Abstract
Free full text

EHEC Adhesins
Abstract
Adhesins are a group of proteins in enterohemorrhagic Escherichia coli (EHEC) that are involved in the attachment or colonization of this pathogen to abiotic (plastic or steel) and biological surfaces, such as those found in bovine and human intestines. This review provides the most up-to-date information on these essential adhesion factors, summarizing important historical discoveries and analyzing the current and future state of this research. In doing so, the proteins intimin and Tir are discussed in depth, especially regarding their role in the development of attaching and effacing lesions and in EHEC virulence. Further, a series of fimbrial proteins (Lpf1, Lpf2, curli, ECP, F9, ELF, Sfp, HCP, and type 1 fimbriae) are also described, emphasizing their various contributions to adherence and colonization of different surfaces and their potential use as genetic markers in detection and classification of different EHEC serotypes. This review also discusses the role of several autotransporter proteins (EhaA-D, EspP, Saa and Sab, and Cah), as well as other proteins associated with adherence, such as flagella, EibG, Iha, and OmpA. While these proteins have all been studied to varying degrees, all of the adhesins summarized in this chapter have been linked to different stages of the EHEC life cycle, making them good targets for the development of more effective diagnostics and therapeutics.
Introduction
Among the thousands of bacterial species contained within the intestinal gut flora, it is accepted that each species requires the use of adhesin proteins, or some combination thereof, that bring the bacteria closer to the epithelia and allow them to colonize the intestine. In a similar way, enteric pathogens also require surface-localized adhesins for colonization of the host intestine and eventual establishment of disease. Enterohemorrhagic Escherichia coli (EHEC), and in general, Shiga toxin-producing E. coli strains are known to contain a large number of proteins responsible for adhesion and contribute to establishment, persistence, and tissue tropism observed during infection with these pathogens. Understanding how these adhesins work is critical to having a full picture of the pathogenic and pathophysiological process associated with EHEC. Further, because adhesins play such an important role in virulence, they are targets for therapeutic intervention. Thus, this chapter summarizes the current knowledge on the adhesive proteins in EHEC, emphasizing up-to-date information and discussing gaps in knowledge and future directions in the study of these virulence factors.
Locus of Enterocyte Effacement (LEE)
The study of adhesion in E. coli strains that produce an intimate attachment to the epithelia dates back to 1990, when a single gene, eae, was discovered in enteropathogenic E. coli (EPEC) using a transposon-based mutagenesis system. It was established that the product of this gene was necessary for the formation of attaching and effacing lesions (A/E lesions) (1). A follow-up study indicated that this gene was conserved in EHEC (2), and early in vivo studies showed that this gene’s protein product, intimin, was important for intimate attachment and colonization of the intestine in both piglets and humans (3,4). Though intimin and other Locus of Enterocyte Effacement (LEE)-encoded factors linked to pathogenesis have been extensively reviewed elsewhere (5,6), we will briefly describe some historical information and focus on recent advances in the understanding of LEE-encoded Tir and Intimin interactions.
The eae gene is part of a larger set of genes that make up the LEE pathogenicity island. The LEE was first described as a 35 kb locus that was conserved in different isolates of EHEC and EPEC, but was not present in non-pathogenic strains of E. coli (7). Two independent sequencing projects determined the DNA sequence of the LEE and described a locus that was divided into 5 major operons containing up to 41 genes (8,9) (Figure 1). An additional 11 genes were identified in the LEE of EHEC that were not found in EPEC and were associated with a pro-phage coding region present on the terminal end of the operon (9). Other parallel studies verified the presence of the LEE in a large number of pathogenic E. coli strains, all of which were associated with A/E lesion formation, including the E. coli RDEC-1 strain found in rabbits, EHEC O26:H-, O15:H-, O103:H2 and Citrobacter rodentium (9,10,11,12). Further studies identified specific gene products within this island as being required for the colonization of the bovine gut (13,14), and others verified that the LEE was associated with the development of enteritis in other animal models, such as mice (15), calves (16), sheep (17), and rabbits (18,19).

Illustration of the EHEC prototype strain EDL933 LEE pathogenicity island. The five LEE operons are depicted, specifically emphasizing those genes-encoded proteins associated with adhesion and A/E lesion formation. These include the genes encoding for the regulatory proteins Ler, GrlA and GrlR, the translocated receptor protein Tir, and the adhesin protein intimin.
A large portion of the LEE represents the coding region for a type 3 secretion system (T3SS), a complex structural system that allows for translocation of effector proteins out of the bacterial cell and into the surrounding environment or directly into a host cell. Early studies in EPEC suggested that this pathogen was capable of protein secretion, that these proteins were required for full virulence and the formation of A/E lesions (20,21), and that this secretion was likely mediated by a T3SS (22). The relationship between the T3SS and the LEE was confirmed by the publication of the aforementioned sequencing projects (8), which helped identify individual components of the T3SS and their role in pathogenesis (23,24,25).
Since the LEE and its associated effector proteins play such a significant role in the virulence of EHEC, understanding the factors that impact the expression of these genes became a priority. A number of studies have identified a series of transcriptional regulators that impact the expression of various LEE-associated genes and include several proteins, such as QseA (26), H-NS (27), and LEE-encoded regulators Ler (28), GrlA, and GrlR (29). In addition, there are very specific environmental conditions that impact LEE expression, including pH, osmolarity, Fe(NO3)2, Ca2+, temperature (30), quorum sensing (31,32), and HCO3 (33,30). More generally, LEE expression is also modulated by carbon catabolite repression in vitro (34) and by a combination of factors found in spent media from epithelial cell culture (35) or by post-transcriptional regulators like CsrA (36).
H-NS and Ler as important regulators of EHEC adhesion
Of all the factors involved in the regulation of the LEE, two of the most extensively studied and closely associated with virulence are the negative regulator (silencer) H-NS and the positive regulator (anti-silencer) Ler. The Ler protein was first identified as a member of the LEE1 operon, and it is a transcription factor that positively regulates LEE2, LEE3, and LEE4, and may also contribute to regulation of its own transcription (28,37,38,39). As such, Ler and its regulatory activity are required for adherence, A/E lesion formation, and virulence of EHEC (40). Certain ler mutants have also shown modified adhesion capabilities and displayed a novel set of fimbriae on the surface of the bacterial cell (39). Ler is involved in multiple regulatory systems, and its transcription is mediated by a large number of factors, including available sugars (41), quorum-sensing associated proteins such as QseA (26), the non-coding RNA DsrA (42), and the universal regulator Hfq (43). Ler is also regulated by GrlA, which completes an auto-regulatory loop, as Ler is responsible for initial GrlA activation (44).
Ler imparts its positive regulatory effects by directly interfering with the negative histone-like negative regulator H-NS. This protein regulates a huge number of genes throughout the EHEC chromosome by binding to specifically curved DNA commonly found in promoter regions (45). The Ler protein originates from a similar protein family as H-NS, and the ability of both of these proteins to affect their target promoters requires a capacity to form long oligomers. Recent studies suggest that these two proteins contain similar DNA binding domains, and differ only in the behavior surrounding oligomerization (46). This difference seems to represent the mechanism by which Ler is able to antagonize the binding capacity of H-NS to the target promoter regions (38).
