Abstract
Background
Artemisinin resistance in Plasmodium falciparum has emerged in Southeast Asia and now poses a threat to the control and elimination of malaria. Mapping the geographic extent of resistance is essential for planning containment and elimination strategies.Methods
Between May 2011 and April 2013, we enrolled 1241 adults and children with acute, uncomplicated falciparum malaria in an open-label trial at 15 sites in 10 countries (7 in Asia and 3 in Africa). Patients received artesunate, administered orally at a daily dose of either 2 mg per kilogram of body weight per day or 4 mg per kilogram, for 3 days, followed by a standard 3-day course of artemisinin-based combination therapy. Parasite counts in peripheral-blood samples were measured every 6 hours, and the parasite clearance half-lives were determined.Results
The median parasite clearance half-lives ranged from 1.9 hours in the Democratic Republic of Congo to 7.0 hours at the Thailand-Cambodia border. Slowly clearing infections (parasite clearance half-life >5 hours), strongly associated with single point mutations in the "propeller" region of the P. falciparum kelch protein gene on chromosome 13 (kelch13), were detected throughout mainland Southeast Asia from southern Vietnam to central Myanmar. The incidence of pretreatment and post-treatment gametocytemia was higher among patients with slow parasite clearance, suggesting greater potential for transmission. In western Cambodia, where artemisinin-based combination therapies are failing, the 6-day course of antimalarial therapy was associated with a cure rate of 97.7% (95% confidence interval, 90.9 to 99.4) at 42 days.Conclusions
Artemisinin resistance to P. falciparum, which is now prevalent across mainland Southeast Asia, is associated with mutations in kelch13. Prolonged courses of artemisinin-based combination therapies are currently efficacious in areas where standard 3-day treatments are failing. (Funded by the U.K. Department of International Development and others; ClinicalTrials.gov number, NCT01350856.).Free full text

Spread of Artemisinin Resistance in Plasmodium falciparum Malaria
Abstract
BACKGROUND
Artemisinin resistance in Plasmodium falciparum has emerged in Southeast Asia and now poses a threat to the control and elimination of malaria. Mapping the geographic extent of resistance is essential for planning containment and elimination strategies.
METHODS
Between May 2011 and April 2013, we enrolled 1241 adults and children with acute, uncomplicated falciparum malaria in an open-label trial at 15 sites in 10 countries (7 in Asia and 3 in Africa). Patients received artesunate, administered orally at a daily dose of either 2 mg per kilogram of body weight per day or 4 mg per kilogram, for 3 days, followed by a standard 3-day course of artemisinin-based combination therapy. Parasite counts in peripheral-blood samples were measured every 6 hours, and the parasite clearance half-lives were determined.
RESULTS
The median parasite clearance half-lives ranged from 1.9 hours in the Democratic Republic of Congo to 7.0 hours at the Thailand–Cambodia border. Slowly clearing in fections (parasite clearance half-life >5 hours), strongly associated with single point mutations in the “propeller” region of the P. falciparum kelch protein gene on chromosome 13 (kelch13), were detected throughout mainland Southeast Asia from southern Vietnam to central Myanmar. The incidence of pretreatment and post-treatment gametocytemia was higher among patients with slow parasite clearance, suggesting greater potential for transmission. In western Cambodia, where artemisinin-based combination therapies are failing, the 6-day course of antimalarial therapy was associated with a cure rate of 97.7% (95% confidence interval, 90.9 to 99.4) at 42 days.
CONCLUSIONS
Artemisinin resistance to P. falciparum, which is now prevalent across mainland Southeast Asia, is associated with mutations in kelch13. Prolonged courses of artemisinin-based combination therapies are currently efficacious in areas where standard 3-day treatments are failing. (Funded by the U.K. Department of International Development and others; ClinicalTrials.gov number, NCT01350856.)
Artemisinin derivatives are highly potent, rapidly eliminated antimalarial drugs with a broad stage specificity of action. They clear parasitemia more rapidly than all other currently available antimalarial agents. In the 1990s, resistance to available antimalarial drugs such as chloroquine and sulfadoxine–pyrimethamine worsened across areas of the world where malaria is endemic. As a direct consequence, morbidity and mortality associated with malaria increased, especially among African children, who account for most deaths from malaria.1 The artemisinin-based combination therapies were introduced in the mid-1990s, when there was an imminent prospect of untreatable malaria in Southeast Asia, where resistance to all available antimalarial drugs had developed. In 2005, the World Health Organization (WHO) recommended that artemisinin-based com bination therapies be used as first-line treatments for falciparum malaria in all countries where malaria was endemic. Recent, marked increases in the availability and use of artemisinin-based combination therapies, together with the increased use of insecticide-treated bed nets, have substantially reduced global morbidity and mortality from malaria.2 These gains, and the prospects for the elimination of malaria, are now threatened by the emergence of artemisinin resistance in Plasmodium falciparum. Artemisinin resistance is characterized by slow parasite clearance,3,4 which reflects the reduced susceptibility of ring-stage parasites.5-9 It has recently been linked with point mutations in the “propeller” region of a P. falciparum kelch protein.10
Artemisinin resistance was reported first in western Cambodia,3,4 where failure rates for artemisinin-based combination therapies are rapidly increasing11 and where resistance to previous first-line antimalarial drugs also first emerged. Artemisinin resistance has since spread, emerged independently, or both in other areas of mainland Southeast Asia.12-15 On the Thailand–Myanmar border, the geometric mean half-life for parasite clearance increased from 2.6 hours in 2001 to 3.7 hours in 2010, as compared with a half-life of 5.5 hours in western Cambodia between 2007 and 2010.12 Defining the extent and severity of artemisinin resistance is essential for planning containment and elimination strategies.
The Tracking Resistance to Artemisinin Collaboration was created in 2011 to provide evidence and tools to halt or slow the spread of artemisinin resistance. The principal objective of our clinical research was to map the current extent and severity of artemisinin resistance.
METHODS
STUDY DESIGN AND OVERSIGHT
We conducted an open-label, randomized trial at 15 sites in 10 countries (Fig. 1): Cambodia (4 sites), Thailand (3 sites), and Laos, Vietnam, Myanmar, Bangladesh, India, Nigeria, Kenya, and the Democratic Republic of Congo (1 site each). The study was coordinated by the Mahidol–Oxford Tropical Medicine Research Unit (MORU) in Bangkok.
