Abstract
Background
Esophageal squamous cell carcinoma (ESCC) has the highest mortality rates in China. The 5-year survival rate of ESCC remains dismal despite improvements in treatments such as surgical resection and adjuvant chemoradiation, and current clinical staging approaches are limited in their ability to effectively stratify patients for treatment options. The aim of the present study, therefore, was to develop an immunohistochemistry-based prognostic model to improve clinical risk assessment for patients with ESCC.Methods
We developed a molecular prognostic model based on the combined expression of axis of epidermal growth factor receptor (EGFR), phosphorylated Specificity protein 1 (p-Sp1), and Fascin proteins. The presence of this prognostic model and associated clinical outcomes were analyzed for 130 formalin-fixed, paraffin-embedded esophageal curative resection specimens (generation dataset) and validated using an independent cohort of 185 specimens (validation dataset).Results
The expression of these three genes at the protein level was used to build a molecular prognostic model that was highly predictive of ESCC survival in both generation and validation datasets (P = 0.001). Regression analysis showed that this molecular prognostic model was strongly and independently predictive of overall survival (hazard ratio = 2.358 [95% CI, 1.391-3.996], P = 0.001 in generation dataset; hazard ratio = 1.990 [95% CI, 1.256-3.154], P = 0.003 in validation dataset). Furthermore, the predictive ability of these 3 biomarkers in combination was more robust than that of each individual biomarker.Conclusions
This technically simple immunohistochemistry-based molecular model accurately predicts ESCC patient survival and thus could serve as a complement to current clinical risk stratification approaches.Free full text

A Molecular Prognostic Model Predicts Esophageal Squamous Cell Carcinoma Prognosis
Abstract
Background
Esophageal squamous cell carcinoma (ESCC) has the highest mortality rates in China. The 5-year survival rate of ESCC remains dismal despite improvements in treatments such as surgical resection and adjuvant chemoradiation, and current clinical staging approaches are limited in their ability to effectively stratify patients for treatment options. The aim of the present study, therefore, was to develop an immunohistochemistry-based prognostic model to improve clinical risk assessment for patients with ESCC.
Methods
We developed a molecular prognostic model based on the combined expression of axis of epidermal growth factor receptor (EGFR), phosphorylated Specificity protein 1 (p-Sp1), and Fascin proteins. The presence of this prognostic model and associated clinical outcomes were analyzed for 130 formalin-fixed, paraffin-embedded esophageal curative resection specimens (generation dataset) and validated using an independent cohort of 185 specimens (validation dataset).
Results
The expression of these three genes at the protein level was used to build a molecular prognostic model that was highly predictive of ESCC survival in both generation and validation datasets (P=
0.001). Regression analysis showed that this molecular prognostic model was strongly and independently predictive of overall survival (hazard ratio
=
2.358 [95% CI, 1.391–3.996], P
=
0.001 in generation dataset; hazard ratio
=
1.990 [95% CI, 1.256–3.154], P
=
0.003 in validation dataset). Furthermore, the predictive ability of these 3 biomarkers in combination was more robust than that of each individual biomarker.
Conclusions
This technically simple immunohistochemistry-based molecular model accurately predicts ESCC patient survival and thus could serve as a complement to current clinical risk stratification approaches.
Introduction
Among all types of cancer, esophageal cancer (EC) has the eighth and sixth highest incidence and mortality rates worldwide, respectively [1]. Although esophageal adenocarcinoma (EAC) has become the predominant histological subtype in some western countries, esophageal squamous cell carcinoma (ESCC) remains dominant in China, with almost 90% of newly diagnosed patients exhibiting this cancer subtype [2]. The 5-year survival rate for ESCC remains dismal, despite improvements in treatments such as surgical resection and adjuvant chemoradiation. In current clinical practice, pathological tumor-node-metastasis (pTNM) stage is considered the optimal prognostic indicator. However, this clinical staging approach is limited in its ability to precisely stratify patients for treatment options due to wide variation in survival rates, such as that observed among T3N1 patients [3]. Clearly, identifying effective biomarkers to complement current clinical staging approaches is highly important. According to national guidelines [4], [5], biomarkers should be sensitive, specific, cost-effective, fast, robust against variability, and more accurate than current clinical stages. A single biomarker, however, may be unlikely to fulfill all of these requirements.