Intimin and Tir
Though H-NS and Ler regulate multiple genes located throughout the chromosome, it is their ability to regulate the proteins intimin and Tir and the long polar fimbriae (discussed below) that highlight their capacity to impact EHEC adhesion. As mentioned, the intimin protein was originally identified in EPEC (47) and researchers quickly identified a homolog in EHEC (2). The EPEC and EHEC intimin proteins share 86% homology at the nucleotide level and 83% homology at the protein level, with most of the divergence between these two proteins at their C-terminal end, which is the domain responsible for target interaction (2,4). Over the following decade, it became firmly established that intimin was the primary molecule in both EHEC and EPEC associated with intimate bacterial interaction with the epithelia. Cell culture studies using EHEC confirmed that this protein is essential for intimate adhesion, and disruption of the eae gene abolishes this phenotype (4). Furthermore, intimin has been shown to be essential for A/E lesion formation in both gnotobiotic pigs and colostrum-deprived calves (48,49,14). Finally, antibodies against intimin inhibits EHEC adherence in a cell-culture model (49,14,50). This central role of intimin in the interaction with the intestinal epithelia spurred further research towards understanding this protein’s function and role in virulence.
DNA sequencing and structural studies indicated that intimin is a 95 kDa (939 amino acids) protein that can be divided into two functional regions. The N-terminal region (residues 1–550) contains a signal peptide region (1–39) that is responsible for its interaction with the bacterial Sec pathway and eventual secretion to the bacterial outer membrane, as well as a LysM-type region that allows for integration into peptidoglycan (51). From residues 189–550, a β-domain spans the outer membrane of the bacteria and is responsible for promotion of surface exposure for host-cell interaction (52). The C-terminal region contains 4 smaller domains (D1-D4), each of which are independent bacterial immunoglobulin-like (BIG) domains that form a surface-exposed rod that binds to Tir and is capped by a C-type lectin domain (53,54). The interaction between intimin and its binding partner Tir primarily occurs between a “superdomain” formed between domain 3 and domain 4 of intimin and a β-hairpin motif on Tir (55).
The effort to characterize the Tir and intimin interaction originally started as an effort to identify what was thought to be a host-cell receptor protein mediating EHEC adhesion. This receptor was eventually identified in EPEC and called Hp90, whose phosphorylation was shown to mediate the actin rearrangements visible in A/E lesion formation and directly interact with intimin (56). Further studies in EPEC recognized that this was actually the first (and only) example of a bacterial protein being translocated into the host-cell membrane, acting as its own receptor for host-cell interaction (30). This protein used the T3SS to reach the host-cell cytoplasm and was named Tir for “translocated intimin receptor” (55). In EHEC, the previously discovered protein EspE was shown to be a homolog of Tir and was renamed to reflect this identity. Once translocation into the host cell is complete, Tir integrates itself into the host-cell membrane, exposing both terminal ends to the cytoplasm and an extracellular domain for interaction with intimin (57,58). Upon integration, however, Tir (EHEC) and Tir (EPEC) behave differently. Tir (EPEC) function is dependent on the phosphorylation of tyrosine 474, which is lacking in EHEC Tir (59,60,61). Further, EHEC Tir is incapable of complementing EPEC Tir on account of this amino acid difference (60,61,62). Since Tir (EHEC) lacks tyrosine 474, EHEC-mediated actin rearrangement is instead controlled by a second bacterial translocated virulence factor, EspFU (formerly TccP) (63, 64). This protein directly interacts with EHEC Tir upon its translocation into the host cell (63), and these two proteins form a larger complex with two host proteins, IRSp53 and IRTKS (64,65,66). The complex recruits and activates the host proteins N-WASP and Arp2/3, which leads to the reorganization of actin within the host cell and the pedestal formation commonly-associated with A/E lesion formation (63,65,66).
Though this interaction between Tir and intimin is likely the primary means by which EHEC is able to intimately adhere to epithelial cells, other studies attempting to understand the relationship between these two proteins revealed that adhesion is a more complex process than originally realized. Frankel et al. used purified EPEC intimin to determine if and how intimin was interacting with HEp-2 cells in vitro (67). In this scenario, intimin would lack its translocated binding partner Tir. Despite this, fluorescence was observed on the surface of these cells, suggesting that intimin may be capable of interacting with host cells independent of Tir. Follow up studies using an eae mutant were able to identify a single amino acid in the C-terminal 150 residues of intimin that was responsible for this interaction (68). Further, using solid-phase binding assays, intimin was shown to interact with the host cell-surface located protein β1 integrin (69). This interaction, however, is not required for EPEC adhesion, and mutation of the gene encoding β1 integrin did not have a negative impact on A/E lesion formation or the attachment of EPEC to the surface of cells in vitro (70). Finally, studies to map the binding domain within intimin identified the previously discussed lectin domain located on the C-terminal end of intimin, and removal of this domain completely abrogated Tir-independent binding (71). Similar studies characterizing Tir-independent binding in EHEC-derived intimin were done by Sinclair et al., who used fluorescent microscopy to co-localize β1 integrin and intimin (72), and a separate study further strengthened this conclusion by abrogating the interaction between EHEC and cultured epithelial monolayers by adding heparin and heparin sulfate (73). These molecules directly interact with integrin and act in a similar fashion as a monoclonal antibody, interfering with the binding of these two proteins.
In addition to the intimin-β1 integrin interaction, a second Tir-independent interaction was discovered during these initial characterizations. The first studies looking closely at Tir-independent binding in EHEC identified a second binding event occurring between intimin and nucleolin (74). Still associated with the C-terminal end of intimin, the binding of this lectin domain was calculated to have an affinity of around 100 nM to host cells, and through affinity purification and sequencing steps, nucleolin was identified as a binding partner mediating this interaction. More detailed studies on this interaction showed that both EPEC- and EHEC-derived intimin bound to nucleolin with the same affinity, but that this interaction was weaker than that between intimin and Tir (75). These results laid the foundation for a hypothesis indicating that the intimin-nucleolin and the intimin-integrin interactions were only secondary binding events during infection and that intimin-Tir are required to keep the pathogen in place while the T3SS and its effector proteins were injected into the host. Finally, some more recent data further illustrated the importance of intimin’s interaction with both nucleolin and β1 integrin through the effects of EHEC-encoded Shiga toxin (Stx). During infection, Stx is internalized via receptor-mediated endocytosis and is capable of modifying the transcription of nucleolin and β1 integrin. In the absence of Stx, EHEC adhesion was significantly reduced, suggesting that the pathogen is capable of modifying host transcription patterns to increase its chances of successful adhesion and attachment of epithelial cells (76).