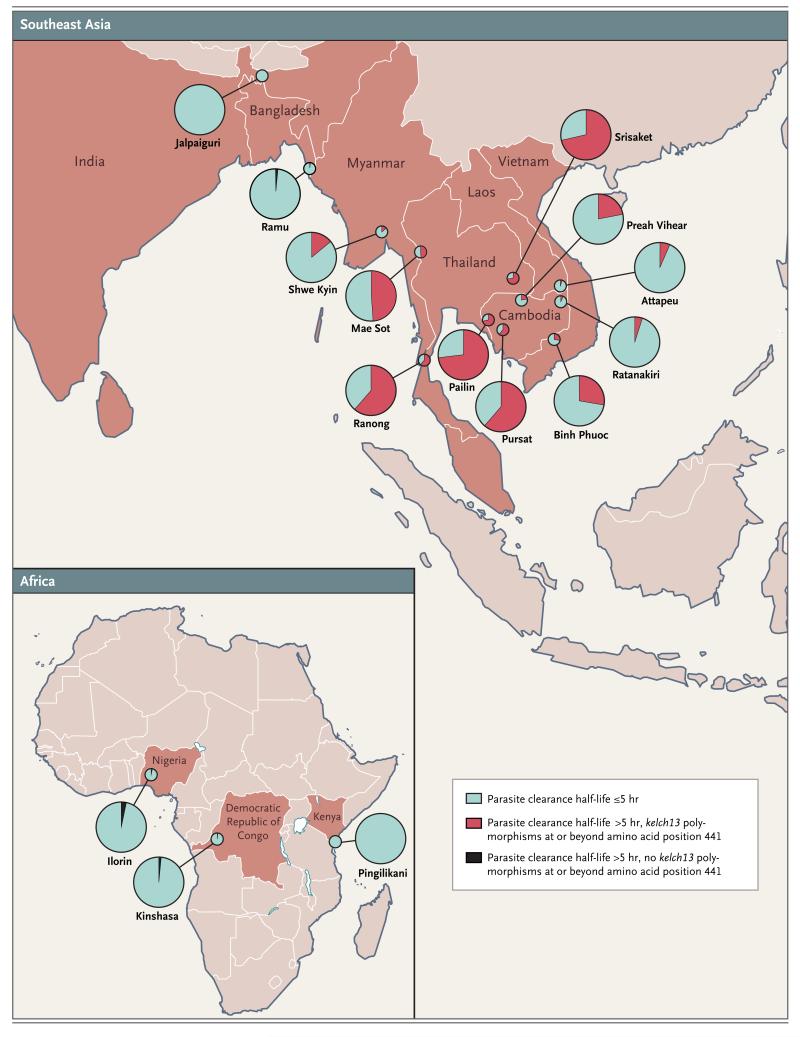
Artemisinin resistance was defined by a parasite clearance half-life longer than 5 hours, with some Plasmodium falciparum isolates having kelch13 polymorphisms (at or beyond amino acid position 441).
The protocol (available with the full text of this article at NEJM.org) was approved by the Oxford Tropical Research Ethics Committee and the institutional review board, national ethics committee, or both for each site. The trial was monitored by the MORU Clinical Trials Support Group, in collaboration with the Oxford University Clinical Research Unit–Vietnam and the Kenya Medical Research Institute–Wellcome Trust Clinical Trials Facility. All serious adverse events were reported to an independent data and safety monitoring committee. Quality-assured artesunate manufactured by Guilin Pharmaceutical was provided by the WHO Global Malaria Programme, and artemisinin-based combination therapies were provided free of charge by Beijing Holley-Cotec Pharmaceuticals and Sigma-Tau Pharmaceuticals; these companies did not play a role in the study design, data collection or analysis, or writing of the manuscript.
STUDY SITES AND PATIENTS
Patients were eligible for enrollment in the study if they were 6 months to 65 years of age and had acute, uncomplicated falciparum malaria (including mixed infections with non-falciparum species), parasitemia with a parasite count between 10,000 and 200,000 per cubic millimeter, and fever (a tympanic temperature >37.5°C) or a history of fever. Patients or parents or guardians of minors who were unable to read or write provided oral informed consent in the presence of an impartial witness. Assent was obtained from minors older than 10 years of age. All other patients provided written informed consent. The main exclusion criteria were receipt of an artemisinin antimalarial drug in the previous week, pregnancy or breast-feeding, and a low hematocrit (<25% in Asia and <15% in Africa).
DRUG THERAPY AND FOLLOW-UP ASSESSMENTS
At most sites, patients were randomly assigned in blocks of 20 to receive artesunate (Guilin Pharmaceutical), administered orally at a dose of either 2 mg per kilogram of body weight per day or 4 mg per kilogram per day, for 3 days, followed by a standard 3-day course of an artemisinin-based combination therapy (dihydroartemisinin–piperaquine, artemether–lumefantrine, artesunate–sulfadoxine–pyrimethamine, or artesunate-mefloquine, according to local treatment policies). Treatment assignments were concealed in numbered opaque envelopes, which were opened sequentially. In western Cambodia, and Srisaket, Thailand, where higher-grade artemisinin resistance was already established, only the higher dose of artesunate (4 mg per kilogram per day) was evaluated. In response to review by local scientific or ethics committees, the protocol was modified as follows: in India, only the higher dose of artesunate was studied, patients in the Democratic Republic of Congo received either artemether-lumefantrine alone or artesunate at a dose of 4 mg per kilogram per day for 3 days, followed by artemether-lumefantrine, and in Kenya, artesunate was administered at a daily dose of 2 mg per kilogram per day for 7 days.
Patients were admitted to the hospital for supervised treatment. Body temperature and hematocrit were measured and blood smears for parasite counts were obtained at 0, 4, 6, 8, and 12 hours and then every 6 hours thereafter until two consecutive slides were reported as negative.
Follow-up assessments were performed on days 7 and 14 at all study sites; patients were followed weekly for 28 or 42 days at six sites. For recurrent infections, polymerase-chain-reaction (PCR) genotyping was performed with the use of msp1, msp2, and glurp as genetic markers to distinguish a recrudescence from a newly acquired infection.16 In Kenya, only msp2 was used for genotyping.17
GENETIC MARKERS OF ARTEMISININ RESISTANCE
After PCR amplification, the full sequence of kelch13 was determined from P. falciparum isolates obtained at admission (see the Supplementary Appendix, available at NEJM.org).10
MICROSCOPY QUALITY-CONTROL MEASURES
Quality control of microscopy throughout the study was performed by experienced microscopists at four of the study sites. All slides obtained at admission and at 72 hours were rechecked. Complete patient slide sets were rechecked if the standard deviation of residuals for the fit to the linear segment of the parasite-time profile (see below) was greater than 1, the estimated lag phase was longer than 12 hours, the clearance estimate was based on two positive counts, or the last positive parasite count was greater than 1000 per cubic millimeter.
