Abstract
Free full text

Protection Against Chikungunya Virus Induced Arthralgia Following Prophylactic Treatment with Adenovirus Vectored Interferon (mDEF201)
Abstract
Recent outbreaks of Chikungunya virus (CHIKV) infection have resulted in millions of cases of disease with significant morbidity. No approved antiviral treatments exist for the prevention or treatment of this viral disease. Infection with CHIKV results in a high rate of symptomatic disease that primarily includes a debilitating arthralgia. To model this cardinal disease manifestation, adult DBA/1J mice were challenged with CHIKV by footpad injection. Viremia and hind limb virus titers increased ~100-fold while spleen virus increased >1,000-fold within 1 day post-virus infection (dpi). Footpad swelling was measured over a 10-day period, with peak swelling observed between 6 and 7 dpi. Histology of the hind leg at the site of virus challenge showed evidence of myositis and synovitis starting on 5 dpi. Cytokine profiling of the hind limb at the site of inoculation revealed a biphasic inflammatory response represented by an increase in IL-6, MCP-1, IFN-γ, MIP-1α, RANTES, and IL-17. To investigate the prophylactic capacity of IFN, mice were treated with mDEF201, an adenovirus-vectored IFN-α. Intranasal administration of a single 107 pfu/ml dose of mDEF201 administered 21 days to 24 h prior to infection, significantly reduced footpad swelling, virus titers in the hind leg and spleen, and several inflammatory cytokines. Efficacy was not observed when treatment was initiated 24 h after virus challenge. This arthralgia model of CHIKV recapitulates relevant disease features commonly observed in human disease making it applicable to preclinical testing of therapies that target both viral replication and the associated joint disease.
1. Introduction
Chikungunya virus (CHIKV) is associated with significant morbidity and mortality worldwide. Historically, the primary vector for spread of CHIKV was Aedes aegypti, although recent adaptive mutations within the virus have resulted in a more efficient infection of Aedes albopictus (Tsetsarkin et al., 2006). This has resulted in outbreaks outside the historical range, making this emerging virus a major public health concern.
The rapid emergence of CHIKV and the millions of cases of disease underscore the importance of the development of countermeasures to prevent or treat the symptoms of CHIKV. Fever and arthralgia are common symptoms of CHIKV infection, although a small number (5–18%) of infected people, especially those below age 25, are asymptomatic (Dupuis-Maguiraga et al., 2012). Mortality as a result of CHIKV infection is rare and is generally associated with underlying health issues (Thiberville et al., 2013). Rheumatic manifestations typically affect the extremities, primarily including ankles, wrists and phalanges (Kennedy et al., 1980). A study conducted after the La Reunion Island outbreak identified persistent arthritis in over half of the participants 15 months after acute infection, with 43% of those with persistent arthritis significantly impaired in carrying out daily or household activities (Sissoko et al., 2009). As the rheumatic disease is debilitating and very common in persons infected with CHIKV, it would be important to prevent or treat this aspect of the disease.
An animal model that replicates human arthralgia is essential for the development of countermeasures for the prevention and treatment of disease associated with CHIKV infection. Macaques have been used to study CHIKV, and this model mimics many acute symptoms seen in humans such as fever, rash, and high viral titers but displays inconsistent swelling of the joints and lacks the joint and muscle pathology typically observed after infection with CHIKV (Higgs and Ziegler, 2010).
Various mouse models have been developed which model different aspects of the disease. The C57BL/6 neonatal mouse model includes relevant disease manifestations such as joint swelling. There is also consistency between disease seen in children less than one year of age and this model, with virus being found in similar organs and a strong age-dependent correlation with severity of disease (Couderc et al., 2008). Interferon receptor knockout mice were highly susceptible to infection with CHIKV and rapidly succumbed to infection (Couderc et al., 2008), which serves to model severe infection and mortality that is occasionally seen. Infection of these immunocompromised mice results in dissemination of the virus to all tissue types, including the central nervous system (Partidos et al., 2011). This pathology may have relevance in light of recently reported neurologic complications of encephalopathy in newborns and meningitis and encephalitis in older children and adults (Arpino et al., 2009). Inoculation of the footpad of 14-day old C57BL/6 mice resulted in swelling, arthritis, tenosynovitis and myositis (Morrison et al., 2011), but a neonatal model has limitations, especially in regard to testing vaccines. Others have developed a model of footpad swelling and joint involvement in adult C57BL/6 by injecting virus into the footpad (Gardner et al., 2010).
A replication-deficient adenovirus type 5 vector containing a gene for mouse IFN-α, mDEF201, is administered intranasally where it enters nasal epithelial cells and expresses the transgene (Julander et al., 2011). This results in the production of systemic levels of IFN several hours after treatment (Wu et al., 2007). With non-lethal infections such as CHIKV, type I interferon (IFN) plays a role in coordinating early antiviral immune response (Havenar-Daughton et al., 2006), and plays a central role in controlling CHIKV infection, acting indirectly as an antiviral through the induction of IFN-stimulated genes that inhibit viral replication (Schilte et al., 2010). The nonstructural proteins (nsPs) of CHIKV antagonize the IFN response as infection progresses, so the timing of IFN intervention is critical (Fros et al., 2010).
This manuscript details the establishment of an adult mouse model of CHIKV arthritis and swelling. Footpad swelling, virus replication, and cytokine profiles are used as parameters for the characterization of the antiviral effect of mDEF201 against CHIKV. These results demonstrate the utility of this model for use in antiviral studies.
2. Materials and Methods
2.1 Cells
C6/36 cells were obtained from ATCC (Manassas, VA) and were used for virus propagation. These cells were grown in RPMI at 28°C with 5% fetal bovine serum (FBS). Vero 76 cells, maintained in minimal essential medium (MEM) supplemented with 10% FBS, were also obtained from ATCC and were used in viral titer assays. Media was obtained from Hyclone Laboratories, Logan, UT.