In recent decades, the identification of combinations of biomarkers instead of single biomarkers has become a popular research endeavor. Multi-gene signatures of breast cancer, colorectal cancer, esophageal and gastroesophageal junction adenocarcinoma, and other cancer types have served as successful prognostic indicators [3], [6]–[9]. The ability of these gene signatures to accurately predict survival provides a foundation on which to build molecular classification systems and individualized treatment approaches. To date, however, the application of molecular prognostic signatures is less advanced for ESCC than for other cancer subtypes.
In a previous study, we showed that ESCC was associated with the overexpression of Fascin, which was regulated by phosphorylated Specificity protein 1 (p-Sp1) via activation of the epidermal growth factor (EGF)/extracellular signal-regulated kinase (ERK) signaling pathway [10]. Although the clinical significance of this pathway remains unclear, the EGF receptor (EGFR), a transmembrane glycoprotein belonging to the HER family of receptors, is recognized as a negative prognostic indicator [11], [12] and has shown clinical relevance as a molecular target of cancer therapies [13], [14]. Fascin, an actin bundling protein, is also recognized as a prognostic indicator, with its overexpression associated with aggressive clinical phenotypes and poor survival [15]–[17]. Based on the clinical significance of EGFR and Fascin, we hypothesized that a combination of molecules from the EGFR/ERK/Fascin signaling pathway could accurately predict cancer outcome. Indeed, we found that a three-gene signature comprised of expression of EGFR, p-Sp1, and Fascin proteins independently predicted ESCC patient survival. This molecular prognostic model could give rise to a new molecular stratification system and provide a useful framework for future work on prognostic signatures for ESCC and other cancers.
Materials and Methods
Patients and specimens
Paraffin-embedded tissues were derived from two independent cohorts of ESCC patients undergoing curative resection at Shantou Central Hospital between 2007 and 2009 (generation dataset, n=
130) or between 1987 and 1997 (validation dataset, n
=
185). Patients in the generation dataset were followed up for a median time period of 35.0 months, with follow-ups terminated on November 9, 2012. Patients in the validation dataset were followed up for a median and maximum time period of 33.6 and 131.3 months, respectively. Overall survival rate (OS) was calculated during the period between surgery and death or final observation. Information on patient age, gender, stage of disease, therapy, and histopathology was obtained from medical records (Table 1). The study was approved by the ethical committee of the Central Hospital of Shantou City and the ethical committee of the Medical College of Shantou University, and written informed consent was obtained from all surgical patients to use resected samples for research.
Table 1
Clinical and pathological indexes | Generation dataset | Validation dataset | ||
No. | % | No. | % | |
Specimens | 130 | 185 | ||
Mean age | 59 | 58 | ||
Age (year) | ||||
< Mean age | 70 | 53.8 | 87 | 47.0 |
≥ Mean age | 60 | 46.2 | 98 | 53.0 |
Gender | ||||
Male | 103 | 79.2 | 140 | 75.7 |
Female | 27 | 20.8 | 45 | 24.3 |
Differentiation | ||||
G1 | 21 | 16.2 | 44 | 23.8 |
G2 | 97 | 74.6 | 111 | 60.0 |
G3 | 12 | 9.2 | 30 | 16.2 |
T-stage | ||||
T1+T2 | 17 | 13.1 | 32 | 17.3 |
T3+T4 | 113 | 86.9 | 153 | 82.7 |
N-stage | ||||
N0 | 63 | 48.5 | 122 | 65.9 |
N1 | 67 | 51.5 | 63 | 34.1 |
M-stage | ||||
M0 | 130 | 100 | 178 | 96.2 |
M1 | 0 | 0 | 7 | 3.8 |
pTNM-stage | ||||
IA+IB+IIA+IIB | 68 | 52.3 | 125 | 67.6 |
IIIA+IIIB+IIIC+IV | 62 | 47.7 | 60 | 32.4 |
Therapy | ||||
Only Surgery | 84 | 64.6 | 119 | 64.3 |
Surgery + chemo | 19 | 14.6 | 39 | 21.1 |
Surgery + radio | 25 | 19.2 | 20 | 10.8 |
Surgery + chemo + radio* | 2 | 1.6 | 7 | 3.8 |
Tissue microarrays (TMAs) and immunohistochemistry (IHC)
TMAs were constructed as previously described [17]–[19]. The primary antibodies used in this study were mouse anti-EGFR (ready-to-use; ZSGB-BIO, Beijing, China), rabbit anti-Sp1(phospho T453, 1100 dilution; Abcam, Cambridge, UK), and mouse anti-human Fascin-1(clone 55K-2, 1
100 dilution; Dako, Carpinteria, CA). IHC was carried out using a two-step protocol (PV-9000 Polymer Detection System, ZSGB-BIO, Beijing, China) as previously described [19].