STUDY OUTCOMES
The primary outcome was the parasite clearance half-life, a measure of the parasite clearance rate derived from the linear segment of the log parasitemia–time curve (parasite clearance half-life = loge2 divided by the parasite clearance rate).18-20 Secondary outcomes were the proportions of patients with a parasite clearance half-life of more than 5 hours, parasitemia detected on microscopy21 on day 3, gametocytemia after treatment, the incidence of anemia, and the efficacy of the 6-day artesunate regimen plus artemisinin-based combination therapy, corrected by means of PCR genotyping.17
STATISTICAL ANALYSIS
Sample-size calculations were based on parasite clearance rates among 1952 patients treated with oral artesunate between 2001 and 2010 in northwest Thailand,12 which had a log-normal distribution, with geometric mean clearance rates between 0.19 and 0.24 per hour and log-scale standard deviations between 0.40 and 0.55 (coefficient of variation on the original scale, 43 to 49%). On the basis of the log-linear component of the parasitemia–time slope, we calculated that a sample of 50 patients per group at each site would provide 12, 15, and 17% precision and 80% power to detect a 20%, 25%, and 27% decrease in the geometric mean of parasite clearance rates for rates between 0.17 and 0.30 per hour, assuming log-transformed standard-deviation values of 0.40, 0.50, and 0.55, respectively. We calculated that we would need to enroll 60 patients per group to allow for a 10% loss to follow-up or incomplete data.
Data were double-entered into a Web-based database (OpenClinica, version 3.0) and cleaned and analyzed with the use of Stata software, version 13 (StataCorp). Parasite clearance was assessed with the use of the WorldWide Antimalarial Resistance Network Parasite Clearance Estimator.19,20 Half-life values were excluded from numerical comparisons in 14 patients, since these values were based on only two data points. The relationships between kelch13 mutations and parasite clearance measures were assessed by means of multivariable analysis. A parasite clearance half-life longer than 5.0 hours was considered prospectively to indicate artemisinin resistance on the basis of data from the Thailand–Myanmar border (where this was the 90th centile for parasite clearance half-life in 2004, when resistance had just emerged).12 Resolution of fever and treatment efficacy corrected by means of PCR genotyping were estimated with the use of Kaplan–Meier survival analysis.22,23 A meta-analysis of the differences in mean log-transformed parasite clearance half-life values between treatment groups was performed according to study site with the use of the inverse-variance method and a fixed-effects model. Heterogeneity was evaluated with the use of the I2 statistic.24 All comparisons were stratified according to study site with the use of random-effects or fixed-effects models. Anemia was defined as a hemoglobin level below 11 g per deciliter (<10 g per deciliter in children younger than 5 years of age). Full details of the study conduct and analyses are provided in the protocol with the statistical analysis plan.
RESULTS
STUDY PATIENTS
Between May 2011 and April 2013, a total of 18,865 patients were screened and 1241 patients with falciparum malaria were enrolled, of whom 829 (67%) were febrile on admission. The coincident strengthening of efforts to control malaria and the decreasing incidence of malaria prevented 7 of the 15 sites from meeting recruitment targets. The majority of patients were men (74%). The median age was 21 years (interquartile range, 11 to 31) (Table 1).
Table 1
Study Site | No. of Patients | Artesunate | Artemisinin-Based Combination Therapy | Male Sex | Weight | Median Age (range) | Median Duration of Fever (range) | Parasite Count (range) | Gametocytemia on Day 0 | Mixed Infection | Hematocrit |
---|---|---|---|---|---|---|---|---|---|---|---|
mg/kg | no. of patients (%) | kg | yr | days | no./mm3 | no. of patients/total no. (%) | no. of patients (%) | % | |||
All sites | 1241 | 920 (74) | 42±17 | 21 (11–31) | 3 (2–4) | 52,250 (2560–605,329) | 125/1225 (10) | 67 (5) | 37±6.5 | ||
Jalpaiguri, India | 1 | 4 | AS-SP | 1 | 49 | 38 | — | 93,600 | 0/1 | 0 | 30 |
Ramu, Bangladesh | 56 | 2 or 4 | AL | 48 (86) | 46±10 | 26 (19–30) | 4 (3–5) | 27,130 (10,048–224,196) | 0/56 (0) | 0 | 41±4.3 |
Shwe Kyin, Myanmar | 80 | 2 or 4 | AL | 66 (82) | 52±7.6 | 24 (19–31.5) | 3 (2–4) | 66,066 (10,640–420,006) | 9/80 (11) | 4(5) | 39±5.7 |
Thailand | |||||||||||
![]() | 120 | 2 or 4 | AS-MQ | 94 (78) | 52±6.8 | 29 (22.5–37) | 3 (2–4) | 38,685 (2560–327,062) | 11/118 (9) | 5 (4) | 38±6 |
![]() | 41 | 4 | AS-MQ | 41 (100) | 58±7.5 | 29 (22–38) | 3 (2–4) | 31,902 (4346–192,997) | 1/38 (3) | 3 (7) | 41±5.4 |
![]() | 24 | 2 or 4 | AS-MQ | 16 (67) | 59±8.4 | 33 (26–39) | 3 (2–4) | 38,999 (5903–94,451) | 0/23 | 0 | 39±4.5 |
Attapeu, Laos | 120 | 2 or 4 | AL | 85 (71) | 43±14 | 20.5 (13.5–28) | 3 (3–4) | 46,346 (10,048–198,574) | 6/120 (5) | 4(3) | 38±4.1 |
Cambodia | |||||||||||
![]() | 100 | 4 | DP | 87 (87) | 52±9.6 | 25 (19–37.5) | 2.5 (1.5–3.5) | 45,216 (3712–389,988) | 19/100 (19) | 16 (16) | 39±5.1 |
![]() | 120 | 2 or 4 | DP | 82 (68) | 42±14 | 20 (14–29) | 2.8 (2.0–3.0) | 56,583 (13,942–311,237) | 6/120 (5) | 2 (2) | 37±5.8 |
![]() | 120 | 2 or 4 | DP | 78 (65) | 35±15.2 | 14 (9–19.5) | 2 (2–3) | 62,109 (5024–310,860) | 7/120 (6) | 19 (16) | 37±5.7 |
![]() | 120 | 4 | DP | 109 (91) | 50±12.3 | 25 (19–33.5) | 3 (3–4) | 56,583 (9797–284,861) | 22/120 (18) | 4 (3) | 39±5.7 |
Binh Phuoc, Vietnam | 120 | 2 or 4 | DP | 92 (77) | 51±11.3 | 26 (18.5–38.5) | 2 (2–3) | 49,738 (9797–205,230) | 7/119 (6) | 9 (8) | 38±4.5 |
ilorin, Nigeria | 40 | 2 or 4 | AL | 29 (72) | 16±7.1 | 3 (2–6) | 2 (1–3) | 52,250 (3391–219,549) | 1/35 (3) | 1 (2) | 32±4.7 |
Kinshasa, Democratic Republic of Congo | 120 | 4 or 0 | AL | 63 (52) | 15±5 | 5 (3–6) | 3 (2–4) | 60,037 (11,555–60,5329) | 36/117 (31) | 0 | 31±4.7 |
Pingilikani, Kenya | 59 | 2 | — | 28 (47) | 15±4.7 | 5 (3–8) | 2 (1–3) | 91,562 (16,579–488,333) | 0/58 | 0 | 30±4.8 |
PARASITE CLEARANCE
There were marked differences in therapeutic responses across the Southeast Asian sites. Median parasite clearance half-life values ranged from 2 hours in Attapeu, Laos, to 7 hours in Srisaket, Thailand (approximately 250 km [155 mi] to the west) (Table 2). There was a gradient of prolonged parasite clearance, with the highest proportion of patients with a prolonged parasite clearance half-life (>5 hours) in western Cambodia and eastern Thailand (49 to 73%), as compared with 14 to 28% in northern Cambodia, Vietnam, and eastern Myanmar, and very low proportions elsewhere. (Fig. 1 and and22 and Table 2). The proportions of patients with parasitemia detectable on microscopy at 72 hours (day 3), a widely used criterion for artemisinin resistance,21 ranged from 0% in Kenya to 68% in eastern Thailand (Table 2). Among the African sites, median parasite clearance half-life values were similar in Kenya and Nigeria (P = 0.75) but were significantly shorter in the Democratic Republic of Congo than in these two African sites (P<0.001) (Fig. 2 and Table 2).
