Abstract
Free full text

Glucocorticoid actions on synapses, circuits, and behavior: Implications for the energetics of stress
Abstract
Environmental stimuli that signal real or potential threats to homeostasis lead to glucocorticoid secretion by the hypothalamic-pituitary-adrenocortical (HPA) axis. Glucocorticoids promote energy redistribution and are critical for survival and adaptation. This adaptation requires the integration of multiple systems and engages key limbic-neuroendocrine circuits. Consequently, glucocorticoids have profound effects on synaptic physiology, circuit regulation of stress responsiveness, and, ultimately, behavior. While glucocorticoids initiate adaptive processes that generate energy for coping, prolonged or inappropriate glucocorticoid secretion becomes deleterious. Inappropriate processing of stressful information may lead to energetic drive that does not match environmental demand, resulting in risk factors for pathology. Thus, dysregulation of the HPA axis may promote stress-related illnesses (e.g. depression, PTSD). This review summarizes the latest developments in central glucocorticoid actions on synaptic, neuroendocrine, and behavioral regulation. Additionally, these findings will be discussed in terms of the energetic integration of stress and the importance of context-specific regulation of glucocorticoids.
1. Introduction
The scientific understanding of ‘stress’ and its ramifications for the organism have continually evolved. Based on Claude Bernard's theory of the internal milieu, Walter Cannon first used the concept of homeostasis to explain the ‘fight-or-flight’ response of an organism presented with a threat (Cannon, 1932). In a biological sense, Hans Selye coined the term ‘stress’ as the non-specific response of the body to any homeostatic demand (Selye, 1936). While it is still generally accepted that the physiological role of the stress response is to coordinate autonomic, neuroendocrine, and immune responses to potential homeostatic threats, an emerging concept in stress neurobiology suggests that the primary role of stress responding is to mobilize energy to promote context-specific survival and not necessarily sustain homeostatic systems at levels maintained prior to a challenge (Dallman et al., 2006; Nederhof and Schmidt, 2013). Given this framework, responses to both acute and chronic stress are considered adaptive, up to a point, and prepare the organism for current and future demands. Thus, for the purpose of the current review, stress will be defined as a stimulus that mobilizes energetic systems to respond to an ongoing or anticipated challenge.
Responding to stress involves the concerted activity of multiple, interacting central stress-regulatory systems to mobilize energy for the organism. Activation of the stress response occurs either as a consequence of, or in anticipation of, a challenge (Myers et al., 2012b). Anticipatory responses require the organism to reference prior experiences to predict the need for energy mobilization, primarily mediated by multi-synaptic forebrain projections to the medial parvocellular paraventricular nucleus (PVN) of the hypothalamus. Systemic challenges are largely reflexive responses to physiological disruption generated by direct projections from the hindbrain to the PVN, though there is considerable overlap and integration at various nodes throughout the brain (Herman et al., 2012, 2003; Ulrich-Lai and Herman, 2009). Thus, the neuroendocrine response to stress is a highly-regulated, temporal process, involving the integration of sensory information from multiple modalities to rapidly activate, as well as inhibit the secretion of glucocorticoids.
The neuroendocrine stress cascade, comprising the hypothalamic-pituitary-adrenocortical (HPA) axis, begins with the release of adrencorticotropic hormone (ACTH) secretagogues from neurosecretory neurons in the medial parvocellular PVN, which project to hypophysial portal vessels in the external zone of the median eminance (Bruhn et al., 1984). Secretagogues travel via the portal veins to the anterior pituitary, where they can access corticotropes (De Wied et al., 1957; Gibbs and Vale, 1982; McCann and Fruit, 1957; Saffran and Schally, 1956). The pioneering work of Wylie Vale and colleagues provided initial identification of corticotropin releasing factor (CRF) as the primary driver of pituitary ACTH release (Bale and Chen, 2012; C. Rivier et al., 1983; Rivier et al., 1982; J. Rivier et al., 1983; Spiess et al., 1981; Swanson et al., 1983; Vale et al., 1981). Subsequent studies, also by Vale and colleagues, revealed the existence of several co-secretagogues that synergize with CRF, including arginine vasopressin (AVP) (Rivier and Vale, 1983a, 1983b; Sawchenko et al., 1984; Vale et al., 1983, 1981). By way of the systemic circulation, ACTH acts at the level of the adrenal cortex to induce the release of glucocorticoids, cortisol in some species (e.g., humans, non-human primates) and corticosterone in others (e.g., rats, mice) (Dallman and Jones, 1973; Dallman et al., 1987). At the adrenal, cortisol/corticosterone is released in pulses, the timing of which dictates the overall magnitude of both baseline activity and stress responses (Lightman et al., 2008; Young et al., 2004). Pulsatile patterns of glucocorticoid release are dictated by ultradian rhythms (for review see de Kloet and Sarabdjitsingh, 2008; Sarabdjitsingh et al., 2012a). This rhythmicity of glucocorticoid release is essential for maintaining cellular responsiveness and promotes wide-ranging glucocorticoid actions from gene transcription to behavior (Conway-Campbell et al., 2010; Sarabdjitsingh et al., 2010b). Glucocorticoids then travel throughout the body, exerting a multitude of effects in the periphery including glycogen breakdown and gluconeogenesis (Coderre et al., 1991; Exton, 1979; Munck et al., 1984; for detailed reviews of brain circuits regulating glucose homeostasis see Schwartz et al., 2000; Seeley and Woods, 2003; Woods et al., 1998). Glucocorticoids cross the blood-brain-barrier, and primarily bind to mineralocorticoid (MR) and glucocorticoid receptors (GR) in neurons and/or glia. In the brain, MR has high-binding affinity for corticosteroids and, consequently, is activated at basal levels (de Kloet and Sarabdjitsingh, 2008; de Kloet et al., 1998). Thus, MR is thought to sense resting levels of glucocorticoids and promote key functions associated with low glucocorticoid levels, including circadian drive of the HPA axis and mnemonic function (de Kloet et al., 2005). Conversely, GR has a lower binding affinity for glucocorticoids and is largely unoccupied at basal levels. Thus, GR is thought to be particularly important in signaling mediated by stress levels of glucocorticoids (Boyle et al., 2005; de Kloet and Reul, 1987; de Kloet et al., 2005; Reul and de Kloet, 1985). GR is abundantly expressed throughout the brain, including the primary stress-regulatory sites discussed in this review: medial prefrontal cortex (mPFC), hippocampus, amygdala, bed nucleus of the stria terminalis (BST), hypothalamus, and hindbrain (Fuxe et al., 1987; Meaney et al., 1985; Reul and de Kloet, 1986). MR has a more restricted distribution that overlaps with that of GR in several key regions, including the mPFC, hippocampus, and amygdala (de Kloet and Reul, 1987; Reul and de Kloet, 1985).
There is a vital need for glucocorticoid activity to be tightly regulated, requiring systems coordination from cellular to behavioral levels. In response to stress, glucocorticoid signaling promotes organismal adaptation to environmental conditions and helps to meet the resulting energetic demands. This adaptation requires the integration of multiple systems and engages key limbic-neuroendocrine circuits. Forebrain, hypothalamic, and hindbrain circuits are activated by glucocorticoids and participate in the coordination of physiological and behavioral output. However, when energetic drive does not appropriately match environmental demand, or the organism is chronically activating these systems, risk factors emerge for a variety of stress-related pathologies. The present article will review the actions of glucocorticoids in central stress-regulatory circuits, focusing on the rodent literature, in the context of the adaptive role of the stress response for the organism. The review will summarize the role of central glucocorticoid actions on synaptic, neuroendocrine, and behavioral regulation, highlighted by a discussion of the energetic integration of stress and the importance of context-specific regulation of glucocorticoids. The energy mobilizing effects of glucocorticoids require integration of cellular activity, circuit connectivity, and behavioral output to coordinate context-appropriate adaptation. We propose that glucocorticoid-mediated energetic drive generates an adaptive capacity in response to environmental demand; however, the cost of repeatedly or excessively driving adaptive systems may compromise performance under conditions of elevated environmental pressure. Thus, we will examine the integrative actions of glucocorticoids on the primary limbic sites mediating organismal stress responsiveness within the framework of context-specific adaptation.
2. Synaptic Actions of Glucocorticoids
The cellular actions of glucocorticoids are largely dependent on brain site and the relative expression of GR and MR (de Kloet, 2013a) (Table 1). Glucocorticoids also act in concert with monoaminergic and peptide neurotransmitters, particularly noradrenergic (Quirarte et al., 1997; Roozendaal et al., 2008, 2006a, 2006b, 2002) and CRF systems (Bale and Vale, 2004; Bale, 2005; Meng et al., 2011), which have been reviewed elsewhere (de Kloet, 2013b; Ferry and McGaugh, 2000; Heinrichs and Koob, 2004; Roozendaal, 2000). Further, the synaptic actions of glucocorticoids are critically affected by the recent stress history of the organism, a concept known as ‘metaplasticity’, which will be expanded on in the following section (for review see: Schmidt et al., 2013). Acute and chronic stress often yield different effects on cellular function to meet context-specific energetic demands placed on the organism. Thus, we will discuss the effects of glucocorticoids on cellular function in light of these considerations.
Table 1
Site-specific effects of glucocorticoids on synaptic transmission and plasticity
Reference | Brain Site | GR/MR Manipulation | Stress Condition | Rapid Effects | Delayed Effects |
---|---|---|---|---|---|
Yuen et al. 2009 | mPFC | CORT | Restraint or Platform or CORT i.p. | ↑ eEPSC ampl | |
GR Antagonist i.p. | Forced Swimming | ↔ eEPSC ampl | |||
Musazzi et al. 2010 | mPFC | None | Acute Foot Shock | ↑ sEPSC amplitude | |
Hill et al. 2011 | plPFC | CORT | 20 min Slice Incubation | ↓ mIPSC freq | |
CORT + CB1 Antagonist | 20 min Slice Incubation | ↔ mIPSC freq | |||
Yuen et al. 2012 | mPFC | None | Chronic Stress | ↓ mEPSC ampl and freq, ↓ eEPSC ampl | |
CORT i.c. | Chronic CORT mPFC | ↓ eEPSC ampl | |||
GR Antagonist i.p. or mPFC | Chronic Stress | ↔ eEPSC ampl | |||
PFC cultures | CORT | 7 d CORT Incubation | ↓ mEPSC ampl and freq | ||
CORT + GR Antagonist | 7 d CORT Incubation | ↔ mEPSC ampl and freq | |||
Karst et al. 2005 | CA1 | CORT or CORT:BSA | Bath Application | ↑ mEPSC freq | |
GR Agonist | Bath Application | ↔ mEPSC freq | |||
CORT + GR Antagonist or CORT + GR KO | Bath Application | ↑ mEPSC freq | |||
MR Agonist + GR Antagonist | Bath Application | ↑ mEPSC freq | |||
MR KO + CORT | Bath Application | ↔ mEPSC freq | |||
Karst and Joels 2005 | CA1 | CORT | 20 min Slice Incubation | ↑ mEPSC ampl, ↑ eEPSC ampl | |
GR Agonist | 20 min Slice Incubation | ↑ mEPSC ampl | |||
Oligslagers et al. 2008 | CA1 | CORT + MR Antagonist | Bath Application | ↔ mEPSC freq and ampl | |
CORT | Bath Application | ↔ mIPSC freq and ampl | |||
Pavlides et al. 1996 | CA1 | GR Agonist s.c. | None | ↓ LTP | |
MR Agonist s.c. | None | ↑ LTP | |||
Alfarez et al. 2002 | CA1 | CORT | 20 min Slice Incubation | ↓ LTP | |
Alfarez et al. 2003 | CA1 | None | Chronic Stress | ↓ LTP | |
Yang et al. 2004 | CA1 | None | Inescapable Shock | ↓ LTP, ↑ LTD | |
GR Antagonist i.p. | Inescapable Shock | ↔ LTP, ↔ LTD | |||
Krugers et al. 2006 | CA1 | None | Chronic Stress | ↓ LTP | |
GR Antagonist (oral) | Chronic Stress | ↔ LTP | |||
CORT | 20 min Slice Incubation | ↓ LTP | |||
Wiegert et al. 2006 | CA1 | CORT | Bath Application | ↑ LTP | |
Alfarez et al. 2003 | DG | None | Chronic Stress | ↓ LTP | |
Pasricha et al. 2011 | DG | CORT | Bath Application | ↑ mEPSC freq | |
Maggio and Segal 2009a | Dorsal Hipp | CORT or GR Agonist | Bath Application | ↑ sIPSC Ampl | |
CORT + MR Antagonist | Bath Application | ↑ sIPSC Ampl | |||
Ventral Hipp | CORT | Bath Application | ↓ sIPSC Freq | ||
GR Agonist or CORT + MR Antagonist | Bath Application | ↑ sIPSC Ampl | |||
Maggio and Segal 2009a | Ventral Hipp | MR Agonist or CORT + GR Antagonist | Bath Application | ↓ sIPSC Freq | |
Maggio and Segal 2007 | Dorsal Hipp | CORT | 1 h Slice Incubation | ↓ LTP | |
CORT + GR Antagonist | 1 h Slice Incubation | ↑ LTP | |||
Ventral Hipp | CORT | 1 h Slice Incubation | ↑ LTP | ||
CORT + MR Antagonist | 1 h Slice Incubation | ↔ LTP | |||
Maggio and Segal 2009b | Dorsal or Ventral Hipp | GR Agonist | 1 h Bath Application | ↑ LTD | |
Dorsal Hipp | GR Antagonist s.c. | Forced Swimming | LTD converted to LTP | ||
Dorsal or Ventral Hipp | MR Agonist | 1 h Bath Application | LTD converted to LTP | ||
Ventral Hipp | MR Antagonist s.c. | Forced Swimming | ↑ LTD | ||
GR Antagonist s.c. | Forced Swimming | LTD converted to LTP | |||
Duvarci and Pare 2007 | BLA | CORT | 20 min Incubation | ↓ eIPSPs | |
Karst et al. 2010 | BLA | CORT | Bath Application | ↑mEPSC Freq | |
CORT | 20 min Pulse | ↑ mEPSC Freq (several hours later) | |||
GR KO + CORT | Bath Application | ↑ mEPSC Freq | ↔ mEPSC Freq | ||
MR KO + CORT | Bath Application | ↔ mEPSC Freq | ↔ mEPSC Freq | ||
MR Agonist + GR Antagonist | Bath Application | ↑ mEPSC Freq | ↔ mEPSC Freq | ||
GR Agonist | Bath Application | ↓ mEPSC Freq | ↓ mEPSC Freq | ||
Karst et al. 2010 | BLA | CORT + Cycloheximide | Bath Application | ↑ mEPSC Freq | ↔ mEPSC Freq |
CORT | 2 Pulse Application | ↓ mEPSC Freq | |||
CORT | Acute Restraint + Bath Application | ↓ mEPSC Freq | ↓ mEPSC Freq | ||
GR KO + CORT | Acute Restraint + Bath Application | ↔ mEPSC Freq | ↔ mEPSC Freq | ||
GR Agonist | Acute Restraint + Bath Application | ↓ mEPSC Freq | ↔ mEPSC Freq | ||
GR Antagonist + MR Agonist | Acute Restraint + Bath Application | ↔ mEPSC Freq | ↔ mEPSC Freq | ||
CORT + Cyclohexamide | Acute Restraint + Bath Application | ↓ mEPSC Freq | ↓ mEPSC Freq | ||
CB1 Antagonist | Acute Restraint + Bath Application | ↔ mEPSC Freq | ↔ mEPSC Freq | ||
Hubert et al. 2013 | BLA | None | Chronic Stress | ↑ mEPSC Freq | |
Vouimba et al. 2004 | BLA | None | Platform Stress | ↑ LTP | |
None | Chronic Stress | ↓ LTP | |||
Maroun 2006 | BLA | None | Elevated Platform | ↑ LTP, ↔ LTD | |
Sarabdjitsingh et al. 2012 | BLA | GR Antagonist i.p. | Acute Restraint | ↓ LTP | ↓ LTP |
MR Antagonist i.p. | Acute Restraint | ↔ LTP | ↓ LTP (Gradual) | ||
GR Antagonist i.p. | None | ↔ LTP | ↔ LTP | ||
MR Antagonist i.p. | None | ↔ LTP | ↓ LTP (Gradual) | ||
Karst et al. 2010 | CeA | CORT | Bath Application | ↔ mEPSC Freq | ↔ mEPSC Freq |
2.1 Medial Prefrontal Cortex
The mPFC is the executive control center of the brain, providing top-down regulation of behavioral function. Thus, it is a key site for glucocorticoid actions and regulation of the HPA axis (Akana et al., 2001; Diorio et al., 1993; McKlveen et al., 2013). The rodent mPFC is comprised of three subdivisions, based on connectivity and cytoarchitecture: the anterior cingulate, prelimbic (plPFC), and infralimbic (ilPFC) cortices (Uylings et al., 2003; Vertes, 2004). On the basis of structure and function, these regions are thought to be homologous to human Brodmann areas 24b, 32, and 25, respectively (Gabbott et al., 2005; Uylings et al., 2003).
Molecular and functional studies indicate that glucocorticoids acutely increase glutamatergic output from the mPFC (Popoli et al., 2012). Microdialysis and microelectrode sampling experiments indicate increased extracellular glutamate in vivo in the mPFC following acute stress (Bagley and Moghaddam, 1997; Hascup et al., 2010; Moghaddam, 1993). Acute foot shock increases depolarization-evoked release of glutamate in isolated synaptosomes via a GR-dependent mechanism and increases the amplitude of excitatory postsynaptic currents (EPSCs) in mPFC pyramidal neurons (Musazzi et al., 2010). In adolescent rats, acute stress also increases NMDA- and AMPA-mediated excitatory currents by up-regulating the expression of these receptors on the postsynaptic membrane (via serum- and glucocortico-idinducible kinases) (Yuen et al., 2011, 2009). Thus, existing data suggest that acute stress activates mPFC neurons, permitting down-stream activation of target regions (see below).
Very few studies have assayed inhibitory neurotransmission after acute corticosterone application. A recent study in mice found that corticosterone decreased miniature inhibitory postsynaptic currents (mIPSCs) and increased paired pulse inhibition, suggesting that acute glucocorticoid exposure disinhibits glutamatergic output from the mPFC (Hill et al., 2011). The effects of corticosterone on mIPSCs were prevented by CB1 antagonism, suggesting that the effect of acute stress on disinhibition of mPFC pyramidal neurons is likely endocannabinoid-dependent (Hill et al., 2011). Overall, the present data suggest that glucocorticoids acutely increase glutamatergic neurotransmission and decrease inhibitory neurotransmission in the mPFC. It remains to be determined whether reduced inhibition contributes to enhanced mPFC excitability.
The synaptic effects of glucocorticoids in the mPFC during chronic stress are not as well established, and the effects on excitatory and inhibitory neurotransmission are largely unknown. Repeated restraint stress, chronic unpredictable stress, or chronic corticosterone treatment decrease apical dendritic complexity of pyramidal neurons (Cerqueira et al., 2007, 2005; Cook and Wellman, 2004; Goldwater et al., 2009; Liston et al., 2006; Radley et al., 2006, 2004; Wellman, 2001). Conversely, repeated restraint stress increases the complexity and transcriptional activity of prefrontal GABAergic interneurons (Gilabert-Juan et al., 2012). While the functional consequence of these morphologic modifications is unknown, the direction of changes suggests both decreased pyramidal cell excitability and increased capacity for interneuron-mediated inhibition.