As previously mentioned, intimin contains two functional regions: the N-terminal region required for anchoring the protein into the bacterial membrane, and the C-terminal region responsible for host-cell and Tir interactions. As a result of this extracellular localization, it has been shown that this region is recognized by the immune system, as antibodies are known to be developed against intimin during the normal course of infection in patients suffering from hemorrhagic colitis (HC) and hemolytic-uremic syndrome (HUS) (77,78,79). It has been speculated that to avoid recognition by the immune system, both EPEC- and EHEC-derived intimin proteins have shown a high degree of plasticity and subsequent variability in this exposed C-terminal region (67). This variability has allowed for allele-specific subtyping of the intimin protein, and overall, 27 total variants of the intimin protein have been identified which are made up of eighteen different types and nine subtypes. These include α, α2, β1, β2, β3, γ1, γ2, δ, ε, ε2, ε3, ε4, ζ, η, η2, θ, ι, ι2, κ, λ, µ, ν, ξ, ο, π, ρ, and σ (80,81,82,83,84,85,86,87). The most common clinically relevant subtypes are those within α-, β-, and γ-type. The α-type is found in multiple EPEC strains, including O127:H6, as is the β-type, with the only exception being EHEC O26:H11. The γ-type includes the EHEC O157:H7, as well as two other EPEC strains, O55:H- and O55:H7 (6).
Because one unique feature between different types of A/E-inducing pathogenic E. coli relates to tissue tropism, it is logical to propose that these different intimin subtypes are one of the driving forces behind the diverse tropism and variability in EHEC and EPEC interaction with host cells. Indeed, early studies prior to the establishment of intimin typing system established that substituting intimin from a γ-subtype (O157:H7) to a β-type (O127:H6), completely changed the target of infection from the large intestine, which is commonly seen in EHEC, to both the small and large intestine, which is more frequently associated with EPEC infections (48). Later studies using in vitro organ cultures supported this hypothesis by showing that restoring different intimin subtypes into a O157:H7 eae mutant strain shifted the adhesion from Peyer’s patches, common in EHEC, to the small intestine (88). Further, this study validated grouping within the intimin γ-type, as EPEC O55:H7 was also associated with Peyer’s patches in the large intestine. Other studies, using subtypes from α-, β-, and γ-types, showed shifting tissue tropism using both in vitro organ culture models and in vivo infections in mice (89).
While there is strong evidence suggesting that the emergence of these intimin types and subtypes are a way to predict tissue tropism, these differences may have originally been the result of immune system avoidance. As mentioned, antibodies against intimin are detectable in patients suffering from EHEC and EPEC infection, and these antibodies are generally specific to their serotype (90,91). The presence of antibodies against intimin, combined with the fact that this protein is extracellular, has made antibody-mediated protection a promising solution to both EPEC and EHEC infections. This protection likely occurs as a result of antibody-mediated interference with intimin interaction with Tir, β1 integrin, or nucleolin. Initial studies have shown that antibodies did in fact significantly limit the amount of adherence observed on the surface of in vitro-cultured epithelial cells (50,92), and vaccination using recombinant intimin was able to induce specific antibody responses (93). Further, a wide range of protection studies have been undertaken using many different types of host organisms and delivery systems and have allowed for a number of interesting conclusions. First, multiple studies have reinforced the fact that antibody-mediated protection is subtype-specific. For example, γ-intimin-vaccinated mice generated antibodies against EHEC infection, and displayed a decreased shedding time (15). Similar results were seen using intimin-α and EPEC (94). Intimin-based vaccines have also been shown to be effective in rabbits (95) and adult cattle (96), and infant calves were protected by anti-intimin antibodies obtained from colostrum (97). Second, there have also been a number of different vector systems used to produce intimin, such as developing a plant-derived edible version of intimin (98), or using attenuated Salmonella as the delivery vehicle (99,100). Ultimately, the ideal vaccine should protect against all major intimin subtypes found in EHEC and EPEC, and it could still be used to protect bovine or porcine herds from colonization, thus indirectly diminishing the potential exposure of these categories of E. coli strains to humans.
Long-Polar Fimbriae (Lpf1 and Lpf2)
Cumulative evidence supports the fact that although intimin is the primary adhesin in EHEC/STEC, it is by no means the only contributing factor. This became clear when a novel STEC O113:H21 strain was isolated from infected humans and showed to be lacking the intimin gene (101,102). Not only was this strain infectious, but it was able to adhere to in vitro cultured cells, implicating other proteins in contributing to the adhesive capabilities of pathogenic E. coli. In addition to this observation, it was also been shown that two different EPEC O55 serotypes, O55:H6 and O55:H7, express intimin-α and -γ, respectively, which should result in specific tropism to two different regions of the intestine. However, it was observed that these strains are both restricted to interaction with the follicle-associated endothelium (FAE), suggesting that perhaps other factors are contributing to tissue tropism in addition to intimin (103).
Full-genome sequencing and subsequent analysis of two prototype O157:H7 strains identified approx. 1.34 Mbp of DNA sequence present in O157:H7 but absent in the non-pathogenic K-12 strain (104,105). These regions are distributed throughout the genome as “O islands,” and two of these, islands #141 and #154, contained regions with homology to Salmonella enterica serovar Typhimurium. The proteins encoded in these regions were predicted to produce the components of the long polar fimbriae (Lpf), originally characterized in Salmonella (106), and which was found to contribute to the pathogen’s adherence to the human intestine (Table 1). A mutation in the gene encoding the Salmonella major fimbrial subunit (lpfA) showed a significant reduction in adhesion using in vitro tissue cultured cells, and specifically, this mutation seemed to impact the bacterial interaction with Peyer’s patches and M-cells (107). As a result of the homology with the Salmonella lpf operon, the O157:H7 regions O-141 and O-154 were named lpf1 and lpf2.
Table 1
Major characteristics of the EHEC fimbrial proteins
EHEC fimbria name | Primary Structural gene | Representative strains | Ligand | Cell lines used to show binding |
---|---|---|---|---|
Long polar fimbriae 1 | lpfA1 | O157:H7, O55:H7, O127:H6 | Fibronectin, collagen IV, laminin | Caco-2, HeLa |
Long polar fimbriae 2 | lpfA2 | O157:H7 | Unknown | Caco-2 |
Curli | csgA | O157:H7, O26:H11 | Abiotic surfaces, lettuce leaves | T84 |
E. coli common pilus | ecpA | O157:H7, O26:H11, O32:H37, O104:H4 | unknown | HT-29, HEp- 2, HeLa |
F9 fimbriae | z2200 | O157:H7, O26:H-, O104:H4, O55:H7, O127:H6 | Fibronectin | EBL, HeLa |
E. coli laminin-binding fimbriae | ycbQ | O157:H7, O26:H11, O104:H4 | Laminin | HT-29, HEp- 2, MDBK |
Sorbitol-fermenting fimbriae protein | sfpA | O157:H-, O157:NM, O165:H25 | Unknown | Caco-2, HCT- 8 |
Type 1 fimbriae | fimA | O26:H11, O118, O157:H7, O157:H-, O55:H- | Abiotic surfaces | REC |
Hemorrhagic E. coli protein | hcpA | O157:H7 | Laminin, fibronectin | T84, Caco-2, HeLa, HEp-2, MDBK |
The lpf1 operon consists of 5 genes with functions predicted to be similar to those described for in S. Typhimurium (108,109,110). The first gene, lpfA, encodes the major fimbrial subunit protein while lpfB is a chaperone predicted to participate in proper folding. The third gene, lpfC, is the outer membrane usher protein and contains a stop codon within this predicted reading frame, dividing the gene into lpfC and lpfC’. The lpfD gene represents the coding region for a minor fimbrial subunit. Finally, the lpfE gene encodes for another predicted subunit of the fimbriae. The lpf2 operon contains a duplication of lpfD called lpfD’ and lacks the lpfE gene (110). On account of these genetic differences, predicted structural variations have been proposed and may contribute to some small differences in function between Lpf1 and Lpf2. Further, the lpf operons are not limited to EHEC, and are in fact seen in a wide range of pathogenic E. coli and even some non-pathogenic commensal strains (109). Similar to intimin, the lpfA gene has been divided into allele groups, of which 5 exist for the lpfA1 gene and 3 exist for the lpfA2 gene (112,113). Interestingly, there seems to be a correlation between the different Lpf allele group combinations and the subtype of intimin associated with a given E. coli strain which may be contributing to tissue tropism within the intestine (112).
