Abstract
Free full text

Tumor suppressor and deubiquitinase BAP1 promotes DNA double-strand break repair
Associated Data
Significance
BAP1 is a deubiquitinase of histone H2A involved in chromatin remodeling. Several studies identified BAP1 as major tumor suppressor inactivated in various cancers. Nonetheless, the manner in which BAP1 protects against cancer development remains enigmatic. We now show that BAP1 is recruited to double-strand DNA break sites and promotes error-free repair of these lesions. We also provide the first evidence that phosphorylation coordinates the function of BAP1 in promoting cellular recovery from DNA damage. Thus, our study represents a significant advance in the field of ubiquitin signaling in the context of cancer development.
Abstract
The cellular response to highly genotoxic DNA double-strand breaks (DSBs) involves the exquisite coordination of multiple signaling and repair factors. Here, we conducted a functional RNAi screen and identified BAP1 as a deubiquitinase required for efficient assembly of the homologous recombination (HR) factors BRCA1 and RAD51 at ionizing radiation (IR) -induced foci. BAP1 is a chromatin-associated protein frequently inactivated in cancers of various tissues. To further investigate the role of BAP1 in DSB repair, we used a gene targeting approach to knockout (KO) this deubiquitinase in chicken DT40 cells. We show that BAP1-deficient cells are (i) sensitive to IR and other agents that induce DSBs, (ii) defective in HR-mediated immunoglobulin gene conversion, and (iii) exhibit an increased frequency of chromosomal breaks after IR treatment. We also show that BAP1 is recruited to chromatin in the proximity of a single site-specific I-SceI–induced DSB. Finally, we identified six IR-induced phosphorylation sites in BAP1 and showed that mutation of these residues inhibits BAP1 recruitment to DSB sites. We also found that both BAP1 catalytic activity and its phosphorylation are critical for promoting DNA repair and cellular recovery from DNA damage. Our data reveal an important role for BAP1 in DSB repair by HR, thereby providing a possible molecular basis for its tumor suppressor function.
After induction of double-strand breaks (DSBs), a convoluted ubiquitin-mediated signaling cascade culminates in the assembly of multiple repair proteins at the site of DNA damage (1). These early ubiquitin signaling events involve, most notably, the recruitment of the RING finger E3 ligases RNF8/RNF168. RNF168 catalyzes K63-linked ubiquitin chain formation on histones H2A/H2AX, which is required for the recruitment of key downstream factors including tumor protein p53 binding protein 1 (53BP1), breast cancer 1 (BRCA1), and RAD51 homologue 1 (RAD51) (2). 53BP1 and BRCA1/RAD51 promote, in a cell cycle-dependent manner, DSB repair by nonhomologous end joining (NHEJ) and homologous recombination (HR), respectively (3). In parallel, another ubiquitin signaling pathway involving the Polycomb group complex PRC1 also contributes to coordinate the DSB response. PRC1 catalyzes the monoubiquitination of H2A on K119 residue (H2Aub), a critical chromatin modification involved in regulating gene expression and DNA damage/repair responses (4). It was proposed that H2Aub promotes silencing of transcription in chromatin regions flanking the DSBs, thus facilitating DNA repair (5, 6).
Several deubiquitinases (DUBs) have also been linked to DSB signaling, and growing evidence suggests that deubiquitination might exert an extensive control on the recruitment and/or disassembly of proteins at the site of DNA damage. For instance, BRCA1/BRCA2-containing complex subunit 36 (BRCC36), a K63 chain-specific DUB, regulates the recruitment of repair proteins by modulating the level of ubiquitin chains (7, 8). POH1/rpn11/PSMD14, a regulatory subunit of the 19S proteasome, deconjugates ubiquitin chains at DSB sites and promotes the recruitment of RAD51 (9). Ubiquitin specific peptidase 3 (USP3) and OTUB1 have also been reported to be important for DSB signaling and repair (10, 11).
The DUB BRCA1-associated protein 1 (BAP1) is a tumor suppressor inactivated in various types of cancer (12). BAP1 forms multiprotein complexes with several chromatin-associated proteins, notably the host cell factor 1 (HCF-1), and regulates transcription (13). The Drosophila BAP1, Calypso, was shown to deubiquitinate H2Aub (14). Thus, BAP1 might be involved in the DNA damage response by coordinating H2A ubiquitination. Notably, proteomic studies revealed BAP1 among phosphorylated proteins during DNA damage (15). Nonetheless, the role of BAP1 in the DNA damage response and more generally, the mechanism of tumor suppression exerted by this DUB remain unclear. In the current study, we identify BAP1 as a regulator of DSB repair, which in turn may elucidate the molecular underpinnings of its poorly understood tumor suppressor function.