In adolescent rats, chronic stress decreases NMDA- and AMPA-mediated currents in the mPFC through increased degradation of postsynaptic glutamate receptors (Yuen et al., 2012). Notably, adolescence is a developmental period marked with pruning of prefrontal glutamatergic synapses, particularly those to the basolateral amygdala (BLA) (Cressman et al., 2010). Therefore, it remains to be determined whether increased degradation of glutamate receptors is due to chronic stress or stress/development interactions (for review on adolescent synaptic plasticity see Selemon, 2013). In adult animals, chronic corticosterone administration decreases expression of NMDA subunit NR2B and AMPA subunits GluR2/GluR3 in the ventral mPFC (Gourley et al., 2009). However, the impact of chronic stress on inhibitory neurotransmission in the mPFC has not been directly tested, further highlighting the need for a better understanding of chronic glucocorticoid effects on synaptic physiology in adult animals.
2.2 Hippocampus
The hippocampus is critical for processes related to memory, particularly spatial and contextual learning and memory retrieval (Roozendaal and McGaugh, 1997a; Roozendaal et al., 2001). The hippocampus consists of multiple subregions, including the CA1 and CA3 regions, the dentate gyrus, and the ventral subiculum (Swanson and Cowan, 1977). The actions of glucocorticoids in the hippocampus have long been recognized (McEwen et al., 1968) and have been studied in great detail. Both MR and GR are abundantly expressed in hippocampus, and memory processing is heavily influenced by circulating levels of glucocorticoids (Fuxe et al., 1987; Meaney et al., 1985; Oitzl and de Kloet, 1992; Reul and de Kloet, 1986). Moreover in addition to a role in memory processes, the hippocampus (specifically the ventral subiculum) inhibits HPA axis responses to psychogenic stressors (Herman et al., 2003, 1998, 1989).
The cellular actions of glucocorticoids in the hippocampus (predominantly CA1 pyramidal neurons) have been very well characterized, particularly by Joëls and colleagues (for review see Joëls et al., 2012). The rapid, non-genomic effects of glucocorticoids (occurring within minutes) are mediated primarily by the membrane-associated MR at the pre- and postsynaptic membrane (Joëls et al., 2008; Karst et al., 2005; Olijslagers et al., 2008; Pasricha et al., 2011). Activation of MR increases neuronal excitability by increasing the probability of glutamate release, suppressing potassium conductance, and increasing glutamate receptor trafficking (Groc et al., 2008; Karst et al., 2005; Olijslagers et al., 2008). Conversely, the delayed effects of glucocorticoids are mediated primarily by GR (Karst and Joëls, 2005). Activation of GR causes delayed suppression of neuronal excitability (due to enhanced calcium influx, decreased calcium efflux, and increased calcium-dependent potassium current) and synaptic plasticity (impaired long-term potentiation (LTP) due to lateral diffusion of glutamate receptors), presumably to normalize hippocampal activity after stress and protect information acquired during the stressful experience, respectively (Bhargava et al., 2002; Chameau et al., 2007; Groc et al., 2008; Joëls and de Kloet, 1990, 1989; Joëls, 2006; Joëls et al., 2009, 2007; Karst et al., 1994; Kim and Diamond, 2002; Pavlides et al., 1996). The ventral part of the hippocampus responds to glucocorticoids much differently than the dorsal hippocampus. Upon corticosterone application, the ventral hippocampus has reduced firing frequency accommodation and more depolarization-associated spikes (Maggio and Segal, 2009a). Further, unlike CA1, stress does not lead to impaired LTP in the ventral subiculum (Maggio and Segal, 2010, 2009b, 2007). These differential effects in the ventral hippocampus may allow for a longer window of acquisition when this brain region is activated during a stressful experience (Joëls et al., 2012). The prominent role of the ventral hippocampus/subiculum in inhibiting HPA axis stress responses raises the possibility that differential actions of stress/glucocorticoids on synaptic function may be relevant to stress regulation.
The effect of chronic stress on cellular physiology in the hippocampus is fairly well characterized. In chronically stressed animals, LTP in the CA1 hippocampal area and dentate gyrus is impaired at basal levels of corticosterone and is not further impaired in the CA1 hippocampal area or dentate gyrus following corticosterone application (Alfarez et al., 2003). The effects of chronic stress on synaptic plasticity in the CA1 hippocampal area are likely GR-mediated, as blockade of GR during the last 4 days of chronic stress blocks the effects of stress on LTP (Krugers et al., 2006). Further, animals with a history of chronic stress have GR-dependent increases in calcium influx into CA1 neurons at basal levels of corticosterone, which may contribute to impaired synaptic plasticity (Karst and Joëls, 2007). These impairments of synaptic plasticity in the hippocampus following chronic stress likely underlie some of the cognitive impairments observed with chronic stress exposure.
2.3 Amygdala
The amygdala integrates emotional and sensory information for the expression of fear and anxiety (Charney and Deutch, 1996; Davis, 1997; Phelps and LeDoux, 2005; Weiskrantz, 1956). Additionally, the amygdala is involved in the learning and consolidation of emotional memories and has an essential role in conditioned responses (Davis, 1992; LeDoux, 2012). The amygdala is composed of multiple subnuclei that are anatomically and physiologically heterogeneous. Of these subnuclei, the basolateral (BLA), central (CeA), and medial (MeA) cell groups appear to be most closely linked to regulation of stress responses (Sah et al., 2003; Swanson and Petrovich, 1998; Ulrich-Lai and Herman, 2009).
Similar to the hippocampus, glucocorticoids rapidly increase mEPSCs in the BLA via the membrane associated-MR (Karst et al., 2010). In contrast to the suppressive and normalizing effects of glucocorticoids on cellular responses in the hippocampus following acute stress, glucocorticoids in the BLA prolong excitatory synaptic responses via GR (Duvarci and Paré, 2007; Karst et al., 2002; Liebmann et al., 2008; Rainnie et al., 2004). Further, subsequent corticosterone application or acute stress prior to slice preparation decreases mEPSC frequency in the BLA via the GR and CB1 receptor (Karst et al., 2010). Thus, the cellular responses of BLA neurons to glucocorticoids depend on the recent history of stress exposure, an effect known as metaplasticity (Joëls et al., 2012; Karst et al., 2010). In response to acute noise stress, amygdalar c-fos mRNA expression increases only during the rising phase of pulsatile glucocorticoid infusion in adrenalectomized animals, as opposed to the falling phase or during constant infusion of corticosterone, further suggesting that rapid reactivity of the amygdala to glucocorticoids is highly dependent on recent changes in glucocorticoid status (Sarabdjitsingh et al., 2010a). Stress also impairs LTP in projections from the BLA to the plPFC and in the ventral hippocampus-mPFC pathway (Maroun and Richter-Levin, 2003; Richter-Levin and Maroun, 2010). The stress-induced impairment of LTP in the ventral hippocampus-mPFC pathway does not occur in previously stressed animals if the BLA is stimulated or if animals receive a second bout of stress (Richter-Levin and Maroun, 2010), another example of metaplasticity. While stress impairs LTP in BLA projections, LTP in afferents to the BLA is strengthened, including input from the entorhinal cortex, external capsule, and plPFC (Kavushansky et al., 2006; Maroun, 2006; Rodríguez Manzanares et al., 2005; Vouimba et al., 2004). Further, unpredictable footshock facilitates LTP in the BLA, which may be due to a stress-induced increase in GluR1 in spines from dendritic stores (Li et al., 2011; Hubert et al., 2013). The effects of stress on LTP in the BLA appear to be primarily mediated by GR as MR is involved in the maintenance of LTP, regardless of circulating glucocorticoid levels (Sarabdjitsingh et al., 2012b).
3. Connectivity and Integration of Glucocorticoid-Responsive Circuits
Stress integrative functions are regulated by forebrain circuits, primarily involving the above mentioned regions (mPFC, hippocampus, and amygdala) (Ulrich-Lai and Herman, 2009) (Table 2). Notably, these interconnected limbic forebrain sites do not send substantial projections to stress-effector neurons in the PVN. Thus, their influence on HPA axis output is communicated through intervening PVN-projecting neurons. Inhibitory GABAergic inputs to the PVN emanate from several structures in the basal forebrain and hypothalamus, including the BST, preoptic area (POA), and dorsomedial hypothalamus (DMH) (Boudaba et al., 1996; Radley et al., 2009; Roland and Sawchenko, 1993). In contrast, glutamatergic inputs to the PVN originate predominantly from hypothalamic nuclei, including the ventromedial hypothalamus (VMH), posterior hypothalamus (PH), as well as DMH (Ulrich-Lai et al., 2011). Neurons in these regions, including those that project to the PVN, show pronounced activation by stressful stimuli (Cullinan et al., 1996, 1995), consistent with a role in stress regulation. All hypothalamic PVN-projecting regions receive mixed GABAergic and glutamatergic input from other hypothalamic nuclei (Myers et al., 2013), which may be responsible for intrahypothalamic mechanisms governing the integration of forebrain limbic inputs and stress responsiveness based on metabolic demand.
Table 2
Site-specific effects of glucocorticoids on limbic circuits regulating HPA axis activity
Reference | Brain Site | GR/MR Manipulation | Stress Condition | HPA Outcome |
---|---|---|---|---|
Diorio et al. 1993 | mPFC | CORT | Acute Restraint | ↓ ACTH, ↓ CORT |
Ether | ↔ ACTH, ↔ CORT | |||
Akana et al. 2001 | mPFC | CORT | Acute Restraint | ↓ ACTH, ↔ CORT |
Repeated Cold | ↓ ACTH, ↔ CORT | |||
McKlveen et al. 2013 | plPFC | GR Knockdown | Acute Restraint | ↑ ACTH, ↑ CORT |
Chronic Variable Stress | ↑ Basal CORT | |||
ilPFC | GR Knockdown | Acute Restraint | ↑ CORT | |
Chronic Variable Stress | ↑ CORT | |||
Van Haarst et al. 1997 | Hipp | GR Antagonist | Diurnal Peak | ↓ ACTH, ↔ CORT |
MR Antagonist | Diurnal Peak | ↑ ACTH, ↑ CORT | ||
Feldman and Weidenfeld 1999 | GR Antagonist | Audiogenic or Phototic | ↑ ACTH, ↑ CORT | |
MR Antagonist | Audiogenic or Phototic | ↑ ACTH, ↑ CORT | ||
Fitzsimons et al. 2013 | Hipp | GR Knockdown | Basal (AM & PM) | ↔ CORT |
Conditioned Fear | ↔ CORT | |||
Mitra et al. 2009 | BLA | MR Overexpression | Acute Restraint | ↓ CORT |
Akana et al. 2001 | CeA | CORT | Acute Restraint | ↔ ACTH, ↔ CORT |
Repeated Cold | ↔ ACTH, ↔ CORT | |||
Shepard et al. 2003 | CeA | CORT | Elevated Plus Maze | ↑ CRF, ↑ CORT |
Kolber et al. 2008 | CeA | GR KO | Conditioned Fear | ↔ CRF |
Myers and Greenwood-Van Meerveld 2012 | CeA | GR Antagonist | Repeated Water Avoidance | ↓ CORT |
MR Antagonist | Repeated Water Avoidance | ↓ CORT |
Importantly, GR is expressed in numerous hypothalamic PVN-projecting neurons, including the POA, DMH, and arcuate nucleus (Fuxe et al., 1987). In the PVN, glucocorticoids signal by non-genomic mechanisms to rapidly inhibit activation of parvocellular neurons and consequent drive on the HPA axis (Evanson et al., 2010; Tasker and Herman, 2011). These ‘fast feedback’ glucocorticoid effects are non-genomic in nature, acting through an endocannabinoid-dependent mechanism to inhibit glutamatergic drive (Tasker and Herman, 2011). Fast inhibitory effects of glucocorticoids are attenuated in animals with PVN GR deletion, consistent with action via GR (Haam et al., 2010). However, the role of GR in other PVN-projecting hypothalamic cell groups remains to be determined.
3.1 Medial Prefrontal Cortex
Previous studies determined that glucocorticoids act at the mPFC to inhibit HPA axis responses to psychogenic stress (e.g. restraint) (Akana et al., 2001; Diorio et al., 1993). More recently, we established that glucocorticoids bind specifically to GR in the plPFC or ilPFC to regulate glucocorticoid responses to acute psychogenic stress (McKlveen et al., 2013). Glucocorticoids also mobilize endocannabinoids in the mPFC in response to acute stress, and cannabinoid receptor 1 (CB1) antagonism disinhibits the HPA axis (Hill et al., 2011). Thus, glucocorticoids acutely inhibit the HPA axis via GR and consequent effects on endocannabinoid signaling. Our lab has also demonstrated that chronic stress selectively recruits ilPFC glucocorticoid signaling to inhibit the HPA axis stress response during chronic stress, while the plPFC appears to be involved in maintaining basal glucocorticoid levels during chronic stress (McKlveen et al., 2013). Thus, glucocorticoids in the mPFC play a region- and context-specific role in HPA axis regulation during acute and chronic stress. Prefrontal output neurons are almost exclusively glutamatergic, and projections from the plPFC to GABAergic neurons in the anterior BST mediate inhibition of HPA responses to acute stress (Radley et al., 2009). The intervening structures relaying the influence of the ilPFC to the PVN have yet to be determined. Preliminary studies from our group indicate the PH may be important for integrating ilPFC output with HPA responses (Myers et al., 2012a).
3.2 Hippocampus
The hippocampus is involved in terminating HPA axis responses to acute psychogenic stress, consistent with a role in memory and emotion processing (Cullinan et al., 1993; Herman et al., 1989; Mueller et al., 2004; Radley and Sawchenko, 2011). The importance of the hippocampus for glucocorticoid negative feedback is highlighted by the dense expression of GR and MR in this area (Reul and de Kloet, 1985), as well as functional studies demonstrating diminished feedback efficacy following hippocampal lesions (Herman and Mueller, 2006; Mueller et al., 2006). Surprisingly, feedback effects of glucocorticoids in the hippocampus may be mediated by MR, as multiple studies indicate that MR antagonists in the hippocampus increase HPA axis output (Feldman and Weidenfeld, 1999; van Haarst et al., 1997). The role of hippocampal GR in glucocorticoid negative feedback is more equivocal. Although GR antagonism has been shown to increase HPA axis activation in response to psychogenic stress (Feldman and Weidenfeld, 1999), other studies indicate that local GR inactivation actually decreases ACTH release at the diurnal peak of glucocorticoid secretion (van Haarst et al., 1997). Further, recent studies employing viral knockdown of GR in the hippocampus found no effect on either basal or stress-induced levels of glucocorticoids (Fitzsimons et al., 2013). As was the case for the mPFC, the output of the hippocampus is largely glutamatergic, and therefore excitatory. Consequently, numerous studies suggest that hippocampal inhibition of responses to psychogenic stress is mediated by activation of PVN-projecting GABAergic neurons in the BST, POA, DMH, and possibly the peri-PVN region (Cullinan et al., 1993; Herman et al., 2002; Mueller et al., 2006; Myers et al., 2013; Radley and Sawchenko, 2011).
3.3 Amygdala
The BLA is an activator of acute HPA axis stress responses, as excitotoxic lesions of the BLA dampen ACTH and corticosterone secretion following acute but not repeated restraint (Bhatnagar et al., 2004). Furthermore, BLA inhibition induced by potassium channel overexpression inhibits glucocorticoid responses to restraint (Mitra et al., 2009a). Overexpression of MR in the BLA is sufficient to attenuate corticosterone responses to restraint (Mitra et al., 2009b), suggesting that as in the hippocampus, BLA MR serves a role in feedback inhibition. As with the mPFC, endocannabinoid signaling in the BLA is involved in HPA axis regulation. The CB1 receptor may be acting as a ‘gatekeeper’ for BLA-driven glucocorticoid responses after acute stress, as stress decreases inhibitory endocannabinoid signaling specifically in the BLA (Hill et al., 2009). Thus, the data suggest the BLA increases the magnitude of HPA axis responses to acute psychogenic stress. Projection neurons of the BLA are largely glutamatergic, and heavily innervate down-stream GABAergic neurons in the CeA and MeA (Bienkowski and Rinaman, 2012; Pare et al., 1995). The BLA also innervates the BST (Myers et al., 2013), which may link BLA activation with HPA modulation.
Anatomical studies indicate that the CeA provides major output to regions that mediate not only fear and anxiety-related behaviors but also HPA axis responses (LeDoux et al., 1988; Myers et al., 2013; Swanson and Petrovich, 1998). PVN projecting GABAergic neurons of the POA receive inhibitory input from the CeA (Prewitt and Herman, 1998), suggesting the CeA disinhibits HPA axis responses. However, there are somewhat divergent reports on regulation of glucocorticoid release by the CeA. Bilateral lesions of the CeA inhibit ACTH responses to immobilization (Beaulieu et al., 1987, 1986) and corticosterone responses to footshock (Roozendaal et al., 1991), but do not affect responses to acute restraint (Prewitt and Herman, 1997). Other lesion studies have suggested that the CeA activates the HPA axis in response to systemic but not psychogenic stressors (Dayas et al., 1999; Xu et al., 1999). Importantly, corticosterone implanted on the CeA potentiates HPA axis responses to acute psychogenic stress (Shepard et al., 2003). Further, glucocorticoid responses to repeated psychogenic stress can be inhibited by GR or MR antagonism in the CeA (Myers and Greenwood-Van Meerveld, 2012), suggesting that the CeA has feed-forward effects on the HPA axis that may be linked to context-specific enhanced stress excitability. The MeA appears to be selectively involved in generating HPA responses to acute, but not chronic, psychogenic stress (Dayas et al., 1999; Ma and Morilak, 2005; Solomon et al., 2010). Although very little is known about the actions of glucocorticoids in the MeA, this region provides mixed glutamatergic and GABAergic innervation of the BST and hypothalamus, indicating the potential for subregional differences in the effects of the MeA on stress responding (Myers et al., 2013; Poulin et al., 2008).
3.4 Bed Nucleus of the Stria Terminalis
The BST provides direct input to the PVN and is a hub for forebrain inputs and trans-synaptic input to the PVN. Regulation of the HPA axis occurs at the level of the BST in a regional- and context-specific manner. The anterolateral BST receives projections from the plPFC and the ventral subiculum and sends GABAergic projections to the PVN that inhibit HPA axis responses to acute stress (Radley and Sawchenko, 2011). Additionally, lesions of the principal nucleus in the posterior BST increase glucocorticoid responses to acute and chronic stress, suggesting an inhibitory role of this region in HPA axis regulation (Choi et al., 2008b, 2007). Lesions of the dorsomedial/fusiform nuclei in the anteroventral BST decrease HPA axis responses to acute stress, whereas lesions to this same region under chronic stress increase HPA axis responses (Choi et al., 2008a, 2007). Thus, the anteroventral BST appears to be sensitive to circulating glucocorticoid levels such that this region is involved in promotion and inhibition of HPA axis responses to acute and chronic stress, respectively. Moreover, the functional ‘switch’ in anteroventral BST suggests stress-induced metaplasticity at the physiologic level, as prior experience alters the role this nucleus from excitation to inhibition.