2.2 Virus
Chikungunya virus strain S27 (VR-64) was obtained from ATCC. Stock virus was prepared by harvesting supernatant from C6/36 cells 2 days after inoculation.
2.3 Animals
Seven-week old DBA/1J mice were obtained from Jackson Laboratory. Mice were quarantined for 48 hours prior to infection.
2.4 Test Materials
A stock of mDEF201 was provided by Defyrus (Toronto, ON, Canada), which had a titer of 1.45 × 109 pfu/ml (construction of vector described fully in Wu et al, 2007). An empty Ad-5 vector was also provided by Defyrus, which was used as a negative control.
2.5 Experimental Design
For model characterization studies, DBA/1J mice were randomly assigned to groups of 10 animals. Mice were challenged under isoflorane anesthesia by footpad and hock injection with 108 CCID50/0.1 ml of virus (0.05 ml at each site) diluted in MEM. On days 1–10, 14, and 21, 4 mice were necropsied and serum, hind legs, and spleen were collected, weighed and prepared for virus titration and cytokine profiling. Animals were also weighed throughout the study to determine the effect of infection on weight change.
For treatment studies, mice were randomly distributed into groups of 10 to 15 animals and were treated with mDEF201, empty vector or saline. Sham infected controls were also included to monitor potential toxicity of treatment. In the first study, animals were treated with a range of mDEF201 doses. At 2 dpi, 5 animals per group were necropsied and spleens and hind limbs were harvested, weighed, and the virus titer was determined. Virus titer and cytokine levels were determined from hind limb homogenates. The remaining animals were weighed on days 0, 2, 4, 6, 8, & 10. The dorsoventral thickness of the footpad at the site of virus challenge was measured with a digital caliper and the percent increase as compared to the contralateral foot was calculated.
In subsequent studies, a dose of 107 of mDEF201 was administered intranasally at various times prior to or after virus challenge to determine the effect of extended prophylaxis or therapeutic treatment on CHIKV infection. Footpad increase in all animals was measured on 6 dpi as described above. Tissues were then harvested from a subset of animals on 6 dpi with virus and cytokine titers determined as described below.
2.6 Tissue virus titer determination
Samples were homogenized and diluted in 1 ml MEM, containing 0.05 mg/ml gentamicin. The homogenate was centrifuged for 10 min at 2300 RCF added to Vero 76 cells in triplicate and examined for cytopathic effect (CPE) on 3 dpi. Virus titers were determined by end point titration as described previously (Reed and Muench, 1938).
2.7 Cytokine analysis
Homogenized leg samples were evaluated for proinflammatory cytokine/chemokine levels using the Quansys Q-plex™ Mouse Cytokine Array (Quansys Biosciences, Logan, UT). The Quansys array quantifies levels of murine IL-1α, IL-1β, IL-2, IL-3, IL-4, IL-5, IL-6, IL-10, IL12p70, IL-17, MCP-1, IFNγ, TNFα, MIP-1α, GMCSF and RANTES. Samples were diluted in sample diluent at 1:5 and 1:20 concentrations. A standard curve for each antigen was generated by serial dilution of antigen standards from 1:3 to 1:729. Standards were plated in duplicate on opposite sides of the plate and samples were plated at two dilutions. Manufactures instructions were followed for adding substrate, incubating and washing. Plates were quantified on a Quansys Q-View™ Imager with Q-View™ software.
2.8 Histopathology
Right and left hind legs were collected at 6 and 12 h, and days 1–10, 14 and 21 post-infection. Samples were fixed in 10% formalin and sent for analysis to the Utah State University Veterinary Diagnostic Laboratory. Samples were paraffin-embedded and sectioned for histopathologic evaluation. Slides were stained with hematoxylin and eosin.
2.9 Statistical analysis
Statistical analyses were done using one-way ANOVA using a Bonferroni group comparison (Prism 5, GraphPad Software, Inc).
2.10 Ethics statement
This study was conducted in accordance with the approval of the Institutional Animal Care and Use Committee of Utah State University under the approved protocol numbers 1526 and 2339. The work was done in the AAALAC-accredited and PHS Animal Welfare Assurance-approved Laboratory Animal Research Center of Utah State University.
3. RESULTS
3.1 Characterization of CHIKV infection of DBA/1 mice
Several mouse strains, including C57BL/6, AG129, CAST/EiJ and DBA/1J, were inoculated with CHIKV and disease signs, including footpad swelling, differences in behavior, weight change and mortality, were observed. The DBA/1J strain was selected based on the development of robust footpad swelling, availability and use in arthritis research (Chiba et al., 2012; Eros et al., 2009; Nishida et al., 2002). To characterize CHIKV replication and disease in this mouse strain, DBA/1J mice were inoculated with S27 CHIKV in the rear hind footpad and footpad swelling, virus titer of various tissues, cytokine levels, and weight change were quantified. Significant swelling of the footpad, ankle and hock at the site of virus challenge was observed as early as 6 dpi (Figure 1A). The contralateral footpad was normal and served as a control for measuring percent increase in footpad thickness. Swelling was time-dependent and showed a dose responsive increase depending on virus challenge dose (data not shown). Significant swelling (P<0.001) of the footpad was initially observed on 6 dpi, with peak swelling on 7 dpi. Footpad thickness returned to baseline levels by 9 dpi.