Results
DUB RNAi Screen Reveals Several Regulators of HR Proteins Assembly at Ionizing Radiation-Induced Foci.
We sought to identify DUBs required for the recruitment or dispersion of repair proteins at ionizing radiation (IR) -induced foci (IRIF). A human DUB RNAi library was used to screen for DUBs whose depletion affect the number of RAD51 or BRCA1 foci at DSB sites (Fig. 1A). A 24-h time point post-IR was selected for our studies, a time at which 50–60% of cells still exhibit DSB foci, thus facilitating detection of any potential increase or decrease of foci formation (Fig. 1B). Several DUBs were identified in this manner as associated with either increased or more often, decreased RAD51 and/or BRCA1 foci (Fig. 1C and Table S1). As proof of validity, we also identified BRCC36, USP3, and PSMD14, which have been previously reported to regulate DSB signaling by impacting RAD51 and/or BRCA1 foci formation (9, 10, 16). The DUB candidates whose knockdown induced a decrease in BRCA1/RAD51 foci formation include BAP1, DUB3, STAMBP, STAMBPL1, and COPS5 (Fig. 1C). We also identified candidate DUBs whose knockdown results in increased BRCA1/RAD51 foci formation, notably ZRANB1 (Fig. 1C). Immunostainings of BRCA1 and RAD51 foci after depletion of the known DSB regulator PSMD14 and the candidate BAP1 are shown (Fig. 1D).
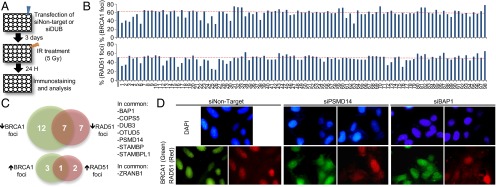
DUB screen identifies several regulators of HR protein assembly at IRIF. (A) Schematic representation of DUB loss-of-function screen for IRIF regulators. U2OS cells were transfected with individual siRNA pool targeting DUBs, exposed to IR, and collected for staining. (B) Graphs represent the percentage of cells with more than 10 foci of BRCA1 or RAD51. Dashed red line shows the percentage of cells with protein foci for the control sample. (C) Venn diagrams showing DUBs associated with reduced or increased percentage of cells with foci. DUBs having the same phenotype with both BRCA1 and RAD51 foci are indicated. (D) Representative staining of BRCA1 and RAD51 foci in PSMD14- and BAP1-depleted cells.
BAP1 Promotes the Recruitment of HR Proteins at IRIF.
We focused on further characterization of BAP1 in the DNA damage signaling/repair processes. We used two additional shRNA constructs targeting BAP1 and found that, in each case, both BRCA1 and RAD51 foci were significantly reduced (Fig. S1A). Next, we monitored the dynamics of IRIF formation for several key proteins in BAP1-depleted cells. Primary human fibroblasts (LF1) were transfected either with control or BAP1 siRNA constructs, irradiated, and analyzed at different time points postdamage (Fig. 2 A and B and Fig. S1B). Relative to control cells, the majority of BAP1-depleted cells exhibited less than 10 BRCA1 and RAD51 foci per cell at all time points, although γH2AX focus formation was similar. It is known that 53BP1 inhibits BRCA1-mediated HR and promotes NHEJ during the G1 phase (17). However, cell cycle analysis did not reveal any substantial accumulation of cells in the G1 phase (Fig. S2A). In addition, we did not observe any significant increase in 53BP1 foci either before or after IR treatment (Fig. 2 A and B). Consistent with these results, staining for foci containing autophosphorylated DNA-PK, a kinase required for NHEJ (18), did not reveal significant differences between BAP1-depleted and control cells (Fig. 2 A and B). Interestingly, constitutive BRCA1 foci, which are distinct from IRIF, were also reduced in BAP1-depleted cells, indicating that this DUB might be involved in coordinating BRCA1 association with chromatin under normal growth conditions. Of note, BRCA1/RAD51 protein expression was not significantly different after BAP1 depletion (Fig. 2C). We note that IR-induced accumulation of the p53 tumor suppressor was essentially similar in control vs. BAP1-depleted cells (Fig. S2B). As expected, BAP1-depleted cells manifested a global increase of H2Aub (Fig. 2C and Fig. S2C).