Evaluation of IHC variables
Tissue sections were independently and blindly assessed by three histopathologists (Cao HH, Wang SH, and Shen JH). Discrepancies were resolved by consensus. The EGFR expression was scored using the HercepTest criterion [20]. EGFR scoring criteria: 0 corresponded to no staining at all, or membrane staining in less than 10% of the tumour cells was observed, 1+ corresponded to a faint/barely perceptible membrane staining was detected in more than 10% of the tumour cells. The cells were only stained in part of their membrane, 2+ corresponded to a weak to moderate staining of the entire membrane was observed in more than 10% of the tumour cells and 3+ was a strong staining of the entire membrane was observed in more than 10% of the tumour cells. EGFR staining was predominantly located in the cell membrane, cytoplasmic staining was considered non-specific and not included in the scoring. For statistical analysis, we divided EGFR scores into two groups; scores of 0–2+ were considered low-expression and scores of 3+ were considered high-expression.
Fascin expression was assessed by staining of cell cytoplasm. Its expression was scored as described by Zhao et al.17 Each separate tissue core was scored on the basis of the intensity and area of positive staining. The intensity of positive staining was scored as follows: 0, negative; 1, weak staining; 2, moderate staining; 3, strong staining. The rate of positive cells was scored on a 0–4 scale as follows: 0, 0–5%; 1, 6–25%; 2, 26–50%; 3, 51–75%; 4, >75%. If the positive staining was homogeneous, a final score was achieved by multiplication of the two scores, producing a total range of 0–12. When the staining was heterogeneous, we scored it as follows: each component was scored independently and summed for the results. For example, a specimen containing 25% tumor cells with moderate intensity (1×2=
2), 25% tumor cells with weak intensity (1×1
=
1), and 50% tumor cells without immunoreactivity (2×0
=
0), received a final score of 2+1+0
=
3. For statistical analysis, we divided Fascin scores into two groups; scores of 0–10 were considered low-expression and scores of more than 10 were considerd high-expression.
p-Sp1 expression was assessed by staining of cell nuclei. Cytoplasmic staining was considered non-specific and not included in the scoring. p-Sp1 expression levels were scored on a scale ranging from 0 to 3+: 0 indicated no positive staining; 1+ indicated only a few scattered stained cells or weak staining in less than 30% of cells within a visual field; 2+ indicated cluster(s) of moderate to strong staining in less than 30% of cells or weak staining in more than 30% of cells; 3+ indicated cluster(s) of moderate to strong staining in more than 30% of cells. For statistical analysis, we divided p-Sp1 scores into two groups; scores of 0–2+ were considered low-expression, and scores of 3+ were considered high-expression.
Construction of a weighted OS predictive model
Cox proportional hazards regression analysis was used to evaluate the association between biomarker expression and OS. We then constructed a model to estimate risk by summing the expression level of each biomarker (high-expression=
1, low-expression
=
0) multiplied by its regression coefficient [21]–[23]. Patients were dichotomized into high- or low-risk groups using the 50th percentile (i.e., median) risk score as a cut-off value.
Statistical analysis
Statistical analyses were performed using SPSS 13.0 for Windows. Cumulative survival time was calculated by the Kaplan-Meier method and analysed by the log-rank test. Spearman’s two-sided rank correlation was used to explore the correlation levels between three proteins expression. Univariate and multivariate analyses were based on the Cox proportional hazards regression model. Receiver operating characteristic (ROC) curve analysis was used to determine the predictive value of the parameters, and the differences in the area under the curve (AUC) were detected by using GraphPad Prism 5. The Kendall tau-b rank correlation analysis was used to evaluate the association between the expression of the prognostic model and clinicopathological factors. P value less than 0.05 was considered statistically significant.