In addition to slight differences in their genetic make-up, lpf1 and lpf2 are regulated in slightly unique ways as well. Studies indicate that transcription of lpf1 is increased during late log phase in Dulbecco’s Modified Eagle Medium (DMEM) at 37°C, pH of 6.5, and in response to salt concentrations (108). The lpf2 is positively impacted by iron depletion, which suggests that the regulatory protein Fur might participate in this activation (114). At least for the lpf1 operon, the relationship between the environment and its expression is linked to two regulatory proteins: H-NS and Ler. The H-NS and Ler relationship with the lpf1 operon was originally established using E. coli O157:H7 hns and ler mutant strains and β-galactosidase assays in which the lpf1 promoter region was fused to a reporter gene (115). The hns mutation showed an increase in β-galactosidase activity, and the ler mutant showed a corresponding decrease in activity. The regulatory control of these proteins was further established using quantitative real-time RT-PCR analysis, primer extension and electrophoretic mobility shift assays. These experiments also helped identify two σ70-type promoters in the lpf1 regulatory region and showed that both Ler and H-NS were able to bind those regions (115). Further analysis allowed for the precise definition and localization of 3 individual binding sites for H-NS (116).
Once conditions are appropriate for the expression of Lpf, these proteins, particularly Lpf1 fimbriae, display characteristics similar to those described for Lpf in Salmonella. Early studies showed that expression of Lpf1 from a plasmid containing the lpf operon and transformed into an E. coli K-12 strain increased bacterial adhesion to tissue cultured cells and also increased the number of microcolonies that were formed in vitro (108). Further, the Lpf fimbriae were visible using transmission electron microscopy and was determined that they displayed a long, fine structure. In contrast, when the lpf2 genes were cloned into this same strain of K-12, a reduction in the adherence phenotype was observed (110). Further, a mutation of the lpfA2 gene in the wild-type O157:H7 did not have a significant effect on the adhesive properties in vitro while interacting with HeLa cells. However, when Caco-2 cells were used, a reduction in adhesion was visible at early time points, suggesting that Lpf2 may contribute to early adhesion while Lpf1 may contribute to later steps in the adherence process, perhaps during the time where Ler and H-NS are also regulating the expression of the LEE-encoded proteins (110). Other studies in EHEC strain O113:H21 showed the presence of a single lpf operon located in the same chromosomal position as Lpf2. This operon contained a shortened lpfD gene (similar to REPEC O15:H-) that was non-duplicated (making it distinct from Lpf2 from EHEC O157:H7), and also lacked an lpfE gene (found in EHEC O157:H7 Lpf1) (109). When this operon was deleted from the genome of O113:H21, the bacteria were significantly less capable of adhering to CHO-K1 epithelial cells, emphasizing the fact that Lpf proteins are critical for adherence in vitro. Finally, to further define the contribution of H-NS and Ler regulation on the function of EHEC Lpf1 and its adhesion characteristics (117). HeLa cells were exposed to EHEC carrying single- or double-knockout mutations in hns and ler. It was further demonstrated that an hns mutation caused an increase in adhesion and a ler mutation resulted in a decrease. This reduction in adhesion was also observed when anti-Lpf antibodies were added during an in vitro adhesion assay with the wild-type strain. Interestingly, when both hns and ler genes were deleted, a 6-fold increase in adhesion was observed, suggesting that other positive regulators influencing Lpf1 might exist and have not yet been described.
Some progress has been made on establishing the host binding partner for Lpf1, as it was shown that wild-type EHEC is able to interact with the extracellular matrix (ECM) proteins, specifically fibronectin, laminin, and collagen IV (118). Further, anti-LpfA1 antibodies were added to these interaction assays and it was observed that binding to these proteins decreased. Deletion of lpf1 and lpf2 reduced binding to the ECM proteins, and deletions of both hns and ler genes caused a similar decrease in the binding phenotype. It was also noted that purified recombinant LpfA1 was capable of interacting directly with these ECM proteins, and when T84 cells were pre-incubated with ECM proteins and then incubated with O157:H7 wild-type strains, an increase in bacterial adherence was observed.
The first in vivo models to study the role of Lpf during infection used sheep, conventional pigs, and gnotobiotic piglets infected with the lpfA1 single or lpfA1/lpfA2 double mutant strains (119). The study sought to differentiate stages of colonization in lieu of the previously discussed results suggesting that Lpf1 may play a role early in colonization (110). The early colonization results, in which germ-free pigs were sacrificed 24 h post-infection, indicated that the lpfA1 lpfA2 double mutant strain colonized significantly less in the spiral colon, but no statistical difference was detected in the cecum. Over an extended period of time, the lpf double mutant also shed significantly less in sheep starting at 2 weeks post infection, and the lpfA1/lpfA2 double mutant was not recovered from any intestinal tissue after sacrifice, in contrast to the wild type strain which was detected at 100 CFU/g of tissue. A different study used in vitro organ cultures from human intestinal tissue and sought to better understand the effects that Lpf had on tissue tropism (120). This study indicated that the wild type strain bound only to the Follicle-Associated Epithelium (FAE) of Peyer’s patches, but was unable to interact with the duodenum, terminal ileum, or the transverse colon. However, when a knockout was introduced into either or both lpfA1 and lpfA2 genes, the strains were able to interact with all of these intestinal regions except the transverse colon. This result further emphasized the hypothesis that Lpf may be specifically driving adhesion to the FAE and working together with intimin to dictate EHEC tissue tropism. This FAE specificity is especially relevant with the understanding that O157:H7 tends to colonize areas in the intestine where FAE is found, thus providing a role for Lpf during colonization to the human intestine. A study by Fitzhenry et al., identified expression changes in 124 genes between the lpf mutants and the wild-type strain (120). The expression changes involved genes encoding some surface-exposed structures (FimG and YagZ) as well as some transcriptional regulators, which suggest that EHEC contains alternative mechanisms associated with cellular adhesion independent of tissue specificity (120). The presence of these alternative mechanisms has been suggested in another study using the rabbit infant model (18). This model uses rabbit intragastric infections, which is considered to be the most effective way to reproduce EHEC infection in vivo using an animal model. In this study, the lpfA1 lpfA2 double mutant mixed with the wild type strain was inoculated into the rabbits and it was found that the mutant was outcompeted for colonization in the ileum, cecum, and mid-colon, reinforcing the fact that Lpf was an important contributing adherence factor (121). Unexpectedly, when this double mutant was used in in vitro adhesion assays with colonic epithelial cells (Caco-2), adhesion was observed to increase when compared to wild type strain. Transmission electron microscopy indicated the presence of a novel structure that appeared to be curli, a thin afimbrial adhesin factor implicated in biofilm formation and induction of inflammation (122). When the major structural subunit of curli (CsgA) was deleted from the parent O157:H7 strain, in vitro adhesion was not significantly decreased, suggesting that curli may only be expressed under conditions in which Lpf expression was decreased or eliminated. This also suggested that there was some sort of common regulatory mechanism between these two surface structures, though changes in the transcription of csgA were not observed in the double mutant strain (123).