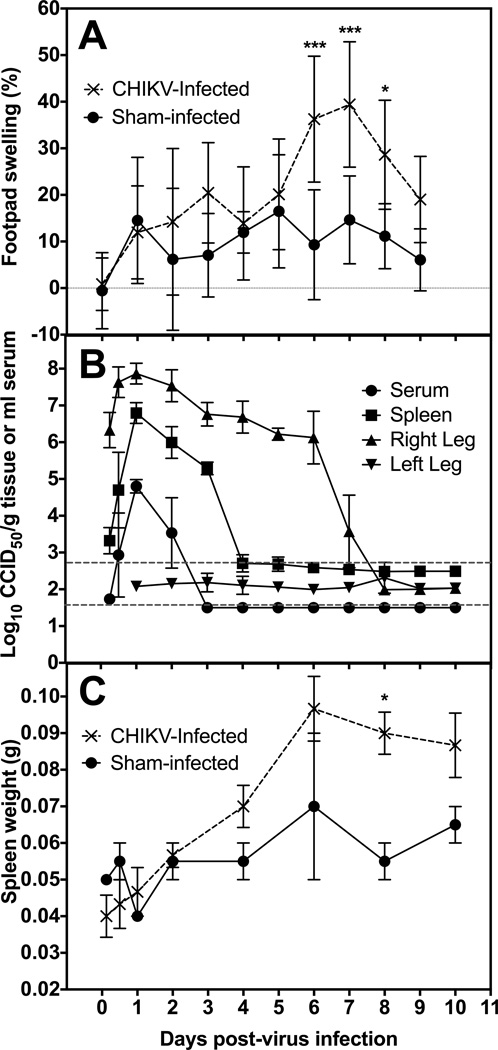
Disease parameters of mice infected with the S27 strain of CHIKV. A) The effect of CHIKV infection on the time course of footpad swelling of DBA/1J mice at the site of virus challenge when compared to contralateral footpad. B) Time-course of virus in serum, spleen, right hind leg, and left hind leg of DBA/1J mice infected with CHIKV. Viral titer is plotted as 50% cell culture infectious dose (CCID50) per gram of tissue or ml of serum. Upper dashed line is the average limit of detection for tissue homogenates and lower line is for serum. C) Comparison of Sham infected and CHIKV infected spleen weights in grams throughout course of infection (***P<0.001, *P<0.05, as compared with sham-infected controls).
Average virus titer in the right hind leg at the site of inoculation was approximately 6 log10 CCID50/g at 6 h after virus challenge (Figure 1B), which was due to the footpad inoculation at this site. Titers increased approximately 100-fold to just below 8 log10 CCID50/g at 24 h after virus challenge. The right hind leg virus titers persisted at an average titer of 6–7 log10 CCID50/g until 6 dpi, at which point they began to return to baseline levels and were below the limit of detection on 8 dpi. In contrast, no virus was observed in the left hind leg contralateral to the injection site.
Virus titer in the spleen was detected as early as 12 h after virus challenge. Spleen titers peaked at around 7 log10 CCID50/g tissue, which was >1,000-fold increase in virus above the level of detection in that organ (Figure 1B). Virus was reduced below the limits of detection by 4 dpi. A similar increase in serum virus was also observed, although a more rapid clearance of viremia was observed with virus titer reduction to undetectable levels a day earlier on 3 dpi as compared with spleen titers (Figure 1B). No virus was detected in any of the tissues assayed from sham-infected animals (data not shown).
Splenomegaly was observed in mice infected with CHIKV as early as 2 dpi, with spleen size continuing to increase through 10 dpi (Figure 1C). Weight change of CHIKV-infected mice did not differ significantly from that of sham-infected animals (data not shown).
Histologic analysis of the right hind limbs demonstrated cellulitis, myositis, tendinitis, and teno and arthrosynovitis as early as 5 dpi, which continued over the course of the infection (Figure 2). No significant histological lesions were seen on the left hind limb (Figure 2A and C).

Hematoxylin and eosin stained sections of skeletal muscle (A, B) and footpads (C, D) show pathologic changes after CHIKV infection. A) Normal skeletal muscle taken 5 dpi from control mice. B) Myositis (arrow head) and tendinitis (arrow) are apparent in the skeletal muscle of a CHIKV-infection mouse taken on 5 dpi (400× magnification of skeletal muscle, bar = 50 µm). C) Normal footpad section from a mouse on 7 days after mock infection. D) Cellulitis due to mixed inflammatory cell infiltration is observed on 7 dpi in footpad sections taken 7 dpi from mice challenged with CHIKV (200× magnification of footpad sections, bar = 200 µm).
Several proinflammatory cytokines were significantly elevated in tissue homogenate of the limb inoculated with CHIKV. There appeared to be a biphasic increase in cytokine levels in the hind leg with an initial peak in IL-6, MCP-1, IFN-γ, MIP-1α, RANTES, and IL-17 around 1 dpi with a subsequent peak in levels 5–7 dpi (Figure 3). Other cytokines, including IL-1α, IL-1β, IL-2, IL-3, IL-5, IL-10, IL-12p70, TNF-α, and GM-CSF did not appear to be affected by CHIKV infection of mice as compared with control mice (data not shown). Cytokine levels in the serum of infected animals were not significantly altered after CHIKV infection (data not shown). There did appear to be some slight increase in serum IL-6, MCP-1, MIP-1α, and RANTES on 2 dpi, which corresponded with the initial peak observed in the hind limb at the site of virus inoculation, but the second peak around 8 dpi was only observed with IL-6 and MCP-1 (Table 1), suggesting a more localized effect at the site of inoculation. Cytokine titers in the spleen were similar to those in the serum, with the exception of RANTES levels that were elevated 20 to 87-fold in the spleen homogenates as compared with serum on 2 and 9 dpi (Table 1). Sham-infected animals also had higher levels of RANTES in the spleen as compared with serum, suggesting higher baseline levels in this tissue.