Results
IHC characteristics of EGFR, p-Sp1, and Fascin biomarkers
Three potential biomarkers from the EGFR/ERK/Fascin signaling pathway were stained using IHC. EGFR and p-Sp1 staining were mainly observed in cell membranes and nuclei, respectively, whereas Fascin staining was more diffuse throughout the cytoplasm. Representative images of different staining scores are shown in Figure 1. However, positive staining of EGFR and Fascin was apparent only in basal layer of epithelium tissue adjacent to carcinoma, while p-Sp1 was weak staining in higher granular layer of the epithelium (Figure S1). Our results were the same as other reports in ESCC, while no report of Sp1 in ESCC [24], [25].
Correlations between the three biomarkers
In both the generation dataset and the validation dataset, the Spearman’s rank correlation showed that the expression of EGFR was closely associated with the Fascin expression (r=
0.299, P
=
0.001 and r
=
0.154, P
=
0.037), while no correlation between EGFR and p-Sp1 or between p-Sp1 and Fascin. Detail information was in Figure S2.
Prognostic significance of EGFR, p-Sp1, and Fascin expression and other clinical/pathological characteristics
In the generation dataset, the 1- and 3-year OS were 83.1% and 57.5%, respectively. In the validation dataset, the 1-, 3-, and 5-year OS were 93.5%, 62.4%, and 50%, respectively. Univariate analysis revealed that the three biomarkers (EGFR, p-Sp1, and Fascin), as well as four pathological factors (Differentiation [G3 vs. G1], N-stage, M-stage, and pTNM-stage), were significantly associated with OS (Table 2). However, EGFR did not significantly predict OS in the generation dataset, perhaps due to heterogeneity in EGFR expression patterns between the two datasets. Kaplan-Meier analysis provided further support that EGFR, p-Sp1, and Fascin were significant predictors of OS in both generation and validation datasets, except for EGFR in the validation dataset (Figure S3). In the generation dataset, the 3-year OS was significantly lower for the p-Sp1 and Fascin high-expression groups than the low-expression groups. In the validation dataset, the 3- and 5-year OS were significantly lower for the EGFR, p-Sp1, and Fascin high-expression groups than the low-expression groups.
Table 2
Generation dataset | Validation dataset | |||||||
Sig.* | HR | 95% CI for HR | Sig.* | HR | 95% CI for HR | |||
Variables | Lower | Upper | Lower | Upper | ||||
Univariate analyses | ||||||||
Age (≥ Mean age vs < Mean age) | 0.749 | 1.084 | 0.661 | 1.778 | 0.711 | 1.087 | 0.699 | 1.692 |
Gender (Female vs Male) | 0.372 | 1.333 | 0.709 | 2.509 | 0.333 | 0.762 | 0.440 | 1.320 |
Differentiation | 0.095 | 0.074 | ||||||