Curli
Curli has been recognized as an adherence-related factor that is capable of mediating interactions between EHEC and host cells (Table 1), specifically with the ECM proteins laminin and fibronectin, along with plasminogen and MHC Class 1 molecules (124,125). Curli is formed by thin fibers that aggregate on the surface of the cells and are characterized by their binding to Congo red dye (124,126). In addition to potentially acting as a compensatory mechanism for adhesion in the EHEC lpfA double mutant, curli and Lpf share a number of other characteristics. Curli and Lpf1 genes are both negatively regulated by H-NS and contribute to in vitro adhesion of tissue cultured cells (127,122). Curli is transcriptionally influenced by environmental signals, allowing curli transcription to respond to a number of different stimuli, such as pH, temperature, and nutrient limitations (128,129,130). Curli exists at the genetic level as two distinct and divergently transcribed operons: csgDEFG and csgAB (127,131). CsgD is the primary transcriptional activator of csgA, and the gene encoding this regulator is under direct control of H-NS, RpoS, Crl, MlrA, Rcs, as well as the two-component systems OmpR/EnvZ and CpxA/CpxR, IHF, and SdiA (122,132,133,134,135,136).
In addition to assuming the role of a primary adhesin of EHEC in the absence of Lpf, the primary function of curli is to mediate bacterial interaction with abiotic surfaces and protection of the pathogen from antiseptic chemicals. One study indicated that curli can directly contribute to bacterial attachment to stainless steel and promotes bacterial resistance to chlorine (137). Other studies have identified a large number of other abiotic surfaces to which curli mediates binding, including polystyrene, glass, and rubber (138). Perhaps most importantly is its role in adhesion of EHEC to vegetable leaves, making curli of particular interest in the investigation of recent outbreaks associated with contaminated spinach and lettuce. Curli-expressing strains of pathogenic E. coli have been isolated from multiple outbreaks worldwide, and a recent study indicates that CsgA is up-regulated 20-fold during interactions with lettuce leaves (139). The csgA mutant showed a decrease in adhesion to leaf tissue during both short-term and long-term colonization assays. As discussed recently by Lloyd et al., the difference in positive regulators may explain the difference in expression patterns for Lpf and curli. While Lpf is the default “on” adhesion factor and expressed under conditions mimicking the intestine, curli is strictly controlled by several regulators, possibly giving EHEC the opportunity to colonize and survive on other surfaces until more favorable environmental conditions are available (121).
Other Fimbrial Adhesin Proteins
ECP (Mat) fimbriae
In addition to EHEC Lpf1, Lpf2, and curli, a study by Low et al., was able to use genomic data analysis to predict and analyze the expression of 13 more fimbrial operons, many of which have proven to be functionally relevant (Table 1) (140). One of these operons encodes the E. coli YagZ homologue (also known as MatB) that was later re-named as an E. coli Common Pilus (ECP) (141). The isolate O18:K1:H7 is an E. coli strain associated with newborn meningitis and septicemia (MENEC) and provided the first information regarding Mat/ECP function. This fimbriae was first identified after attempting to create a strain of MENEC that was completely devoid of fimbriae, and despite a triple-knockout of known fimbriae proteins, some fimbrial structures were still present on the surface of these cells (142). The fimbriae were purified and identified, and its coding region was verified in a wide range of pathogenic E. coli strains, including EHEC, EPEC, ETEC, EAEC, and non-pathogenic E. coli K-12. Despite this, the study was only able to verify Mat/ECP expression in MENEC strains, and even then, expression was only observed at 20°C in rich media and expression involved both transcriptional and post-transcriptional events. The mat (ecp) mutant strains showed a loss of Mat/ECP on the surface of their respective strains, and these structures could be restored in trans via plasmid-expressed Mat/ECP (142). Later studies in EHEC showed that ECP is also critical for adherence to HEp-2 and HeLa cells and that this adherence phenotype can be abolished by mutating ecpA (141). The study also assayed for EcpA expression in 176 different strains of E. coli, verifying production of the fimbriae in almost 72% of strains tested, which included EHEC O157 and non-O157, as well as non-EHEC strains. In comparison with previous studies, these strains were grown at 26°C in DMEM and showed a lack of expression at 37°C and in rich media. This study also showed that ECP expression increases under low oxygen and high CO2, environmental conditions that are experienced in the intestinal tract. Further, studies in EPEC also verified this environmental dependence on the expression of ecpA (143). Purified EPEC EcpA was able to bind to and interact with the surface of HT-29 epithelial cells, reaffirming the fact that this protein is in fact an adhesin (143).
F9 fimbriae
Another fimbrial gene cluster analyzed in the study by Low et al., was the F9 fimbriae. This protein had been previously identified as a factor associated with tissue colonization when a series of 59 mutants were obtained by transposon mutagenesis in O157:H7 screened for intestinal colonization in calves (16) and in a second study using O26:H- in cattle (144). A subsequent study on this fimbriae was done by Low et al., as a follow up analysis of one of four candidate adhesins from their original screen that showed expression under in vitro conditions (145). Deletion of the z2200 gene in O157:H7 significantly reduced the pathogen’s ability to colonize the calf intestine, but it was also shown that the fimbriae was not essential for bacterial shedding or required for proper tissue tropism (145). F9 fimbriae also seem to have an affinity for ECM proteins, as it was shown to interact with fibronectin. Interestingly, when the F9 operon is transformed into an F9-deficient O157 strain, the in vitro adhesion decreased significantly (145). A later study identified CadA as a protein associated with F9 fimbriae expression when a microarray analysis indicated that the F9 fimbriae are up-regulated in a cadA mutant (146). Further, the O157 cadA mutant resulted in a hyperadherent phenotype in the ileum of an infant rabbit, implying that the F9 fimbriae may also be contributing to tissue-specific adherence during infection.
ELF and Sfp
Another set of genes analyzed by Low et al. have been associated with adhesion to host cells, but no extensive work has been done to further confirm their relationship with adherence or their functional roles (140). One of these proteins, originally identified as “locus 5,” was identified as part of the ycbQRST fimbrial-like operon and was renamed as ELF for “E. coli laminin-binding fimbriae” (147). The ELF fimbriae was purified and shown to interact with the ECM protein laminin, and its expression was verified on the surface of O157:H7 during adherence to epithelial cells (147). Like many of the previously discussed fimbriae, this protein is expressed maximally during growth in minimal media and in the presence of host cells, and mutation of the coding region resulted in a loss of adhesion to HEp-2, HT-29, and MDBK (bovine kidney) cells.