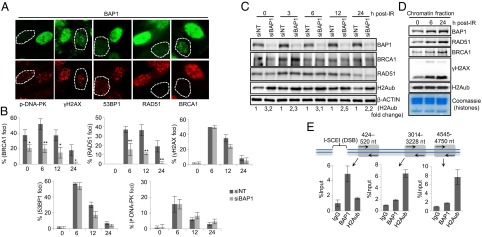
BAP1 promotes IRIF formation and is recruited to the site of DSBs. LF1 cells were transfected with either control (NT) or BAP1 siRNA; 3 d after transfection, control and BAP1 RNAi cells were combined (1:1) and treated with IR (7.5 Gy). Cells were fixed and stained for IRIF proteins in BAP1-depleted cells. Representative staining of cells at 12 h post-IR treatment is shown in A, and the data are presented as mean ± SD in B. Dashed white lines encircle cells with effectively reduced BAP1 expression. Statistical analysis was performed using Student t test. *P < 0.05; **P < 0.01. (C) Protein levels of BRCA1, RAD51, and other proteins were determined by Western blotting. (D) BAP1 is recruited to chromatin after DNA damage. HeLa cells were treated with IR (15 Gy), and chromatin was isolated and analyzed for the indicated proteins. (E) BAP1 is recruited at the proximity of a single DSB in MCF7 cells carrying an I-SceI site. (Upper) Schematic representation of the DSB created by I-SceI and the position of the primers used for the ChIP assay. (Lower) Enrichment of endogenous BAP1 and H2Aub on regions at the proximity to the DSB was determined by ChIP and calculated as percentage of the input. Experiments were repeated two times independently, and real-time PCR was performed three times for each experiment. Data are presented as mean ± SEM.
BAP1 does not distinctly accumulate at IRIF (Fig. S1A) but might be transiently and dynamically recruited to DSBs. To assess the potential recruitment of BAP1 to DNA breaks, we fractionated cellular extracts from untreated vs. IR-treated cells and observed a consistent increase of BAP1 in the chromatin fraction in response to IR (Fig. 2D). As expected, accumulation of RAD51 and BRCA1 on chromatin was readily observed. Of note, no obvious change of global H2Aub was observed in the chromatin fractions, suggesting that DNA damage-induced H2A ubiquitination marginally contributes to the global H2Aub signal. We also probed whether BAP1 is, indeed, recruited to DSB sites by ChIP (Fig. 2E). Real-time PCR quantification of immunoprecipitated chromatin by BAP1 in the vicinity of a unique DSB created by I-SceI in vivo indicated that BAP1 is enriched near the DSB site. Importantly, at the break site, H2Aub levels were inversely correlated with BAP1 recruitment. In contrast, no recruitment of BAP1 was detected distal to the break, where high levels of H2Aub were observed.
BAP1 KO DT40 Cells Are Sensitive to DSB-Inducing Agents and Defective in HR-Mediated Surface IgM Gene Conversion.
To further investigate the function of BAP1 in HR, we generated conditional BAP1 KO chicken B-lymphoma DT40 cells (Fig. 3A). BAP1 is highly conserved between human and chicken (Fig. S3A). Southern blot analysis indicated that both alleles were ablated (Fig. 3B). Targeting of one allele included an expression cassette with the human BAP1 cDNA flanked by two loxP sites, allowing Cre-mediated excision. The DT40 Cre-1 cell line used for the KO generation stably expresses a tamoxifen-inducible Cre recombinase (19). Thus, after tamoxifen treatment, >95% of the cells lose BAP1 expression (Fig. S3B). To obtain cell populations that are completely BAP1-deficient, we isolated two single-cell KO clones with complete absence of BAP1 expression (Fig. 3C). BAP1-deficient DT40 cells show, as expected, a global increase of H2Aub (Fig. 3C). Cell proliferation was also delayed in BAP1 KO cells (Fig. S4).