G2 vs G1 | 0.127 | 1.853 | 0.838 | 4.097 | 0.163 | 1.529 | 0.842 | 2.778 |
G3 vs G1 | 0.030 | 3.196 | 1.118 | 9.137 | 0.023 | 2.256 | 1.121 | 4.541 |
T-stage (T3+T4 vs T1+T2) | 0.771 | 0.901 | 0.444 | 1.825 | 0.799 | 0.928 | 0.520 | 1.654 |
N-stage (N1 vs N0) | 0.003 | 2.174 | 1.297 | 3.645 | 0.000 | 2.306 | 1.481 | 3.593 |
M-stagea (M1 vs M0) | 0.015 | 2.829 | 1.228 | 6.518 | ||||
pTNM-stage (III+IV vs I+II) | 0.002 | 2.220 | 1.335 | 3.690 | 0.003 | 1.982 | 1.270 | 3.092 |
Therapy (Comprehensive Therapyb vs Only Surgery) | 0.655 | 0.887 | 0.525 | 1.501 | 0.103 | 1.443 | 0.923 | 2.258 |
EGFR (high vs low) | 0.580 | 1.151 | 0.699 | 1.896 | 0.038 | 1.614 | 1.027 | 2.536 |
p-Sp1 (high vs low) | 0.004 | 2.087 | 1.271 | 3.425 | 0.030 | 1.672 | 1.052 | 2.657 |
Fascin (high vs low) | 0.027 | 1.749 | 1.065 | 2.873 | 0.017 | 1.721 | 1.104 | 2.684 |
prognostic model (high vs low) | 0.001 | 2.381 | 1.408 | 4.029 | 0.001 | 2.131 | 1.348 | 3.369 |
Multivariate analysis | ||||||||
pTNM-tage (III+IV vs I+II) | 0.003 | 2.199 | 1.319 | 3.667 | 0.008 | 1.826 | 1.167 | 2.856 |
prognostic model (high vs low) | 0.001 | 2.358 | 1.391 | 3.996 | 0.003 | 1.990 | 1.256 | 3.154 |
Predictive molecular prognostic model
Our molecular prognostic model was Calculated as Y=
(β1)×(EGFR)+(β2)×(p-Sp1)+(β3)×(Fascin), with Y equal to risk score and βn equal to each gene’s coefficient value from univariate Cox proportional hazards regression analysis. In the generation dataset, β1
=
0.141, β2
=
0.736, and β3
=
0.559. In the validation dataset, β1
=
0.479, β2
=
0.514, and β3
=
0.543. Patients were ranked and divided into high- and low-risk groups using the 50th percentile (i.e., median) risk score as the cut-off value.
In the generation dataset, the 3-year OS for the high-risk group was significantly lower than that for the low-risk group (73.6% vs. 43.3%; Figure 2A). Similar results were found in the validation dataset, that the 3- and 5-year OS for the high-risk group were significantly lower than those for the low-risk group (73.6% and 61.8% vs. 51.4% and 37.2%, respectively; Figure 2A). Multivariate Cox proportional hazards regression analysis showed that the three-gene signature, along with pTNM-stage, was a strong and independent predictor of OS (Table 2).
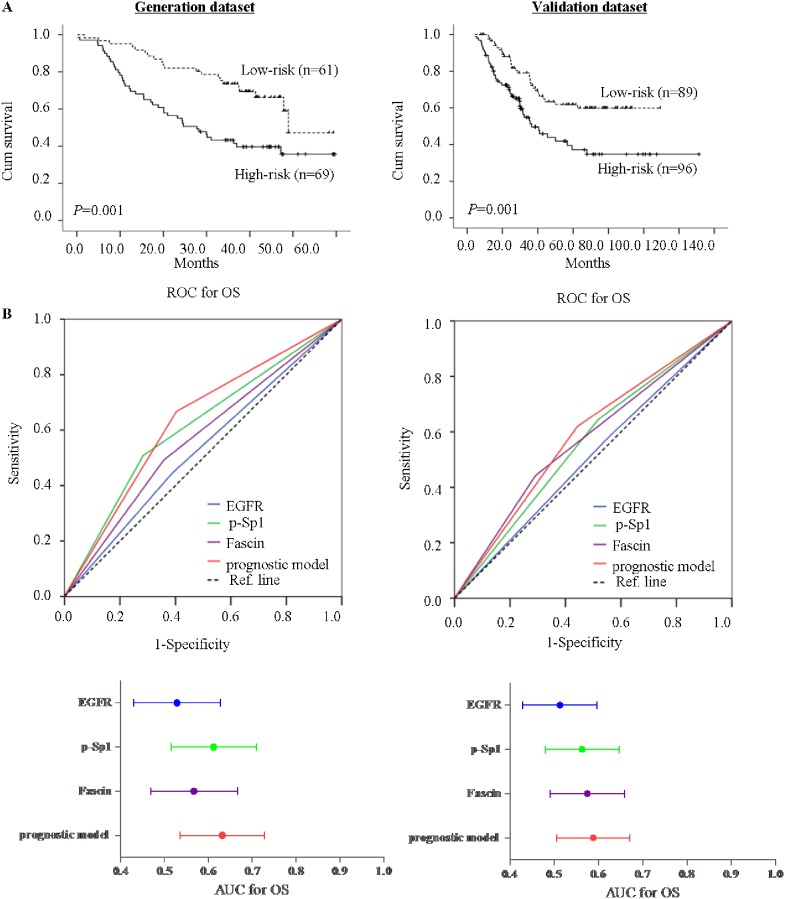
A, Kaplan-Meier analysis of OS for low-risk and high-risk ESCC patients based on expression of the molecular prognostic model in generation and validation datasets. B, Predictive ability of the molecular prognostic model compared with individual biomarker shown by receiver operating characteristic (ROC) curves and area under the curve (AUC) in generation and validation datasets.