Another fimbriae described in the EHEC pathogroup is the sorbitol-fermenting fimbriae protein (Sfp) that was originally identified in an O157:H- strain from Germany and showed a high degree of similarity to the pyelonephritis-associated pili (pap) genes in uropathogenic E. coli (148). Sfp was also identified in EHEC O157:NM and O165:H25/NM strains, and shown to express poorly under nutrient rich and aerobic conditions. However, under anaerobic conditions and on media simulating that of the colonic environment, induction was increased and corresponded with an increased level of adhesion to Caco-2 and HCT-8 cells (149,150).
HCP
Other fimbriae recently described are the hemorrhagic E. coli pilus (HCP). The fimbriae, originally referred to as TFP (type IV pilus) or simply PpdD (predicted protein D), was originally identified in a study that visualized the fimbriae on the surface of O157:H7 grown in minimal media (151). This study also showed that HCP was involved in EHEC interactions with human colonic cells (T84 and Caco-2), non-intestinal cells (HeLa and HEp-2), and bovine kidney (MDBK) epithelial cells, as disruption in the hcp coding region caused a significant decrease in adhesion to these cell types. HCP was also shown to contribute to adhesion using pig and cow intestinal explants, and it was suggested that HCP interaction works in concert with other adhesins and serves as a second binding interaction on the surface of host cells (151). Later studies characterized a series of other functions, some of which are currently unique to HCP, as compared to other EHEC fimbriae, and include involvement in the invasion of host epithelial cells, hemagglutination of erythrocytes, the formation of biofilms, and binding to the ECM proteins laminin and fibronectin (152).
Type 1 fimbriae
The final fimbrial protein discussed here is the Type 1 fimbriae, which has been extensively studied as common fimbriae in E. coli; however, it has also been shown to mediate pathogenic E. coli adhesion to epithelial cells in the rumen of cattle (153). Early studies identified the type 1 fimbriae in the EHEC strains O26 and O118 (154) after having been genetically traced to other EHEC strains, including O157:H7, O157:H-, and O55- (155). Despite the presence of the coding regions in the genome of these strains, type 1 fimbriae expression was not observed in these EHEC strains. This phenomenon was attributed to the presence of a “fim switch”, a 16 bp region in the regulatory region of fimA. When absent, the switch is considered to in the “off” position and will not allow the expression of fimA, and when present, is considered to be in the “on” position and expression is allowed to proceed (155,156). The fim switch is turned on in the bovine EHEC strains O26 and O118, and studies indicate that type 1 fimbriae is a contributing factor to their virulence in the cattle infection model (156). When the fim switch from these strains is cloned into the regulatory region of fimA from O157:H7, expression is restored and the adhesion to host cells increases (156). The mutation of the fimA gene in non-O157 STEC and O128:H2 was shown to affect adherence to abiotic surfaces, such as polystyrene and glass (157).
Autotransporters
EhaA-D
In addition to the different fimbrial proteins, there is a second group of surface-exposed structures that contribute to EHEC interaction with host cells. These have the capacity to be secreted independently of the conventional cell translocation machinery and are thus referred to as autotransporters. These proteins are listed in Table 2, and generally contain a signal sequence at the N-terminus of the polypeptide, a passenger domain in the middle of the protein that determines the activity of the protein, and a C-terminal region that contains a β-barrel transmembrane region that embeds into the outer membrane and provides the passenger domain the ability to be translocated to the extracellular milieu (Reviewed in (158)). These proteins are often associated with the formation and maintenance of biofilms and other virulence traits in pathogens and related commensals (159). One study identified a series of four putative autotransporter-encoding genes, ehaA-D present in EHEC O157:H7 (160). EhaA was cloned in trans into an avirulent strain of E. coli and expressed under control of an arabinose promoter. Upon expression, EhaA was able to induce cellular aggregation as determined by fluorescence microscopy, and further, this aggregation was the product of EhaA-EhaA interactions. This protein is also capable of inducing the formation of biofilms, but because the protein is physically shorter than other surface structures, its adhesive capacity can be eliminated by the expression of type 1 fimbriae, which is considerably longer than EhaA. Further, 24 out of 50 STEC strains were tested for the presence of EhaA and showed expression of this protein under growth in minimal media, perhaps implicating a mechanism where cell-to-cell adhesion is preferred under conditions where type 1 fimbriae is not expressed (160). Another study verified a similar set of characteristics for EhaB, and expressing this protein in E. coli K-12 conferred an ability to form biofilms (161). Expression of EhaB produced an E. coli K-12 that was able to interact with the ECM proteins collagen and laminin. When the ehaB gene was disrupted in EHEC O157:H7, the mutation did not affect bacterial adhesion to primary epithelial cells derived from the bovine recto-anal junction or to Caco-2 cells. Interestingly, this protein did react to serum from infected cattle, indicating that it was expressed during infection and is likely to be exposed to the immune system (161).
Table 2
EHEC autotransporters and their functions
Autotransporter Protein | Primary function | Binding target |
---|---|---|
EhaA | Biofilms | EhaA-EhaA |
EhaB | Biofilms | Collagen, Laminin |
EspP | Protease activity, biofilms | EspP (ropes) |
Saa | Host cell adhesion | HEp-2, Caco-2 |
Sab | Host cell adhesion, biofilms | HEp-2 |
Cah | Autoaggregation, Ca+ binding, leaf binding | Alfalfa sprouts |
EspP
Another important autotransporter protein in EHEC is EspP. This protein, a homolog to EspC from EPEC, is a member of the SPATE (serine protease autotransporters of Enterobacteriaceae) family of proteins which, in addition to having adhesive capabilities, also contains protease activity (162). The initial reports indicated that this protein cleaves porcine pepsin A, human coagulation factor V (from the blood coagulation cascade pathway), apolipoprotein A-1, and further, the purified form of the protein was shown to be cytotoxic to Vero cells (163). Regarding its pathogenic association, this protein is also capable of cleaving the complement proteins C3/C3b and C5, which may help in immunomodulation, and further, is able to cleave and subsequently inactivate EHEC hemolysin, which might help in controlling and modulating the degree or timing of its own pathological effect (164,165). Mutations in espP in O157:H7 abolished adhesion in a calf model and to primary bovine intestinal epithelial cells (164). Addition of exogenous, purified EspP to the in vitro system caused the restoration of the adhesive phenotype of the espP mutant strain. A later study investigated the presence of “rope-like fibers” during growth of both EHEC and EPEC strains (167). These fibers were actually made of pure EspP that had oligomerized into fibers measuring up to 2 cm in length. The EspP-based ropes were capable of binding Congo red dye and it has been proposed that the ropes may partially function in the protection of the bacterial cells from antibiotics and detergents. Further, E. coli cells were found to interact directly with this EspP-derived rope, possibly using this protein-based structure as a foundation for biofilm-mediated interactions between bacterial cells (167). This protein was capable of interacting with cultured epithelial cells and with fibronectin, and the interaction with the cultured cells resulted in a cytopathic, but not cytotoxic, effect. Finally, antibodies against EspP were observed in patients suffering from HUS, indicating that this protein was being expressed during clinical infection and might play a role in the pathogenesis of EHEC (167).