3.5 Hindbrain
Hindbrain monosynaptic inputs to the PVN are also modulated by glucocorticoids and interact with forebrain stress-regulatory circuits. The PVN receives dense input from brainstem noradrenergic and adrenergic neurons primarily from the NTS, as well as C1–C3 (Cunningham Jr. and Sawchenko, 1988; Cunningham Jr. et al., 1990). Lesions of these neurons attenuate HPA axis responses to systemic stressors, while adrenergic receptor stimulation activates CRF and ACTH secretion, indicating that catecholamines are necessary and sufficient for HPA axis activation (Plotsky, 1987; Plotsky et al., 1989). PVN-projecting neurons in the NTS also contain peptidergic neuromodulators (e.g., glucagon-like peptide-1 (GLP-1), neuropeptide Y) that are HPA axis excitatory (Harfstrand, 1987; Merchenthaler et al., 1999; Sawchenko et al., 1985; Tauchi et al., 2008). The NTS receives descending projections from forebrain limbic stress-regulatory regions, including the mPFC and CeA (Schwaber et al., 1982; van der Kooy et al., 1984), indicating possible involvement of the NTS in emotional and cognitive stress responses. In agreement with this hypothesis, GR activation in the NTS facilitates memory consolidation through involvement of the BLA (Roozendaal et al., 1999).
In the NTS, expression of preproglucagon (PPG) mRNA, which encodes the stress-excitatory GLP-1, is suppressed following acute and chronic stress in a glucocorticoid-dependent manner (Ghosal et al., 2013; Zhang et al., 2009). Loss of PPG mRNA is correlated with a reduction in GLP-1 fiber density in the PVN, indicative of reduced capacity for NTS neurons to release GLP-1 and stimulate ACTH secretion (Tauchi et al., 2008; Zhang et al., 2009). Glucocorticoid-induced reductions in PPG mRNA occur within 30 min and are not accompanied by reduced PPG gene transcription, indicating that glucocorticoid effects are likely mediated by destabilization of existing RNA pools (Zhang et al., 2009). Glucocorticoids decrease RNA stability in vitro, and this may represent an adaptive mechanism for limiting PVN excitation following stress (Stellato, 2004).
Serotonin (5-HT) participates in HPA axis activation by way of projections from the midbrain and pontine raphe nuclei (Lowry, 2002; Sawchenko et al., 1983). Lesion studies indicate serotonin provides excitation of the HPA axis (Jorgensen et al., 1998). However, direct serotonin input to the PVN is somewhat limited as the majority of serotoninergic fibers terminate in the peri-PVN (Sawchenko et al., 1983). In addition, 5-HT heavily innervates most limbic regions that project to the PVN (Kosofsky and Molliver, 1987; Palkovits et al., 1977), and may modulate HPA axis responses indirectly. Additionally, glucocorticoids regulate the expression of tryptophan hydroxylase-2 (TPH2), an enzyme involved in 5-HT synthesis (Clark et al., 2005). Dexamethasone reduces TPH2 mRNA and protein in the raphe leading to reduced 5-HT synthesis in the mPFC, all of which is blocked by co-administration of a GR antagonist (Clark et al., 2008, 2005). Furthermore, daily rhythms in TPH2 mRNA are dependent on fluctuations in circulating glucocorticoids (Malek et al., 2007). Together, these studies indicate the importance of glucocorticoids for 5-HT activity throughout the brain.
3.6 Limbic Integration of Glucocorticoid Signaling
Based on the substantial interconnectedness of limbic forebrain regions and hypothalamic PVN-projecting nuclei, it is highly likely that glucocorticoid actions in a given cell population or brain region can have pronounced effects on the function and output of other regions. Few studies have investigated how GR signaling is integrated at a circuit level, largely focusing on downstream effects in the PVN. For instance, glucocorticoids in the CeA increase CRF expression in the PVN (Shepard et al., 2000). Although glucocorticoids in the CeA have been shown to increase CRF mRNA in the anterior BST (Shepard et al., 2006), in-depth studies of glucocorticoid interactions within the forebrain are lacking. A recent study employing selective deletions of GR within the mesolimbic dopaminergic system supports this hypothesis of integrated GR signaling. In these experiments, GR knockout in neurons of the nucleus accumbens decreased the firing rate of ventral tegmental dopamine-releasing neurons, resulting in social aversion (Barik et al., 2013). Interestingly, these effects could not be produced by deleting GR directly within the tegmental dopamine-releasing neurons. More studies focused on the integrative actions of glucocorticoids within the limbic forebrain are necessary to decipher the mechanisms of central stress regulation.
4. Behavioral Effects of Glucocorticoids
Activation of glucocorticoid responsive pathways, particularly within the limbic system, can lead to pronounced changes in behavior that have long-term implications for organismal well-being (Arnett et al., 2011) (Table 3). Functional changes at the synaptic and circuit levels affect a variety of neurobehavioral systems. For instance, mice with deletion of GR in the forebrain (mPFC, hippocampus, and BLA) exhibit increased despair-like behavior in the forced swim test (FST) and tail-suspension test (TST), as well as anhedonia in the sucrose preference test (SPT) (Boyle et al., 2005). In addition, mice overexpressing MR in the forebrain exhibit decreased anxiety-like behavior in the open field test (OFT) and elevated-plus maze (EPM) (Rozeboom et al., 2007). These studies support a role for forebrain glucocorticoid signaling in inhibiting behavioral responses indicative of depression and anxiety. Although numerous psychiatric disorders are associated with dysregulation of stress systems, the precise role of glucocorticoid dyshomeostasis in pathology remains to be determined.
Table 3
Site-specific effects of glucocorticoids on limbic circuits regulating depression-, anxiety-, and fear-related behaviors
Reference | Brain Site | GR/MR Manipulation | Behavioral Paradigm | Behavioral Outcome |
---|---|---|---|---|
Roozendaal et al. 2004 | plPFC | GR Agonist | Delayed Alternation T-Maze | ↑ Error Rate |
Barsegyan et al. 2010 | plPFC | GR Agonist | Inhibitory Avoidance | ↑ Shock Avoidance |
CORT:BSA | Delayed Alternation T-Maze | ↑ Error Rate | ||
Inhibitory Avoidance | ↑ Shock Avoidance | |||
McKlveen et al. 2013 | plPFC | GR Knockdown | Forced Swim Test | ↔ Immobility |
Open Field | ↔ Center Time | |||
ilPFC | GR Knockdown | Forced Swim Test | ↓ Immobility | |
Open Field | ↔ Center Time | |||
Donley et al. 2005 | Dorsal Hipp | GR Antagonist | Conditioned Fear | ↔ Freezing |
Ventral Hipp | GR Antagonist | Conditioned Fear | ↓ Freezing | |
Fitzsimons et al. 2013 | Hipp | GR Knockdown | Conditioned Fear | ↓ Freezing |
Roozendaal and McGaugh 1997 | BLA | GR Agonist | Inhibitory Avoidance | ↑ Shock Avoidance |
Donley et al. 2005 | BLA | GR Antagonist | Conditioned Fear | ↓ Freezing |
Mitra et al. 2009 | BLA | MR Overexpression | Elevated Plus Maze | ↑ Open Arm Time |
Shepard et al. 2000 | CeA | CORT | Elevated Plus Maze | ↓ Open Arm Time |
Kolber et al. 2008 | CeA | GR KO | Conditioned Fear | ↓ Freezing |
Myers and Greenwood-Van Meerveld 2007 | CeA | CORT + GR Antagonist | Elevated Plus Maze | ↔ Open Arm Time |
CORT + MR Antagonist | Elevated Plus Maze | ↔ Open Arm Time | ||
Myers and Greenwood-Van Meerveld 2010 | CeA | GR Agonist | Elevated Plus Maze | ↓ Open Arm Time |
MR Agonist | Elevated Plus Maze | ↓ Open Arm Time |
4.1 Medial Prefrontal Cortex
Glucocorticoids have profound effects on prefrontal-mediated behaviors, including working memory, executive function, behavioral flexibility, and affect. Acutely, glucocorticoids act through the cAMP pathway in the plPFC to impair working memory and enhance memory consolidation (Barsegyan et al., 2010; Roozendaal et al., 2004). Thus, glucocorticoid signaling reinforces consolidation of emotionally salient events while minimizing competing information, underscoring the context-specific actions of glucocorticoids. Chronically, glucocorticoids also impair performance in working memory tasks (Mizoguchi et al., 2004, 2000). Acute restraint stress impairs the ability of animals to execute the delayed spatial win-shift task, a prefrontal-mediated task, which relies on the ability to use information acquired prior to a delay to prospectively plan where to retrieve food in a radial arm maze (Butts et al., 2011). Chronic unpredictable stress or repeated restraint stress also impair behavioral flexibility (set-shifting and/or reversal learning) in the extra-dimensional set-shifting task, which requires animals to inhibit a previously learned response (Birrell and Brown, 2000; Bondi et al., 2008; Liston et al., 2006). Further, chronic stress favors habitual strategies in two instrumental operant tasks involving evaluation of outcome value and action-outcome contingency, reducing the ability of animals to employ flexible, goal-directed decision-making (Dias-Ferreira et al., 2009). Glucocorticoids in the mPFC also play a major role in affect. Knockdown of GR in the ilPFC increases immobility in the forced swim test, indicative of increased depression-like behavior (McKlveen et al., 2013). These behaviors share a common ‘prefrontal-related’ feature, in that the organism must coordinate an appropriate context-specific response by maintaining attention and flexibly inhibiting inappropriate responses.
4.2 Hippocampus
As with the cellular effects of glucocorticoids in the hippocampus, the role of glucocorticoids in hippocampal-dependent behaviors (e.g. learning and memory) has been extensively studied. Similar to glucocorticoid actions at the synapse, the effects of glucocorticoids on learning and memory are temporally- and context-dependent (Diamond et al., 1994). In the short term, stress can facilitate the formation of emotionally salient memories, that are important for adaptation (de Kloet et al., 1999; Smeets et al., 2009). In line with this, MR is important for initial appraisal and strategy selection in a novel spatial orientation task and for acquisition of fear memory (Khaksari et al., 2007; Oitzl and de Kloet, 1992). Conversely, the GR mediates consolidation of context-relevant information to optimize adaptation and survival in a variety of spatial and fear-related memory tasks (Abrari et al., 2009; Chen et al., 2012; Conrad et al., 1999; Donley et al., 2005; Fitzsimons et al., 2013; Gutièrrez-Mecinas et al., 2011; Oitzl and de Kloet, 1992; Pugh et al., 1997; Revest et al., 2010; Sandi et al., 1997). Thus, elevated glucocorticoid levels are important for the acquisition and consolidation of affectively relevant information and the disruption of memory processes that are less relevant for adaptation. Conversely, acute levels of glucocorticoids disrupt memory retrieval in tasks that have already been acquired (Roozendaal, 2002), including spatial memory in a radial arm task or Morris water maze (de Quervain et al., 1998; Diamond and Rose, 1994; Diamond et al., 1999; Ferguson and Sapolsky, 2008), novel object recognition (Baker and Kim, 2002), inhibitory avoidance (Schutsky et al., 2011) and contextual fear conditioning (Atsak et al., 2012a). Chronic stress exposure impairs hippocampal-dependent tasks as well (Conrad et al., 1996; Kleen et al., 2006; Krugers et al., 1997; Luine et al., 1994). For instance, chronic stress disrupts spectral coherence (matching of temporal structure in signals recorded from the hippocampus and the mPFC) in the projection from the ventral hippocampus to the mPFC, which may contribute to stress-induced deficits in spatial reference memory (Oliveira et al., 2013). Therefore, the timing of enhanced glucocorticoid levels must be appropriate for the context. Thus, glucocorticoids act within specific time domains in the hippocampus to facilitate the acquisition and consolidation of spatial and emotionally salient information relevant to adaptation and disrupt memory processes less essential for survival.
4.3 Amygdala
The amygdala organizes fear and anxiety responses (Adamec, 1990; Davis et al., 2010; Kopchia et al., 1992; LeDoux, 2012; Shepard et al., 2000). Roozendaal and colleagues indicate that glucocorticoids in the BLA play a prominent role in promoting learning and memory of aversive stimuli (Roozendaal et al., 1996). Specifically, injections of GR agonists and antagonists in the BLA immediately after training in an inhibitory avoidance task point to the necessity and sufficiency of GR activation for long-term retention (Roozendaal and McGaugh, 1997b; Roozendaal, 2000). GR antagonist injection in the BLA prior to training also impairs inhibitory avoidance learning (Roozendaal and McGaugh, 1997b). In addition, blockade of GR prior to contextual fear conditioning decreases freezing in a 24 hour retention test (Donley et al., 2005). Glucocorticoids in the BLA are also involved in memory consolidation and reconsolidation of auditory fear conditioning as GR antagonism disrupts both of these memory processes (Jin et al., 2007). Ipsilateral lesions of the BLA block the memory enhancing effects of GR agonist infusion into the mPFC after inhibitory avoidance training (Roozendaal et al., 2009), suggesting that the effects of GR on memory consolidation depend on bidirectional communication between the BLA and the mPFC. The BLA is also necessary for glucocorticoid modulation of memory functions primarily mediated by the hippocampus and mPFC, such as memory retrieval and working memory, respectively (Nathan et al., 2004; Roozendaal and McGaugh, 1997a; Roozendaal et al., 2004, 2003). The effects of glucocorticoids on learning and memory may be mediated by endocannabinoids, as intra-BLA CB1 antagonism blocks glucocorticoid-mediated post-training enhancement of memory consolidation (Akirav, 2013; Atsak et al., 2012b; Campolongo et al., 2009).
The lateral portion of the BLA targets the CeA, which is considered the output nucleus for the behavioral expression of conditioned fear responses (Amorapanth et al., 2000). Growing evidence suggests that the CeA is also necessary for learning and consolidation of conditioned fear (Nader et al., 2001; Wilensky et al., 2006). However, recent studies have defined an amygdala microcircuit wherein BLA projections activate inhibitory neurons within the CeA that gate behavioral output (Ciocchi et al., 2010; Haubensak et al., 2010; Tye et al., 2011), suggesting the amygdala has the ability to both facilitate and inhibit behaviors indicative of fear and anxiety. Viral cre recombinase injections into the CeA of GR floxed mice produce a CeA-specifc GR knockout that exhibits deficits in conditioned fear (Kolber et al., 2008). Glucocorticoids localized to the CeA increase anxiety-like behavior in the EPM (Shepard et al., 2000), an effect that can be prevented by GR or MR antagonists in the CeA (Myers and Greenwood-Van Meerveld, 2007). In addition, specific GR or MR agonists injected in the CeA promote anxiety-like behavior (Myers and Greenwood-Van Meerveld, 2010a). The long-term anxiogenic effects of glucocorticoid signaling in the CeA are thought to be mediated by transcriptional interactions with CRF, perhaps driving synaptic plasticity (Kolber et al., 2010; Laryea et al., 2013; Myers and Greenwood-Van Meerveld, 2010b; Shekhar et al., 2005). In addition, downstream activation of the BST may play a role in behavioral responses to stress (Poulos et al., 2010; Singewald et al., 2003; Sullivan et al., 2004). Chronic glucocorticoid signaling in the BST appears to induce a shift toward anxiogenic behavior and enhance unconditioned fear and stress-induced learning (Bangasser et al., 2005; Shepard et al., 2009; Ventura-Silva et al., 2012). However, more work delineating the actions of glucocorticoids underlying BST-mediated behaviors during stress exposure is needed.
5. Clinical Relevance of Glucocorticoid Signaling
Recent technological advances have allowed for more in-depth analysis of glucocorticoid effects in humans. In healthy controls, glucocorticoids have profound time-dependent effects on memory and decision-making. For instance, the synthetic glucocorticoid hydrocortisone given 30 min prior to a memory encoding task impairs memory contextualization performance, while hydrocortisone given 210 min prior to the task has the opposite effect (van Ast et al., 2013). These findings suggest that the rapid effects of glucocorticoids promote memory of the most emotionally salient aspects of an experience, whereas the delayed effects of glucocorticoids may enhance cognitive function. Similarly, hydrocortisone given before sleep in the evening after the presentation of emotional imagery enhances memory consolidation of emotional information and suppresses amygdalar activation during retrieval (van Marle et al., 2013). Acute stress also shifts hippocampal-dependent learning toward habitual striatum-dependent learning, an effect that can be prevented by pretreatment with an oral MR antagonist (Schwabe et al., 2013). Additionally, this stress-induced shift in memory processing correlates with altered coupling of the amygdala with the hippocampus and dorsal striatum, marked by decoupling of amygdala-hippocampal connectivity and strengthening of amygdala-putamen connectivity. The effects of stress on alterations in amygdala connectivity with the hippocampus and dorsal striatum are also prevented by MR antagonist pretreatment (Schwabe et al., 2013). Thus, the importance of glucocorticoids for regulating cognitive function and the brain regions mediating glucocorticoid activity during these processes are beginning to be characterized in humans. While there has been a recent increase in the number of studies assessing the central actions of glucocorticoids in humans, there is still a vital need to establish the mechanistic effects of glucocorticoids in health as well as psychopathology.
Glucocorticoid signaling is essential for adaptation to stress and alterations in glucocorticoid activity may underlie transitions to pathology in humans. In fact, several polymorphisms of the glucocorticoid receptor gene have been reported in individuals with posttraumatic stress disorder (PTSD), as well as major depression. For instance, the single nucleotide polymorphism (SNP) Bcl-1 (C to G nucleotide change involving a restriction site in intron 2) is associated with glucocorticoid hypersensitivity and lower cortisol levels (Derijk et al., 2008; Hauer et al., 2011; Huizenga et al., 1998; Kumsta et al., 2008; van Rossum et al., 2006, 2003). Further, homozygous carriers of the Bcl-1 SNP(G allele) have more traumatic memories 6 months after intensive care unit care than heterozygous or non-carriers of the SNP (Hauer et al., 2011). Similarly SNPs in the steroid receptor chaperone FK506 binding protein 5 (FKBP5) increase glucocorticoid receptor sensitivity leading to lower basal cortisol levels and increased risk for PTSD following traumatic experience (Binder et al., 2008; Klengel et al., 2013; Wüst et al., 2004; Yehuda et al., 2009). Further, stress-level doses of hydrocortisone reduce PTSD symptomtology in patients following traumatic experience (Aerni et al., 2004; Delahanty et al., 2013; Schelling et al., 2003, 2001, 1999; Weis et al., 2006; Zohar et al., 2011). In healthy controls, stress enhances the consolidation of emotionally salient information (Andreano and Cahill, 2006; Buchanan and Lovallo, 2001), while also impairing memory retrieval (de Quervain et al., 2009, 2007). Therefore, hydrocortisone has been proposed to impair the retrieval of traumatic memories resulting in decreased PTSD symptoms following a traumatic experience (Hauer et al., 2013). Glucocorticoids also reduce local oxygen metabolism selectively in the amygdala and parahippocampal gyrus immediately following stress-level hydrocortisone exposure (Lovallo et al., 2010). Collectively, these studies suggest that stress-level doses of hydrocortisone may prove useful, particularly in patients with polymorphisms of the GR gene or genes affecting glucocorticoid sensitivity (Hauer et al., 2013; Schelling et al., 2013). In contrast to the low levels of cortisol generally associated with PTSD, patients with major depression typically display hypercortisolemia (Yehuda et al., 2004). Hypometabolism (decreased uptake of fluorine deoxyglucose) as well as hypermetabolism (increased regional cerebral blood flow) have been reported in the subgenual anterior cingulate cortex (Brodmann area 25) of patients with major depression (Drevets, 2000; Mayberg et al., 2005). Additionally, hydrocortisone decreases subgenual cingulate oxygen utilization evoked by sad stimuli, suggesting that this region is a key target for glucocorticoid-mediated effects on emotional processes (Sudheimer et al., 2013). Thus, the aggregate data suggest that glucocorticoids have pronounced effects on local metabolic activity within forebrain limbic circuits and alterations in glucocorticoid signaling within these areas may play a prominent role in stress-related neuropsychiatric disorders.