Cytokines levels, including IL-6, MCP-1, IFN-γ, MIP-1α, RANTES and IL-17, in hind leg homogenates at the site of virus injection were elevated as compared with levels in tissue taken from sham-infected animals.
Table 1
Serum and spleen levels (pg/ml) of selected cytokines of DBA/1J mice challenged with CHIKV. Virus challenge did not appear to cause an increase in cytokines in these tissues as compared with cytokine levels at the site of virus challenge.
2 dpi | 9 dpi | ||||||||
---|---|---|---|---|---|---|---|---|---|
Tissue | Challenge | IL-6 | MCP-1 | MIP-1a | RANTES | IL-6 | MCP-1 | MIP-1a | RANTES |
Serum | CHIKV | 6.6 ± 9.4a | 223 ± 147 | 10 ± 0.8 | 50 ± 20 | 10.4 | 383 ± 503 | 14 ± 0.8 | 35 ± 1.8 |
Sham | bdlb | 25 | 9.9 ± 2.0 | 31 ± 0.8 | bdl | 26 ± 0.4 | 16 ± 4.4 | 34 ± 1.1 | |
Spleen | CHIKV | bdl | 107 ± 41 | 219 ± 181 | 4351 ± 661 | 9.9 ± 1.6 | 29 ± 4.3 | 20 ± 9.1 | 669 ± 7.7 |
Sham | bdl | 26 | 28 | 1056 | bdl | 26 | 23 | 1959 |
3.2 Treatment of CHIKV with mDEF201
To determine the effect of mDEF201 treatment on the development of disease after CHIKV infection, animals were treated with a single intranasal instillation 24 h prior to virus challenge. Evaluation parameters used to determine mDEF201 efficacy included percent footpad swelling as compared with the contralateral foot, virus titers in the hind leg and spleen and cytokine levels in the hind leg.
Footpad swelling was significantly (P<0.001) reduced on 7 dpi in animals treated with 107 pfu/ml of mDEF201 as compared with placebo- and empty vector-treated controls and was comparable with sham-infected controls (Figure 4A). A significant (P<0.001) reduction of viral load was observed on 2 dpi in hind limbs and spleens of animals treated with 107 pfu/ml of mDEF201 as compared with placebo- or empty vector-treated animals (Figure 4B and 4C, respectively). Average titers in these tissues were reduced approximately 2 log10 CCID50/g. Footpad swelling and tissue titers in groups treated with lower doses of mDEF201 were similar to those parameters of saline- or empty vector-treated controls and had no observed effect on disease (Figures 4A–4C).

The effect of mDEF201 administered intranasally 24 h prior to virus challenge at doses of 107, 106, or 105 pfu/animal. A) The percent increase in footpad swelling at the site of virus challenge was significantly reduced as compared with the contralateral footpad on 7 days post infection (dpi) in animals treated with the highest dose of mDEF201. Virus titer of B) the inoculated limbs and C) spleen of mDEF201-treated or control mice on 2 dpi. Significant reduction in virus titer was observed at the highest dose of mDEF201. Cytokines including D) IL-6, E) MCP-1, F) MIP-1α, G) RANTES, all cytokines show a trend towards reduction with mDEF201 treatment. Cytokine reduction was verified in a subsequent study and a similar trend towards reduction of H) IL-6, I) MCP-1, J) MIP-1α, and K) RANTES was observed in the hind limb on 6 dpi after prophylactic treatment with 107 pfu of mDEF201.
Reduced levels of key cytokines, including IL-6, MCP-1, MIP-1α, and RANTES, were observed on 2 dpi in the virus-inoculated hind limb of mice treated with the highest dose of mDEF201 as compared with saline treatment (Figures 4D–4G, respectively). These reductions were significant (P<0.05) for MCP-1 and RANTES (Figures 4E and 4G, respectively). In a subsequent study, cytokine levels from hind leg samples taken 6 dpi from animals treated with 107 mDEF201 or with saline were quantified and showed a similar trend towards reduction of IL-6, MCP-1, and MIP-1α (Figures 4H–4J, respectively), as well as a significant (P<0.001) reduction in RANTES (Figure 4K).
To determine the effect of treatment with mDEF201 after virus challenge, 107 pfu was administered 24, 48 or 72 h post-inoculation. Administration of mDEF201 at these time points failed to significantly impact footpad thickness, virus titer or reduction of cytokine levels in the hind limb (data not shown). As a positive control to this study, mDEF201 was administered at 24 h prior to virus challenge, which reduced footpad thickness as in previous studies. Virus titers measured in hind limb samples taken on 6 dpi, however, were not significantly reduced (data not shown), despite a previously observed reduction of virus titer on 2 dpi after treatment with DEF201 given along the same schedule (Figure 4B).
Extended prophylaxis evaluation was conducted to test the efficacy of mDEF201 when administered as early as 21 days prior to infection. Swelling of the footpad was significantly reduced (p-value<0.001) in animals treated one day prior to virus challenge (Figure 5A), as seen in previous studies A single treatment with mDEF201 on 7, 14, or 21 days prior to virus challenge prevented footpad swelling at the site of virus inoculation (right hind leg). Treatment at these time points resulted in footpad thickness similar that observed for sham-infected, treated controls, and were significantly (P<0.001) lower than that of infected animals treated with empty vector or with placebo (Figure 5A).

The effect of prophylactic mDEF201 treatment administered i.n. on 21, 14, 7, or 1 day prior to virus challenge at a dose of 107 pfu. (A) Footpad swelling was significantly reduced on 6 days post infection in all treatment groups when compared with empty vector and saline treated control groups. (B) Virus titer in the hind limb homogenates on 6 days post infection were reduced in earlier treatment groups and (C) IL-6, (D) MCP-1, (E) MIP-1α and (F) RANTES were generally reduced on 6 days post infection.