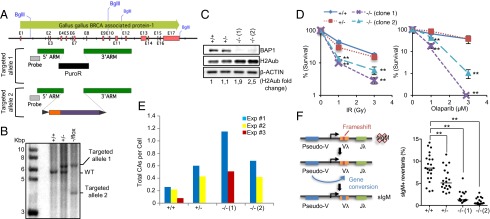
BAP1 KO DT40 cells are sensitive to DNA damaging agents and defective in HR-mediated gene conversion at sIgM locus. (A) Schematic for the strategy used to generate BAP1 KO in DT40 cells. (B) Southern blot confirming BAP1-targeted alleles. (C) DT40 BAP1 KO clones 1 and 2 isolated after Cre-mediated excision of BAP1. (D) Clonogenic survival of BAP1 KO DT40 cells treated with IR or Olaparib. Statistical analysis was performed using Student t test. **P < 0.01. (E) BAP1 KO DT40 cells have increased chromosome breaks after DNA damage. Cells were treated with IR (2 Gy) and fixed after 3.5 h. Three independent experiments were done, and chromosome aberrations (CAs; isochromatid/chromatid gaps and breaks and radial figures) were scored in 100 cells for each experiment. Results are reported as total aberrations per cell. (F, Left) Schema representing the mechanism of sIgM reversion in DT40 cells by gene conversion. (F, Right) sIgM− cells, isolated by flow cytometry, were expanded for 90 generations, and the proportion of sIgM+ revertant cells of each subpopulation was determined. The experiment was done two times independently, and the graph compiles the results of both experiments, with medians indicated by horizontal lines. Statistical analysis was performed using Student t test. **P < 0.01.
To assess the role of BAP1 in DSB repair, we conducted survival assays with BAP1 KO DT40 cells treated with DNA damaging agents that induce DSBs. Because BAP1 KO cells proliferate slower than WT cells (Fig. S4), cell numbers were adjusted before treatment to compensate for any potential bias that could be introduced as a consequence of unequal cell proliferation. We observed that the BAP1−/− cells are more sensitive to IR than WT cells (Fig. 3D). BAP1 KO sensitivity to IR is accompanied by an elevated level of chromosome aberrations (Fig. 3E and Fig. S5). HR-deficient cells, such as cancer cells harboring inactivating mutations in BRCA1 or -2, are hypersensitive to poly (ADP ribose) polymerase (PARP) inhibition (20). We analyzed the response of BAP1−/− cells to the PARP inhibitor, Olaparib. Indeed, BAP1−/− cells are strikingly sensitive to PARP inhibition relative to BAP1+/+ and BAP1+/− cells (Fig. 3D). The high sensitivity of BAP1 KO cells to IR and Olaparib is consistent with the recently reported sensitivity of renal carcinoma-derived BAP1-deficient cells to DSB-inducing agents (21).
To confirm a role for BAP1 in HR, we took advantage of the fact that DT40 cells constitutively diversify their immunoglobulin (Ig) loci by gene conversion (22). The DT40 Cre-1 cell line harbors a frameshift in the rearranged V segment of the Ig light-chain gene, which results in a surface IgM− (sIgM−) phenotype. This frameshift can be repaired by HR-based gene conversion in a fraction of the cells, leading to the reexpression of sIgM (Fig. 3F). Thus, the proportion of sIgM+ revertants in the population can be used to quantify gene conversion efficiency. sIgM− cells were sorted and expanded to allow gene reversion for the same number of population doublings. Although ~8% of BAP1+/+ cells reverted, both BAP1−/− clones were relatively defective (1% and 0.5%), whereas BAP1+/− cells showed an intermediate phenotype (5%) (Fig. 3F).
Phosphorylation of BAP1 After IR Treatment Promotes DNA Repair and Cellular Recovery from DNA Damage.
In global proteomics studies, BAP1 was reported to be phosphorylated on S592 [an Ataxia telangiectasia mutated (ATM) and ataxia telangiectasia and Rad3-related (ATR) SQ/TQ motif] after IR treatment (15). We conducted a large-scale immunopurification of BAP1 post-IR from HeLa cells followed by MS analysis. We identified another SQ phosphosite (S276) and several IR-induced phosphorylation sites, two of which are conserved between human and chicken (Fig. 4A and Fig. S6 A–C).