Predictive power of the molecular prognostic model
In both the generation and validation datasets, receiver operating characteristic (ROC) analysis showed that the predictive power of the prognostic model was higher than that for each biomarker individually. In the generation dataset, specificity and sensitivity were 66.7% and 59.7%, respectively, and area under the curve (AUC) for OS with 95% CI was 0.632. Similar results were found in the validation dataset, with 62% specificity, 55.7% sensitivity, and 0.588 AUC (Figure 2B). Furthermore, in the generation dataset, the predictive ability of the prognostic model was not only higher than that of EGFR, p-Sp1, and Fascin individually but also higher than all clinical/pathological characteristics. However, in the validation dataset, the AUC for the prognostic model was not larger than that for N-stage and pTNM-stage, but specificity and sensitivity were optimal (Figure S4).
Correlations between the prognostic model and clinical/pathological characteristics
Kendall tau-b correlation analysis indicated that the prognostic model was significantly related to N-stage (Table 3). In the generation dataset, the proportion of high-risk in patients suffering regional lymph node metastasis (N1) were significantly higher than that of high-risk in patients without regional lymph node metastasis (N0) (62.7% [42/67] vs. 42.9% [27/63], P=
0.035). Similar results were obtained in the validation dataset (63.5%[40/63] vs. 45.9% [56/122], P
=
0.030). Other clinical/pathological characteristics such as age, gender, differentiation, T-stage, M-stage, pTNM-stage, and therapy, however, were not significantly different between high-risk and low-risk patients.
Table 3
Variables | Generation dataset | P * | Validation dataset | P * | ||
Low-risk | High-risk | Low-risk | High-risk | |||
Age (year) | ||||||
< Mean age | 35 | 35 | 0.484 | 41 | 46 | 0.883 |
≥ Mean age | 26 | 34 | 48 | 50 | ||
Gender | ||||||
Male | 44 | 59 | 0.083 | 68 | 72 | 0.865 |
Female | 17 | 10 | 21 | 24 | ||
Differentiation | ||||||
G1 | 9 | 12 | 0.465 | 23 | 21 | 0.905 |
G2 | 45 | 52 | 49 | 62 | ||
G3 | 7 | 5 | 17 | 13 | ||
T-stage | ||||||
T1+T2 | 4 | 13 | 0.066 | 15 | 17 | 1.000 |
T3+T4 | 57 | 56 | 74 | 79 | ||
N-stage | ||||||
N0 | 36 | 27 | 0.035 | 66 | 56 | 0.030 |
N1 | 25 | 42 | 23 | 40 | ||
M-stage | ||||||
M0 | 61 | 69 | - | 86 | 92 | 1.000 |
M1 | 0 | 0 | 3 | 4 | ||
pTNM-stage | ||||||
IA+IB+IIA+IIB | 37 | 31 | 0.081 | 65 | 60 | 0.157 |
IIIA+IIIIB+IIIC+IV | 24 | 38 | 24 | 36 | ||
Therapy | ||||||
Only Surgery | 38 | 46 | 0.714 | 57 | 62 | 1.000 |
Comprehensive Therapya | 23 | 23 | 32 | 34 |
Combination of the prognostic model and N-stage
As our results indicate that both the prognostic model and N-stage are involved in ESCC prognosis, we next considered these characteristics together. Patients were subdivided into four subgroups: N0+low-risk, N0+high-risk, N1+low-risk, and N1+high-risk. N1+high-risk patients had the poorest prognoses, whereas the other three groups showed no notable differences in prognoses (data not shown); therefore, these three groups were merged into a single group. Kaplan-Meier curves showed significant differences in OS between the two groups (Figure 3). In the generation dataset, the 3-year OS was 25.6% for the N1+high-risk group compared with 72.7% for the other group. In the validation dataset, the 3- and 5-year OS were 42.5% and 26.5%, respectively, for the N1+high-risk group, compared with 67.9% and 56.3% for the other group.