Saa and Sab
Two other autotransporter proteins, Saa and Sab, are both plasmid-encoded adhesins that have been identified primarily in the EHEC LEE-negative O113:H21 strain. Saa was the first adhesin protein to be identified in an LEE-negative strain, and the original studies were done in vitro and showed that their expression in trans resulted in a nearly 10-fold increase in bacterial adhesion to HEp-2 cells. Conversely, mutation of the saa gene resulted in a significant reduction in this binding (168) . There are multiple variants of the saa gene based on the presence or absence of a series of repeats on the 3’ end, and it had been hypothesized that variations of this 3’ end is a mechanism for bacterial regulation of the adhesive affinity, and that shorter variants are less adhesive than longer variants (168,169). This hypothesis was explored in more depth in a study that verified the presence of the gene in 32 different STEC strains and explored whether these strains adhere to HEp-2 and Caco-2 cells (170). While there were differences in degrees of adhesion in these strains, the study could not find a correlation between repeat length and adhesive capabilities, nor was any correlation between Saa expression levels and adhesive properties statistically significant (170). Sab has not been characterized as extensively as Saa, but it has been shown to mediate adhesion to HEp-2 cells, and the mutant strains were less adhesive than wild-type O113 strain (171). Further, the study showed that the protein is present on the surface of these O113 strains, that these proteins could interact with HEp-2 cells in vitro, and that mutant strains were less capable of forming biofilms than their wild-type counterparts (171).
Cah
The final autotransporter protein that has been associated with EHEC infections is the calcium-binding antigen 43 homolog (Cah). When expressed in an E. coli K-12 strain this protein caused bacterial autoaggregation and was also able to bind to Ca+ ions in solution (172). The cah gene is duplicated in EHEC (cah1 and cah2) and their expression is maximized under low nutrient conditions, and these proteins were also shown to contribute to the formation of biofilms when grown in minimal media (172). Further studies indicated that this protein was also an important factor in EHEC adherence to alfalfa sprouts and seed coats, a feature of especially high interest when discussing persistence of EHEC in produce (173). These proteins, when provided in trans to an E. coli K-12 strain, showed increased adhesion to these two alfalfa surfaces, and it was hypothesized that this protein may contribute more to a “docking stage” of adhesion, where initial contact is made with the binding surface, than the “primary stage” of adhesion, where interactions of a higher affinity promote the long-term adhesion and colonization to a given surface (173).
Flagella
Flagella, a protein associated with bacterial motility, was first characterized as an adherence factor in pathogenic E. coli in a study that characterized the fliC gene in strains O127:H6, O119:H6, O128:H2, and O127:H40 (174). The in vitro studies found an association between flagella, adherence, and the formation of microcolonies on both HeLa cells and HEp-2 cells, and also confirmed that these proteins were responsible for the E. coli motility phenotype. Flagella were identified as an adhesin in EHEC when a fliC mutation was found to reduce the degree of virulence in a chick infection model (175). The virulence properties in the intestine are likely a product of a direct interaction with the mucus layer, as EHEC flagella has been shown to interact directly with Muc2, and the EPEC flagella has been shown to interact with Muc1 (176). Interestingly, the EPEC flagellin was capable of interacting with collagen, laminin, and fibronectin, but this interaction was not observed using EHEC flagella (174). The flagella’s role as an adhesin protein was further confirmed by a series of standard in vitro adhesion studies showing that a mutation in the fliC gene reduced bacterial adhesion to terminal rectum epithelial cells, and complementation of this mutation restored the binding phenotype (177). Further, purified flagella were capable of interacting with these cells in vitro and of interfering with bacterial binding. Finally, it was shown that the EHEC flagella are actually down-regulated after contact with the epithelium, suggesting that binding may be required only for early stages and provides a means for the T3SS to increase transcription of its components and mediate a more long-term binding event via the A/E lesion (177). Other studies have indicated that flagella are responsible, at least in part, for EHEC binding to leafy greens. Ultrastructural analysis showed the presence of flagella on the surface of EHEC during this bacteria-plant interaction, and mutation of the fliC gene negatively affect the binding (152).
Other adhesin proteins
Three final adhesins are not included in any of the fimbrial/afimbrial families described above, but their contribution to colonization is still significant to EHEC pathogenesis. Two of these, Iha and EibG, are generally regarded as adhesin proteins, though their adhesive characteristics have not been fully demonstrated as some others discussed in this review. Iha is a homolog of the Vibrio cholerae adhesive protein IrgA and is present in O157:H7, but is absent in many other strains of EHEC (178). It was originally identified during a random screen, and mutations in this gene showed decreased adhesive capabilities to HeLa and MDBK cells, and subsequently showed increases in binding when added in trans to otherwise non-piliated E. coli strain (178). The evidence for its contribution to EHEC adhesion was displayed in two studies. The first of these showed that transcription increases under growth conditions simulating short-chain fatty acid concentrations present in the human gut; however, transcription was not regulated by the presence of iron (179). The other study showed that a mutation of the EHEC iha gene resulted in a decrease in colonization in ligated pig intestine, but adherence in vitro seemed to be unaffected (180). Despite these data, evidence of Iha directly interacting with cells in vivo or in vitro has not been shown, and the presence of the protein has not been verified over the course of a natural infection, so these effects may be of an indirect nature.
E. coli immunoglobulin-binding protein (EibG) is present in 15% of eae-negative strains and exists as three different subtypes (α, β, and γ) (181,182). Transposon mutagenesis experiments identified an eibG mutation in a strain that was no longer able to form its “chain like adhesion” pattern, and this deletion also abrogated in vitro adhesion to HEp-2 cells, eliminated its ability to auto-agglutinate red blood cells and to interact with IgG and IgA (181). Later, in vitro studies using both HCT-8 cells and bovine intestinal epithelial cells indicated that the chain-like adhesion pattern varied in size according to which allele of the eibG gene was present in the genome, even within a given subtype (182).
Finally, the role of the outer membrane protein A (OmpA) in adherence was first characterized in EHEC during a transposon mutagenesis screen for a hyperadherent phenotype (183). This screen identified the transcriptional regulator TdcA as a regulator of OmpA. Further investigation led to a specific mutation of ompA, which reduced the hyperadherent phenotype in the tdcA mutant strain. Further, antibodies specific to OmpA decreased adhesion by 25% during in vitro adhesion assays with Caco-2 and HeLa cells (184). In addition to being present in EHEC, this protein is commonly present in other commensal and pathogenic strains of E. coli, but it is only known to mediate adhesion to brain microvascular cells by neonatal meningitis E. coli (NMEC) (185,186,187). Purified OmpA has been crystallized (188) and is known to stimulate dendritic cells migration through polarized epithelial cells (184).
Closing Remarks
As evidenced by the wide scope of this chapter, there are a large number of proteins within the EHEC proteome that contribute to varying degrees to its interaction with plant leaf surfaces, to cattle and human intestine, and to abiotic surfaces, such as glass and polystyrene. Figure 2 attempts to graphically depict some of these critical interactions, emphasizing some of the adhesin proteins that have been demonstrated to be important in intestinal colonization. Further, the variety of surfaces capable of interacting with the bacteria suggests that EHEC possesses a complex regulatory system to control the expression of multiple adhesins, and the temporal regulation adds another layer of complexity to understand this complex network. While some of these systems may be redundancies built into the pathogen, others likely have a very specific function that is not fully characterized. Future research will attempt to address these questions through structural and functional studies, and it seems probable that new adhesins and their host receptors will be discovered as well. Finally, as we begin to understand EHEC pathogenesis more thoroughly, adhesion models can become more refined and can begin to tease out the specific why, when, and how this hugely diverse set of proteins are mediating EHEC colonization of the intestine or persistence in the environment.