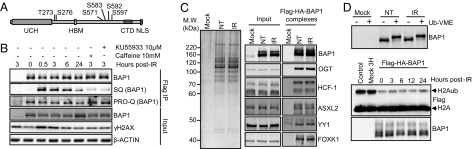
BAP1 is phosphorylated after DNA damage on multiple sites. (A) Schematic representation of BAP1 showing its main domains and motifs: ubiquitin C-terminal hydrolase (UCH), HCF-1 binding motif (HBM), C-terminal domain (CTD), and nuclear localization signal (NLS) along with the identified phosphorylation sites. (B) ATM is required for phosphorylation of BAP1. HeLa cells expressing Flag-HA-BAP1 were incubated with ATM inhibitors and then treated with IR (7.5 Gy). Immunoprecipitated BAP1 was subjected to immunoblotting or PRO-Q stain. IP, immunoprecipitation. (C) BAP1 complexes were purified at 0h (NT, non-treated) and 3 h post-IR and subjected to (Left) silver staining and (Right) Western blot analysis. MW, molecular mass. (D, Upper) Purified BAP1 complexes were incubated with or without the Ubiquitin-Vinyl Methyl Ester (Ub-VME) probe for 2 h and analyzed by Western blot. (D, Lower) In vitro deubiquitination assay of nucleosomal H2Aub using purified BAP1 complexes. Flag-HA-BAP1 complexes were isolated at different times post-IR and incubated with nucleosomes for 4 h.
Using an anti-pSQ/TQ (G) antibody that is expected to recognize pS592, we found that mutation of S592, indeed, abolished the phosphorylation of BAP1 on this site (Fig. S7A). Next, using this antibody, we found that inhibition of ATM with caffeine or KU-55933 resulted in decreased phosphorylation of BAP1 S592 (Fig. 4B). However, ATR inhibition only resulted in a slight decrease of the S592 phosphorylation signal, whereas ATR-mediated CHK1 phosphorylation was abrogated (Fig. S7B). Using DNA-PK–deficient cells, we found that this kinase is not responsible for phosphorylation of BAP1 S592 after IR (Fig. S7C). Of note, HCF-1 is not required for BAP1 phosphorylation by ATM, because the S592 phosphorylation signal on BAP1 lacking the HCF-1 binding motif (HBM) is not decreased after IR treatment (Fig. S7D). Cyclin-dependent kinases (CDKs) are involved in DSB repair (23, 24) and might, in concert with ATM, phosphorylate BAP1 to coordinate its function. Using chemical inhibitors in conjunction with the anti-pSQ/TQ (G) antibody, we did not observe a requirement of CDKs for BAP1 phosphorylation by ATM (Fig. S7E). Using the PRO-Q phosphostain, we found that the global phosphorylation state of BAP1 did not significantly change after IR treatment or CDK inhibition (Fig. S7E), likely because of the high level of constitutive phosphorylation of BAP1.
Next, we found that the stable components of the BAP1 complexes are unaffected by IR treatment (Fig. 4C and Fig. S6D). We then used an activity-directed ubiquitin probe that binds to the catalytic site of cysteine protease DUBs and found that the probe labeled purified BAP1 from untreated and IR-treated cells with similar efficiency (Fig. 4D, Upper). Similar results were observed for endogenous BAP1 in HeLa or U2OS cells (Fig. S6E). Next, we evaluated BAP1 DUB activity to H2A using purified nucleosomes incubated with BAP1 complexes isolated from untreated vs. IR-treated cells (Fig. 4D, Lower), and no significant difference was observed. Therefore, we sought to determine the importance of the IR-specific phosphosites of BAP1 for its DNA damage function in vivo. We generated a set of BAP1 phosphomutants, including the individual mutants: T273A, S276A, and S592A, and the combined mutants: SQ sites S276A/S592A (termed SQ-MUT) and IR-phosphorylated residues (all the six phosphosites converted to alanine, termed P-MUT). These mutants were used, along with the BAP1 ΔHBM and the BAP1 catalytically dead C91S, to stably reconstitute the BAP1-deficient lung carcinoma cell line H226 (Fig. 5A). As previously shown (25), expression of BAP1 WT, but not the catalytic dead mutant, induced a delay in H226 cell proliferation (Fig. 5B). BAP1 that was deficient in interaction with HCF-1 did not affect cell proliferation. Interestingly, the BAP1 P-MUT also failed to reduce cell proliferation. To further determine the sensitivity of these cells to IR, a clonogenic survival assay was performed. To exclude any potential bias that can be introduced by the unequal cell proliferation of the BAP1 stable cell lines, survival rates were normalized to untreated cells. Thus, although H226 cells expressing BAP1 WT proliferate relatively slowly, they were more resistant to IR compared with H226 cells expressing the empty vector. BAP1 C91S, BAP1 ΔHBM, and BAP1 P-MUT were the most sensitive to IR (Fig. 5C). Based on the above information, we concluded that phosphorylation of BAP1 on multiple sites and catalytic activity are required for promoting cell survival after IR. Of note, no overt apoptosis is induced after IR treatment of H226 expressing the WT or mutant forms of BAP1 (Fig. S8B). Next, we conducted ChIP analysis and found that, although the recruitment of BAP1 SQ-MUT to the site of DSB was partially decreased, the recruitment of BAP1 P-MUT was totally abolished (Fig. 5D). Of note, similar to the WT BAP1, the P-MUT assembled protein complexes (Fig. S7 F and G) and efficiently deubiquitinated nucleosomal H2A in vitro (Fig. S7H). To directly analyze DSB repair, we determined the levels of γH2AX in H226 stably expressing BAP1 WT or mutants after IR treatment (Fig. 5E). We found that the BAP1 WT, but not the C91S or the P-MUT, reduced both the constitutive and IR-induced accumulation of γH2AX. BAP1 WT, but not the C91S mutant, promoted a strong deubiquitination of H2A. Interestingly, although expression of BAP1 P-MUT also significantly promoted deubiquitination of H2A, the remaining levels of H2Aub were consistently two times higher in cells expressing the BAP1 P-MUT than cells expressing the WT form (Fig. 5E).
