Abstract
Free full text

In this issue of the Biophysical Journal, Jarecki et al. (1) investigate the possibility of combining two strategies commonly used to measure distances between loci in a number of systems.
The first strategy, Förster resonance energy transfer (FRET), is a spectroscopic method based on energy transfer between two fluorescent molecules: upon transferring its excitation energy to an acceptor via a long-range dipole-dipole coupling mechanism, the donor molecule returns to a ground state (2,3). Because this energy transfer can be measured and is inversely proportional to the sixth power of the distance between both fluorophores, the FRET technique lends itself to determining small inter- or intramolecular distances in vitro or in living cells (3). As a result, FRET has been a key technology in revealing the molecular dynamics underlying various biological phenomena (2). However, the rather large size of commonly used genetically encoded fluorophore probes, their relative orientation, and the need for flexible linkers that occupy a substantial conformational space, complicate accurate distance measurements below ~20 Å (2,3). Nonetheless, experiments to improve FRET distance estimates by incorporating transition metal-ion binding sites within proteins or employing small cysteine-reactive organic fluorophores such as bimane, have shown great promise (4).
The second strategy to examine protein dynamics on a nanometer scale involves the use of chemical spacers capped by functional groups. One application from the ion channel field is to measure avidin accessibility to tethered biotin reagents linked to cysteine residues engineered into the channel. Due to the large size of avidin, the availability of a partnering biotinylated cysteine within the channel will depend on the length of the biotin spacer and the position of that cysteine residue (5). Alternatively, varying the linker length of channel pore blockers tethered to a region within the voltage sensor can be used to determine distances between the binding site of the blocking agent within the pore and the voltage-sensing domain in a particular conformation (6). However, limitations of this approach include the necessity to synthesize various molecules as well as challenges in obtaining distance information for more than one functional state.
By combining fluorophores with chemical spacers or rulers, Jarecki et al. (1) provide a conceptual framework to overcome the limitations of both techniques and succeed in accurately estimating molecular distances starting from ~4 Å (1). In particular, the authors synthesized a library of bifunctional probes consisting of a quencher molecule attached to a thiol-reactive maleimide anchor via variable-length spacers. Next, they demonstrated that subnanometer distance information can be obtained between the anchor of this probe and a chosen fluorescently labeled location within the target protein through Dexter energy transfer, a FRET-related collisional quenching mechanism.
An appropriate analogy may be that of a dog (quencher) on a leash (spacer) attached to a ground stake (anchor). When the leash is too short, the dog will be unable to reach a nearby treat (fluorophore) whereas a long leash provides the dog with freedom to explore objects that are far more interesting when compared to the treat (see Fig. 1). However, if the leash has just the right length, the dog will grab the treat and eat it (quenching occurs). Before moving into a complex system, this innovative approach was calibrated using stiff functionalized polyproline chains of varying lengths with the bifunctional probe attached to one end and a fluorophore to the other.
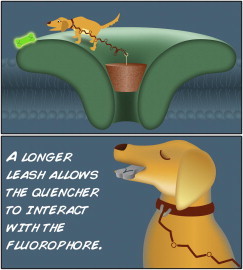
Bifunctional spectroscopic probes as rulers to determine molecular distances. Shown is a cartoon depicting an analogy of the approach designed by Jarecki et al. (1). When the leash (spacer or ruler) attached to a ground stake (anchor) within a protein (green stylized ion channel) is too short (upper panel), the dog (quencher) will be unable to reach a nearby treat (fluorophore) whereas a leash of the right length will allow the dog to grab the treat and eat it (quenching occurs; lower panel). To see this figure in color, go online.
Supported by molecular-dynamics simulations, these experiments revealed a striking relationship between quenching efficiency and varying spacer lengths up to ~30 Å.
