived suppressor cells.
Cancer Immunol Immunother, 68(8):1303-1315, 05 Jul 2019
Cited by: 16 articles | PMID: 31278476 | PMCID: PMC6682577
Stem cell-based interventions for the prevention and treatment of germinal matrix-intraventricular haemorrhage in preterm infants.
Cochrane Database Syst Rev, 9:CD013201, 24 Sep 2019
Cited by: 7 articles | PMID: 31549743 | PMCID: PMC6757514
Review Free full text in Europe PMC
Go to all (15) article citations
Data
Data behind the article
This data has been text mined from the article, or deposited into data resources.
BioStudies: supplemental material and supporting data
GEO - Gene Expression Omnibus (2)
- (3 citations) GEO - GSE18326
- (3 citations) GEO - GSE18765
Similar Articles
To arrive at the top five similar articles we use a word-weighted algorithm to compare words from the Title and Abstract of each citation.
Activating receptor NKG2D targets RAE-1-expressing allogeneic neural precursor cells in a viral model of multiple sclerosis.
Stem Cells, 32(10):2690-2701, 01 Oct 2014
Cited by: 7 articles | PMID: 24898518 | PMCID: PMC4165828
Role of natural-killer group 2 member D ligands and intercellular adhesion molecule 1 in natural killer cell-mediated lysis of murine embryonic stem cells and embryonic stem cell-derived cardiomyocytes.
Stem Cells, 27(2):307-316, 01 Feb 2009
Cited by: 36 articles | PMID: 18988711
Evasion from NK cell immunity by MHC class I chain-related molecules expressing colon adenocarcinoma.
J Immunol, 171(12):6891-6899, 01 Dec 2003
Cited by: 208 articles | PMID: 14662896
Role of Polymorphisms of NKG2D Receptor and Its Ligands in Acute Myeloid Leukemia and Human Stem Cell Transplantation.
Front Immunol, 12:651751, 30 Mar 2021
Cited by: 5 articles | PMID: 33868289 | PMCID: PMC8044845
Review Free full text in Europe PMC
Funding
Funders who supported this work.
American Society for Transplantation
California Institute of Regenerative Medicine (1)
Grant ID: CIRM RC1-00131
NIMH NIH HHS (1)
Grant ID: R01 MH071472
NINDS NIH HHS (1)
Grant ID: R01 NS076756
National Institutes of Health/NIMH (2)
Grant ID: RO1 NS076756
Grant ID: R01 MH071472