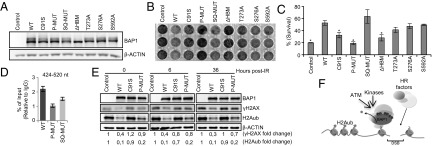
Phosphorylation of BAP1 after IR promotes cellular recovery from DNA damage. (A) Generation of H226 cells stably expressing BAP1 WT and mutants. (B) Effects of BAP1 WT and mutant forms on H226 cells proliferation. The same number of cells was seeded and allowed for colony formation visualized by crystal violet staining. Experiment was done at least three times. (C) H226 cell lines were treated with IR (20 Gy), and surviving colonies were quantified by crystal violet staining and normalized to the untreated controls. Experiment was done three times, and data are presented as mean ± SD. Statistical analysis was performed using Student t test. *P < 0.01. (D) ChIP analysis of the recruitment of phosphorylation-deficient mutants of BAP1 at the proximity of DSB in MCF7 cells carrying an I-SceI site. Experiment was repeated two times independently, and real-time PCR was performed three times for each experiment. Data are presented as mean ± SEM. (E) H226 BAP1-deficient cells that express BAP1 WT or mutant forms were treated with IR (15 Gy) and harvested for Western blotting. (F) Model for the role of BAP1 in the DSB response. BAP1 is phosphorylated after DNA damage, thus promoting its recruitment to the DSB site for H2A deubiquitination and allowing the recruitment of downstream DSB signaling and repair proteins.
Discussion
We report the identification of DUB candidates that might play important roles in the cellular response to DSBs. Notably, STAMBP and COPS5 seem to be interesting candidates. These DUBs are zinc-dependent metalloproteases of the JAMM/MPN+ family, which has intrinsic specificity to K63-linked ubiquitin chains (26). Because K63 chains are highly involved in the DSB response, it is possible that these DUBs regulate DSB repair. Notably, we also identify BAP1 as a critical regulator of HR. Consistently, BAP1 KO phenocopies BRCA1 KO and RAD51 KO in DT40 cells, both being hypersensitive to DSB-inducing agents accompanied with high levels of chromosome breaks (27, 28). We emphasize that BAP1 heterozygous clones also exhibit chromosomal defects and decreased HR-mediated sIgM reversion. These observations suggest that BAP1 dosage is critical, which may reflect the fact that all nuclear BAP1 is contained within multiprotein complexes (13). Indeed, BAP1 heterozygous mutations are found in human tumors (29).
Several mechanisms, not necessarily mutually exclusive, might explain how BAP1 regulates HR proteins. BAP1 depletion decreases the assembly of constitutive BRCA1 foci, which are associated with replication of heterochromatin (30). Thus, it is plausible that BAP1 depletion affects the expression of genes involved in BRCA1 recruitment on chromatin. It is also possible that the effects of BAP1 on the recruitment of HR proteins might be directly linked to its previously reported interaction with BRCA1/BARD1 (31, 32). Although our studies failed to reveal BRCA1/BARD1 as stable components of the BAP1 complexes (13) and BAP1 exerts BRCA1-independent effects on cell proliferation (25), it is possible that BRCA1 interaction with BAP1 is transient and associated with DNA damage-dependent and -independent events. Thus, the implication of BAP1 in HR revealed herein provides impetus for future studies to determine the exact significance of the interaction between BAP1 and BRCA1/BARD1.