Treatment of mice with mDEF201 1 or 7 days prior to virus challenge had no effect on reducing virus replication in the hind leg (at the site of virus challenge) as measured on 6 dpi (Figure 5B). However, mice that were treated 14 or 21 days prior to virus challenge had significantly lower (P< 0.01) virus titers than animals treated with empty vector or placebo.
Cytokine levels were measured from hind leg homogenates of the inoculated limb (also used for virus titration), which were taken from a subset of animals on 6 dpi. In general, treatment with mDEF201 at all time points prior to virus challenge resulted in significant reduction (P<0.001) in IL-6, MCP-1, MIP-1α, and RANTES when measured 6 dpi (Figure 5C–5F, respectively). One exception was the lack of significant reduction of IL-6 in animals treated one day prior to virus challenge (not shown), which was consistent with previous results (Figure 4H).
4. Discussion
Infection of DBA/1J mice with CHIKV mirrors several disease signs observed in humans, including arthralgia, high acute viral titers and increased cytokine levels (Couderc and Lecuit, 2009). This non-lethal model focuses primarily on the arthritic disease manifestations that occur most commonly in infected individuals. The primary clinical and economic burden of human CHIKV disease is arthralgia, which renders infected individuals unable to work and perform other necessary activities, which in a setting of limited resources, can be a large drain to the financial wellbeing of hundreds of thousands of people. Treatment or prevention of arthralgia in infected individuals, therefore, would be very important to reducing the burden of this viral disease.
Increased levels of several cytokines, accompanied by swelling and severe tissue damage, were observed in mice infected with CHIKV 6–7 dpi. Infected patients have elevated IL-6, GM-CSF and MCP-1 during infection (Chirathaworn et al., 2013; Lohachanakul et al., 2012), further supporting the relevance of this mouse model to human infection. In addition, mDEF201 prophylactic treatment alleviated cytokine elevation and footpad swelling. The immune response to infection, including recruitment of macrophages by MCP-1 and activation of these cells by IL-6 within the joint, plays an important role in the immunopathogenesis associated with CHIKV infection and is a common mechanism of disease of various viral arthritides (Suhrbier and Mahalingam, 2009). It is likely that the arthrogenic immune response in CHIKV infection is excessive and may be targeted as an immunopathology, as evidenced herein by progressive clearance of the virus as the joint swelling and cytokine response mounts. This must be further delineated, but future studies will focus on targeting specific aspects of the immune response to determine the effect of immunotherapy as a treatment for arthralgia associated with CHIKV infection.
The DBA/1J mouse strain appears to better model arthralgia after CHIKV infection than other mouse strains tested in our lab. Infection of C57BL/6 mice with the S27 East African isolate of CHIKV was also conducted in parallel with infection of DBA/1J mice but no significant swelling was observed. A virus inoculum of 108 CCID50/mouse was used, which would be much higher than the amount transferred after the bite of a mosquito during natural infection. Infection with high challenge doses of virus has been shown to alter disease profile in non-human primate models, with higher virus challenges with a Reunion Island isolate resulting in a more severe disease phenotype and death (Labadie et al., 2010). Subsequent studies in our lab have utilized the same Reunion Island strain (LR2006-OPY1) of CHIKV, which requires around approximately 100,000-fold less virus infectious doses in DBA/1J mice to cause a similar disease profile as reported herein (manuscript in preparation).
Despite the utility observed with the C57BL/6 model in other labs, including use in vaccine studies (Wang et al., 2011), the DBA/1J mice appear to be more sensitive to infection with CHIKV as compared during parallel challenge studies using the same virus inoculum in both mouse strains. In addition to infection of DBA/1J and C57BL/6 mice, we also challenged AG129 mice in parallel (data not shown). Similar results including rapid mortality were observed as previously reported (Partidos et al., 2011). The CAST/EiJ mouse has previously been shown to be susceptible to infection with monkeypox, a virus that does not cause disease in BALB/c, C57BL/6, and other commonly used inbred strains (Americo et al., 2010). For this reason, we included CAST/EiJ mice in our mouse strain screen to determine if they would be suitable for modeling CHIKV disease. Significant swelling of the foot and hock was observed at the site of virus injection after footpad inoculation, which was similar to swelling observed in DBA/1J mice. The CAST/EiJ mice, however, are limited in availability and were abandoned in favor of the more readily available DBA/1J strain.
Neonatal mouse models of CHIKV are also commonly used. Intradermal infection of 12-day old mice results in morbidity and mortality (Couderc et al., 2008), representing severe disease phenotype. Virus is detected in the muscle, joint, skin and brain tissue of these mice, which is similar to rare cases of encephalitic disease of patients infected with CHIKV (Gerardin et al., 2008; Ramful et al., 2007; Robin et al., 2008). While virus was very rarely detected in the brains of infected DBA/1J mice (data not shown), detectable titers were observed in various tissues including joints, spleen, and serum. Use of adult DBA/1J mice allows for virus interaction with a developed immune system and may better model aspects of human disease that arise as a result of immunopathogenesis, which may be different in neonatal mice.
Another potential benefit of the DBA/1J model is the consistency of swelling among animals. All infected mice had fairly consistent swelling, as compared with inconsistent swelling of joints and rash observed in infected macaques in a recent study (Chen et al., 2010). In addition, no evidence of arthritis was observed in the macaques, as compared with histolopathology consistent with arthritis in DBA/1J mice after CHIKV infection. Notably, no rash is detected in these mice, despite being a common disease sign associated with CHIKV infection in man (Schmidt-Chanasit et al., 2012).