6. Stress, Energetics, and Adaptation
Responses to stress occur at the level of the individual, requiring both appraisal systems (limbic forebrain) and effector sites (hypothalamus and hindbrain) in the brain, as well as peripheral physiological systems. Consequently, regulation of the HPA axis is a distributed process integrated by multiple sites with considerable overlap and redundancy to permit compensation. For instance, both the plPFC and the ventral hippocampus provide inhibition of the HPA axis via convergent projections onto PVN-projecting neurons in the aBST (Radley and Sawchenko, 2011). Neuroendocrine responses to stress are initiated by the brain and result in the secretion of glucocorticoids to promote energy mobilization for current or anticipated needs. In turn, glucocorticoids act as a signal that influences cellular function, gene transcription, circuit activity, and, ultimately, behavior. Thus, responses to both acute and chronic stress promote context-specific adaptation based on the real or perceived needs of the individual. Although responses to chronic stress may promote adaptation, there is a cost of adaptation. Consequently, the adaptive cost may be deleterious for the organism when stress effector systems are repeatedly activated to meet the energetic demands of severe or prolonged stress. The physiological and behavioral consequences of driving energetic systems may encompass both stress resilience, as well the transition to pathology. Thus, the stress response leads to a redistribution of energy resources to meet emergent or anticipated needs driven by a glucocorticoid signal that influences cellular function, neurocircuits, and behavior. This signal pushes physiological systems to adapt until the adaptive cost becomes greater than the adaptive capacity of the individual. In this framework, the adaptive capacity can be defined as the degree to which the organism can deploy energy mobilizing systems (e.g. HPA axis) to successfully meet current or anticipated needs.
The relationship between stress and energetics can be summarized by examining the relationship between systems performance, environmental demand, and energy input (Figure 1). The performance of the individual is largely dependent on energetic status, environmental demand (i.e. context), adaptive capacity, and adaptive cost. Glucocorticoids regulate the energetic status of the individual and are a crucial determinant of both adaptive capacity and cost. In turn, the adaptive capacity and cost directly influence the performance of the individual across varying ranges of environmental demand. The actions of glucocorticoids have been proposed to follow an inverted U-shape, such that high or low levels of glucocorticoids can impair systems performance (de Kloet et al., 1999; Herman, 2013). This is especially true for processes dependent on the hippocampus (Joëls, 2006). For example, a high dose of corticosterone or adrenalectomy increases behavioral reactivity to novel versus familiar objects, whereas a low dose of corticosterone normalizes this behavior (Oitzl et al., 1994). Glucocorticoid secretion must also be context appropriate. Hypersecreting glucocorticoids under conditions of low demand can be detrimental, but so can hyposecretion in the face of high demand. Therefore, the same concentration of glucocorticoids could be considered deleterious or beneficial depending on the energetic status of the individual. For instance, the same dose of exogenous corticosterone improves performance in a water maze task when animals are trained in warm water, but not cold water (Sandi et al., 1997). Thus, an optimal context-specific amount of glucocorticoid secretion is necessary for maintaining optimal performance (de Kloet et al., 1999; Herman, 2013; Joëls, 2006).
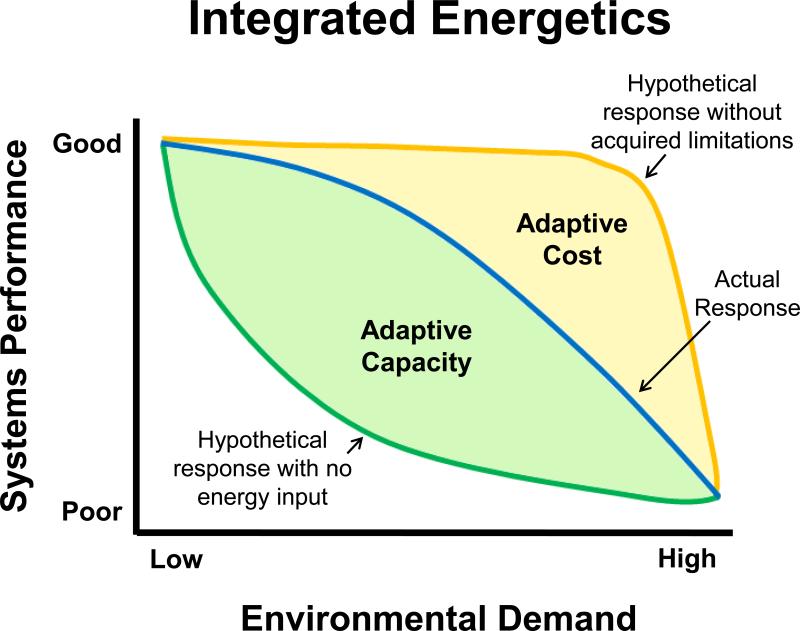
Stress, energetics, and adaptation. The relationship between stress and energetic can be explained visually by examining the relationship between systems performance and environmental demand. Under conditions of low environmental demand, high levels of systems performance can be achieved without an energetic cost to the individual. With increasing demand, maintaining systems performance requires energetic input to generate an adaptive capacity (indicated by green shaded area), which is opposed by the adaptive cost (yellow shaded area). Without the acquired limitations that generate the adaptive cost, organismal performance could hypothetically be maintained at high levels across a wide range of environmental demand (depicted by the yellow line). Conversely, the absence of energy input would lead to poor performance even under conditions of moderate environmental pressure (depicted by the green line). The actual response (depicted by the blue line) falls between these two theoretical extremes and is determined for each individual as a function of their adaptive capacity and adaptive cost. With optimal energy redistribution and appropriate appraisal of context, the organism is able to maintain systems performance across a wide range of demand. As the adaptive cost of the organism grows, greater energy input will be required to generate systems performance over a more narrow range of demand. Ultimately, the adaptive cost may be greater than the adaptive capacity, leading to a breaking point where systems performance is compromised and risk factors for pathology emerge.
Energy regulatory systems must work in concert to meet the needs of the individual and use overlapping mechanisms to control and fine-tune energy reallocation (multiple feedback sites and pathways). As a result, the individual is able to maintain adaptive capacity across a broad range of environmental demand. For example, the behavioral and physiological changes accompanying responses to chronic stress are typically considered maladaptive. However, if these changes are interpreted based on the environmental context of the individual experiencing chronic stress, many responses could be considered adaptive for organismal survival. Chronic stress can be a time of high energetic need and glucocorticoids activate glycogen metabolism and glucose synthesis. Glucocorticoids also inhibit energy utilization by systems that are not necessary for immediate survival, including growth, immune, and reproductive processes (Munck et al., 1984). These steps in energy redistribution and reallocation are essential for increasing the adaptive capacity of the individual. In terms of behavior, the effects of chronic glucocorticoids on measures of anxiety, depression, and behavioral flexibility may be deleterious in some environmental contexts; however, in the face of chronic stress, diminished behavioral output could be considered beneficial. For instance, the neophobia and behavioral withdrawal assessed by many tests of anxiety- and depression-like behavior may serve to minimize risk exposure and energy expenditure in contexts of prolonged challenge. Further, a switch to habitual strategies under chronic stress may lead to greater efficiency in predictable tasks by allowing the individual to respond instinctually and bypass the appraisal process (Dias-Ferreira et al., 2009; Schwabe et al., 2013). Thus, limiting risky or energy-demanding behaviors and energy-intensive physiologic processes (e.g. growth, reproduction, immune responses, etc) represent major components of the organismal adaptive capacity. However, when these responses are inappropriate to environmental demand or are sustained of prolonged time periods, the individual generates an adaptive cost that can decrease overall performance.
Glucocorticoid responses promote survival during periods of environmental demand but, over longer time frames, glucocorticoid secretion can impair behavioral and physiological health. There is a certain cost of adaptation that is likely dependent on how the individual's genetic background and life history interact with their appraisal of environmental demand. Thus, inappropriate regulatory balance in stress systems may comprise a risk factor for pathology (increasing adaptive cost). Adaptive cost may be further increased by energy depletion due to overdrive of energetic systems, physiological energy ‘sinks’ (e.g. infection, inflammation, metabolic disease, etc), design faults (inefficient energy redistribution systems, genetic polymorphisms), or poor decision making (misinterpretation of energetic need). As mentioned previously, habitual strategies are beneficial under chronic stress when the task and environment remain the same. However, if the environment changes to a context that requires appraisal and flexible strategies, individuals that have adopted habitual strategies may be at a disadvantage. For example, a recent study found that chronically stressed humans are unable to appropriately appraise outcome values. Further, this behavior is associated with a shift from activation of associative areas to increased activation of sensorimotor areas, mPFC and caudate atrophy, and increased putamen volume (Soares et al., 2013). In this case, misappraisal and energy depletion likely increase the adaptive cost. Depression and PTSD are stress-related disorders characterized by cognitive, affective, and physiological responses that are inappropriate to environmental context. Thus, psychopathologies may emerge when adaptive costs outweigh adaptive capacity, leading to a ‘break point’ in the process of adaptation.
6. Conclusions
A substantial and evolving body of work indicates that glucocorticoids are vital for central stress integration. Accordingly, we propose that the actions of glucocorticoids on neural systems from synapses to circuits generate the energetic resources to promote behavioral stress adaptation. These actions also illustrate the importance of appropriate context appraisal for successful adaptive responses. Misappraisal and/or inadequate adaptive capacity increase the risk for stress-related disorders, including depression and PTSD. Future studies addressing the mechanisms by which glucocorticoid signaling is integrated across multiple brain regions and peripheral sites will improve our understanding of the etiology of stress-related illness. In order to meet this challenge we must consider the sensitivity of current models and endpoints for determining the transition from adaptive to deleterious stress responses, as well as ways to increase translation of these findings into improved clinical outcomes.
Acknowledgements
B. Myers is supported by American Heart Association Grant 13POST17070152. J.M. McKlveen is supported by National Institutes of Health (NIH) Grant MH097430. J.P. Herman is supported by NIH Grants MH090574, MH069860, and MH049698.
References
- Abrari K, Rashidy-Pour A, Semnanian S, Fathollahi Y, Jadid M. Post-training administration of corticosterone enhances consolidation of contextual fear memory and hippocampal long-term potentiation in rats. Neurobiol. Learn. Mem. 2009;91:260–5. [Abstract] [Google Scholar]
- Adamec RE. Amygdala kindling and anxiety in the rat. Neuroreport. 1990;1:255–8. [Abstract] [Google Scholar]
- Aerni A, Traber R, Hock C, Roozendaal B, Schelling G, Papassotiropoulos A, Nitsch RM, Schnyder U, de Quervain DJ-F. Low-dose cortisol for symptoms of posttraumatic stress disorder. Am. J. Psychiatry. 2004;161:1488–90. [Abstract] [Google Scholar]
- Akana SF, Chu A, Soriano L, Dallman MF. Corticosterone exerts site-specific and state-dependent effects in prefrontal cortex and amygdala on regulation of adrenocorticotropic hormone, insulin and fat depots. J. Neuroendocrinol. 2001;13:625–37. [Abstract] [Google Scholar]
- Akirav I. Cannabinoids and glucocorticoids modulate emotional memory after stress. Neurosci. Biobehav. Rev. 2013 [Abstract] [Google Scholar]
- Alfarez DN, Joëls M, Krugers HJ. Chronic unpredictable stress impairs long-term potentiation in rat hippocampal CA1 area and dentate gyrus in vitro. Eur. J. Neurosci. 2003;17:1928–34. [Abstract] [Google Scholar]
- Amorapanth P, LeDoux JE, Nader K. Different lateral amygdala outputs mediate reactions and actions elicited by a fear-arousing stimulus. Nat. Neurosci. 2000;3:74–9. [Abstract] [Google Scholar]
- Andreano JM, Cahill L. Glucocorticoid release and memory consolidation in men and women. Psychol. Sci. 2006;17:466–70. [Abstract] [Google Scholar]
- Arnett MG, Kolber BJ, Boyle MP, Muglia LJ. Behavioral insights from mouse models of forebrain--and amygdala-specific glucocorticoid receptor genetic disruption. Mol. Cell. Endocrinol. 2011;336:2–5. [Europe PMC free article] [Abstract] [Google Scholar]
- Atsak P, Hauer D, Campolongo P, Schelling G, McGaugh JL, Roozendaal B. Glucocorticoids interact with the hippocampal endocannabinoid system in impairing retrieval of contextual fear memory. Proc. Natl. Acad. Sci. U. S. A. 2012a;109:3504–9. [Europe PMC free article] [Abstract] [Google Scholar]
- Atsak P, Roozendaal B, Campolongo P. Role of the endocannabinoid system in regulating glucocorticoid effects on memory for emotional experiences. Neuroscience. 2012b;204:104–16. [Abstract] [Google Scholar]
- Bagley J, Moghaddam B. Temporal dynamics of glutamate efflux in the prefrontal cortex and in the hippocampus following repeated stress: effects of pretreatment with saline or diazepam. Neuroscience. 1997;77:65–73. [Abstract] [Google Scholar]
- Baker KB, Kim JJ. Effects of stress and hippocampal NMDA receptor antagonism on recognition memory in rats. Learn. Mem. 2002;9:58–65. [Europe PMC free article] [Abstract] [Google Scholar]
- Bale TL. Sensitivity to stress: dysregulation of CRF pathways and disease development. Horm. Behav. 2005;48:1–10. [Abstract] [Google Scholar]
- Bale TL, Chen A. Minireview: CRF and Wylie Vale: a story of 41 amino acids and a Texan with grit. Endocrinology. 2012;153:2556–61. [Europe PMC free article] [Abstract] [Google Scholar]
- Bale TL, Vale WW. CRF and CRF receptors: role in stress responsivity and other behaviors. Annu. Rev. Pharmacol. Toxicol. 2004;44:525–57. [Abstract] [Google Scholar]
- Bangasser DA, Santollo J, Shors TJ. The bed nucleus of the stria terminalis is critically involved in enhancing associative learning after stressful experience. Behav. Neurosci. 2005;119:1459–66. [Europe PMC free article] [Abstract] [Google Scholar]
- Barik J, Marti F, Morel C, Fernandez SP, Lanteri C, Godeheu G, Tassin J-P, Mombereau C, Faure P, Tronche F. Chronic stress triggers social aversion via glucocorticoid receptor in dopaminoceptive neurons. Science. 2013;339:332–5. [Abstract] [Google Scholar]
- Barsegyan A, Mackenzie SM, Kurose BD, McGaugh JL, Roozendaal B. Glucocorticoids in the prefrontal cortex enhance memory consolidation and impair working memory by a common neural mechanism. Proc. Natl. Acad. Sci. U. S. A. 2010;107:16655–60. [Europe PMC free article] [Abstract] [Google Scholar]
- Beaulieu S, Di Paolo T, Barden N. Control of ACTH secretion by the central nucleus of the amygdala: implication of the serotoninergic system and its relevance to the glucocorticoid delayed negative feedback mechanism. Neuroendocrinology. 1986;44:247–254. [Abstract] [Google Scholar]
- Beaulieu S, Di Paolo T, Cote J, Barden N. Participation of the central amygdaloid nucleus in the response of adrenocorticotropin secretion to immobilization stress: opposing roles of the noradrenergic and dopaminergic systems. Neuroendocrinology. 1987;45:37–46. [Abstract] [Google Scholar]
- Bhargava A, Mathias RS, McCormick JA, Dallman MF, Pearce D. Glucocorticoids prolong Ca(2+) transients in hippocampal-derived H19-7 neurons by repressing the plasma membrane Ca(2+)-ATPase-1. Mol. Endocrinol. 2002;16:1629–37. [Abstract] [Google Scholar]
- Bhatnagar S, Vining C, Denski K. Regulation of chronic stress-induced changes in hypothalamic-pituitary-adrenal activity by the basolateral amygdala. Ann N Y Acad Sci. 2004;1032:315–319. [Abstract] [Google Scholar]
- Bienkowski MS, Rinaman L. Common and distinct neural inputs to the medial central nucleus of the amygdala and anterior ventrolateral bed nucleus of stria terminalis in rats. Brain Struct Funct. 2012 [Europe PMC free article] [Abstract] [Google Scholar]
- Binder EB, Bradley RG, Liu W, Epstein MP, Deveau TC, Mercer KB, Tang Y, Gillespie CF, Heim CM, Nemeroff CB, Schwartz AC, Cubells JF, Ressler KJ. Association of FKBP5 polymorphisms and childhood abuse with risk of posttraumatic stress disorder symptoms in adults. JAMA. 2008;299:1291–305. [Europe PMC free article] [Abstract] [Google Scholar]
- Birrell JM, Brown VJ. Medial frontal cortex mediates perceptual attentional set shifting in the rat. J. Neurosci. 2000;20:4320–4. [Europe PMC free article] [Abstract] [Google Scholar]
- Bondi CO, Rodriguez G, Gould GG, Frazer A, Morilak DA. Chronic unpredictable stress induces a cognitive deficit and anxiety-like behavior in rats that is prevented by chronic antidepressant drug treatment. Neuropsychopharmacology. 2008;33:320–31. [Abstract] [Google Scholar]