However, to facilitate DNA repair, transcription seems to be blocked at DSB by PRC1-mediated H2A ubiquitination (5). Accordingly, DUBs that remove H2Aub at DSBs are expected to inhibit HR. However, we observed the opposite effect (i.e., the H2A DUB BAP1 promotes HR). In fact, the BAP1 complex might act in concert with the PRC1 complex to promote dynamic ubiquitination/deubiquitination of H2A, thereby ensuring the proper dosage of this modification at the site of DSB. Based on our ChIP analysis for BAP1 and H2Aub near the DSB site, it is possible that BAP1 deubiquitinates H2A in the proximity of the DSB site to increase chromatin accessibility at this specific region to allow, for example, DNA resection during HR. Thus, BAP1 depletion causing an increase of H2Aub might interfere with specific chromatin and/or histone modifications events at DSBs, which might explain the observed defect in HR. Moreover, it is possible that more than one H2A DUB is involved in the signaling at DSBs. Some DUBs might assist in chromatin organization to promote DNA repair, whereas others could play a role in foci resolution. Consistent with this model, it was reported that another H2A DUB, USP16, regulates the level of this histone modification to control transcription at DSB sites (6).
In support of BAP1 function in the cellular response to DSBs, we showed that its phosphorylation is required for promoting survival after IR. Because BAP1 phosphorylation does not directly impact intrinsic BAP1 DUB activity, it is possible that it rather promotes BAP1 interaction with other factors to facilitate the recruitment to DSBs, where it regulates H2Aub levels. Indeed, the residual levels of H2Aub are consistently higher in H226 cells expressing the BAP1 P-MUT than the WT form, probably reflecting an inability of the mutant to deubiquitinate the small pool of H2Aub associated with DSBs. We also note that several sites of BAP1 are phosphorylated after IR treatment, including SQ/TQ and non SQ/TQ sites, indicating that BAP1 is phosphorylated by multiple DNA damage-responsive kinases. Thus, BAP1 involvement in the DNA damage response might be more complex than anticipated. Indeed, the use of BAP1 P-MUT to reconstitute BAP1-deficient H226 cells indicated that the decrease of cell proliferation after reintroduction of BAP1 WT depends on its phosphorylation, even in the absence of exogenously inflicted DNA damage. The effect of BAP1 phosphorylation on cell proliferation likely reflects a role of this DUB in DNA damage-induced checkpoint responses. In fact, normally growing H226 cells have elevated levels of γH2AX and a severe genomic instability (Fig. S8 C and D), indicative of high rates of spontaneous DNA damage in these BAP1-deficient cells. These cancer cells must necessarily harbor defects in DNA damage checkpoints that allow them to proliferate under such genomic instability. Therefore, the expression of BAP1 WT in H226 cells might reactivate certain DNA damage checkpoints, thus causing decreased cell proliferation.
In summary, we provide strong evidence indicating that BAP1 is a DNA damage signaling and repair enzyme (Fig. 5F). Loss of BAP1 is expected to decrease HR, an error-free repair mechanism. Under such conditions, cells might become much more reliant on NHEJ, an error-prone repair mechanism, resulting in the net accumulation of mutations and chromosomal aberrations that cause genomic instability. Moreover, as a consequence of BAP1 inactivation, defects in checkpoint(s) signaling could promote the survival of cells harboring damaged DNA, thus driving neoplastic transformation.
Materials and Methods
RNAi, Gene Targeting, and Phenotypic Analysis.
Cells were transfected with siRNA or shRNA targeting DUBs or nontarget control and harvested as indicated. The DT40 Cre-1 cell line was used to generate the BAP1 KO. Clonogenic survival assay, cytogenetic analysis, sIgM gene conversion assay, preparation of cell extracts, and chromatin fraction for Western blotting were done as described in SI Materials and Methods.
Immunofluorescence and Flow Cytometry Analysis.
Cells were immunostained as previously described (33). Flow cytometry determination of DNA content, BAP1, phosphohistone H3 serine 10, and BrdU incorporation were conducted using the LSRII Flow Cytometer, and data were processed with FlowJo V887 software.
ChIP on an I-SceI–Induced DSB.
Induction of a single DSB after I-SceI expression, ChIP experiments, and real-time PCR were done as described in SI Materials and Methods.
Purification of BAP1 Complexes and Identification of Phosphorylation Sites.