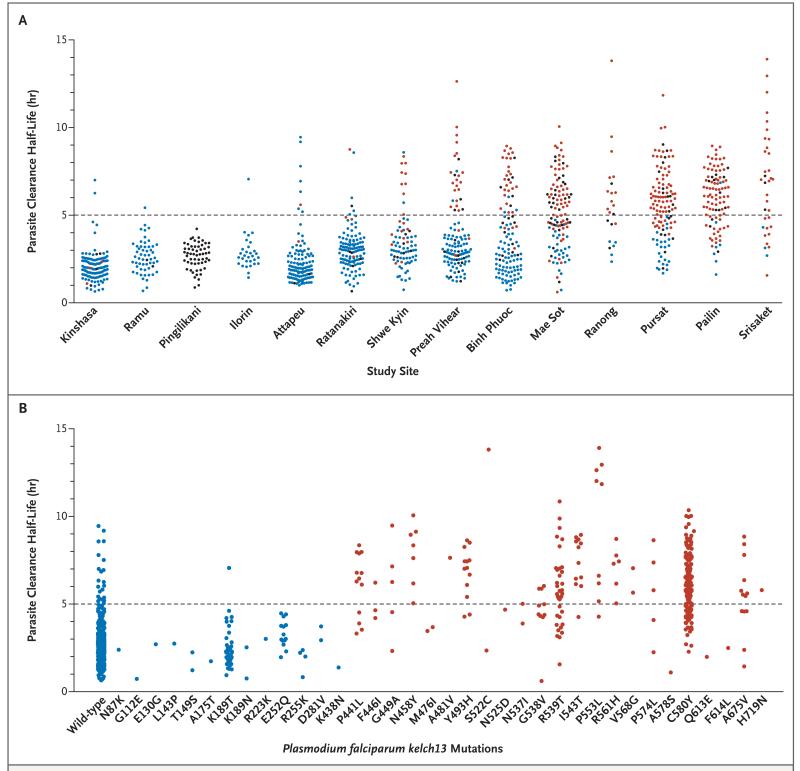
Panel A shows the parasite clearance half-life according to study site (treatment groups have been pooled in all sites), and Panel B shows the location of P. falciparum kelch13 nonsynonymous polymorphisms. One circle represents one patient. Infections with mutations in the P. falciparum kelch13 are indicated by blue circles (wild-type or mutation before amino acid position 441), red circles (mutation after position 440), or black circles (at least part of kelch13 sequence is missing or heterozygous). Two patients had double mutations (n87k and k92n, and p441l and n725y); the site of the first mutation is shown.
Table 2
Study Site | Median Time to Resolution of Fever (IQR) | Median Parasite Clearance Half-Life (range) | Median Time to 50% Parasite Clearance (range) | Median Time to 90% Parasite Clearance (range) | Parasite Clearance Half-Life >5 Hr | Positive for Parasitemia on Day 3 | Nonsynonymous SNPs kelchl3 after Position 440† |
---|---|---|---|---|---|---|---|
hours | no. of patients/total no. (%) | ||||||
Jalpaiguri, India | 12 | 2.8 | 8.2 | 11.9 | 0 | 0/1 | — |
Ramu, Bangladesh | 12 (4–18) | 2.5 (0.7–5.4) | 5 (2.1–12.1) | 11 (3.7–22.4) | 1/55 (2) | 1/53 (2) | 0/55 |
Shwe Kyin, Myanmar | 6 (4–18) | 3.1 (1.3–8.6) | 7.4 (2.3–22.1) | 15.7 (4.6–39.7) | 11/79 (14) | 7/80 (9) | 19/73 (26) |
Thailand | |||||||
![]() | 24 (8–36) | 4.9 (0.6–10.1) | 7.9 (2.0–27.5) | 20.8 (3.7–50.4) | 57/117 (49) | 41/118 (35) | 60/89 (67) |
![]() | 36 (12–36) | 7.0 (1.6–13.9) | 7.7 (2.1–27.3) | 28 (3.9–60.6) | 23/35 (66) | 23/34 (68) | 30/33 (91) |
![]() | 36 (30–36) | 5.4 (2.4–13.8) | 8 (2.9–18.9) | 29.7 (11.6–44.2) | 14/23 (61) | 11/22 (50) | 13/18 (72) |
Attapeu, Laos | 18 (7–31) | 2 (1.1–9.5) | 7.9 (2.4–23.1) | 13.7 (5.4–28.8) | 7/108 (6) | 6/115 (5) | 2/116 (2) |
Cambodia | |||||||
![]() | 36 (24–60) | 6.1 (2.4–9.0) | 9.5 (2.1–22.3) | 26.4 (3.7–47.4) | 71/97 (73) | 65/98 (66) | 78/85 (92) |
![]() | 18 (6–24) | 3.0 (1.2–12.6) | 7.7 (2.0–42.6) | 16.2 (3.6–59.5) | 26/120 (22) | 21/120 (18) | 22/104 (21) |
![]() | 12 (6–18) | 3 (0.7–8.8) | 6.9 (2.0–26.0) | 15.5 (3.6–36.3) | 6/119 (5) | 8/119 (7) | 4/114 (4) |
![]() | 36 (24–42) | 5.6 (1.7–11.8) | 9.6 (2.3–32.1) | 25.7 (6.4–66.5) | 73/119 (61) | 71/118 (60) | 76/100 (76) |
Binh Phuoc, Vietnam | 18 (8–30) | 3.1 (0.7–8.9) | 8.5 (2.0–33.3) | 16.4 (3.6–53.3) | 33/118 (28) | 32/118 (27) | 28/102 (27) |
ilorin, Nigeria | 8 (4–18) | 2.6 (1.4–7.1) | 6.8 (2.7–22.0) | 13.8 (5.7–33.3) | 1/29 (3) | 1/27 (4) | 0/30 |
Democratic Republic of Congo | |||||||
![]() | 4 (4–12) | 1.9 (0.7–7.0) | 6.0 (2.0–16.0) | 11.3 (3.6–20.0) | 2/59 (3) | 1/59 (2) | 1/58 (2) |
![]() | 12 (4–24) | 2.2 (1.2–4.6) | 8.9 (2.3–18.8) | 16.7 (4.6–23.6) | 0/57 | 0/57 | 2/56 (4) |
Pingilikani, Kenya | 18 (4–24) | 2.8 (0.9–4.2) | 5.3 (1.3–13) | 11.6 (3.4–27.5) | 0/56 | 0/46 | — |
Geometric mean parasite clearance half-life values were similar in the group that received artesunate at a dose of 2 mg per kilogram and the group that received artesunate at a dose of 4 mg per kilogram; the overall difference, stratified according to site, was −4.9% (95% confidence interval [CI], −10.7 to 1.4; P = 0.13). In Kin shasa, median parasite clearance half-life values were significantly longer with artemether–lumefantrine than with artesunate at a dose of 4 mg per kilogram (2.2 hours [range, 1.2 to 4.6] vs. 1.9 hours [range, 0.7 to 7.0]; P = 0.003).