- Boudaba C, Szabo K, Tasker JG. Physiological mapping of local inhibitory inputs to the hypothalamic paraventricular nucleus. J Neurosci. 1996;16:7151–7160. [Europe PMC free article] [Abstract] [Google Scholar]
- Boyle MP, Brewer JA, Funatsu M, Wozniak DF, Tsien JZ, Izumi Y, Muglia LJ. Acquired deficit of forebrain glucocorticoid receptor produces depression-like changes in adrenal axis regulation and behavior. Proc Natl Acad Sci U S A. 2005;102:473–478. [Europe PMC free article] [Abstract] [Google Scholar]
- Bruhn TO, Plotsky PM, Vale WW. Effect of paraventricular lesions on corticotropin-releasing factor (CRF)-like immunoreactivity in the stalk-median eminence: studies on the adrenocorticotropin response to ether stress and exogenous CRF. Endocrinology. 1984;114:57–62. [Abstract] [Google Scholar]
- Buchanan TW, Lovallo WR. Enhanced memory for emotional material following stress-level cortisol treatment in humans. Psychoneuroendocrinology. 2001;26:307–17. [Abstract] [Google Scholar]
- Butts KA, Weinberg J, Young AH, Phillips AG. Glucocorticoid receptors in the prefrontal cortex regulate stress-evoked dopamine efflux and aspects of executive function. Proc. Natl. Acad. Sci. U. S. A. 2011;108:18459–64. [Europe PMC free article] [Abstract] [Google Scholar]
- Campolongo P, Roozendaal B, Trezza V, Hauer D, Schelling G, McGaugh JL, Cuomo V. Endocannabinoids in the rat basolateral amygdala enhance memory consolidation and enable glucocorticoid modulation of memory. Proc. Natl. Acad. Sci. U. S. A. 2009;106:4888–93. [Europe PMC free article] [Abstract] [Google Scholar]
- Cannon WB. The wisdom of the body. W W Norton & Co; New York, NY.: 1932. [Google Scholar]
- Cerqueira JJ, Pêgo JM, Taipa R, Bessa JM, Almeida OFX, Sousa N. Morphological correlates of corticosteroid-induced changes in prefrontal cortex-dependent behaviors. J. Neurosci. 2005;25:7792–800. [Europe PMC free article] [Abstract] [Google Scholar]
- Cerqueira JJ, Taipa R, Uylings HBM, Almeida OFX, Sousa N. Specific configuration of dendritic degeneration in pyramidal neurons of the medial prefrontal cortex induced by differing corticosteroid regimens. Cereb. Cortex. 2007;17:1998–2006. [Abstract] [Google Scholar]
- Chameau P, Qin Y, Spijker S, Smit AB, Smit G, Joëls M. Glucocorticoids specifically enhance L-type calcium current amplitude and affect calcium channel subunit expression in the mouse hippocampus. J. Neurophysiol. 2007;97:5–14. [Abstract] [Google Scholar]
- Charney DS, Deutch A. A functional neuroanatomy of anxiety and fear: implications for the pathophysiology and treatment of anxiety disorders. Crit. Rev. Neurobiol. 1996;10:419–46. [Abstract] [Google Scholar]
- Chen DY, Bambah-Mukku D, Pollonini G, Alberini CM. Glucocorticoid receptors recruit the CaMKIIα-BDNF-CREB pathways to mediate memory consolidation. Nat. Neurosci. 2012;15:1707–14. [Europe PMC free article] [Abstract] [Google Scholar]
- Choi DC, Evanson NK, Furay AR, Ulrich-Lai YM, Ostrander MM, Herman JP. The anteroventral bed nucleus of the stria terminalis differentially regulates hypothalamic-pituitary-adrenocortical axis responses to acute and chronic stress. Endocrinology. 2008a;149:818–826. [Europe PMC free article] [Abstract] [Google Scholar]
- Choi DC, Furay AR, Evanson NK, Ostrander MM, Ulrich-Lai YM, Herman JP. Bed nucleus of the stria terminalis subregions differentially regulate hypothalamic-pituitary-adrenal axis activity: implications for the integration of limbic inputs. J Neurosci. 2007;27:2025–2034. [Europe PMC free article] [Abstract] [Google Scholar]
- Choi DC, Furay AR, Evanson NK, Ulrich-Lai YM, Nguyen MM, Ostrander MM, Herman JP. The role of the posterior medial bed nucleus of the stria terminalis in modulating hypothalamic-pituitary-adrenocortical axis responsiveness to acute and chronic stress. Psychoneuroendocrinology. 2008b;33:659–669. [Europe PMC free article] [Abstract] [Google Scholar]
- Ciocchi S, Herry C, Grenier F, Wolff SBE, Letzkus JJ, Vlachos I, Ehrlich I, Sprengel R, Deisseroth K, Stadler MB, Müller C, Lüthi A. Encoding of conditioned fear in central amygdala inhibitory circuits. Nature. 2010;468:277–82. [Abstract] [Google Scholar]
- Clark JA, Flick RB, Pai L-Y, Szalayova I, Key S, Conley RK, Deutch AY, Hutson PH, Mezey E. Glucocorticoid modulation of tryptophan hydroxylase-2 protein in raphe nuclei and 5-hydroxytryptophan concentrations in frontal cortex of C57/Bl6 mice. Mol. Psychiatry. 2008;13:498–506. [Europe PMC free article] [Abstract] [Google Scholar]
- Clark JA, Pai L-Y, Flick RB, Rohrer SP. Differential hormonal regulation of tryptophan hydroxylase-2 mRNA in the murine dorsal raphe nucleus. Biol. Psychiatry. 2005;57:943–6. [Abstract] [Google Scholar]
- Coderre L, Srivastava AK, Chiasson JL. Role of glucocorticoid in the regulation of glycogen metabolism in skeletal muscle. Am J Physiol. 1991;260:E927–32. [Abstract] [Google Scholar]
- Conrad CD, Galea LA, Kuroda Y, McEwen BS. Chronic stress impairs rat spatial memory on the Y maze, and this effect is blocked by tianeptine pretreatment. Behav. Neurosci. 1996;110:1321–34. [Abstract] [Google Scholar]
- Conrad CD, LeDoux JE, Magariños AM, McEwen BS. Repeated restraint stress facilitates fear conditioning independently of causing hippocampal CA3 dendritic atrophy. Behav. Neurosci. 1999;113:902–13. [Abstract] [Google Scholar]
- Conway-Campbell BL, Sarabdjitsingh RA, McKenna MA, Pooley JR, Kershaw YM, Meijer OC, De Kloet ER, Lightman SL. Glucocorticoid ultradian rhythmicity directs cyclical gene pulsing of the clock gene period 1 in rat hippocampus. J. Neuroendocrinol. 2010;22:1093–100. [Europe PMC free article] [Abstract] [Google Scholar]
- Cook SC, Wellman CL. Chronic stress alters dendritic morphology in rat medial prefrontal cortex. J. Neurobiol. 2004;60:236–48. [Abstract] [Google Scholar]
- Cressman VL, Balaban J, Steinfeld S, Shemyakin A, Graham P, Parisot N, Moore H. Prefrontal cortical inputs to the basal amygdala undergo pruning during late adolescence in the rat. J. Comp. Neurol. 2010;518:2693–709. [Europe PMC free article] [Abstract] [Google Scholar]
- Cullinan WE, Helmreich DL, Watson SJ. Fos expression in forebrain afferents to the hypothalamic paraventricular nucleus following swim stress. J Comp Neurol. 1996;368:88–99. [Abstract] [Google Scholar]
- Cullinan WE, Herman JP, Battaglia DF, Akil H, Watson SJ. Pattern and time course of immediate early gene expression in rat brain following acute stress. Neuroscience. 1995;64:477–505. [Abstract] [Google Scholar]
- Cullinan WE, Herman JP, Watson SJ. Ventral subicular interaction with the hypothalamic paraventricular nucleus: evidence for a relay in the bed nucleus of the stria terminalis. J Comp Neurol. 1993;332:1–20. [Abstract] [Google Scholar]
- Cunningham ET, Jr., Bohn MC, Sawchenko PE. Organization of adrenergic inputs to the paraventricular and supraoptic nuclei of the hypothalamus in the rat. J Comp Neurol. 1990;292:651–667. [Abstract] [Google Scholar]
- Cunningham ET, Jr., Sawchenko PE. Anatomical specificity of noradrenergic inputs to the paraventricular and supraoptic nuclei of the rat hypothalamus. J Comp Neurol. 1988;274:60–76. [Abstract] [Google Scholar]
- Dallman MF, Akana SF, Cascio CS, Darlington DN, Jacobson L, Levin N. Regulation of ACTH secretion: variations on a theme of B. Recent Prog Horm Res. 1987;43:113–173. [Abstract] [Google Scholar]
- Dallman MF, Jones MT. Corticosteroid feedback control of ACTH secretion: effect of stress-induced corticosterone ssecretion on subsequent stress responses in the rat. Endocrinology. 1973;92:1367–1375. [Abstract] [Google Scholar]
- Dallman MF, Pecoraro NC, La Fleur SE, Warne JP, Ginsberg AB, Akana SF, Laugero KC, Houshyar H, Strack AM, Bhatnagar S, Bell ME. Glucocorticoids, chronic stress, and obesity. Prog Brain Res. 2006;153:75–105. [Abstract] [Google Scholar]
- Davis M. The role of the amygdala in fear and anxiety. Annu. Rev. Neurosci. 1992;15:353–75. [Abstract] [Google Scholar]
- Davis M. Neurobiology of fear responses: the role of the amygdala. J. Neuropsychiatry Clin. Neurosci. 1997;9:382–402. [Abstract] [Google Scholar]
- Davis M, Walker DL, Miles L, Grillon C. Phasic vs sustained fear in rats and humans: role of the extended amygdala in fear vs anxiety. Neuropsychopharmacology. 2010;35:105–35. [Europe PMC free article] [Abstract] [Google Scholar]
- Dayas CV, Buller KM, Day TA. Neuroendocrine responses to an emotional stressor: evidence for involvement of the medial but not the central amygdala. Eur J Neurosci. 1999;11:2312–2322. [Abstract] [Google Scholar]
- De Kloet ER. Functional profile of the binary brain corticosteroid receptor system: Mediating, multitasking, coordinating, integrating. Eur. J. Pharmacol. 2013a [Abstract] [Google Scholar]
- De Kloet ER. Lifetime achievement from a brain-adrenal perspective: On the CRF-urocortin-glucocorticoid balance. J. Chem. Neuroanat. 2013b [Abstract] [Google Scholar]
- De Kloet ER, Joels M, Holsboer F. Stress and the brain: from adaptation to disease. Nat Rev Neurosci. 2005;6:463–475. [Abstract] [Google Scholar]
- De Kloet ER, Oitzl MS, Joëls M. Stress and cognition: are corticosteroids good or bad guys? Trends Neurosci. 1999;22:422–6. [Abstract] [Google Scholar]
- De Kloet ER, Reul JM. Feedback action and tonic influence of corticosteroids on brain function: a concept arising from the heterogeneity of brain receptor systems. Psychoneuroendocrinology. 1987;12:83–105. [Abstract] [Google Scholar]
- De Kloet ER, Sarabdjitsingh RA. Everything has rhythm: focus on glucocorticoid pulsatility. Endocrinology. 2008;149:3241–3243. [Abstract] [Google Scholar]
- De Kloet ER, Vreugdenhil E, Oitzl MS, Joels M. Brain corticosteroid receptor balance in health and disease. Endocr Rev. 1998;19:269–301. [Abstract] [Google Scholar]
- De Quervain DJ, Roozendaal B, McGaugh JL. Stress and glucocorticoids impair retrieval of long-term spatial memory. Nature. 1998;394:787–90. [Abstract] [Google Scholar]
- De Quervain DJ-F, Aerni A, Roozendaal B. Preventive effect of beta-adrenoceptor blockade on glucocorticoid-induced memory retrieval deficits. Am. J. Psychiatry. 2007;164:967–9. [Abstract] [Google Scholar]
- De Quervain DJ-F, Aerni A, Schelling G, Roozendaal B. Glucocorticoids and the regulation of memory in health and disease. Front. Neuroendocrinol. 2009;30:358–70. [Abstract] [Google Scholar]
- De Wied D, Bouman PR, Smelik PG. The influence of a hypothalamus extract and of pitressin on the release of ACTH from the pituitary gland. Acta Physiol. Pharmacol. Neerl. 1957;5:489–90. [Abstract] [Google Scholar]
- Delahanty DL, Gabert-Quillen C, Ostrowski SA, Nugent NR, Fischer B, Morris A, Pitman RK, Bon J, Fallon W. The efficacy of initial hydrocortisone administration at preventing posttraumatic distress in adult trauma patients: a randomized trial. CNS Spectr. 2013;18:103–11. [Europe PMC free article] [Abstract] [Google Scholar]
- Derijk RH, van Leeuwen N, Klok MD, Zitman FG. Corticosteroid receptor-gene variants: modulators of the stress-response and implications for mental health. Eur. J. Pharmacol. 2008;585:492–501. [Abstract] [Google Scholar]
- Diamond DM, Fleshner M, Rose GM. Psychological stress repeatedly blocks hippocampal primed burst potentiation in behaving rats. Behav. Brain Res. 1994;62:1–9. [Abstract] [Google Scholar]
- Diamond DM, Park CR, Heman KL, Rose GM. Exposing rats to a predator impairs spatial working memory in the radial arm water maze. Hippocampus. 1999;9:542–52. [Abstract] [Google Scholar]
- Diamond DM, Rose GM. Stress impairs LTP and hippocampal-dependent memory. Ann. N. Y. Acad. Sci. 1994;746:411–4. [Abstract] [Google Scholar]
- Dias-Ferreira E, Sousa JC, Melo I, Morgado P, Mesquita AR, Cerqueira JJ, Costa RM, Sousa N. Chronic stress causes frontostriatal reorganization and affects decision-making. Science. 2009;325:621–5. [Abstract] [Google Scholar]
- Diorio D, Viau V, Meaney MJ. The role of the medial prefrontal cortex (cingulate gyrus) in the regulation of hypothalamic-pituitary-adrenal responses to stress. J Neurosci. 1993;13:3839–3847. [Europe PMC free article] [Abstract] [Google Scholar]
- Donley MP, Schulkin J, Rosen JB. Glucocorticoid receptor antagonism in the basolateral amygdala and ventral hippocampus interferes with long-term memory of contextual fear. Behav. Brain Res. 2005;164:197–205. [Abstract] [Google Scholar]
- Drevets WC. Neuroimaging studies of mood disorders. Biol. Psychiatry. 2000;48:813–29. [Abstract] [Google Scholar]
- Duvarci S, Paré D. Glucocorticoids enhance the excitability of principal basolateral amygdala neurons. J. Neurosci. 2007;27:4482–91. [Europe PMC free article] [Abstract] [Google Scholar]
- Evanson NK, Tasker JG, Hill MN, Hillard CJ, Herman JP. Fast feedback inhibition of the HPA axis by glucocorticoids is mediated by endocannabinoid signaling. Endocrinology. 2010;151:4811–4819. [Europe PMC free article] [Abstract] [Google Scholar]
- Exton JH. Regulation of gluconeogenesis by glucocorticoids. Monogr Endocrinol. 1979;12:535–546. [Abstract] [Google Scholar]
- Feldman S, Weidenfeld J. Glucocorticoid receptor antagonists in the hippocampus modify the negative feedback following neural stimuli. Brain Res. 1999;821:33–7. [Abstract] [Google Scholar]
- Ferguson D, Sapolsky R. Overexpression of mineralocorticoid and transdominant glucocorticoid receptor blocks the impairing effects of glucocorticoids on memory. Hippocampus. 2008;18:1103–11. [Abstract] [Google Scholar]
- Ferry B, McGaugh JL. Role of amygdala norepinephrine in mediating stress hormone regulation of memory storage. Acta Pharmacol. Sin. 2000;21:481–93. [Abstract] [Google Scholar]
- Fitzsimons CP, van Hooijdonk LWA, Schouten M, Zalachoras I, Brinks V, Zheng T, Schouten TG, Saaltink DJ, Dijkmans T, Steindler DA, Verhaagen J, Verbeek FJ, Lucassen PJ, de Kloet ER, Meijer OC, Karst H, Joels M, Oitzl MS, Vreugdenhil E. Knockdown of the glucocorticoid receptor alters functional integration of newborn neurons in the adult hippocampus and impairs fear-motivated behavior. Mol. Psychiatry. 2013;18:993–1005. [Abstract] [Google Scholar]
- Fuxe K, Cintra A, Agnati LF, Harfstrand A, Wikstrom AC, Okret S, Zoli M, Miller LS, Greene JL, Gustafsson JA. Studies on the cellular localization and distribution of glucocorticoid receptor and estrogen receptor immunoreactivity in the central nervous system of the rat and their relationship to the monoaminergic and peptidergic neurons of the brain. J Steroid Biochem. 1987;27:159–170. [Abstract] [Google Scholar]
- Gabbott PLA, Warner TA, Jays PRL, Salway P, Busby SJ. Prefrontal cortex in the rat: projections to subcortical autonomic, motor, and limbic centers. J. Comp. Neurol. 2005;492:145–77. [Abstract] [Google Scholar]
- Ghosal S, Myers B, Herman JP. Role of central glucagon-like peptide-1 in stress regulation. Physiol Behav. 2013 [Europe PMC free article] [Abstract] [Google Scholar]
- Gibbs DM, Vale W. Presence of corticotropin releasing factor-like immunoreactivity in hypophysial portal blood. Endocrinology. 1982;111:1418–1420. [Abstract] [Google Scholar]
- Gilabert-Juan J, Castillo-Gomez E, Guirado R, Moltó MD, Nacher J. Chronic stress alters inhibitory networks in the medial prefrontal cortex of adult mice. Brain Struct. Funct. 2012 [Abstract] [Google Scholar]
- Goldwater DS, Pavlides C, Hunter RG, Bloss EB, Hof PR, McEwen BS, Morrison JH. Structural and functional alterations to rat medial prefrontal cortex following chronic restraint stress and recovery. Neuroscience. 2009;164:798–808. [Europe PMC free article] [Abstract] [Google Scholar]
- Gourley SL, Kedves AT, Olausson P, Taylor JR. A history of corticosterone exposure regulates fear extinction and cortical NR2B, GluR2/3, and BDNF. Neuropsychopharmacology. 2009;34:707–16. [Europe PMC free article] [Abstract] [Google Scholar]
- Groc L, Choquet D, Chaouloff F. The stress hormone corticosterone conditions AMPAR surface trafficking and synaptic potentiation. Nat. Neurosci. 2008;11:868–70. [Abstract] [Google Scholar]
- Gutièrrez-Mecinas M, Trollope AF, Collins A, Morfett H, Hesketh SA, Kersanté F, Reul JMHM. Long-lasting behavioral responses to stress involve a direct interaction of glucocorticoid receptors with ERK1/2-MSK1-Elk-1 signaling. Proc. Natl. Acad. Sci. U. S. A. 2011;108:13806–11. [Europe PMC free article] [Abstract] [Google Scholar]
- Haam J, Halmos K, Muglia L, Tasker J. Rapid synaptic modulation of hypothalamic neurons by glucocorticoids requires the glucocorticoid receptor. Soc. Neurosci. 2010 Abstr. 389.1. [Google Scholar]
- Harfstrand A. Brain neuropeptide Y mechanisms. Basic aspects and involvement in cardiovascular and neuroendocrine regulation. Acta Physiol Scand Suppl. 1987;565:1–83. [Abstract] [Google Scholar]
- Hascup ER, Hascup KN, Stephens M, Pomerleau F, Huettl P, Gratton A, Gerhardt GA. Rapid microelectrode measurements and the origin and regulation of extracellular glutamate in rat prefrontal cortex. J. Neurochem. 2010;115:1608–20. [Europe PMC free article] [Abstract] [Google Scholar]