HeLa S3 cells expressing stable Flag-HA-BAP1 or the empty vector were treated with IR and used for immunopurification and MS. Additional details are provided in SI Materials and Methods.
Deubiquitination Assays.
Ubiquitin-Vinyl Methyl Ester probe labeling and in vitro H2Aub deubiquitination assay were done as described in SI Materials and Methods.
Acknowledgments
We thank Huib Ovaa for providing us with the DUB probe and Sylvie Lavallée for cytogenetic analysis. This work was supported by grants from the Cancer Research Society (to E.B.A.), the Natural Sciences and Engineering Research Council of Canada (to E.D.), and the Canadian Institutes of Health Research (CIHR; to J.Y.M.). This work was also supported by Canadian Cancer Society Research Institute Grant 700348 (to J.M.D.N.). H.Y. was supported by a PhD Scholarship from the Cole Foundation and the CIHR. J.Y.M. is a Le Fonds de la Recherche en Santé du Québec National Investigator. J.M.D.N. is supported by a Canada Research Chair Tier 2. E.B.A. is a scholar of the CIHR and Le Fonds de la Recherche en Santé du Québec.
Footnotes
The authors declare no conflict of interest.
This article is a PNAS Direct Submission.
This article contains supporting information online at www.pnas.org/lookup/suppl/10.1073/pnas.1309085110/-/DCSupplemental.
References
Articles from Proceedings of the National Academy of Sciences of the United States of America are provided here courtesy of National Academy of Sciences
Full text links
Read article at publisher's site: https://doi.org/10.1073/pnas.1309085110
Read article for free, from open access legal sources, via Unpaywall:
https://www.pnas.org/content/pnas/111/1/285.full.pdf
Citations & impact
Impact metrics
Citations of article over time
Alternative metrics
Smart citations by scite.ai
Explore citation contexts and check if this article has been
supported or disputed.
https://scite.ai/reports/10.1073/pnas.1309085110
Article citations
BAP1 regulates HSF1 activity and cancer immunity in pancreatic cancer.
J Exp Clin Cancer Res, 43(1):275, 30 Sep 2024
Cited by: 0 articles | PMID: 39350280 | PMCID: PMC11441124
Case report: ATM A1159T mutation in malignant peritoneal mesothelioma may be associated with tumor recurrence.
SAGE Open Med Case Rep, 12:2050313X241271818, 16 Aug 2024
Cited by: 0 articles | PMID: 39161923 | PMCID: PMC11331467
Targeting DNA Damage Response Deficiency in Thoracic Cancers.
Drugs, 84(9):1025-1033, 13 Jul 2024
Cited by: 0 articles | PMID: 39001941
Review
Potential roles of UCH family deubiquitinases in tumorigenesis and chemical inhibitors developed against them.
Am J Cancer Res, 14(6):2666-2694, 15 Jun 2024
Cited by: 0 articles | PMID: 39005671
Review
The deubiquitinating enzyme USP4 regulates BRCA1 stability and function.
NPJ Breast Cancer, 10(1):35, 11 May 2024
Cited by: 0 articles | PMID: 38734703 | PMCID: PMC11088691
Go to all (212) article citations
Data
Data behind the article
This data has been text mined from the article, or deposited into data resources.
BioStudies: supplemental material and supporting data
Similar Articles
To arrive at the top five similar articles we use a word-weighted algorithm to compare words from the Title and Abstract of each citation.
Polo-like kinase 1 mediates BRCA1 phosphorylation and recruitment at DNA double-strand breaks.
Oncotarget, 7(3):2269-2283, 01 Jan 2016
Cited by: 22 articles | PMID: 26745677 | PMCID: PMC4823034
BRCA1 and CtIP Are Both Required to Recruit Dna2 at Double-Strand Breaks in Homologous Recombination.
PLoS One, 10(4):e0124495, 24 Apr 2015
Cited by: 23 articles | PMID: 25909997 | PMCID: PMC4409214
Remodeling and spacing factor 1 (RSF1) deposits centromere proteins at DNA double-strand breaks to promote non-homologous end-joining.
Cell Cycle, 12(18):3070-3082, 20 Aug 2013
Cited by: 44 articles | PMID: 23974106 | PMCID: PMC3875681
Functional Link between BRCA1 and BAP1 through Histone H2A, Heterochromatin and DNA Damage Response.
Curr Cancer Drug Targets, 16(2):101-109, 01 Jan 2016
Cited by: 15 articles | PMID: 26517537
Review
Funding
Funders who supported this work.