GENETIC CORRELATES OF SLOW PARASITE CLEARANCE
Single point mutations in the propeller domain of kelch13 after position 440 were associated with a mean increase in the parasite clearance half-life of 116% (95% CI, 103 to 131; P<0.001) (Fig. 2). In a multivariate logistic-regression model, with adjustment for age, baseline parasite count, and dose of artesunate, an infection associated with a kelch13 propeller mutation was substantially more likely to have a parasite clearance half-life longer than 5 hours (odds ratio, 94.7; 95% CI, 54.6 to 164.0; P<0.001). In this study, kelch13 propeller mutations had 91.8% sensitivity and 88.4% specificity in identifying a parasite clearance half-life longer than 5 hours. Among infections with wild-type kelch13 alleles, 3.4% (21 of 611) had a parasite clearance half-life longer than 5 hours. The parasite clearance half-life values for mutant infections were generally similar, except that the values associated with G538V were lower and those associated with I543T and N458Y were higher than the half-life values associated with the other mutations (P = 0.02).
GAMETOCYTE CARRIAGE
Patent gametocytemia (gametocyte densities above the microscopy level of detection) was reported in 125 patients at enrollment, with the highest rates in the Democratic Republic of Congo (31%) and western Cambodia (19% in Pailin and 18% in Pursat). The median duration of patent gametocytemia was 6.7 days (range, <6 hours to 10.5 days); it did not differ significantly between the sites (P = 0.36) and was not correlated with the parasite clearance half-life. Gametocytemia developed in an additional 48 patients within 24 hours and in another 47 patients between day 1 and day 7 (median time to appearance, 42 hours; range, 24 to 169). The prevalence of gametocytemia at enrollment was higher among patients at sites with slow parasite clearance. Excluding the Democratic Republic of Congo, which has substantially higher malaria transmission intensity than the other sites, patent gametocytemia was also more likely to develop in patients with slower parasite clearance after treatment (odds ratio for doubling of the parasite clearance half-life, 2.40; 95% CI, 1.53 to 3.76; P<0.001).
HEMATOLOGIC FINDINGS
More than half the African children enrolled in the study had anemia, as compared with 8 to 37% of Asian patients. The lowest hematocrit occurred on day 3, with partial recovery by day 14. Four patients (one child in the Democratic Republic of Congo and three adults in Thailand) received a blood transfusion during the first week. After adjustment for the baseline hematocrit, baseline parasite count, age, and the total dose of the artemisinin derivative received, delayed recovery from anemia on day 21 was associated with slow parasite clearance (odds ratio for anemia with a doubling of the parasite clearance half-life, 1.53; 95% CI, 1.06 to 2.21; P = 0.02), but there was no association by day 28. Apart from the baseline hematocrit, other covariates associated with anemia on day 21 were age (odds ratio with a doubling of age, 1.53; 95% CI, 1.12 to 2.11; P = 0.008) and initial parasite count (odds ratio with an increase in the count by a factor of 10, 2.09; 95% CI, 1.00 to 4.35; P = 0.05). The development of anemia was not associated with the dose of the artemisinin derivative.
TREATMENT EFFICACY
Patients were followed for 28 days in Kinshasa and for 42 days in Pailin, Attapeu, Binh Phuoc, Shwe Kyin, and Pingilikani. P. falciparum parasitemia recurred after day 14 in 39 patients (6 cases of recrudescence). Treatment efficacy corrected by means of PCR genotyping was uniformly high for all regimens at all sites (Table 3).
Table 3
Study Site | Treatment | Duration of Treatment | Duration of Follow-up | Total No. of Patients | No. of Patients with Recurrence of Infection | Efficacy | |
---|---|---|---|---|---|---|---|
Without PCR Correction | With PCR Correction† | ||||||
days | % (95% CI) | ||||||
Pailin, Cambodia | AS4-DP | 6 | 42 | 100 | 2 | 97.7 (90.9–99.4) | 97.7 (90.9–99.4) |
Vietnam | |||||||
![]() | AS2-DP | 6 | 42 | 60 | 0 | 100 (93.2–100) | 100 (93.2–100) |
![]() | AS4-DP | 6 | 42 | 60 | 0 | 100 (93.0–100) | 100 (93.0–100) |
Laos | |||||||
![]() | AS4-AL | 6 | 42 | 60 | 1 | 98.2 (88.0–99.8) | 100 (93.5–100) |
![]() | AS2-AL | 6 | 42 | 60 | 2 | 96.6 (86.9–99.1) | 100 (93.6–100) |
Myanmar | |||||||
![]() | AS4-AL | 6 | 42 | 40 | 0 | 100 (87.9–100) | 100 (87.9–100) |
![]() | AS2-AL | 6 | 42 | 40 | 0 | 100 (89.0–100) | 100 (89.0–100) |
Pingilikani, Kenya§ | AS2 | 7 | 42 | 59 | 19 | 65.5 (51.3–76.4) | 93.4 (80.8–97.9) |
Democratic Republic of Congo | |||||||
![]() | AS4-AL | 6 | 28 | 60 | 3 | 94.6 (84.3–98.2) | 100 (93.6–100) |
![]() | AL | 3 | 28 | 60 | 12 | 77.4 (64.3–88.2) | 98.3 (88.4–99.8) |
SAFETY
A total of 12 serious adverse events were reported at seven sites: acute alcohol withdrawal (in 2 patients), anemia requiring blood transfusion (in 3 patients), bacterial infection (in 3 patients), and acute asthma, viral respiratory tract infection, upper gastrointestinal bleeding, and a febrile convulsion (in 1 patient each) (Table S5 in the Supplementary Appendix).