Discussion
Although many prospective studies have assessed potential biomarkers of cancer using high-throughput screening techniques [21]–[23], there is often little to no biological connection among the individual biomarkers. Furthermore, single biomarker predictor models often have limited power to predict cancer patient survival [26]–[28]. Therefore, the three-gene signature discovered in the present study, which is comprised of molecules within the EGFR/ERK/Fascin signaling pathway, may represent a useful preclinical model for improving ESCC treatment and clinical outcome. Using two independent cohorts of ESCC patients, our study both generates and validates this molecular prognostic model, which predicts poor prognosis. We investigated our molecular prognostic model at the protein level for two reasons. First, formalin-fixed, paraffin-embedded tissue is far more available than other types of samples such as fresh-frozen tissue. Second, IHC is technically simple, fast, economical, clinical applicable, and robust, in contrast to assessments of gene expression at the mRNA level, which require standardization of techniques to allow comparison of data across days, laboratories, and types of samples [3].
This prognostic model made it possible to identify a cohort of ESCC patients with a 5-year survival of 52%, which is remarkable for this disease. Combining the prognostic model with N-stage, we found that N1+high-risk patients had the poorest clinical outcome, whereas N1+low-risk and N0+high/low-risk patients had similar prognoses. This result, while surprising, could serve to guide treatment options. That is, N1 and high-risk patients may urgently require therapeutic intervention to improve their prognosis. EGFR is a particularly promising molecular target of therapy, as EGFR inhibitors have been widely applied to a variety of solid tumors, such as lung cancer [13], [14], colorectal cancer [29], breast cancer [30], and even ESCC [31]. Some of these therapeutic strategies have been subject to clinical trials, with four EGFR inhibitors currently approved by the US Food and Drug Administration, including gefitinib, erlotinib, cetuximab, and, most recently, panitumumab. Therefore, the poor clinical outcome of N1+high-risk patients might be improved by a more comprehensive treatment approach, such as chemotherapy or radiotherapy combined with cetuximab treatment. In addition to EGFR, Fascin is also recognized as a therapeutic target [32], as binding with migrastatin analogues inhibits Fascin activity and blocks tumor metastasis [33]. Our prognostic model could therefore lead to new avenues of therapy for patients with ESCC, such as treatment with EGFR and/or Fascin inhibitors.
Besides, in the past, patients once diagnosed with lymphatic metastasis received several simultaneous treatments in an unselective manner. However, such overtreatment often fails to improve prognosis and leads to a massive waste of medical resources. Our results also suggest that N1+low-risk patients could be treated the same as lymphonodus-negative patients. Therefore, this new prognostic stratification could guide treatment choices for patients diagnosed with lymphatic metastasis. As exploring potential biomarkers within a single signaling pathway may still be rather limited, future studies could attempt to evaluate multiple signaling pathways to further elucidate the pathogenesis of ESCC in a deeper and more biologically relevant context. Furthermore, we envision that it will be possible to combine other clinical characteristics, such as pTNM-stage, with multi-molecular prognostic model to more accurately predict ESCC survival.
In conclusion, we found that a molecular prognostic model, comprised of expression of EGFR, p-Sp1, and Fascin proteins, was significantly associated with poor ESCC clinical outcome. Such poor survival of patients could be improved by combining chemoradiation with targeted anti-EGFR or/and anti-Fascin treatment. These findings could give rise to a new prognostic stratification system and provide a useful framework for future work on predictive molecular signatures and therapeutic options for ESCC.
Supporting Information
Figure S1
Representative images of IHC staining for EGFR, p-Sp1, and Fascin in epithelium tissue adjacent to carcinoma. Scale bars=
50 µm.
(PDF)
Figure S2
Correlation analyses between three proteins expression (EGFR, p-Sp1 and Fascin) in generation dataset of 130 cases (A, B and C) and validation dataset of 185 cases (D, E and F).
(PDF)
Figure S3
Kaplan-Meier analysis of overall survival for EGFR, p-Sp1 and Fascin in generation dataset of 130 cases (A, B and C) and validation dataset of 185 cases (D, E and F).