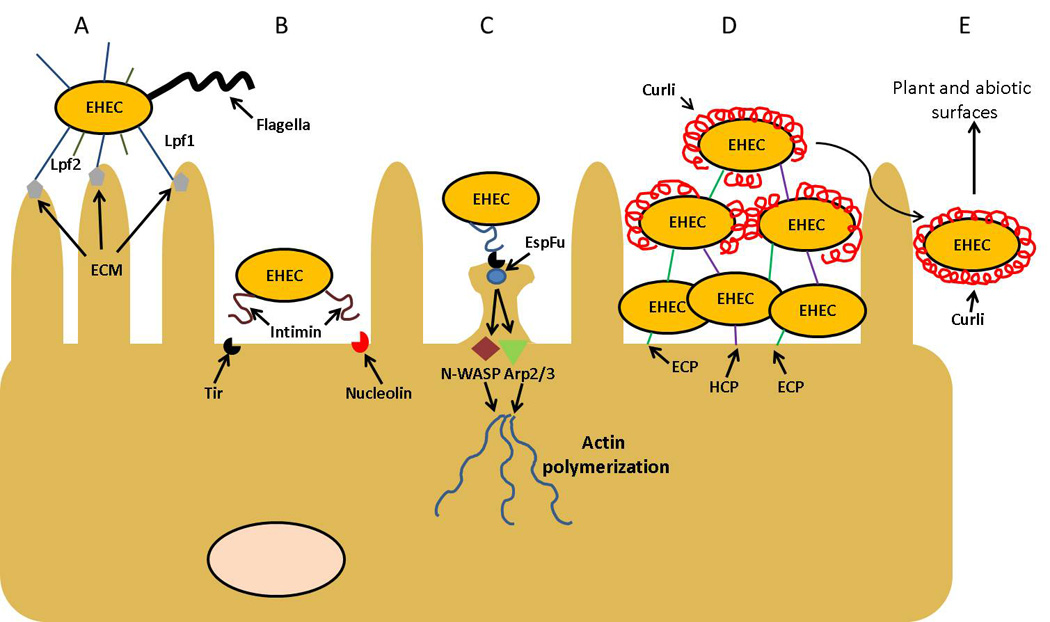
Time line and proposed roles of major fimbrial/afimbrial adhesin proteins in EHEC. In (A), Lpf is shown interacting with the extracellular membrane (ECM) proteins, such as laminin, collagen IV, and fibronectin. After the initial interaction with the intestine is established, EHEC is then able to closely attach to the host cell (B) via an interaction of the surface protein intimin with the translocated receptor protein Tir and nucleolin. In (C), the interaction between Tir and intimin is established, initiating a host-cell actin rearrangement via participation of the translocated bacterial protein EspFu and the recruitment of several host proteins. In (D), ECP and HCP have been proposed to interact with the surface of host intestinal cells, perhaps strengthening the colonization through the formation of microcolonies and/or biofilms. Further, curli has also being shown to contribute in the establishment of biolfilms, though an interaction with ECP and HCP has not been demonstrated. Finally in (E), when curli expression is dominant, it has been proposed that this expression is detrimental for the survival of EHEC in the intestine, which cause that this surface structure might become important for the pathogen interaction with abiotic surfaces and during colonization of the surface of vegetable and plant leaves.
Acknowledgments
Research work in the AGT laboratory is supported by NIH/NIAID grants AI079154 and AI09956001. The contents are solely the responsibility of the authors and do not necessarily represent the official views of the NIAID or NIH.
References
Full text links
Read article at publisher's site: https://doi.org/10.1128/microbiolspec.ehec-0003-2013
Read article for free, from open access legal sources, via Unpaywall:
http://www.asmscience.org/deliver/fulltext/microbiolspec/2/3/EHEC-0003-2013.pdf?itemId=/content/journal/microbiolspec/10.1128/microbiolspec.EHEC-0003-2013&mimeType=pdf&isFastTrackArticle=
Citations & impact
Impact metrics
Citations of article over time
Article citations
Host-Pathogen Interactions during Shiga Toxin-Producing <i>Escherichia coli</i> Adherence and Colonization in the Bovine Gut: A Comprehensive Review.
Microorganisms, 12(10):2009, 03 Oct 2024
Cited by: 0 articles | PMID: 39458318 | PMCID: PMC11509540
Review Free full text in Europe PMC
Transcriptomic and proteomic analysis of the virulence inducing effect of ciprofloxacin on enterohemorrhagic Escherichia coli.
PLoS One, 19(5):e0298746, 24 May 2024
Cited by: 0 articles | PMID: 38787890 | PMCID: PMC11125564
Application of BI-EHEC and BI-EPEC bacteriophages to control enterohemorrhagic and enteropathogenic escherichia coli on various food surfaces.
BMC Res Notes, 16(1):102, 13 Jun 2023
Cited by: 0 articles | PMID: 37312167 | PMCID: PMC10262513
Optimization of Multivalent Gold Nanoparticle Vaccines Eliciting Humoral and Cellular Immunity in an In Vivo Model of Enterohemorrhagic Escherichia coli O157:H7 Colonization.
mSphere, 7(1):e0093421, 19 Jan 2022
Cited by: 4 articles | PMID: 35044806 | PMCID: PMC8769200
Shiga Toxin-Producing Escherichia coli and Milk Fat Globules.
Microorganisms, 10(3):496, 23 Feb 2022
Cited by: 8 articles | PMID: 35336072 | PMCID: PMC8953591
Review Free full text in Europe PMC
Go to all (20) article citations
Similar Articles
To arrive at the top five similar articles we use a word-weighted algorithm to compare words from the Title and Abstract of each citation.
Enterohemorrhagic Escherichia coli Adhesins.
Microbiol Spectr, 2(3), 01 Jun 2014
Cited by: 50 articles | PMID: 26103974
Review
Expression and Functional Characterization of Various Chaperon-Usher Fimbriae, Curli Fimbriae, and Type 4 Pili of Enterohemorrhagic Escherichia coli O157:H7 Sakai.
Front Microbiol, 11:378, 20 Mar 2020
Cited by: 10 articles | PMID: 32265855 | PMCID: PMC7098969
Enterohemorrhagic Escherichia coli O157:H7 produces Tir, which is translocated to the host cell membrane but is not tyrosine phosphorylated.
Infect Immun, 67(5):2389-2398, 01 May 1999
Cited by: 150 articles | PMID: 10225900 | PMCID: PMC115983
Immunization with H7-HCP-tir-intimin significantly reduces colonization and shedding of Escherichia coli O157:H7 in goats.
PLoS One, 9(3):e91632, 14 Mar 2014
Cited by: 7 articles | PMID: 24632795 | PMCID: PMC3954762