DISCUSSION
Artemisinin-resistant P. falciparum is now firmly established in eastern Myanmar, western Cambodia and Thailand, and southern Vietnam, and it is emerging in southern Laos and northeastern Cambodia. Despite the recent spread of resistance, we found that artemisinin-based combination therapies in this region were still highly efficacious, presumably because of increased reliance on the efficacy of the partner drug as the potency of the artemisinin component waned. However, rates of treatment failure with artesunate–mefloquine in Thailand and with dihydroartemisinin–piperaquine in Cambodia have increased by a factor of more than 5.11,25-29 Mathematical modeling, in vitro experiments, and transcriptomic studies all suggest that reduced susceptibility of ring-stage parasites causes the slow parasite clearance.5-9 Strong evidence30,31 of genetic linkage to a region of P. falciparum chromosome 13 has now been translated10 into the discovery of a molecular marker — single-nucleotide polymorphisms (SNPs) in the propeller region of a kelch protein encoded by kelch13.
In this large study, multiple kelch13 SNPs were highly predictive of slow parasite clearance and were associated with more than double the parasite clearance half-life. Some SNPs (notably C580Y) predominated. With one exception, only one SNP per propeller domain was ever present in “clonal” infections. The majority of SNPs were associated with a similar prolongation of the parasite clearance half-life. The geographic distribution of these SNPs confirms that artemisinin resistance has emerged and spread extensively in mainland Southeast Asia. Widespread availability of artemisinin monotherapies, poor-quality artemisinin–based combination therapies and monotherapies containing subtherapeutic amounts of active ingredients, and unregulated use of antimalarial agents, plus the unusual genetic structure of parasites in this region,32 may have contributed to the selection of resistant parasites.
Simple surrogate measures for the parasite clearance rate, such as the proportion of patients with detectable parasitemia on day 3, have been useful in ruling out resistance, but they are imprecise and depend on the pretreatment parasite density, accurate timing of samples, and reliable counting.21 Parasite clearance rates or half-lives are preferable but they are still influenced by many factors, including antimalarial–drug dosing, pharmacokinetics, and pharmacodynamics, as well as host immunity.4,5,12,13,18,33 Sequencing of kelch13 provides a potential tool for rapid epidemiologic assessment.
Patients in Bangladesh, Nigeria, and the Democratic Republic of Congo also occasionally had parasite clearance half-life values of more than 5 hours, but these values were not associated with kelch13 polymorphisms. Conversely, 3 patients in Kinshasa who had kelch13 propeller polymorphisms had very rapid parasite clearance. However, non-synonymous SNPs before kelch13 position 441 (not associated with slow parasite clearance) were also more frequent in Kinshasa (30 of 72 patients). The ratio of proximal SNPs to distal SNPs, relative to the 441 cutoff point, may be informative in epidemiologic assessments. Slow parasite clearance after treatment with artemisinin derivatives was observed infrequently soon after they were introduced in Asia.34 Detection of persistently dormant yet drug-sensitive parasites and splenic hypofunction are possible explanations.35 Another factor is the initial doses of artemisinin derivatives, which vary considerably among different artemisinin-based combination therapies and range from 1.6 mg of artemether per kilogram in artemether–lumefantrine to 2.5 mg of dihydroartemisinin per kilogram in dihydroartemisinin–piperaquine to 4 mg of artesunate per kilogram in other artemisinin-based combination therapies. In Kinshasa, parasite clearance was faster with artesunate at a dose of 4 mg per kilogram per day than with artemether at a dose of 1.6 mg per kilogram per day, suggesting a submaximal effect with artemether.
Efficacy was good with the 6-day treatment course in areas of established artemisinin resistance. The day 42 failure rate of 2% (95% CI, 1 to 9) after 3 days of artesunate followed by 3 days of dihydroartemisinin–piperaquine in Pailin is more than 10 times lower than the recent failure rate of 25% (95% CI, 10 to 51) with the standard 3-day dihydroartemisinin–piperaquine regimen in the same area.26 Prolonged courses of treatment are one option for treating artemisinin-resistant malaria.
The higher proportions of pretreatment and post-treatment gametocytemia in patients with slow parasite clearance suggest that artemisinin-resistant P. falciparum infections have a transmission advantage that may drive the spread of resistance. This increase in post-treatment gametocytemia may be a harbinger of increased treatment failure rates, as reported previously with sulfadoxine–pyrimethamine.36 In 2012, the WHO reinforced its recommendation to add a single gametocyto cidal dose of primaquine to artemisinin-based combination therapies in order to limit the spread of artemisinin resistance,37 but the limited adoption of this policy has been disappointing.
Resistance to artemisinin has not been contained and has now emerged or spread across Southeast Asia. The spread of artemisinin resistance and the consequent emergence of resistance to the increasingly unprotected partner drugs in artemisinin-based combination regimens may well reverse the substantial recent gains in malaria control. New antimalarial drugs are under development but will not be available for several years. Radical measures will be necessary in Southeast Asia to prevent resistance to artemisinins and their partner drugs from spreading to the Indian subcontinent and then to Africa.
Acknowledgments
We thank all the patients who took part in these studies; Dr. Pascal Ringwald and the WHO Global Malaria Programme for providing quality-assured artesunate tablets and artemisinin-based combination therapies and Guilin Pharmaceutical, Beijing Holley-Cotec Pharmaceuticals, and Sigma-Tau Pharmaceuticals for providing treatments free of charge; Grant Dorsey, Rebecca Freeman Grais, and Piero Olliaro (of the data and safety monitoring committee), Prayoon Yuentrakul, Salwaluk Panapipat, Viriya Hantrakun, Praveen Chansrichavala, Tadsanawan Wannakham, and Wasana Saohinkong for site monitoring and training; Nuttapol Panachuenwongsakul, Thatsanun Ngernseng, and Pantira Koomklang for data management; Dr. Dulal Paul for data collection; Sutida Roungboon, Pattamon Tharapan, Nattiya Chanthawongsa, Bongkot Soonthornasata, and Cholrawee Promnarate for laboratory support; and Ketsanee Srinamon, Elisabeth Escher, Stephane Proux and his team at Shoklo Malaria Research Unit, Kenya Medical Research Institute–Wellcome Trust Research Programme, and Oxford University Clinical Research Unit–Vietnam for microscopy quality control.