- Haubensak W, Kunwar PS, Cai H, Ciocchi S, Wall NR, Ponnusamy R, Biag J, Dong H-W, Deisseroth K, Callaway EM, Fanselow MS, Lüthi A, Anderson DJ. Genetic dissection of an amygdala microcircuit that gates conditioned fear. Nature. 2010;468:270–6. [Europe PMC free article] [Abstract] [Google Scholar]
- Hauer D, Kaufmann I, Strewe C, Briegel I, Campolongo P, Schelling G. The role of glucocorticoids, catecholamines and endocannabinoids in the development of traumatic memories and posttraumatic stress symptoms in survivors of critical illness. Neurobiol. Learn. Mem. 2013 [Abstract] [Google Scholar]
- Hauer D, Weis F, Papassotiropoulos A, Schmoeckel M, Beiras-Fernandez A, Lieke J, Kaufmann I, Kirchhoff F, Vogeser M, Roozendaal B, Briegel J, de Quervain D, Schelling G. Relationship of a common polymorphism of the glucocorticoid receptor gene to traumatic memories and posttraumatic stress disorder in patients after intensive care therapy. Crit. Care Med. 2011;39:643–50. [Abstract] [Google Scholar]
- Heinrichs SC, Koob GF. Corticotropin-releasing factor in brain: a role in activation, arousal, and affect regulation. J. Pharmacol. Exp. Ther. 2004;311:427–40. [Abstract] [Google Scholar]
- Herman JP. Neural control of chronic stress adaptation. Front Behav Neurosci. 2013;7:61. [Europe PMC free article] [Abstract] [Google Scholar]
- Herman JP, Cullinan WE, Ziegler DR, Tasker JG. Role of the paraventricular nucleus microenvironment in stress integration. Eur J Neurosci. 2002;16:381–385. [Abstract] [Google Scholar]
- Herman JP, Dolgas CM, Carlson SL. Ventral subiculum regulates hypothalamo-pituitary-adrenocortical and behavioural responses to cognitive stressors. Neuroscience. 1998;86:449–459. [Abstract] [Google Scholar]
- Herman JP, Figueiredo H, Mueller NK, Ulrich-Lai Y, Ostrander MM, Choi DC, Cullinan WE. Central mechanisms of stress integration: hierarchical circuitry controlling hypothalamo-pituitary-adrenocortical responsiveness. Front Neuroendocr. 2003;24:151–180. [Abstract] [Google Scholar]
- Herman JP, McKlveen JM, Solomon MB, Carvalho-Netto E, Myers B. Neural regulation of the stress response: glucocorticoid feedback mechanisms. Braz J Med Biol Res. 2012;45:292–298. [Europe PMC free article] [Abstract] [Google Scholar]
- Herman JP, Mueller NK. Role of the ventral subiculum in stress integration. Behav Brain Res. 2006;174:215–224. [Abstract] [Google Scholar]
- Herman JP, Schafer MK, Young EA, Thompson R, Douglass J, Akil H, Watson SJ. Evidence for hippocampal regulation of neuroendocrine neurons of the hypothalamo-pituitary-adrenocortical axis. J Neurosci. 1989;9:3072–3082. [Europe PMC free article] [Abstract] [Google Scholar]
- Hill MN, McLaughlin RJ, Morrish AC, Viau V, Floresco SB, Hillard CJ, Gorzalka BB. Suppression of amygdalar endocannabinoid signaling by stress contributes to activation of the hypothalamic-pituitary-adrenal axis. Neuropsychopharmacology. 2009;34:2733–45. [Europe PMC free article] [Abstract] [Google Scholar]
- Hill MN, McLaughlin RJ, Pan B, Fitzgerald ML, Roberts CJ, Lee TT-Y, Karatsoreos IN, Mackie K, Viau V, Pickel VM, McEwen BS, Liu Q, Gorzalka BB, Hillard CJ. Recruitment of prefrontal cortical endocannabinoid signaling by glucocorticoids contributes to termination of the stress response. J. Neurosci. 2011;31:10506–15. [Europe PMC free article] [Abstract] [Google Scholar]
- Hubert GW, Li C, Rainnie DG, Muly EC. Effects of stress on AMPA receptor distribution and function in the basolateral amygdala. Brain Struct. Funct. 2013 [Europe PMC free article] [Abstract] [Google Scholar]
- Huizenga NA, Koper JW, De Lange P, Pols HA, Stolk RP, Burger H, Grobbee DE, Brinkmann AO, De Jong FH, Lamberts SW. A polymorphism in the glucocorticoid receptor gene may be associated with and increased sensitivity to glucocorticoids in vivo. J. Clin. Endocrinol. Metab. 1998;83:144–51. [Abstract] [Google Scholar]
- Jin X-C, Lu Y-F, Yang X-F, Ma L, Li B-M. Glucocorticoid receptors in the basolateral nucleus of amygdala are required for postreactivation reconsolidation of auditory fear memory. Eur. J. Neurosci. 2007;25:3702–12. [Abstract] [Google Scholar]
- Joëls M. Corticosteroid effects in the brain: U-shape it. Trends Pharmacol. Sci. 2006;27:244–50. [Abstract] [Google Scholar]
- Joëls M, de Kloet ER. Effects of glucocorticoids and norepinephrine on the excitability in the hippocampus. Science. 1989;245:1502–5. [Abstract] [Google Scholar]
- Joëls M, de Kloet ER. Mineralocorticoid receptor-mediated changes in membrane properties of rat CA1 pyramidal neurons in vitro. Proc. Natl. Acad. Sci. U. S. A. 1990;87:4495–8. [Europe PMC free article] [Abstract] [Google Scholar]
- Joëls M, Karst H, DeRijk R, de Kloet ER. The coming out of the brain mineralocorticoid receptor. Trends Neurosci. 2008;31:1–7. [Abstract] [Google Scholar]
- Joëls M, Karst H, Krugers HJ, Lucassen PJ. Chronic stress: implications for neuronal morphology, function and neurogenesis. Front. Neuroendocrinol. 2007;28:72–96. [Abstract] [Google Scholar]
- Joëls M, Krugers HJ, Lucassen PJ, Karst H. Corticosteroid effects on cellular physiology of limbic cells. Brain Res. 2009;1293:91–100. [Abstract] [Google Scholar]
- Joëls M, Sarabdjitsingh RA, Karst H. Unraveling the time domains of corticosteroid hormone influences on brain activity: rapid, slow, and chronic modes. Pharmacol. Rev. 2012;64:901–38. [Abstract] [Google Scholar]
- Jorgensen H, Knigge U, Kjaer A, Vadsholt T, Warberg J. Serotonergic involvement in stress-induced ACTH release. Brain Res. 1998;811:10–20. [Abstract] [Google Scholar]
- Karst H, Berger S, Erdmann G, Schütz G, Joëls M. Metaplasticity of amygdalar responses to the stress hormone corticosterone. Proc. Natl. Acad. Sci. U. S. A. 2010;107:14449–54. [Europe PMC free article] [Abstract] [Google Scholar]
- Karst H, Berger S, Turiault M, Tronche F, Schütz G, Joëls M. Mineralocorticoid receptors are indispensable for nongenomic modulation of hippocampal glutamate transmission by corticosterone. Proc. Natl. Acad. Sci. U. S. A. 2005;102:19204–7. [Europe PMC free article] [Abstract] [Google Scholar]
- Karst H, Joëls M. Corticosterone slowly enhances miniature excitatory postsynaptic current amplitude in mice CA1 hippocampal cells. J. Neurophysiol. 2005;94:3479–86. [Abstract] [Google Scholar]
- Karst H, Joëls M. Brief RU 38486 treatment normalizes the effects of chronic stress on calcium currents in rat hippocampal CA1 neurons. Neuropsychopharmacology. 2007;32:1830–9. [Abstract] [Google Scholar]
- Karst H, Nair S, Velzing E, Rumpff-van Essen L, Slagter E, Shinnick-Gallagher P, Joëls M. Glucocorticoids alter calcium conductances and calcium channel subunit expression in basolateral amygdala neurons. Eur. J. Neurosci. 2002;16:1083–9. [Abstract] [Google Scholar]
- Karst H, Wadman WJ, Joëls M. Corticosteroid receptor-dependent modulation of calcium currents in rat hippocampal CA1 neurons. Brain Res. 1994;649:234–42. [Abstract] [Google Scholar]
- Kavushansky A, Vouimba R-M, Cohen H, Richter-Levin G. Activity and plasticity in the CA1, the dentate gyrus, and the amygdala following controllable vs. uncontrollable water stress. Hippocampus. 2006;16:35–42. [Abstract] [Google Scholar]
- Khaksari M, Rashidy-Pour A, Vafaei AA. Central mineralocorticoid receptors are indispensable for corticosterone-induced impairment of memory retrieval in rats. Neuroscience. 2007;149:729–38. [Abstract] [Google Scholar]
- Kim JJ, Diamond DM. The stressed hippocampus, synaptic plasticity and lost memories. Nat. Rev. Neurosci. 2002;3:453–62. [Abstract] [Google Scholar]
- Kleen JK, Sitomer MT, Killeen PR, Conrad CD. Chronic stress impairs spatial memory and motivation for reward without disrupting motor ability and motivation to explore. Behav. Neurosci. 2006;120:842–51. [Europe PMC free article] [Abstract] [Google Scholar]
- Klengel T, Mehta D, Anacker C, Rex-Haffner M, Pruessner JC, Pariante CM, Pace TWW, Mercer KB, Mayberg HS, Bradley B, Nemeroff CB, Holsboer F, Heim CM, Ressler KJ, Rein T, Binder EB. Allele-specific FKBP5 DNA demethylation mediates gene-childhood trauma interactions. Nat. Neurosci. 2013;16:33–41. [Europe PMC free article] [Abstract] [Google Scholar]
- Kolber BJ, Boyle MP, Wieczorek L, Kelley CL, Onwuzurike CC, Nettles SA, Vogt SK, Muglia LJ. Transient early-life forebrain corticotropin-releasing hormone elevation causes long-lasting anxiogenic and despair-like changes in mice. J. Neurosci. 2010;30:2571–81. [Europe PMC free article] [Abstract] [Google Scholar]
- Kolber BJ, Roberts MS, Howell MP, Wozniak DF, Sands MS, Muglia LJ. Central amygdala glucocorticoid receptor action promotes fear-associated CRH activation and conditioning. Proc Natl Acad Sci U S A. 2008;105:12004–12009. [Europe PMC free article] [Abstract] [Google Scholar]
- Kopchia KL, Altman HJ, Commissaris RL. Effects of lesions of the central nucleus of the amygdala on anxiety-like behaviors in the rat. Pharmacol. Biochem. Behav. 1992;43:453–61. [Abstract] [Google Scholar]
- Kosofsky BE, Molliver ME. The serotoninergic innervation of cerebral cortex: different classes of axon terminals arise from dorsal and median raphe nuclei. Synapse. 1987;1:153–68. [Abstract] [Google Scholar]
- Krugers HJ, Douma BR, Andringa G, Bohus B, Korf J, Luiten PG. Exposure to chronic psychosocial stress and corticosterone in the rat: effects on spatial discrimination learning and hippocampal protein kinase Cgamma immunoreactivity. Hippocampus. 1997;7:427–36. [Abstract] [Google Scholar]
- Krugers HJ, Goltstein PM, van der Linden S, Joëls M. Blockade of glucocorticoid receptors rapidly restores hippocampal CA1 synaptic plasticity after exposure to chronic stress. Eur. J. Neurosci. 2006;23:3051–5. [Abstract] [Google Scholar]
- Kumsta R, Entringer S, Koper JW, van Rossum EFC, Hellhammer DH, Wüst S. Glucocorticoid receptor gene polymorphisms and glucocorticoid sensitivity of subdermal blood vessels and leukocytes. Biol. Psychol. 2008;79:179–84. [Abstract] [Google Scholar]
- Laryea G, Arnett MG, Wieczorek L, Muglia LJ. Site-specific modulation of brain glucocorticoid receptor and corticotropin-releasing hormone expression using lentiviral vectors. Mol. Cell. Endocrinol. 2013;371:160–5. [Europe PMC free article] [Abstract] [Google Scholar]
- LeDoux J. Rethinking the emotional brain. Neuron. 2012;73:653–76. [Europe PMC free article] [Abstract] [Google Scholar]
- LeDoux JE, Iwata J, Cicchetti P, Reis DJ. Different projections of the central amygdaloid nucleus mediate autonomic and behavioral correlates of conditioned fear. J Neurosci. 1988;8:2517–2529. [Europe PMC free article] [Abstract] [Google Scholar]
- Li C, Hazra R, Stair S, Rainnie D. Repeated shock stress facilitates basolateral amygdala synaptic plasticity through a decrease cAMP-specific phosphodiesterase type IV (PDE4) expression. Soc. Neurosci. 2011 Abstr. 191.05. [Europe PMC free article] [Abstract] [Google Scholar]
- Liebmann L, Karst H, Sidiropoulou K, van Gemert N, Meijer OC, Poirazi P, Joëls M. Differential effects of corticosterone on the slow afterhyperpolarization in the basolateral amygdala and CA1 region: possible role of calcium channel subunits. J. Neurophysiol. 2008;99:958–68. [Abstract] [Google Scholar]
- Lightman SL, Wiles CC, Atkinson HC, Henley DE, Russell GM, Leendertz JA, McKenna MA, Spiga F, Wood SA, Conway-Campbell BL. The significance of glucocorticoid pulsatility. Eur J Pharmacol. 2008;583:255–262. [Abstract] [Google Scholar]
- Liston C, Miller MM, Goldwater DS, Radley JJ, Rocher AB, Hof PR, Morrison JH, McEwen BS. Stress-induced alterations in prefrontal cortical dendritic morphology predict selective impairments in perceptual attentional set-shifting. J. Neurosci. 2006;26:7870–4. [Europe PMC free article] [Abstract] [Google Scholar]
- Lovallo WR, Robinson JL, Glahn DC, Fox PT. Acute effects of hydrocortisone on the human brain: an fMRI study. Psychoneuroendocrinology. 2010;35:15–20. [Europe PMC free article] [Abstract] [Google Scholar]
- Lowry CA. Functional subsets of serotonergic neurones: implications for control of the hypothalamic-pituitary-adrenal axis. J Neuroendocr. 2002;14:911–923. [Abstract] [Google Scholar]
- Luine V, Villegas M, Martinez C, McEwen BS. Repeated stress causes reversible impairments of spatial memory performance. Brain Res. 1994;639:167–70. [Abstract] [Google Scholar]
- Ma S, Morilak DA. Norepinephrine release in medial amygdala facilitates activation of the hypothalamic-pituitary-adrenal axis in response to acute immobilisation stress. J Neuroendocr. 2005;17:22–28. [Abstract] [Google Scholar]
- Maggio N, Segal M. Striking variations in corticosteroid modulation of long-term potentiation along the septotemporal axis of the hippocampus. J. Neurosci. 2007;27:5757–65. [Europe PMC free article] [Abstract] [Google Scholar]
- Maggio N, Segal M. Differential corticosteroid modulation of inhibitory synaptic currents in the dorsal and ventral hippocampus. J. Neurosci. 2009a;29:2857–66. [Europe PMC free article] [Abstract] [Google Scholar]
- Maggio N, Segal M. Differential modulation of long-term depression by acute stress in the rat dorsal and ventral hippocampus. J. Neurosci. 2009b;29:8633–8. [Europe PMC free article] [Abstract] [Google Scholar]
- Maggio N, Segal M. Corticosteroid regulation of synaptic plasticity in the hippocampus. ScientificWorldJournal. 2010;10:462–9. [Europe PMC free article] [Abstract] [Google Scholar]
- Malek ZS, Sage D, Pévet P, Raison S. Daily rhythm of tryptophan hydroxylase-2 messenger ribonucleic acid within raphe neurons is induced by corticoid daily surge and modulated by enhanced locomotor activity. Endocrinology. 2007;148:5165–72. [Abstract] [Google Scholar]
- Maroun M. Stress reverses plasticity in the pathway projecting from the ventromedial prefrontal cortex to the basolateral amygdala. Eur. J. Neurosci. 2006;24:2917–22. [Abstract] [Google Scholar]
- Maroun M, Richter-Levin G. Exposure to acute stress blocks the induction of long-term potentiation of the amygdala-prefrontal cortex pathway in vivo. J. Neurosci. 2003;23:4406–9. [Europe PMC free article] [Abstract] [Google Scholar]
- Mayberg HS, Lozano AM, Voon V, McNeely HE, Seminowicz D, Hamani C, Schwalb JM, Kennedy SH. Deep brain stimulation for treatment-resistant depression. Neuron. 2005;45:651–660. [Abstract] [Google Scholar]
- McCann SM, Fruit A. Effect of synthetic vasopressin on release of adrenocorticotrophin in rats with hypothalamic lesions. Proc. Soc. Exp. Biol. Med. 1957;96:566–7. [Abstract] [Google Scholar]
- McEwen BS, Weiss JM, Schwartz LS. Selective retention of corticosterone by limbic structures in rat brain. Nature. 1968;220:911–912. [Abstract] [Google Scholar]
- McKlveen JM, Myers B, Flak JN, Bundzikova J, Solomon MB, Seroogy KB, Herman JP. Role of Prefrontal Cortex Glucocorticoid Receptors in Stress and Emotion. Biol Psychiatry. 2013 [Europe PMC free article] [Abstract] [Google Scholar]
- Meaney MJ, Sapolsky RM, McEwen BS. The development of the glucocorticoid receptor system in the rat limbic brain. II. An autoradiographic study. Brain Res. 1985;350:165–168. [Abstract] [Google Scholar]
- Meng Q-Y, Chen X-N, Tong D-L, Zhou J-N. Stress and glucocorticoids regulated corticotropin releasing factor in rat prefrontal cortex. Mol. Cell. Endocrinol. 2011;342:54–63. [Abstract] [Google Scholar]
- Merchenthaler I, Lane M, Shughrue P. Distribution of pre-pro-glucagon and glucagon-like peptide-1 receptor messenger RNAs in the rat central nervous system. J Comp Neurol. 1999;403:261–280. [Abstract] [Google Scholar]
- Mitra R, Ferguson D, Sapolsky RM. SK2 potassium channel overexpression in basolateral amygdala reduces anxiety, stress-induced corticosterone secretion and dendritic arborization. Mol. Psychiatry. 2009a;14:847–55. 827. [Europe PMC free article] [Abstract] [Google Scholar]
- Mitra R, Ferguson D, Sapolsky RM. Mineralocorticoid receptor overexpression in basolateral amygdala reduces corticosterone secretion and anxiety. Biol. Psychiatry. 2009b;66:686–90. [Abstract] [Google Scholar]
- Mizoguchi K, Ishige A, Takeda S, Aburada M, Tabira T. Endogenous glucocorticoids are essential for maintaining prefrontal cortical cognitive function. J. Neurosci. 2004;24:5492–9. [Europe PMC free article] [Abstract] [Google Scholar]
- Mizoguchi K, Yuzurihara M, Ishige A, Sasaki H, Chui DH, Tabira T. Chronic stress induces impairment of spatial working memory because of prefrontal dopaminergic dysfunction. J. Neurosci. 2000;20:1568–74. [Europe PMC free article] [Abstract] [Google Scholar]
- Moghaddam B. Stress preferentially increases extraneuronal levels of excitatory amino acids in the prefrontal cortex: comparison to hippocampus and basal ganglia. J. Neurochem. 1993;60:1650–7. [Abstract] [Google Scholar]