The main limitation of CHIKV infection in DBA/1J mice is the lack of the development of a persistent chronic arthralgia that is observed in human patients (Jaffar-Bandjee and Gasque, 2012). Measurable swelling was observed between 5 and 8 days after virus inoculation, while joint pain and swelling in infected patients can be observed for weeks to months, and may even persist for years in some individuals (Couturier et al., 2012). The few days of swelling, however, does provide a measureable parameter of disease that can be used to determine the effect of antiviral or host-directed therapeutics on the amelioration of this important disease manifestation. No animal models have been shown to develop chronic arthralgia, and the basis for the persistence of this disease parameter in man has yet to be elucidated.
Another potential limitation of this model is the lack of mortality associated with CHIKV infection at a relatively high virus challenge. Despite the lack of mortality in this model, other mouse strains, such as AG129 mice deficient in IFN receptors, may be used to further characterize the effect of antiviral therapies against more severe disease manifestations. A study conducted during the 2005–2006 Reunion outbreak identified 610 atypical cases where 546 of these cases had underlying medical conditions and 65 patients died (Economopoulou et al., 2009). This aspect of underlying medical conditions shows the potential for CHIKV to affect immune compromised individuals. Nevertheless, arthralgia, the primary disease manifestation in this mouse model, is still the most prominent and debilitating aspect overall of CHIKV disease in man and should be a focus for the development of therapeutics.
Prophylaxis with mDEF201 may have clinical potential in endemic areas of CHIKV disease. In the event of an outbreak of CHIKV, it would be useful to have a single dose prophylactic agent that could provide protection over several weeks to those at risk of infection. Future studies will be conducted to determine the pre-exposure prophylactic window for mDEF201 utilizing the DBA/1J model.
Studies in the DBA/1J model demonstrate a rapid course of virus replication and clearance, suggesting a limited treatment window for antiviral compounds targeting the lifecycle of the virus. Treatment with DEF201 results in the production of consensus IFN and a decrease in disease when given prior to virus challenge. Virus was significantly reduced at the site of virus challenge on 2 dpi, but virus titers were similar or slightly higher than placebo-treated controls on 6 dpi. While the reasons for this are unknown, there is an apparent disconnect between virus titer in the tissues and footpad swelling. This is evident as treatment with an immune modulator such as mDEF201 may significantly impact footpad swelling while having no effect on virus tissue titers. Additional research will be needed to delineate this effect further. These studies demonstrate prophylaxis with a safe and effective mediator of antiviral response is effective in preventing or ameliorating disease as a result of CHIKV infection. In addition, vaccine development would likely be an effective means of disease prevention within endemic regions.
Acknowledgements
We thank Isaac Wong, Joseph Hagloch, Brett Hurst, Makda Gebre, and Shelby Wilcox for expert technical assistance. This work was supported by funding from the Virology Branch, DMID, NIAID, NIH (HHSN272201000039I/HHSN27200004/A21).
Footnotes
Publisher's Disclaimer: This is a PDF file of an unedited manuscript that has been accepted for publication. As a service to our customers we are providing this early version of the manuscript. The manuscript will undergo copyediting, typesetting, and review of the resulting proof before it is published in its final citable form. Please note that during the production process errors may be discovered which could affect the content, and all legal disclaimers that apply to the journal pertain.
References
- Americo JL, Moss B, Earl PL. Identification of wild-derived inbred mouse strains highly susceptible to monkeypox virus infection for use as small animal models. Journal of virology. 2010;84:8172–8180. [Europe PMC free article] [Abstract] [Google Scholar]
- Arpino C, Curatolo P, Rezza G. Chikungunya and the nervous system: what we do and do not know. Reviews in medical virology. 2009;19:121–129. [Abstract] [Google Scholar]
- Chen CI, Clark DC, Pesavento P, Lerche NW, Luciw PA, Reisen WK, Brault AC. Comparative pathogenesis of epidemic and enzootic Chikungunya viruses in a pregnant Rhesus macaque model. The American journal of tropical medicine and hygiene. 2010;83:1249–1258. [Europe PMC free article] [Abstract] [Google Scholar]
- Chiba A, Mizuno M, Tomi C, Tajima R, Alloza I, di Penta A, Yamamura T, Vandenbroeck K, Miyake S. A 4-trifluoromethyl analogue of celecoxib inhibits arthritis by suppressing innate immune cell activation. Arthritis research & therapy. 2012;14:R9. [Europe PMC free article] [Abstract] [Google Scholar]
- Chirathaworn C, Poovorawan Y, Lertmaharit S, Wuttirattanakowit N. Cytokine levels in patients with chikungunya virus infection. Asian Pacific journal of tropical medicine. 2013;6:631–634. [Abstract] [Google Scholar]