(PDF)
Figure S4
The predictive ability of the molecular prognostic model compared with individual markers and other clinical prognostic parameters by receiver operating characteristic (ROC) curves (A for generation dataset, B for validation dataset). The areas under the curve (AUCs) with 95% CI for OS are shown in C (generation dataset) and D (validation dataset).
(PDF)
Funding Statement
This work was supported by grants from the National Research Program (973 Program No. 2012CB526600), the National High Technology Research and Development Program of China (No. 2012AA02A503 and No. 2012AA02A209), and the Natural Science Foundation of China-GuangDong Joint Fund (No. U0932001 and No. U1301227). The funders had no role in study design, data collection and analysis, decision to publish, or preparation of the manuscript.
Data Availability
The authors confirm that all data underlying the findings are fully available without restriction. All relevant data are within the paper and its Supporting Information files.
References
Articles from PLOS ONE are provided here courtesy of PLOS
Full text links
Read article at publisher's site: https://doi.org/10.1371/journal.pone.0106007
Read article for free, from open access legal sources, via Unpaywall:
https://journals.plos.org/plosone/article/file?id=10.1371/journal.pone.0106007&type=printable
Citations & impact
Impact metrics
Article citations
FSCN1 acts as a promising therapeutic target in the blockade of tumor cell motility: a review of its function, mechanism, and clinical significance.
J Cancer, 13(8):2528-2539, 09 May 2022
Cited by: 11 articles | PMID: 35711849 | PMCID: PMC9174856
Review Free full text in Europe PMC
CircRNA-DOPEY2 enhances the chemosensitivity of esophageal cancer cells by inhibiting CPEB4-mediated Mcl-1 translation.
J Exp Clin Cancer Res, 40(1):361, 15 Nov 2021
Cited by: 30 articles | PMID: 34781999 | PMCID: PMC8591801
Co-Occurrence of Differentiated Thyroid Cancer and Second Primary Malignancy: Correlation with Expression Profiles of Mismatch Repair Protein and Cell Cycle Regulators.
Cancers (Basel), 13(21):5486, 31 Oct 2021
Cited by: 1 article | PMID: 34771648 | PMCID: PMC8582561
Comparative genomic analysis of esophageal squamous cell carcinoma and adenocarcinoma: New opportunities towards molecularly targeted therapy.
Acta Pharm Sin B, 12(3):1054-1067, 30 Sep 2021
Cited by: 18 articles | PMID: 35530133 | PMCID: PMC9069403
Review Free full text in Europe PMC
Emerging Role of Fascin-1 in the Pathogenesis, Diagnosis, and Treatment of the Gastrointestinal Cancers.
Cancers (Basel), 13(11):2536, 21 May 2021
Cited by: 11 articles | PMID: 34064154 | PMCID: PMC8196771
Review Free full text in Europe PMC
Go to all (24) article citations
Data
Data behind the article
This data has been text mined from the article, or deposited into data resources.
BioStudies: supplemental material and supporting data
Similar Articles
To arrive at the top five similar articles we use a word-weighted algorithm to compare words from the Title and Abstract of each citation.
A three-protein signature and clinical outcome in esophageal squamous cell carcinoma.
Oncotarget, 6(7):5435-5448, 01 Mar 2015
Cited by: 32 articles | PMID: 25605255 | PMCID: PMC4467159
Expression of cyclooxygenase-2, vascular endothelial growth factor, and epidermal growth factor receptor in Chinese patients with esophageal squamous cell carcinoma.
J Cancer Res Ther, 11 Suppl 1:C44-8, 01 Aug 2015
Cited by: 4 articles | PMID: 26323923
A novel staging model to classify oesophageal squamous cell carcinoma patients in China.
Br J Cancer, 110(8):2109-2115, 25 Feb 2014
Cited by: 11 articles | PMID: 24569468 | PMCID: PMC3992487
Immunohistochemical prognostic markers of esophageal squamous cell carcinoma: a systematic review.
Chin J Cancer, 36(1):65, 17 Aug 2017
Cited by: 40 articles | PMID: 28818096 | PMCID: PMC5561640
Review Free full text in Europe PMC