- Mueller NK, Dolgas CM, Herman JP. Stressor-selective role of the ventral subiculum in regulation of neuroendocrine stress responses. Endocrinology. 2004;145:3763–3768. [Abstract] [Google Scholar]
- Mueller NK, Dolgas CM, Herman JP. Regulation of forebrain GABAergic stress circuits following lesion of the ventral subiculum. Brain Res. 2006;1116:132–142. [Abstract] [Google Scholar]
- Munck A, Guyre PM, Holbrook NJ. Physiological functions of glucocorticoids in stress and their relation to pharmacological actions. Endocr Rev. 1984;5:25–44. [Abstract] [Google Scholar]
- Musazzi L, Milanese M, Farisello P, Zappettini S, Tardito D, Barbiero VS, Bonifacino T, Mallei A, Baldelli P, Racagni G, Raiteri M, Benfenati F, Bonanno G, Popoli M. Acute stress increases depolarization-evoked glutamate release in the rat prefrontal/frontal cortex: the dampening action of antidepressants. PLoS One. 2010;5:e8566. [Europe PMC free article] [Abstract] [Google Scholar]
- Myers B, Carvalho-Netto EF, Wu C, Wick D, Solomon MB, Ulrich-Lai YM, Herman JP. Stress-activated corticolimbic efferents innervate the posterior hypothalamic nucleus: A novel circuit for stimulating neuroendocrine stress responses. Soc. Neurosci. 2012a Abstr. 525.05. [Google Scholar]
- Myers B, Dolgas CM, Kasckow J, Cullinan WE, Herman JP. Central stress-integrative circuits: forebrain glutamatergic and GABAergic projections to the dorsomedial hypothalamus, medial preoptic area, and bed nucleus of the stria terminalis. Brain Struct Funct. 2013 [Europe PMC free article] [Abstract] [Google Scholar]
- Myers B, Greenwood-Van Meerveld B. Corticosteroid receptor-mediated mechanisms in the amygdala regulate anxiety and colonic sensitivity. Am J Physiol Gastrointest Liver Physiol. 2007;292:G1622–9. [Abstract] [Google Scholar]
- Myers B, Greenwood-Van Meerveld B. Divergent effects of amygdala glucocorticoid and mineralocorticoid receptors in the regulation of visceral and somatic pain. Am J Physiol Gastrointest Liver Physiol. 2010a;298:G295–303. [Abstract] [Google Scholar]
- Myers B, Greenwood-Van Meerveld B. Elevated corticosterone in the amygdala leads to persistent increases in anxiety-like behavior and pain sensitivity. Behav Brain Res. 2010b;214:465–469. [Abstract] [Google Scholar]
- Myers B, Greenwood-Van Meerveld B. Differential involvement of amygdala corticosteroid receptors in visceral hyperalgesia following acute or repeated stress. Am J Physiol Gastrointest Liver Physiol. 2012 [Abstract] [Google Scholar]
- Myers B, McKlveen JM, Herman JP. Neural Regulation of the Stress Response: The Many Faces of Feedback. Cell Mol Neurobiol. 2012b [Europe PMC free article] [Abstract] [Google Scholar]
- Nader K, Majidishad P, Amorapanth P, LeDoux JE. Damage to the lateral and central, but not other, amygdaloid nuclei prevents the acquisition of auditory fear conditioning. Learn. Mem. 2001;8:156–63. [Europe PMC free article] [Abstract] [Google Scholar]
- Nathan S, V, Griffith QK, McReynolds JR, Hahn EL, Roozendaal B. Basolateral amygdala interacts with other brain regions in regulating glucocorticoid effects on different memory functions. Ann. N. Y. Acad. Sci. 2004;1032:179–82. [Abstract] [Google Scholar]
- Nederhof E, Schmidt M., V Mismatch or cumulative stress: toward an integrated hypothesis of programming effects. Physiol Behav. 2013;106:691–700. [Abstract] [Google Scholar]
- Oitzl MS, de Kloet ER. Selective corticosteroid antagonists modulate specific aspects of spatial orientation learning. Behav. Neurosci. 1992;106:62–71. [Abstract] [Google Scholar]
- Oitzl MS, Fluttert M, de Kloet ER. The effect of corticosterone on reactivity to spatial novelty is mediated by central mineralocorticosteroid receptors. Eur. J. Neurosci. 1994;6:1072–9. [Abstract] [Google Scholar]
- Olijslagers JE, de Kloet ER, Elgersma Y, van Woerden GM, Joëls M, Karst H. Rapid changes in hippocampal CA1 pyramidal cell function via pre- as well as postsynaptic membrane mineralocorticoid receptors. Eur. J. Neurosci. 2008;27:2542–50. [Abstract] [Google Scholar]
- Oliveira JF, Dias NS, Correia M, Gama-Pereira F, Sardinha VM, Lima A, Oliveira AF, Jacinto LR, Ferreira DS, Silva AM, Reis JS, Cerqueira JJ, Sousa N. Chronic stress disrupts neural coherence between cortico-limbic structures. Front. Neural Circuits. 2013;7:10. [Europe PMC free article] [Abstract] [Google Scholar]
- Palkovits M, Saavedra JM, Jacoboqitz DM, Kizer JS, Záborszky L, Brownstein MJ. Serotonergic innervation of the forebrain: effect of lesions on serotonin and tryptophan hydroxylase levels. Brain Res. 1977;130:121–34. [Abstract] [Google Scholar]
- Pare D, Smith Y, Pare JF. Intra-amygdaloid projections of the basolateral and basomedial nuclei in the cat: Phaseolus vulgaris-leucoagglutinin anterograde tracing at the light and electron microscopic level. Neuroscience. 1995;69:567–583. [Abstract] [Google Scholar]
- Pasricha N, Joëls M, Karst H. Rapid effects of corticosterone in the mouse dentate gyrus via a nongenomic pathway. J. Neuroendocrinol. 2011;23:143–7. [Abstract] [Google Scholar]
- Pavlides C, Ogawa S, Kimura A, McEwen BS. Role of adrenal steroid mineralocorticoid and glucocorticoid receptors in long-term potentiation in the CA1 field of hippocampal slices. Brain Res. 1996;738:229–35. [Abstract] [Google Scholar]
- Phelps EA, LeDoux JE. Contributions of the amygdala to emotion processing: from animal models to human behavior. Neuron. 2005;48:175–87. [Abstract] [Google Scholar]
- Plotsky PM. Facilitation of immunoreactive corticotropin-releasing factor secretion into the hypophysial-portal circulation after activation of catecholaminergic pathways or central norepinephrine injection. Endocrinology. 1987;121:924–930. [Abstract] [Google Scholar]
- Plotsky PM, Cunningham ET, Jr., Widmaier EP. Catecholaminergic modulation of corticotropin-releasing factor and adrenocorticotropin secretion. Endocr Rev. 1989;10:437–458. [Abstract] [Google Scholar]
- Popoli M, Yan Z, McEwen BS, Sanacora G. The stressed synapse: the impact of stress and glucocorticoids on glutamate transmission. Nat. Rev. Neurosci. 2012;13:22–37. [Europe PMC free article] [Abstract] [Google Scholar]
- Poulin J-F, Castonguay-Lebel Z, Laforest S, Drolet G. Enkephalin co-expression with classic neurotransmitters in the amygdaloid complex of the rat. J. Comp. Neurol. 2008;506:943–59. [Abstract] [Google Scholar]
- Poulos AM, Ponnusamy R, Dong H-W, Fanselow MS. Compensation in the neural circuitry of fear conditioning awakens learning circuits in the bed nuclei of the stria terminalis. Proc. Natl. Acad. Sci. U. S. A. 2010;107:14881–6. [Europe PMC free article] [Abstract] [Google Scholar]
- Prewitt CM, Herman JP. Hypothalamo-Pituitary-Adrenocortical Regulation Following Lesions of the Central Nucleus of the Amygdala. Stress. 1997;1:263–280. [Abstract] [Google Scholar]
- Prewitt CM, Herman JP. Anatomical interactions between the central amygdaloid nucleus and the hypothalamic paraventricular nucleus of the rat: a dual tract-tracing analysis. J Chem Neuroanat. 1998;15:173–185. [Abstract] [Google Scholar]
- Pugh CR, Tremblay D, Fleshner M, Rudy JW. A selective role for corticosterone in contextual-fear conditioning. Behav. Neurosci. 1997;111:503–11. [Abstract] [Google Scholar]
- Quirarte GL, Roozendaal B, McGaugh JL. Glucocorticoid enhancement of memory storage involves noradrenergic activation in the basolateral amygdala. Proc. Natl. Acad. Sci. U. S. A. 1997;94:14048–53. [Europe PMC free article] [Abstract] [Google Scholar]
- Radley JJ, Gosselink KL, Sawchenko PE. A discrete GABAergic relay mediates medial prefrontal cortical inhibition of the neuroendocrine stress response. J Neurosci. 2009;29:7330–7340. [Europe PMC free article] [Abstract] [Google Scholar]
- Radley JJ, Rocher AB, Miller M, Janssen WGM, Liston C, Hof PR, McEwen BS, Morrison JH. Repeated stress induces dendritic spine loss in the rat medial prefrontal cortex. Cereb. Cortex. 2006;16:313–20. [Abstract] [Google Scholar]
- Radley JJ, Sawchenko PE. A common substrate for prefrontal and hippocampal inhibition of the neuroendocrine stress response. J Neurosci. 2011;31:9683–9695. [Europe PMC free article] [Abstract] [Google Scholar]
- Radley JJ, Sisti HM, Hao J, Rocher AB, McCall T, Hof PR, McEwen BS, Morrison JH. Chronic behavioral stress induces apical dendritic reorganization in pyramidal neurons of the medial prefrontal cortex. Neuroscience. 2004;125:1–6. [Abstract] [Google Scholar]
- Rainnie DG, Bergeron R, Sajdyk TJ, Patil M, Gehlert DR, Shekhar A. Corticotrophin releasing factor-induced synaptic plasticity in the amygdala translates stress into emotional disorders. J. Neurosci. 2004;24:3471–9. [Europe PMC free article] [Abstract] [Google Scholar]
- Reul JM, de Kloet ER. Two receptor systems for corticosterone in rat brain: microdistribution and differential occupation. Endocrinology. 1985;117:2505–2511. [Abstract] [Google Scholar]
- Reul JM, de Kloet ER. Anatomical resolution of two types of corticosterone receptor sites in rat brain with in vitro autoradiography and computerized image analysis. J Steroid Biochem. 1986;24:269–272. [Abstract] [Google Scholar]
- Revest J-M, Kaouane N, Mondin M, Le Roux A, Rougé-Pont F, Vallée M, Barik J, Tronche F, Desmedt A, Piazza P., V The enhancement of stress-related memory by glucocorticoids depends on synapsin-Ia/Ib. Mol. Psychiatry. 2010;15:1125, 1140–51. [Europe PMC free article] [Abstract] [Google Scholar]
- Richter-Levin G, Maroun M. Stress and amygdala suppression of metaplasticity in the medial prefrontal cortex. Cereb. Cortex. 2010;20:2433–41. [Abstract] [Google Scholar]
- Rivier C, Brownstein M, Spiess J, Rivier J, Vale W. In vivo corticotropin-releasing factor-induced secretion of adrenocorticotropin, beta-endorphin, and corticosterone. Endocrinology. 1982;110:272–278. [Abstract] [Google Scholar]
- Rivier C, Rivier J, Lederis K, Vale W. In vitro and in vivo ACTH-releasing activity of ovine CRF, sauvagine and urotensin I. Regul Pept. 1983;5:139–143. [Abstract] [Google Scholar]
- Rivier C, Vale W. Interaction of corticotropin-releasing factor and arginine vasopressin on adrenocorticotropin secretion in vivo. Endocrinology. 1983a;113:939–942. [Abstract] [Google Scholar]
- Rivier C, Vale W. Modulation of stress-induced ACTH release by corticotropin-releasing factor, catecholamines and vasopressin. Nature. 1983b;305:325–327. [Abstract] [Google Scholar]
- Rivier J, Spiess J, Vale W. Characterization of rat hypothalamic corticotropin-releasing factor. Proc Natl Acad Sci U S A. 1983;80:4851–4855. [Europe PMC free article] [Abstract] [Google Scholar]
- Rodríguez Manzanares PA, Isoardi NA, Carrer HF, Molina VA. Previous stress facilitates fear memory, attenuates GABAergic inhibition, and increases synaptic plasticity in the rat basolateral amygdala. J. Neurosci. 2005;25:8725–34. [Europe PMC free article] [Abstract] [Google Scholar]
- Roland BL, Sawchenko PE. Local origins of some GABAergic projections to the paraventricular and supraoptic nuclei of the hypothalamus in the rat. J Comp Neurol. 1993;332:123–143. [Abstract] [Google Scholar]
- Roozendaal B. 1999 Curt P. Richter award. Glucocorticoids and the regulation of memory consolidation. Psychoneuroendocrinology. 2000;25:213–38. [Abstract] [Google Scholar]
- Roozendaal B. Stress and memory: opposing effects of glucocorticoids on memory consolidation and memory retrieval. Neurobiol Learn Mem. 2002;78:578–595. [Abstract] [Google Scholar]
- Roozendaal B, Griffith QK, Buranday J, De Quervain DJ-F, McGaugh JL. The hippocampus mediates glucocorticoid-induced impairment of spatial memory retrieval: dependence on the basolateral amygdala. Proc. Natl. Acad. Sci. U. S. A. 2003;100:1328–33. [Europe PMC free article] [Abstract] [Google Scholar]
- Roozendaal B, Koolhaas JM, Bohus B. Attenuated cardiovascular, neuroendocrine, and behavioral responses after a single footshock in central amygdaloid lesioned male rats. Physiol Behav. 1991;50:771–775. [Abstract] [Google Scholar]
- Roozendaal B, McGaugh JL. Basolateral amygdala lesions block the memory-enhancing effect of glucocorticoid administration in the dorsal hippocampus of rats. Eur. J. Neurosci. 1997a;9:76–83. [Abstract] [Google Scholar]
- Roozendaal B, McGaugh JL. Glucocorticoid receptor agonist and antagonist administration into the basolateral but not central amygdala modulates memory storage. Neurobiol. Learn. Mem. 1997b;67:176–9. [Abstract] [Google Scholar]
- Roozendaal B, McReynolds JR, McGaugh JL. The basolateral amygdala interacts with the medial prefrontal cortex in regulating glucocorticoid effects on working memory impairment. J. Neurosci. 2004;24:1385–92. [Europe PMC free article] [Abstract] [Google Scholar]
- Roozendaal B, McReynolds JR, Van der Zee EA, Lee S, McGaugh JL, McIntyre CK. Glucocorticoid effects on memory consolidation depend on functional interactions between the medial prefrontal cortex and basolateral amygdala. J. Neurosci. 2009;29:14299–308. [Europe PMC free article] [Abstract] [Google Scholar]
- Roozendaal B, Okuda S, de Quervain DJ-F, McGaugh JL. Glucocorticoids interact with emotion-induced noradrenergic activation in influencing different memory functions. Neuroscience. 2006a;138:901–10. [Abstract] [Google Scholar]
- Roozendaal B, Okuda S, Van der Zee EA, McGaugh JL. Glucocorticoid enhancement of memory requires arousal-induced noradrenergic activation in the basolateral amygdala. Proc. Natl. Acad. Sci. U. S. A. 2006b;103:6741–6. [Europe PMC free article] [Abstract] [Google Scholar]
- Roozendaal B, Phillips RG, Power AE, Brooke SM, Sapolsky RM, McGaugh JL. Memory retrieval impairment induced by hippocampal CA3 lesions is blocked by adrenocortical suppression. Nat. Neurosci. 2001;4:1169–71. [Abstract] [Google Scholar]
- Roozendaal B, Portillo-Marquez G, McGaugh JL. Basolateral amygdala lesions block glucocorticoid-induced modulation of memory for spatial learning. Behav. Neurosci. 1996;110:1074–83. [Abstract] [Google Scholar]
- Roozendaal B, Quirarte GL, McGaugh JL. Glucocorticoids interact with the basolateral amygdala beta-adrenoceptor--cAMP/cAMP/PKA system in influencing memory consolidation. Eur. J. Neurosci. 2002;15:553–60. [Abstract] [Google Scholar]
- Roozendaal B, Schelling G, McGaugh JL. Corticotropin-Releasing Factor in the Basolateral Amygdala Enhances Memory Consolidation via an Interaction with the - Adrenoceptor-cAMP Pathway: Dependence on Glucocorticoid Receptor Activation. J. Neurosci. 2008;28:6642–6651. [Europe PMC free article] [Abstract] [Google Scholar]
- Roozendaal B, Williams CL, McGaugh JL. Glucocorticoid receptor activation in the rat nucleus of the solitary tract facilitates memory consolidation: involvement of the basolateral amygdala. Eur. J. Neurosci. 1999;11:1317–23. [Abstract] [Google Scholar]
- Rozeboom AM, Akil H, Seasholtz AF. Mineralocorticoid receptor overexpression in forebrain decreases anxiety-like behavior and alters the stress response in mice. Proc. Natl. Acad. Sci. U. S. A. 2007;104:4688–93. [Europe PMC free article] [Abstract] [Google Scholar]
- Saffran M, Schally A., V Effect of histamine, hog vasopressin, and corticotropin-releasing factor (CRF) on ACTH release in vitro. Proc. Soc. Exp. Biol. Med. 1956;92:636–7. [Abstract] [Google Scholar]
- Sah P, Faber ESL, Lopez De Armentia M, Power J. The amygdaloid complex: anatomy and physiology. Physiol. Rev. 2003;83:803–34. [Abstract] [Google Scholar]
- Sandi C, Loscertales M, Guaza C. Experience-dependent facilitating effect of corticosterone on spatial memory formation in the water maze. Eur. J. Neurosci. 1997;9:637–42. [Abstract] [Google Scholar]
- Sarabdjitsingh RA, Conway-Campbell BL, Leggett JD, Waite EJ, Meijer OC, de Kloet ER, Lightman SL. Stress responsiveness varies over the ultradian glucocorticoid cycle in a brain-region-specific manner. Endocrinology. 2010a;151:5369–79. [Abstract] [Google Scholar]
- Sarabdjitsingh RA, Joëls M, de Kloet ER. Glucocorticoid pulsatility and rapid corticosteroid actions in the central stress response. Physiol. Behav. 2012a;106:73–80. [Abstract] [Google Scholar]
- Sarabdjitsingh RA, Kofink D, Karst H, de Kloet ER, Joëls M. Stress-induced enhancement of mouse amygdalar synaptic plasticity depends on glucocorticoid and ß-adrenergic activity. PLoS One. 2012b;7:e42143. [Europe PMC free article] [Abstract] [Google Scholar]
- Sarabdjitsingh RA, Spiga F, Oitzl MS, Kershaw Y, Meijer OC, Lightman SL, de Kloet ER. Recovery from disrupted ultradian glucocorticoid rhythmicity reveals a dissociation between hormonal and behavioural stress responsiveness. J. Neuroendocrinol. 2010b;22:862–71. [Europe PMC free article] [Abstract] [Google Scholar]
- Sawchenko PE, Swanson LW, Grzanna R, Howe PR, Bloom SR, Polak JM. Colocalization of neuropeptide Y immunoreactivity in brainstem catecholaminergic neurons that project to the paraventricular nucleus of the hypothalamus. J Comp Neurol. 1985;241:138–153. [Abstract] [Google Scholar]