Supported by grants from the U.K. Department for International Development, the Worldwide Antimalarial Resistance Network, the Intramural Research Program of the National Institute of Allergy and Infectious Diseases of the National Institutes of Health, and the Bill and Melinda Gates Foundation. The Mahidol-University Oxford Tropical Medicine Research Programme is funded by the Wellcome Trust of Great Britain.
APPENDIX
The authors’ full names and academic degrees are as follows: Elizabeth A. Ashley, M.B., B.S., Ph.D., Mehul Dhorda, Ph.D., Rick M. Fairhurst, M.D., Ph.D., Chanaki Amaratunga, Ph.D., Pharath Lim, M.D., Ph.D., Seila Suon, M.D., Sokunthea Sreng, Jennifer M. Anderson, Ph.D., Sivanna Mao, M.D., Baramey Sam, M.D., Chantha Sopha, M.D., Char Meng Chuor, M.D., M.P.H., Chea Nguon, M.D., Siv Sovan naroth, M.Sc., Sasithon Pukrittayakamee, M.B., D.Phil., Podjanee Jittamala, M.D., Kesinee Chotivanich, Ph.D., Kitipumi Chutasmit, M.D., Chaiyaporn Suchatsoonthorn, M.D., Ratchadaporn Runcharoen, M.D., Tran Tinh Hien, M.D., Ph.D., Nguyen Thanh Thuy-Nhien, Ph.D., Ngo Viet Thanh, M.D., Nguyen Hoan Phu, M.D., Ph.D., Ye Htut, M.B., B.S., M.Sc., Kay-Thwe Han, M.B., B.S., M.Med.Sc., Kyin Hla Aye, B.Sc., Olugbenga A. Mokuolu, M.B., B.S., F.W.A.C.P., Rasaq R. Olaosebikan, M.B., B.S., M.P.H., Olaleke O. Folaranmi, M.B., B.S., Mayfong Mayxay, M.D., Ph.D., Maniphone Khanthavong, M.D., Bouasy Hongvanthong, M.D., Paul N. Newton, M.R.C.P., Marie A. Onyamboko, M.D., M.P.H., Caterina I. Fanello, Ph.D., Antoinette K. Tshefu, M.D., Ph.D., Neelima Mishra, Ph.D., Neena Valecha, M.D., Aung Pyae Phyo, M.D., Francois Nosten, M.D., Ph.D., Poravuth Yi, M.D., Rupam Tripura, M.B., B.S., Steffen Borrmann, M.D., Mahfudh Bashraheil, Judy Peshu, M. Abul Faiz, M.B., B.S., Ph.D., Aniruddha Ghose, F.C.P.S., M. Amir Hossain, F.C.P.S., Rasheda Samad, F.C.P.S., M. Ridwanur Rahman, F.C.P.S., M. Mahtabuddin Hasan, F.C.P.S., Akhterul Islam, M.B., B.S., Olivo Miotto, Ph.D., Roberto Amato, Ph.D., Bronwyn MacInnis, Ph.D., Jim Stalker, M.A., Dominic P. Kwiatkowski, F.R.C.P., Zbynek Bozdech, Ph.D., Atthanee Jeeyapant, M.Sc., Phaik Yeong Cheah, Ph.D., Tharisara Sakulthaew, M.Sc., Jeremy Chalk, Ph.D., Benjamas Intharabut, B.Sc., Kamolrat Silamut, Ph.D., Sue J. Lee, Ph.D., Benchawan Vihokhern, B.Sc., Chanon Kunasol, M.Sc., Mallika Imwong, Ph.D., Joel Tarning, Ph.D., Walter J. Taylor, F.R.C.P., Shunmay Yeung, M.B., B.S., Ph.D., Charles J. Woodrow, M.D., Ph.D., Jennifer A. Flegg, Ph.D., Debashish Das, M.B., B.S., M.P.H., Jeffery Smith, B.A., Meera Venkatesan, Ph.D., Christopher V. Plowe, M.D., M.P.H., Kasia Stepniewska, Ph.D., Philippe J. Guerin, M.D., Ph.D., Arjen M. Dondorp, M.D., Ph.D., Nicholas P. Day, D.M., and Nicholas J. White, F.R.S.
The authors’ affiliations are as follows: Mahidol–Oxford Tropical Medicine Research Unit (E.A.A., C.I.F., A.P.P., F.N., R.T., O.M., A.J., P.Y.C., T.S., J.C., B.I., K. Silamut, S.J.L., B.V., C.K., J.T., W.J.T., C.J.W., A.M.D., N.P.D., N.J.W.), Faculty of Tropical Medicine (S.P., P.J., K. Chotivanich, M.I.), Mahidol University, Bangkok, Phusing Hospital (K. Chutasmit) and Khunhan Hospital (R.R.), Srisaket, Kraburi Hospital, Ranong (C. Suchatsoonthorn), and Shoklo Malaria Research Unit, Mahidol-Oxford Tropical Medicine Research Unit, Faculty of Tropical Medicine, Mahidol University, Mae Sot (A.P.P., F.N.) — all in Thailand; the Centre for Tropical Medicine (E.A.A., M.D., T.T.H., M.M., P.N.N., M.A.O., C.I.F., A.P.P., F.N., P.Y.C., J.C., S.J.L., J.T., W.J.T., C.J.W., J.A.F., D.D., J. Smith, M.V., K. Step-niew ska, P.J.G., A.M.D., N.P.D., N.J.W.) and the Worldwide Antimalarial Resistance Network, Centre for Tropical Medicine (M.D., J.A.F., D.D., J. Smith, M.V., K. Stepniewska, P.J.G.), Nuffield Department of Medicine, the Medical Research Council Centre for Genomics and Global Health (O.M., R.A., B.M., J. Stalker, D.P.K.), and the Wellcome Trust Centre for Human Genetics (R.A., D.P.K.), University of Oxford, Oxford, the Wellcome Trust Sanger Institute, Hinxton, Cambridge (O.M., B.M., J. Stalker, D.P.K.), and the Department of Global Health and Development, Faculty of Public Health and Policy and Clinical Research Department, Faculty of Infectious and Tropical Disease, London School of Hygiene and Tropical Medicine, London (S.Y.) — all in the United Kingdom; the Howard Hughes Medical Institute, Malaria Group, Center for Vaccine Development, University of Maryland School of Medicine, Baltimore (M.D., J. Smith, M.V., C.V.P.); the Laboratory of Malaria and Vector Research, National Institute of Allergy and Infectious Diseases, National Institutes of Health, Rockville, MD (R.M.F., C.A., P.L., J.M.A.); the National Center for Parasitology, Entomology and Malaria Control, Phnom Penh (P.L., S. Suon, S. Sreng, C.M.C., C.N., S. Sovannaroth, P.Y.), Sampov Meas Referral Hospital, Pursat (S.M.), Ratanakiri Referral Hospital, Ratanakiri (B.S.), and Makara 16 Referral Hospital, Preah Vihear (C. Sopha) — all in Cambodia; Oxford University Clinical Research Unit, Hospital for Tropical Diseases (T.T.H., N.T.T.-N., N.V.T., N.H.P.), Ho Chi Minh City, Vietnam; the Department of Medical Research, Lower Myanmar, Yangon, Myanmar (Y.H., K.