- Couderc T, Chretien F, Schilte C, Disson O, Brigitte M, Guivel-Benhassine F, Touret Y, Barau G, Cayet N, Schuffenecker I, Despres P, Arenzana-Seisdedos F, Michault A, Albert ML, Lecuit M. A mouse model for Chikungunya: young age and inefficient type-I interferon signaling are risk factors for severe disease. PLoS Pathog. 2008;4:e29. [Europe PMC free article] [Abstract] [Google Scholar]
- Couderc T, Lecuit M. Focus on Chikungunya pathophysiology in human and animal models. Microbes and infection/Institut Pasteur. 2009;11:1197–1205. [Abstract] [Google Scholar]
- Couturier E, Guillemin F, Mura M, Leon L, Virion JM, Letort MJ, De Valk H, Simon F, Vaillant V. Impaired quality of life after chikungunya virus infection: a 2-year follow-up study. Rheumatology (Oxford) 2012;51:1315–1322. [Abstract] [Google Scholar]
- Dupuis-Maguiraga L, Noret M, Brun S, Le Grand R, Gras G, Roques P. Chikungunya disease: infection-associated markers from the acute to the chronic phase of arbovirus-induced arthralgia. PLoS neglected tropical diseases. 2012;6:e1446. [Europe PMC free article] [Abstract] [Google Scholar]
- Economopoulou A, Dominguez M, Helynck B, Sissoko D, Wichmann O, Quenel P, Germonneau P, Quatresous I. Atypical Chikungunya virus infections: clinical manifestations, mortality and risk factors for severe disease during the 2005–2006 outbreak on Reunion. Epidemiology and infection. 2009;137:534–541. [Abstract] [Google Scholar]
- Eros G, Ibrahim S, Siebert N, Boros M, Vollmar B. Oral phosphatidylcholine pretreatment alleviates the signs of experimental rheumatoid arthritis. Arthritis research & therapy. 2009;11:R43. [Europe PMC free article] [Abstract] [Google Scholar]
- Fros JJ, Liu WJ, Prow NA, Geertsema C, Ligtenberg M, Vanlandingham DL, Schnettler E, Vlak JM, Suhrbier A, Khromykh AA, Pijlman GP. Chikungunya virus nonstructural protein 2 inhibits type I/II interferon-stimulated JAK-STAT signaling. Journal of virology. 2010;84:10877–10887. [Europe PMC free article] [Abstract] [Google Scholar]
- Gardner J, Anraku I, Le TT, Larcher T, Major L, Roques P, Schroder WA, Higgs S, Suhrbier A. Chikungunya virus arthritis in adult wild-type mice. J Virol. 2010;84:8021–8032. [Europe PMC free article] [Abstract] [Google Scholar]
- Gerardin P, Barau G, Michault A, Bintner M, Randrianaivo H, Choker G, Lenglet Y, Touret Y, Bouveret A, Grivard P, Le Roux K, Blanc S, Schuffenecker I, Couderc T, Arenzana-Seisdedos F, Lecuit M, Robillard PY. Multidisciplinary prospective study of mother-to-child chikungunya virus infections on the island of La Reunion. PLoS medicine. 2008;5:e60. [Europe PMC free article] [Abstract] [Google Scholar]
- Havenar-Daughton C, Kolumam GA, Murali-Krishna K. Cutting Edge: The direct action of type I IFN on CD4 T cells is critical for sustaining clonal expansion in response to a viral but not a bacterial infection. J Immunol. 2006;176:3315–3319. [Abstract] [Google Scholar]
- Higgs S, Ziegler SA. A nonhuman primate model of chikungunya disease. The Journal of clinical investigation. 2010;120:657–660. [Europe PMC free article] [Abstract] [Google Scholar]
- Jaffar-Bandjee MC, Gasque P. Physiopathology of chronic arthritis following chikungunya infection in man. Medecine tropicale : revue du Corps de sante colonial. 2012;72:86–87. [Abstract] [Google Scholar]
- Julander JG, Ennis J, Turner J, Morrey JD. Treatment of yellow fever virus with an adenovirus-vectored interferon, DEF201, in a hamster model. Antimicrobial agents and chemotherapy. 2011;55:2067–2073. [Europe PMC free article] [Abstract] [Google Scholar]
- Kennedy AC, Fleming J, Solomon L. Chikungunya viral arthropathy: a clinical description. The Journal of rheumatology. 1980;7:231–236. [Abstract] [Google Scholar]
- Labadie K, Larcher T, Joubert C, Mannioui A, Delache B, Brochard P, Guigand L, Dubreil L, Lebon P, Verrier B, de Lamballerie X, Suhrbier A, Cherel Y, Le Grand R, Roques P. Chikungunya disease in nonhuman primates involves long-term viral persistence in macrophages. J Clin Invest. 2010;120:894–906. [Europe PMC free article] [Abstract] [Google Scholar]
- Lohachanakul J, Phuklia W, Thannagith M, Thonsakulprasert T, Ubol S. High concentrations of circulating interleukin-6 and monocyte chemotactic protein-1 with low concentrations of interleukin-8 were associated with severe chikungunya fever during the 2009–2010 outbreak in Thailand. Microbiology and immunology. 2012;56:134–138. [Abstract] [Google Scholar]
- Morrison TE, Oko L, Montgomery SA, Whitmore AC, Lotstein AR, Gunn BM, Elmore SA, Heise MT. A mouse model of chikungunya virus-induced musculoskeletal inflammatory disease: evidence of arthritis, tenosynovitis, myositis, and persistence. The American journal of pathology. 2011;178:32–40. [Europe PMC free article] [Abstract] [Google Scholar]
- Nishida S, Tsurukami H, Sakai A, Sakata T, Ikeda S, Tanaka M, Ito M, Nakamura T. Stage-dependent changes in trabecular bone turnover and osteogenic capacity of marrow cells during development of type II collagen-induced arthritis in mice. Bone. 2002;30:872–879. [Abstract] [Google Scholar]
- Partidos CD, Weger J, Brewoo J, Seymour R, Borland EM, Ledermann JP, Powers AM, Weaver SC, Stinchcomb DT, Osorio JE. Probing the attenuation and protective efficacy of a candidate chikungunya virus vaccine in mice with compromised interferon (IFN) signaling. Vaccine. 2011;29:3067–3073. [Europe PMC free article] [Abstract] [Google Scholar]