The authors then validated the utility of tethered quenchers with the help of the Shaker voltage-gated potassium channel, one of the best-studied ion channel proteins (7). To conduct these experiments, a new set of tethered quenchers was constructed to include a tetraethylammonium molecule that anchors the whole construct to a precisely defined region within the pore of the Shaker channel. Excitingly, ensuing distance measurements between the center nitrogen of tetraethylammonium and fluorescently labeled residues within the voltage-sensing domains in both activated and resting states were in good agreement with those deduced from a resting-state structural model of a related voltage-gated potassium channel (8), thereby showcasing the robustness of the method developed by Jarecki et al. (1). Nonetheless, the need to synthesize tethered quenchers with stiff, nonsticky linkers may still form an entry barrier, although Click chemistry may simplify their fabrication.
Overall, the authors present a strong case for the suitability of their approach to study protein dynamics, and there is reason to think that the approach can be improved or optimized further. For example, the use of fluorophores smaller than carboxytetramethylrhodamine may enable reliable distance measurements below 4 Å. Similarly, exploring a variety of quencher molecules may yield better results in particular systems (e.g., dibromo moiety to replace the NO radical used by Jarecki et al. (1)). Also, incorporation of (fluorescent) unnatural amino acids into the backbone (9) will reduce the stress of labeling proteins and improve the accuracy of distance measurements by alleviating limitations imposed by fluorophore attachments.
Finally, toxins isolated from animal venom may be used as powerful target- and site-specific tethered-quencher anchors for studying ion-channel dynamics because of their exquisite ability to potently interact with well-defined regions within individual channel or receptor isoforms (10). In addition, toxin binding can be functionally monitored to ensure proper anchoring of the bifunctional probe (11). Altogether, the work by Jarecki et al. (1) adds a new and exciting tool to the realm of protein dynamics explorations which, in turn, may help expose regulatory mechanisms underlying protein function in normal and pathological conditions.
Acknowledgments
The author acknowledges scientific visual communicator Kate Baldwin for providing the illustration in Fig. 1.
The author acknowledges funding by the Human Frontiers Science Program and the National Institute of Neurological Disorders and Stroke of the National Institutes of Health, Bethesda, MD, under award No. R00NS073797.
The content is solely the responsibility of the author and does not necessarily represent the official views of the National Institutes of Health.
References
Articles from Biophysical Journal are provided here courtesy of The Biophysical Society
Full text links
Read article at publisher's site: https://doi.org/10.1016/j.bpj.2013.11.011
Read article for free, from open access legal sources, via Unpaywall:
http://www.cell.com/article/S0006349513012393/pdf
Similar Articles
To arrive at the top five similar articles we use a word-weighted algorithm to compare words from the Title and Abstract of each citation.
Why the Drosophila Shaker K+ channel is not a good model for ligand binding to voltage-gated Kv1 channels.
Biochemistry, 52(9):1631-1640, 20 Feb 2013
Cited by: 12 articles | PMID: 23398369
Atomistic Modeling of Ion Conduction through the Voltage-Sensing Domain of the Shaker K+ Ion Channel.
J Phys Chem B, 121(15):3804-3812, 25 Jan 2017
Cited by: 7 articles | PMID: 28074656 | PMCID: PMC5605276
Tethered spectroscopic probes estimate dynamic distances with subnanometer resolution in voltage-dependent potassium channels.
Biophys J, 105(12):2724-2732, 01 Dec 2013
Cited by: 6 articles | PMID: 24359744 | PMCID: PMC3882458
Involvement of the S4-S5 linker and the C-linker domain regions to voltage-gating in plant Shaker channels: comparison with animal HCN and Kv channels.
Plant Signal Behav, 9(10):e972892, 01 Jan 2014
Cited by: 4 articles | PMID: 25482770 | PMCID: PMC4622754
Review Free full text in Europe PMC
Funding
Funders who supported this work.
Human Frontier Science Program (1)
Grant ID: R00NS073797
NINDS NIH HHS (2)
Grant ID: R00NS073797
Grant ID: R00 NS073797
National Institute of Neurological Disorders and Stroke (1)
Grant ID: R00NS073797
National Institutes of Health (1)
Grant ID: R00NS073797