- Sawchenko PE, Swanson LW, Steinbusch HW, Verhofstad AA. The distribution and cells of origin of serotonergic inputs to the paraventricular and supraoptic nuclei of the rat. Brain Res. 1983;277:355–360. [Abstract] [Google Scholar]
- Sawchenko PE, Swanson LW, Vale WW. Co-expression of corticotropin-releasing factor and vasopressin immunoreactivity in parvocellular neurosecretory neurons of the adrenalectomized rat. Proc Natl Acad Sci U S A. 1984;81:1883–1887. [Europe PMC free article] [Abstract] [Google Scholar]
- Schelling G, Briegel J, Roozendaal B, Stoll C, Rothenhäusler HB, Kapfhammer HP. The effect of stress doses of hydrocortisone during septic shock on posttraumatic stress disorder in survivors. Biol. Psychiatry. 2001;50:978–85. [Abstract] [Google Scholar]
- Schelling G, Hauer D, Pfob M. Reduced cortisol metabolism during critical illness. N. Engl. J. Med. 2013;369:479. [Abstract] [Google Scholar]
- Schelling G, Richter M, Roozendaal B, Rothenhäusler H-B, Krauseneck T, Stoll C, Nollert G, Schmidt M, Kapfhammer H-P. Exposure to high stress in the intensive care unit may have negative effects on health-related quality-of-life outcomes after cardiac surgery. Crit. Care Med. 2003;31:1971–80. [Abstract] [Google Scholar]
- Schelling G, Stoll C, Kapfhammer HP, Rothenhäusler HB, Krauseneck T, Durst K, Haller M, Briegel J. The effect of stress doses of hydrocortisone during septic shock on posttraumatic stress disorder and health-related quality of life in survivors. Crit. Care Med. 1999;27:2678–83. [Abstract] [Google Scholar]
- Schmidt M, V, Abraham WC, Maroun M, Stork O, Richter-Levin G. Stress-induced metaplasticity: From synapses to behavior. Neuroscience. 2013;250:112–20. [Abstract] [Google Scholar]
- Schutsky K, Ouyang M, Castelino CB, Zhang L, Thomas SA. Stress and glucocorticoids impair memory retrieval via β2-adrenergic, Gi/o-coupled suppression of cAMP signaling. J. Neurosci. 2011;31:14172–81. [Europe PMC free article] [Abstract] [Google Scholar]
- Schwabe L, Tegenthoff M, Höffken O, Wolf OT. Mineralocorticoid receptor blockade prevents stress-induced modulation of multiple memory systems in the human brain. Biol. Psychiatry. 2013;74:801–8. [Abstract] [Google Scholar]
- Schwaber JS, Kapp BS, Higgins GA, Rapp PR. Amygdaloid and basal forebrain direct connections with the nucleus of the solitary tract and the dorsal motor nucleus. J. Neurosci. 1982;2:1424–38. [Europe PMC free article] [Abstract] [Google Scholar]
- Schwartz MW, Woods SC, Porte D, Seeley RJ, Baskin DG. Central nervous system control of food intake. Nature. 2000;404:661–71. [Abstract] [Google Scholar]
- Seeley RJ, Woods SC. Monitoring of stored and available fuel by the CNS: implications for obesity. Nat. Rev. Neurosci. 2003;4:901–9. [Abstract] [Google Scholar]
- Selemon LD. A role for synaptic plasticity in the adolescent development of executive function. Transl. Psychiatry. 2013;3:e238. [Europe PMC free article] [Abstract] [Google Scholar]
- Selye H. A Syndrome produced by Diverse Nocuous Agents. Nature. 1936;138:32–32. [Abstract] [Google Scholar]
- Shekhar A, Truitt W, Rainnie D, Sajdyk T. Role of stress, corticotrophin releasing factor (CRF) and amygdala plasticity in chronic anxiety. Stress. 2005;8:209–19. [Abstract] [Google Scholar]
- Shepard JD, Barron KW, Myers DA. Corticosterone delivery to the amygdala increases corticotropin-releasing factor mRNA in the central amygdaloid nucleus and anxiety-like behavior. Brain Res. 2000;861:288–295. [Abstract] [Google Scholar]
- Shepard JD, Barron KW, Myers DA. Stereotaxic localization of corticosterone to the amygdala enhances hypothalamo-pituitary-adrenal responses to behavioral stress. Brain Res. 2003;963:203–213. [Abstract] [Google Scholar]
- Shepard JD, Chambers CO, Busch C, Mount A, Schulkin J. Chronically elevated corticosterone in the dorsolateral bed nuclei of stria terminalis increases anxiety-like behavior. Behav. Brain Res. 2009;203:146–9. [Abstract] [Google Scholar]
- Shepard JD, Schulkin J, Myers DA. Chronically elevated corticosterone in the amygdala increases corticotropin releasing factor mRNA in the dorsolateral bed nucleus of stria terminalis following duress. Behav Brain Res. 2006;174:193–196. [Abstract] [Google Scholar]
- Singewald N, Salchner P, Sharp T. Induction of c-Fos expression in specific areas of the fear circuitry in rat forebrain by anxiogenic drugs. Biol. Psychiatry. 2003;53:275–83. [Abstract] [Google Scholar]
- Smeets T, Wolf OT, Giesbrecht T, Sijstermans K, Telgen S, Joëls M. Stress selectively and lastingly promotes learning of context-related high arousing information. Psychoneuroendocrinology. 2009;34:1152–61. [Abstract] [Google Scholar]
- Soares JM, Sampaio A, Ferreira LM, Santos NC, Marques P, Marques F, Palha JA, Cerqueira JJ, Sousa N. Stress Impact on Resting State Brain Networks. PLoS One. 2013;8:e66500. [Europe PMC free article] [Abstract] [Google Scholar]
- Solomon MB, Jones K, Packard BA, Herman JP. The medial amygdala modulates body weight but not neuroendocrine responses to chronic stress. J Neuroendocr. 2010;22:13–23. [Abstract] [Google Scholar]
- Spiess J, Rivier J, Rivier C, Vale W. Primary structure of corticotropin-releasing factor from ovine hypothalamus. Proc Natl Acad Sci U S A. 1981;78:6517–6521. [Europe PMC free article] [Abstract] [Google Scholar]
- Stellato C. Post-transcriptional and nongenomic effects of glucocorticoids. Proc Am Thorac Soc. 2004;1:255–263. [Abstract] [Google Scholar]
- Sudheimer KD, Abelson JL, Taylor SF, Martis B, Welsh RC, Warner C, Samet M, Manduzzi A, Liberzon I. Exogenous glucocorticoids decrease subgenual cingulate activity evoked by sadness. Neuropsychopharmacology. 2013;38:826–45. [Europe PMC free article] [Abstract] [Google Scholar]
- Sullivan GM, Apergis J, Bush DEA, Johnson LR, Hou M, Ledoux JE. Lesions in the bed nucleus of the stria terminalis disrupt corticosterone and freezing responses elicited by a contextual but not by a specific cue-conditioned fear stimulus. Neuroscience. 2004;128:7–14. [Abstract] [Google Scholar]
- Swanson LW, Cowan WM. An autoradiographic study of the organization of the efferent connections of the hippocampal formation in the rat. J. Comp. Neurol. 1977;172:49–84. [Abstract] [Google Scholar]
- Swanson LW, Petrovich GD. What is the amygdala? Trends Neurosci. 1998;21:323–331. [Abstract] [Google Scholar]
- Swanson LW, Sawchenko PE, Rivier J, Vale WW. Organization of ovine corticotropin-releasing factor immunoreactive cells and fibers in the rat brain: an immunohistochemical study. Neuroendocrinology. 1983;36:165–186. [Abstract] [Google Scholar]
- Tasker JG, Herman JP. Mechanisms of rapid glucocorticoid feedback inhibition of the hypothalamic-pituitary-adrenal axis. Stress. 2011;14:398–406. [Europe PMC free article] [Abstract] [Google Scholar]
- Tauchi M, Zhang R, D'Alessio DA, Stern JE, Herman JP. Distribution of glucagon-like peptide-1 immunoreactivity in the hypothalamic paraventricular and supraoptic nuclei. J Chem Neuroanat. 2008;36:144–149. [Europe PMC free article] [Abstract] [Google Scholar]
- Tye KM, Prakash R, Kim S-Y, Fenno LE, Grosenick L, Zarabi H, Thompson KR, Gradinaru V, Ramakrishnan C, Deisseroth K. Amygdala circuitry mediating reversible and bidirectional control of anxiety. Nature. 2011;471:358–62. [Europe PMC free article] [Abstract] [Google Scholar]
- Ulrich-Lai YM, Herman JP. Neural regulation of endocrine and autonomic stress responses. Nat Rev Neurosci. 2009;10:397–409. [Europe PMC free article] [Abstract] [Google Scholar]
- Ulrich-Lai YM, Jones KR, Ziegler DR, Cullinan WE, Herman JP. Forebrain origins of glutamatergic innervation to the rat paraventricular nucleus of the hypothalamus: differential inputs to the anterior versus posterior subregions. J Comp Neurol. 2011;519:1301–1319. [Europe PMC free article] [Abstract] [Google Scholar]
- Uylings HBM, Groenewegen HJ, Kolb B. Do rats have a prefrontal cortex? Behav. Brain Res. 2003;146:3–17. [Abstract] [Google Scholar]
- Vale W, Spiess J, Rivier C, Rivier J. Characterization of a 41-residue ovine hypothalamic peptide that stimulates secretion of corticotropin and beta-endorphin. Science (80-. ) 1981;213:1394–1397. [Abstract] [Google Scholar]
- Vale W, Vaughan J, Smith M, Yamamoto G, Rivier J, Rivier C. Effects of synthetic ovine corticotropin-releasing factor, glucocorticoids, catecholamines, neurohypophysial peptides, and other substances on cultured corticotropic cells. Endocrinology. 1983;113:1121–1131. [Abstract] [Google Scholar]
- Van Ast VA, Cornelisse S, Meeter M, Joëls M, Kindt M. Time-dependent effects of cortisol on the contextualization of emotional memories. Biol. Psychiatry. 2013;74:809–16. [Abstract] [Google Scholar]
- Van der Kooy D, Koda LY, McGinty JF, Gerfen CR, Bloom FE. The organization of projections from the cortex, amygdala, and hypothalamus to the nucleus of the solitary tract in rat. J. Comp. Neurol. 1984;224:1–24. [Abstract] [Google Scholar]
- Van Haarst AD, Oitzl MS, de Kloet ER. Facilitation of feedback inhibition through blockade of glucocorticoid receptors in the hippocampus. Neurochem Res. 1997;22:1323–1328. [Abstract] [Google Scholar]
- Van Marle HJF, Hermans EJ, Qin S, Overeem S, Fernández G. The effect of exogenous cortisol during sleep on the behavioral and neural correlates of emotional memory consolidation in humans. Psychoneuroendocrinology. 2013;38:1639–49. [Abstract] [Google Scholar]
- Van Rossum EFC, Binder EB, Majer M, Koper JW, Ising M, Modell S, Salyakina D, Lamberts SWJ, Holsboer F. Polymorphisms of the glucocorticoid receptor gene and major depression. Biol. Psychiatry. 2006;59:681–8. [Abstract] [Google Scholar]
- Van Rossum EFC, Koper JW, van den Beld AW, Uitterlinden AG, Arp P, Ester W, Janssen JAMJL, Brinkmann AO, de Jong FH, Grobbee DE, Pols HAP, Lamberts SWJ. Identification of the BclI polymorphism in the glucocorticoid receptor gene: association with sensitivity to glucocorticoids in vivo and body mass index. Clin. Endocrinol. (Oxf) 2003;59:585–92. [Abstract] [Google Scholar]
- Ventura-Silva AP, Pêgo JM, Sousa JC, Marques AR, Rodrigues AJ, Marques F, Cerqueira JJ, Almeida OFX, Sousa N. Stress shifts the response of the bed nucleus of the stria terminalis to an anxiogenic mode. Eur. J. Neurosci. 2012;36:3396–406. [Abstract] [Google Scholar]
- Vertes RP. Differential projections of the infralimbic and prelimbic cortex in the rat. Synapse. 2004;51:32–58. [Abstract] [Google Scholar]
- Vouimba R-M, Yaniv D, Diamond D, Richter-Levin G. Effects of inescapable stress on LTP in the amygdala versus the dentate gyrus of freely behaving rats. Eur. J. Neurosci. 2004;19:1887–94. [Abstract] [Google Scholar]
- Weis F, Kilger E, Roozendaal B, de Quervain DJ-F, Lamm P, Schmidt M, Schmölz M, Briegel J, Schelling G. Stress doses of hydrocortisone reduce chronic stress symptoms and improve health-related quality of life in high-risk patients after cardiac surgery: a randomized study. J. Thorac. Cardiovasc. Surg. 2006;131:277–82. [Abstract] [Google Scholar]
- Weiskrantz L. Behavioral changes associated with ablation of the amygdaloid complex in monkeys. J. Comp. Physiol. Psychol. 1956;49:381–91. [Abstract] [Google Scholar]
- Wellman CL. Dendritic reorganization in pyramidal neurons in medial prefrontal cortex after chronic corticosterone administration. J. Neurobiol. 2001;49:245–53. [Abstract] [Google Scholar]
- Wilensky AE, Schafe GE, Kristensen MP, LeDoux JE. Rethinking the fear circuit: the central nucleus of the amygdala is required for the acquisition, consolidation, and expression of Pavlovian fear conditioning. J. Neurosci. 2006;26:12387–96. [Europe PMC free article] [Abstract] [Google Scholar]
- Woods SC, Seeley RJ, Porte D, Schwartz MW. Signals that regulate food intake and energy homeostasis. Science. 1998;280:1378–83. [Abstract] [Google Scholar]
- Wüst S, Federenko IS, van Rossum EFC, Koper JW, Kumsta R, Entringer S, Hellhammer DH. A psychobiological perspective on genetic determinants of hypothalamus-pituitary-adrenal axis activity. Ann. N. Y. Acad. Sci. 2004;1032:52–62. [Abstract] [Google Scholar]
- Xu Y, Day TA, Buller KM. The central amygdala modulates hypothalamic-pituitary-adrenal axis responses to systemic interleukin-1beta administration. Neuroscience. 1999;94:175–183. [Abstract] [Google Scholar]
- Yehuda R, Cai G, Golier JA, Sarapas C, Galea S, Ising M, Rein T, Schmeidler J, Müller-Myhsok B, Holsboer F, Buxbaum JD. Gene expression patterns associated with posttraumatic stress disorder following exposure to the World Trade Center attacks. Biol. Psychiatry. 2009;66:708–11. [Abstract] [Google Scholar]
- Yehuda R, Halligan SL, Golier JA, Grossman R, Bierer LM. Effects of trauma exposure on the cortisol response to dexamethasone administration in PTSD and major depressive disorder. Psychoneuroendocrinology. 2004;29:389–404. [Abstract] [Google Scholar]
- Young EA, Abelson J, Lightman SL. Cortisol pulsatility and its role in stress regulation and health. Front Neuroendocr. 2004;25:69–76. [Abstract] [Google Scholar]
- Yuen EY, Liu W, Karatsoreos IN, Feng J, McEwen BS, Yan Z. Acute stress enhances glutamatergic transmission in prefrontal cortex and facilitates working memory. Proc. Natl. Acad. Sci. U. S. A. 2009;106:14075–9. [Europe PMC free article] [Abstract] [Google Scholar]
- Yuen EY, Liu W, Karatsoreos IN, Ren Y, Feng J, McEwen BS, Yan Z. Mechanisms for acute stress-induced enhancement of glutamatergic transmission and working memory. Mol. Psychiatry. 2011;16:156–70. [Europe PMC free article] [Abstract] [Google Scholar]
- Yuen EY, Wei J, Liu W, Zhong P, Li X, Yan Z. Repeated stress causes cognitive impairment by suppressing glutamate receptor expression and function in prefrontal cortex. Neuron. 2012;73:962–77. [Europe PMC free article] [Abstract] [Google Scholar]
- Zhang R, Packard BA, Tauchi M, D'Alessio DA, Herman JP. Glucocorticoid regulation of preproglucagon transcription and RNA stability during stress. Proc Natl Acad Sci U S A. 2009;106:5913–5918. [Europe PMC free article] [Abstract] [Google Scholar]
- Zohar J, Yahalom H, Kozlovsky N, Cwikel-Hamzany S, Matar MA, Kaplan Z, Yehuda R, Cohen H. High dose hydrocortisone immediately after trauma may alter the trajectory of PTSD: interplay between clinical and animal studies. Eur. Neuropsychopharmacol. 2011;21:796–809. [Abstract] [Google Scholar]
Full text links
Read article at publisher's site: https://doi.org/10.1016/j.yfrne.2013.12.003
Read article for free, from open access legal sources, via Unpaywall:
https://europepmc.org/articles/pmc4422101?pdf=render
Citations & impact
Impact metrics
Article citations
Behavioral disorders in multiple sclerosis: a comprehensive review.
J Neural Transm (Vienna), 04 Sep 2024
Cited by: 0 articles | PMID: 39231817
Review
Sex-specific cardiac remodeling in aged rats after adolescent chronic stress: associations with endocrine and metabolic factors.
Biol Sex Differ, 15(1):65, 23 Aug 2024
Cited by: 0 articles | PMID: 39180122 | PMCID: PMC11342553
The effects of baicalin in depression: preclinical evidence construction based on meta-analysis.
Front Pharmacol, 15:1425094, 24 Jul 2024
Cited by: 0 articles | PMID: 39114351 | PMCID: PMC11303225
Review Free full text in Europe PMC
Behave yourself: effects of exogenous-glucocorticoid exposure on larval amphibian anti-parasite behaviour and physiology.
Oecologia, 205(1):95-106, 30 Apr 2024
Cited by: 0 articles | PMID: 38689180
Recent advances in the crosstalk between the brain-derived neurotrophic factor and glucocorticoids.
Front Endocrinol (Lausanne), 15:1362573, 05 Apr 2024
Cited by: 1 article | PMID: 38645426 | PMCID: PMC11027069
Review Free full text in Europe PMC
Go to all (140) article citations
Similar Articles
To arrive at the top five similar articles we use a word-weighted algorithm to compare words from the Title and Abstract of each citation.
Novel aspects of glucocorticoid actions.
J Neuroendocrinol, 26(9):557-572, 01 Sep 2014
Cited by: 64 articles | PMID: 24724595 | PMCID: PMC4161987
Review Free full text in Europe PMC
Differential effects of imipramine and CORT118335 (Glucocorticoid receptor modulator/mineralocorticoid receptor antagonist) on brain-endocrine stress responses and depression-like behavior in female rats.
Behav Brain Res, 336:99-110, 01 Sep 2017
Cited by: 11 articles | PMID: 28866130
Brain mechanisms of HPA axis regulation: neurocircuitry and feedback in context Richard Kvetnansky lecture.
Stress, 23(6):617-632, 01 Nov 2020
Cited by: 46 articles | PMID: 33345670 | PMCID: PMC8034599
Review Free full text in Europe PMC
Limbic system mechanisms of stress regulation: hypothalamo-pituitary-adrenocortical axis.
Prog Neuropsychopharmacol Biol Psychiatry, 29(8):1201-1213, 04 Nov 2005
Cited by: 691 articles | PMID: 16271821
Review
Funding
Funders who supported this work.
NIMH NIH HHS (8)
Grant ID: MH069860
Grant ID: F31 MH097430
Grant ID: R21 MH090574
Grant ID: MH097430
Grant ID: R01 MH069860
Grant ID: MH049698
Grant ID: MH090574
Grant ID: R01 MH049698
NINDS NIH HHS (1)
Grant ID: T32 NS007453