- Ramful D, Carbonnier M, Pasquet M, Bouhmani B, Ghazouani J, Noormahomed T, Beullier G, Attali T, Samperiz S, Fourmaintraux A, Alessandri JL. Mother-to-child transmission of Chikungunya virus infection. The Pediatric infectious disease journal. 2007;26:811–815. [Abstract] [Google Scholar]
- Reed LJ, Muench CH. A simple method of estimating fifty percent endpoint. Am J Hyg. 1938;27:493–497. [Google Scholar]
- Robin S, Ramful D, Le Seach F, Jaffar-Bandjee MC, Rigou G, Alessandri JL. Neurologic manifestations of pediatric chikungunya infection. Journal of child neurology. 2008;23:1028–1035. [Abstract] [Google Scholar]
- Schilte C, Couderc T, Chretien F, Sourisseau M, Gangneux N, Guivel-Benhassine F, Kraxner A, Tschopp J, Higgs S, Michault A, Arenzana-Seisdedos F, Colonna M, Peduto L, Schwartz O, Lecuit M, Albert ML. Type I IFN controls chikungunya virus via its action on nonhematopoietic cells. The Journal of experimental medicine. 2010;207:429–442. [Europe PMC free article] [Abstract] [Google Scholar]
- Schmidt-Chanasit J, Schmiedel S, Fleischer B, Burchard GD. Viruses acquired abroad: what does the primary care physician need to know? Deutsches Arzteblatt international. 2012;109:681–691. quiz 692. [Europe PMC free article] [Abstract] [Google Scholar]
- Sissoko D, Malvy D, Ezzedine K, Renault P, Moscetti F, Ledrans M, Pierre V. Post-epidemic Chikungunya disease on Reunion Island: course of rheumatic manifestations and associated factors over a 15-month period. PLoS neglected tropical diseases. 2009;3:e389. [Europe PMC free article] [Abstract] [Google Scholar]
- Suhrbier A, Mahalingam S. The immunobiology of viral arthritides. Pharmacol Ther. 2009;124:301–308. [Abstract] [Google Scholar]
- Thiberville SD, Moyen N, Dupuis-Maguiraga L, Antoine N, Gould EA, Roques P, de Lamballerie X. Chikungunya fever: Epidemiology, clinical syndrome, pathogenesis and therapy. Antiviral research. 2013;99:345–370. [Europe PMC free article] [Abstract] [Google Scholar]
- Tsetsarkin K, Higgs S, McGee CE, De Lamballerie X, Charrel RN, Vanlandingham DL. Infectious clones of Chikungunya virus (La Reunion isolate) for vector competence studies. Vector Borne Zoonotic Dis. 2006;6:325–337. [Abstract] [Google Scholar]
- Wang D, Suhrbier A, Penn-Nicholson A, Woraratanadharm J, Gardner J, Luo M, Le TT, Anraku I, Sakalian M, Einfeld D, Dong JY. A complex adenovirus vaccine against chikungunya virus provides complete protection against viraemia and arthritis. Vaccine. 2011;29:2803–2809. [Europe PMC free article] [Abstract] [Google Scholar]
- Wu JQ, Barabe ND, Huang YM, Rayner GA, Christopher ME, Schmaltz FL. Pre- and post-exposure protection against Western equine encephalitis virus after single inoculation with adenovirus vector expressing interferon alpha. Virology. 2007;369:206–213. [Abstract] [Google Scholar]
Full text links
Read article at publisher's site: https://doi.org/10.1016/j.antiviral.2014.05.004
Read article for free, from open access legal sources, via Unpaywall:
https://europepmc.org/articles/pmc4101997?pdf=render
Citations & impact
Impact metrics
Article citations
Mayaro virus pathogenesis and immunity in rhesus macaques.
PLoS Negl Trop Dis, 17(11):e0011742, 20 Nov 2023
Cited by: 2 articles | PMID: 37983245 | PMCID: PMC10695392
Equine Polyclonal Antibodies Prevent Acute Chikungunya Virus Infection in Mice.
Viruses, 15(7):1479, 29 Jun 2023
Cited by: 0 articles | PMID: 37515166 | PMCID: PMC10384969
Post-Viral Pain, Fatigue, and Sleep Disturbance Syndromes: Current Knowledge and Future Directions.
Can J Pain, 7(2):2272999, 01 Jan 2023
Cited by: 3 articles | PMID: 38239826 | PMCID: PMC10795785
Review Free full text in Europe PMC
Non-replicating adenovirus based Mayaro virus vaccine elicits protective immune responses and cross protects against other alphaviruses.
PLoS Negl Trop Dis, 15(4):e0009308, 01 Apr 2021
Cited by: 10 articles | PMID: 33793555 | PMCID: PMC8051823
Adenovirus vectored IFN-α protects mice from lethal challenge of Chikungunya virus infection.
PLoS Negl Trop Dis, 14(12):e0008910, 03 Dec 2020
Cited by: 5 articles | PMID: 33270642 | PMCID: PMC7738163
Go to all (10) article citations
Similar Articles
To arrive at the top five similar articles we use a word-weighted algorithm to compare words from the Title and Abstract of each citation.
Adenovirus vectored IFN-α protects mice from lethal challenge of Chikungunya virus infection.
PLoS Negl Trop Dis, 14(12):e0008910, 03 Dec 2020
Cited by: 5 articles | PMID: 33270642 | PMCID: PMC7738163
A high-dose inoculum size results in persistent viral infection and arthritis in mice infected with chikungunya virus.
PLoS Negl Trop Dis, 16(1):e0010149, 31 Jan 2022
Cited by: 5 articles | PMID: 35100271 | PMCID: PMC8803182
Therapy and long-term prophylaxis of vaccinia virus respiratory infections in mice with an adenovirus-vectored interferon alpha (mDEF201).
PLoS One, 6(10):e26330, 13 Oct 2011
Cited by: 7 articles | PMID: 22022603 | PMCID: PMC3192798
Chikungunya and arthritis: An overview.
Travel Med Infect Dis, 44:102168, 23 Sep 2021
Cited by: 16 articles | PMID: 34563686
Review
Funding
Funders who supported this work.
NIAID NIH HHS (2)
Grant ID: HHSN272201000039I
Grant ID: N01 AI015435