-T.H., K.H.A.); the Department of Paediatrics and Child Health, University of Ilorin (O.A.M., R.R.O.) and the Department of Histopathology, University of Ilorin Teaching Hospital (O.O.F.), Ilorin, Nigeria; Lao-Oxford-Mahosot Hospital-Wellcome Trust Research Unit, Mahosot Hospital (M.M., P.N.N.), Faculty of Post-graduate Studies, University of Health Sciences (M.M.) and the Center of Malariology, Parasitology, and Entomology (M.K., B.H.), Vientiane, Laos; Kinshasa School of Public Health, Kinshasa, Democratic Republic of Congo (M.A.O., A.K.T.); National Institute of Malaria Research, Indian Council of Medical Research, Sector 8, Dwarka, New Delhi (N.M., N.V.); Kenya Medical Research Institute-Wellcome Trust Research Programme, Kilifi, Kenya (S.B., M.B., J.P.); the Institute for Tropical Medicine, University of Tübingen, and the German Center for Infection Research, Tübingen, Germany (S.B.); the Malaria Research Group and Dev Care Foundation (M.A.F., A.G., M.A.H., R.S., M.R.R.) and Shaheed Suhrawardy Medical College (M.R.R.), Dhaka, and Chittagong Medical College, Chittagong (A.G., M.A.H., R.S., M.M.H.), and Ramu Upazila Health Complex, Cox’s Bazar (A.I.) — all in Bangladesh; and Nanyang Technological University School of Biological Sciences, Singapore, Singapore (Z.B.).
Footnotes
The views expressed and information contained in this article are not necessarily those of or endorsed by the U.K. Department for International Development or by the other funders, which can accept no responsibility for such views or for any reliance placed on them.
No potential conflict of interest relevant to this article was reported.
Disclosure forms provided by the authors are available with the full text of this article at NEJM.org.
REFERENCES
Full text links
Read article at publisher's site: https://doi.org/10.1056/nejmoa1314981
Read article for free, from open access legal sources, via Unpaywall:
https://www.nejm.org/doi/pdf/10.1056/NEJMoa1314981?articleTools=true
Citations & impact
Impact metrics
Citations of article over time
Alternative metrics
Smart citations by scite.ai
Explore citation contexts and check if this article has been
supported or disputed.
https://scite.ai/reports/10.1056/nejmoa1314981
Article citations
Artemisinin-resistant Plasmodium falciparum Kelch13 mutant proteins display reduced heme-binding affinity and decreased artemisinin activation.
Commun Biol, 7(1):1499, 13 Nov 2024
Cited by: 0 articles | PMID: 39538019 | PMCID: PMC11561146
Exploring the genetic progression of MDR1 in <i>Plasmodium falciparum</i>: A decade of multi-regional genetic analysis (2014-2024).
Curr Res Microb Sci, 7:100304, 26 Oct 2024
Cited by: 0 articles | PMID: 39534723
Progressive heterogeneity of enlarged and irregularly shaped apicoplasts in <i>Plasmodium falciparum</i> persister blood stages after drug treatment.
PNAS Nexus, 3(10):pgae424, 24 Sep 2024
Cited by: 0 articles | PMID: 39381646 | PMCID: PMC11460358
Therapeutic efficacy of artemether-lumefantrine in the treatment of uncomplicated Plasmodium falciparum malaria in Arba Minch Zuria District, Gamo Zone, Southwest Ethiopia.
Malar J, 23(1):282, 17 Sep 2024
Cited by: 0 articles | PMID: 39289715 | PMCID: PMC11406784
Anti-infectivity efficacy and pharmacokinetics of WHO recommended single low-dose primaquine in children with acute Plasmodium falciparum in Burkina Faso: study protocol.
Trials, 25(1):583, 03 Sep 2024
Cited by: 0 articles | PMID: 39227956 | PMCID: PMC11373093
Go to all (1,249) article citations
Other citations
Data
Data behind the article
This data has been text mined from the article, or deposited into data resources.
BioStudies: supplemental material and supporting data
Clinical Trials
- (1 citation) ClinicalTrials.gov - NCT01350856
Lay summaries
Press releases
Wellcome Trust press releases
Similar Articles
To arrive at the top five similar articles we use a word-weighted algorithm to compare words from the Title and Abstract of each citation.
A Single Mutation in K13 Predominates in Southern China and Is Associated With Delayed Clearance of Plasmodium falciparum Following Artemisinin Treatment.
J Infect Dis, 212(10):1629-1635, 24 Apr 2015
Cited by: 97 articles | PMID: 25910630 | PMCID: PMC4621243
Defining the in vivo phenotype of artemisinin-resistant falciparum malaria: a modelling approach.
PLoS Med, 12(4):e1001823, 28 Apr 2015
Cited by: 23 articles | PMID: 25919029 | PMCID: PMC4412633
Local emergence in Amazonia of Plasmodium falciparum k13 C580Y mutants associated with in vitro artemisinin resistance.
Elife, 9:e51015, 12 May 2020
Cited by: 82 articles | PMID: 32394893 | PMCID: PMC7217694
Geographic expansion of artemisinin resistance.
J Travel Med, 26(4):taz030, 01 Jun 2019
Cited by: 15 articles | PMID: 30995310
Review
Funding
Funders who supported this work.
Intramural NIH HHS
Medical Research Council (1)
Grant ID: G0600718B
Wellcome Trust (5)
Improving the treatment of malaria.
Prof Sir Nicholas White, University of Oxford
Grant ID: 093956
Resource centre for genomic epidemiology of malaria.
Prof Dominic Kwiatkowski, University of Oxford
Grant ID: 090770
Major overseas programme Thailand. Thailand funds.
Prof Sir Nicholas White, University of Oxford
Grant ID: 077166
Understanding the genetic basis of common human diseases: core funding for the Wellcome Trust Centre for Human Genetics.
Professor Peter Donnelly, University of Oxford
Grant ID: 090532
Grant ID: 093956/Z/10/Z